Abstract
Objective
Focal adhesion kinase (FAK) has critical functions in proliferation and differentiation of many cell types, however, the role of FAK on BMP9-induced osteogenic differentiation in SMSCs has not been characted. The purpose of current study is to explore the mechanism of FAK on the BMP9-induced osteogenesis of SMSCs in vitro and in vivo.
Methods
The optimal dose of BMP9 was determined by incubation in different BMP9 concentrations, then cells were transfected with siRNA-induced FAK knockdown in BMP9-induced osteogenesis. Cell proliferation, migration, the osteogenic capacity, and the underlying mechanism were further detected in vitro. Imaging and pathological examination were conducted to observe the bone formation in vivo.
Results
Our findings suggested that BMP9 could obviously promote FAK phosphorylation in osteogenic conditions. In contrast, FAK knockdown significantly decreased the cell proliferation, migration, the osteogenic capacity of SMSCs. To be specific, FAK knockdown could markedly inhibit the Wnt and MAPK signal pathway of SMSCs induced by BMP9. Besides, FAK knockdown could also effectively inhibit BMP-9-induced bone formation in vivo.
Conclusion
FAK plays a pivotal role in promoting BMP9-induced osteogenesis of SMSCs, which is probably via activating Wnt and MAPK pathway.
Introduction
FAK is an intracellular non-receptor tyrosine kinase and a major mediator of signal transduction by integrins. FAK plays a significant role in the regulation of cell survival, including cell adhesion, migration, cell spreading and differentiation in mammalian cells [Citation1,Citation2]. Previous studies have suggested that FAK acts as a mechanosensor via regulating cell proliferation in response to changes in tissue compliance [Citation3,Citation4]. The role of FAK in osteogenic induction of MSCs remains obscure. Several researches have revealed that FAK contributes to cellular adhesion and spreading of BMSCs [Citation5–7]. Besides, FAK is also known as a signal adaptor, which could cross-talk with multiple signal transduction pathways in mediating cellular apoptosis, cell mobility and differentiation [Citation8–10]. A previous survey demonstrated that FAK could promote osteogenesis of ADSCs via Wnt/β-catenin signal [Citation11]. The function of FAK in osteogenic process of MSCs needs further investigation.
BMP9, known as growth differentiation factor 2 (GDF–2), is the protein that exhibits a variety of biological functions and has been widely concerned due to its induction of osteogenic differentiation [Citation12,Citation13]. However, the potential mechanisms of BMP9 used for bone regeneration still remain unclear.
SMSCs, isolated from synovial tissue, are characterized by high proliferation and multilineage differentiation potential. Our prior study has noted that SMSCs have the capacity of osteogenesis in vitro and vivo [Citation14]. Osteogenesis of MSCs requires orchestrated regulation of cellular signal transduction processes including BMP, Wnt and MAPK [Citation15,Citation16]. Smad1/5/8-dependent signaling transduction can be elicited via the combination BMPs and BMP receptor type I (BMPRI) or BMP receptor type II (BMPRII), which ultimately leads to the expression of bone markers such as ALP, Runx2, Dlx5, OCN and Osx [Citation12,Citation17]. Conversely, loss of BMP/Smad signaling pathways often leads to bone-related diseases, such as osteoporosis [Citation18]. Wnt signal also promotes bone formation through β-catenin dependent or independent signal transduction [Citation19]. Besides, MAPK signal pathway, including JNK, ERK and p38 pathways have been largely reported to promote bone formation in vitro and vivo [Citation20,Citation21]. Although there are complex signaling pathways function in the process of osteogenic differentiation, the involvement of FAK in this process remains to be elucidated.
The objectives of this study were: (i) to determine the mechanism of FAK on the BMP9-induced osteogenic differentiation of SMSCs in vitro; (ii) to test the hypothesis that the BMP9-enhanced osteogenic differentiation of SMSCs is mediated via the FAK activation in vivo.
Materials and methods
SMSCs isolation
A total of 30 male Sprague–Dawley rats of 8 weeks old were used for the experiments. All protocols for animal procedures were approved by the Ethics Committee of the Affiliated Suzhou Hospital of Nanjing Medical University. Synovium of infrapatellar fat pads was obtained from five rats. SMSCs were isolated in the enzyme digestion procedure according to the described study [Citation14]. The culture medium (DMEM/Nutrient Mixture F-12 Ham supplemented with 20% FBS (HyClone Laboratories, Inc., Logan, UT, USA)) was changed every 3 days.
Proliferation assay
The proliferation rate of SMSCs was measured using Cell Counting Kit-8 (CCK-8) assay and the Cell-LightTM EdU DNA Cell Proliferation Kit (RiboBio Co., Guangzhou, China) as previously described [Citation22]. Briefly, the SMSCs were collected and seeded into the 96-well plates at a dose of 5.0 × 103/mL. About 10 μL of CCK-8 solution (Nanjing Jiancheng Biotechnology Institute, Nanjing, China) were added into each well after cell culture from day 1 to day 7, respectively. Besides, 2.0 × 105 cells were fixed with 4% formaldehyde in phosphate-buffered saline (PBS) for 30 min after 5-ethynyl-2′-deoxyuridine (EdU) was applied to cell culture. Hoechst 33342 nucleus staining was also applied for the cells. Then, the images of randomly selected fields obtained on a DMR fluorescence microscope (Leica Microsystems, Bensheim, Germany) were analyzed. Cells were cultured at room temperature for 4 h in the dark. Then, a microplate reader (Bio-Rad, Inc., Hercules CA, USA) was used and the absorbance was measured at 450 nm.
Cell transfection and immunofluorescence
The second passage of 1.0 × 105 SMSCs was transfected with small interfering RNA (siRNA) as previously described [Citation23]. SMSCs were placed into 6-well plates for 24 h. Cells were transfected with specific siRNA (Ambion, Huntingdon, UK) targeting FAK with Lipofectamine 2000 (Invitrogen, Carlsbad, CA, USA). After transfection for 48 h, the cells were washed for immunofluorescence. Briefly, SMSCs were fixed in 4% paraformaldehyde (Beyotime Institute of Biotechnology) for 15 min. Following washing with PBS, SMSCs were permeabilized with 0.25% Triton X-100 (Sorbo Biotechnology Co., Ltd. Shanghai, China) at room temperature for another 15 min. Then, 5% BSA was used to block the cell for 30 min and primary antibodies specific to FAK (1:500, Abcam Trading (Shanghai) Co., Ltd. Shanghai, China) was used to incubate with cells overnight at 4 °C. Secondary antibody (1:2000, BD Bioscience, San Jose, CA, USA) was used for incubation for another 2 h. DAPI (Sigma-Aldrich (Shanghai) Trading Co., Ltd. Shanghai, China) was used for nuclei staining and Image J (Version 1.25, Bethesda, MD, USA) was used to determine the fluorescence intensity.
Transwell invasion and migration assay
About 2.0 × 104 cells of SMSCs were placed into a 24-well plate for transwell migration assay and the cells were cultured in 200 μl stem cell growth medium without any additional cytokines at 37 °C with 5% CO2 for 24 h. Then, the transwell chamber was removed and the cells were washed and stained with 0.1% crystal violet for 20 min. Then, cells of SMSCs were observed using a microscope. The stained cells were randomly counted in six random fields of each group.
ALP and alizarin red staining and quantification
Cells undergoing BMP9-induced osteogenic differentiation were fixed in 10% formalin for 1 h, washed with distilled water, and then the cells were stained using ALP staining Kit (Sigma-Aldrich (Shanghai) Trading Co., Ltd. Shanghai, China), and 1% alizarin red (pH 4.1) at day 7 and day 14, respectively. Cells were observed at 40× magnification on a Nikon Eclipse TE200 (Melville, NY, USA). For quantification, ALP and alizarin red was extracted from each well with 10% cetylpyridinium chloride and read at 405 nm and 562 nm (FLUOstar optima; BMG Labtech, Durham, NC, USA), respectively.
Western blot analysis
SMSCs were harvested on ice in PBS and centrifuged. Total protein was extracted using radioimmunoprecipitation assay lysis buffer (Merck-Millipore, Darmstadt, Germany) and quantified using a BCA assay kit (Beyotime Institute of Biotechnology, Suzhou, China). About 20 μg proteins were loaded onto sodium dodecyl sulfate-polyacrylamide gel electrophoresis (SDS-PAGE) and transferred onto polyvinylidene difluoride membranes (PVDF). Then, the PVDF membranes were blocked with 5% nonfat milk for 3 h at 4 °C and incubated with ALP, Runx2, p-FAK/FAK, GLUT3, OCN, OPN, Wnt1, Wnt3a, β-catenin, p-GSK3β/GSK3β, p-JNK/JNK, p-ERK1/2/ERK1/2, p-p38/p38 antibodies (1:1000; Cell Signaling Technology Inc., Beverly, MA, USA) and GAPDH antibody (1:2000, Sigma-Aldrich, USA) overnight at 4 °C. Then, the membranes were re-incubated with secondary antibodies at room temperature for 3 h. The membranes were washed with TBST three times and were visualized using an HRP chemiluminescent substrate reagent kit (Invitrogen Life Technologies, Carlsbad, CA, USA). Then, an ECL kit (Pierce; Thermo Fisher Scientific, Inc.) was used for immunodetection and densitometry was performed using image J (Version 1.25, Bethesda, MD, USA).
Experiments in vivo
Cylindrical of 3.0 mm in diameter and 5.0 mm in length of nano-Hydroxyapatite/Chitosan/Poly (lactide-co-glycolide) (HCP, provided by the Microscale Laboratory of University of Science and Technology of China) were prepared. After sterilization, HCP scaffolds were incubated overnight in fresh SMSCs-culture medium. Approximately, 1.0 × 105 SMSCs were pipetted aseptically onto HCP and cultured for 24 h. Before implantation, SMSCs were infected with optimal concentrations of Ad-BMP9 (MOI = 60) or co-infected with AdR-si-FAK. Twenty-five 8-week-old male SD rats were randomly divided into HCP group (Blank), SMSCs combined with HCP group (Control), Ad-BMP9/SMSCs combined with HCP group (Ad-BMP9), Ad-BMP9/siFAK/SMSCs combined with HCP group (Ad-BMP9/siFAK) and Ad-BMP9/siNC/SMSCs combined with HCP group (Ad-BMP9/siNC). These cells-scaffold compounds were implanted into muscle bags of lower limbs of rats.
X-ray analysis and histological evaluation
At 4 weeks post-implantation, all rats were executed and the implantation sites were retrieved for X-ray analysis (Scanco Medical AG, Bassersdorf, Switzerland) and histological evaluation staining. The grayscale value was evaluated using Image J. Retrieved tissues were decalcified using standard 10% decalcifying solution HCl (Cal-Ex; Fischer Scientific, Fairlawn, NJ, USA). Serial sections of the embedded specimens were stained with hematoxylin and eosin (H&E). Bone mass was assessed using a T-DH microscope (Nikon Imaging Japan Co., Ltd. Tokyo, Japan).
Statistical analysis
All data are expressed as the mean ± standard deviation (SD). Statistical analyses were performed on SPSS software version 19.0 (IBM SPSS, Armonk, NY, USA). Statistical differences between groups were determined via one-way ANOVA, and Tukey’s multiple comparisons test, with a significance level of p < .05.
Results
BMP9 promotes osteogenesis and FAK expression of SMSCs in vitro
To determine the optional dose of BMP9 that exhibit the best osteogenic efficiency, concentrations ranging from 10−6 to 10−4 mol/l of BMP9 were added with SMSCs. It demonstrated that 10−5 mol/l of BMP9 increased the highest expression of ALP and Runx2, exhibiting the best osteogenic capacity of SMSCs, as shown in . Besides, BMP9 also effectively promoted FAK phosphorylation of SMSCs. Hence, 10−5 mol/l of BMP9 was conducted for further study.
Figure 1. BMP9 suppresses the protein expression of ALP, Runx2 and FAK of SMSCs. (A) The protein expression of ALP, Runx2 and FAK were detected via Western blot following different concentrations of BMP9. (B) Statistical analysis showed that 10–5 mol/l of BMP9 increased the highest expression of ALP and Runx2, exhibiting the best osteogenic capacity of SMSCs. *p < .05, **p < .01, as compared with 0 mol/l of BMP9.
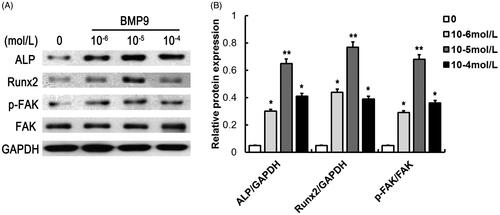
siRNA-induced knockdown suppressed FAK expression of SMSCs in vitro
To determine the transfection efficiency of SMSCs following siRNA-induced FAK knockdown, immunofluorescence and Western blot were conducted. Our findings suggested that the fluorescence intensity and the protein expression of FAK were significantly suppressed following FAK knockdown, as revealed in .
Figure 2. siRNA-induced knockdown decreases FAK expression of SMSCs. (A) Expression of FAK was observed via immunofluorescence assay following siRNA-induced knockdown. (B) Statistical analysis showed that siRNA-induced knockdown significantly inhibited fluorescence intensity of FAK. (C) siRNA-induced knockdown effectively suppressed the FAK expression following Western blot detection. **p < .01, as compared with BMP9-siFAK.
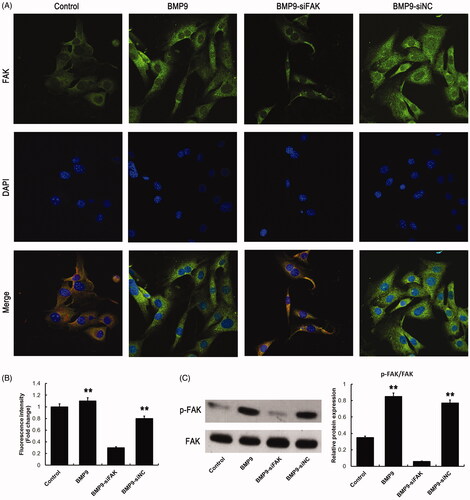
FAK knockdown inhibits BMP9-increased cell proliferation and migration of SMSCs in vitro
To observe the effects of FAK on BMP9-increased cell proliferation and migration, CCK8, Western blot, EdU/Hoechst staining and transwell assay were used. CCK8 and EdU/Hoechst staining suggested that FAK knockdown could significantly suppress cell proliferation as shown in . Western blot assay demonstrated that GLUT3 expression increased by BMP9 could obviously be down-regulated by FAK knockdown (). Transwell detection indicated that FAK knockdown significantly decreased the BMP9-increased migration of SMSCs, as illustrated in .
Figure 3. FAK knockdown inhibits BMP9-induced cell proliferation and migration of SMSCs in vitro. (A) Cell viability was markedly decreased by FAK knockdown following CCK8 assay. (B) GLUT3 expression was effectively suppressed by FAK knockdown following Western blot. (C) Cell proliferation was obviously decreased via FAK knockdown following EdU/ Hoechst staining. (D) Cell migration was significantly inhibited through FAK knockdown following transwell assay. **p < .01, as compared with BMP9-siFAK.
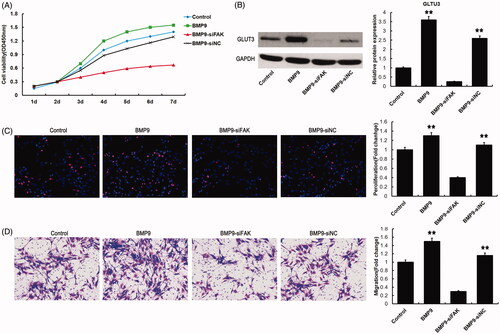
FAK knockdown suppresses BMP9-increased osteogenic differentiation in vitro
To observe the effects of FAK knockdown on BMP9-increased osteogenic differentiation, the specific staining and Western blot were conducted. Our findings suggested that BMP9 effectively increased ALP and calcium deposition of SMSCs, which could markedly be suppressed by FAK knockdown, as shown in . Western blot assay demonstrated that the BMP9-induced osteogenic specific proteins, such as ALP, Runx2, OCN and OPN could effectively be inhibited by FAK knockdown (), which revealed that FAK plays a significant role in BMP9-associated osteogenesis of SMSCs.
Figure 4. FAK knockdown decreases BMP9-induced osteogenesis of SMSCs in vitro. (A) BMP9-stimulated SMSCs were observed via ALP and alizarin red staining. (B) ALP absorption was obviously decreased via FAK knockdown. (C) Absorption of calcium deposition was effectively suppressed by FAK knockdown. (D) The protein expressions of ALP, Runx2, OCN and OPN were significantly inhibited through FAK knockdown following Western blot detection. *p < .05, **p < .01, as compared with BMP9-siFAK.
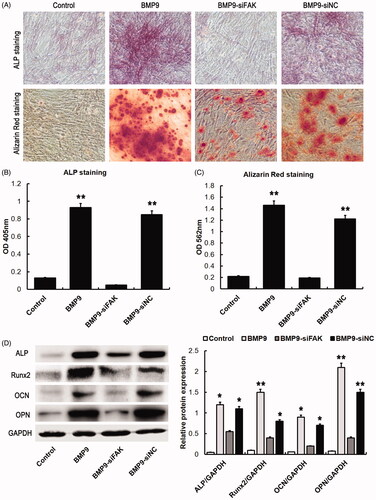
FAK knockdown inhibits BMP9-increased wnt and MAPK pathway in vitro
To determine the mechanism of FAK on the BMP9-induced osteogenic differentiation of SMSCs, Wnt and MAPK pathway were investigated. Result revealed that the BMP9-increased Wnt pathway related proteins, such as Wnt1, Wnt3a, β-catenin and p-GSK3β/GSK3β were significantly inhibited by FAK knockdown, as illustrated in . Similarly, the BMP9-promoted MAPK pathway related proteins, such as p-JNK/JNK, p-ERK1/2/ERK1/2 and p-p38/p38 were obviously suppressed via FAK knockdown, as shown in . These results suggested that Wnt and MAPK signaling pathway may participate in BMP9-induced osteogenic differentiation mediated by FAK.
Figure 5. FAK knockdown inhibits BMP9-stimulated Wnt and MAPK pathway of SMSCs in vitro. (A) The expressions of Wnt related proteins, such as Wnt1, Wnt3a, β-catenin and p-GSK3β/GSK3β were significantly inhibited through FAK knockdown following Western blot detection. (B) The expressions of MAPK related proteins, such as p-JNK/JNK, p-ERK1/2/ERK1/2 and p-p38/p38 were markedly inhibited through FAK knockdown following Western blot detection. *p < .05, **p < .01, as compared with BMP9-siFAK.
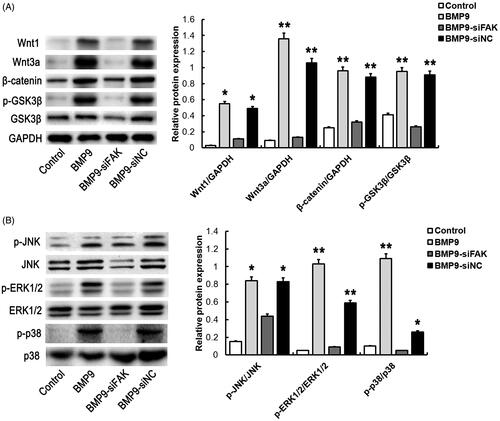
FAK knockdown decreased BMP9-increased bone mass
To verify the hypothesis that the BMP9-induced osteogenic differentiation of SMSCs could be mediated via the FAK activation in vivo, cells planted on scaffolds were transfected with Ad-BMP9 or si-FAK transfection. Four weeks post-transplantation, X-ray revealed that BMP9 exhibited the best bone formation (). HE staining showed that there were a great amount of calcification and trabecular bone could be observed after BMP9 transfected, while only a small amount of mineralized structure was found after FAK knockdown, as shown in . Both X-ray and HE staining demonstrated that FAK knockdown significantly decreased the bone mass increased by BMP9 in vivo ().
Figure 6. FAK knockdown inhibits BMP9-increased bone formation of SMSCs in vivo. (A) Cells-scaffold compound was observed via X-ray detection. (B) Statistical analysis indicated that FAK knockdown effectively suppressed BMP9-increased gray scale value. (C) Cells-scaffold compound was observed via HE staining. (D) Statistical analysis indicated that FAK knockdown markedly decreased BMP9-increased bone mass. **p < .01, as compared with Ad-BMP9/siFAK.
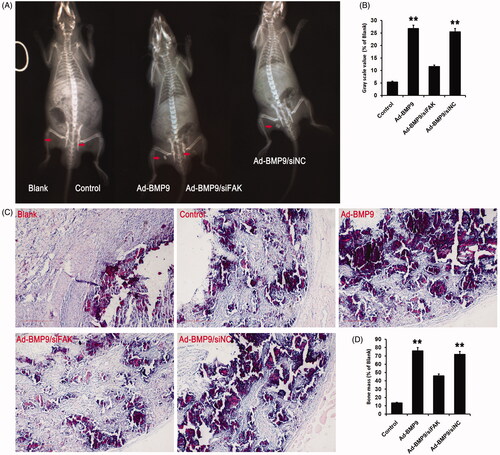
Discussion
SMSCs are characterized by high proliferation and multilineage differentiation potential. To study the osteogenic capacity of SMSCs is of great value for translational medicine. As a promising seed cell, SMSCs are progressively used to promote bone healing and formation [Citation24]. Thus, to further study the osteogenesis mechanism of SMSCs, it might be helpful to improve the osteogenic efficiency for bone regeneration.
FAK is a kind of non-receptor tyrosine kinase that can combine with integrins at focal adhesion. Recent evidence suggested that FAK could provide a binding site for Src and other molecules when FAK was activated via phosphorylation [Citation25]. Subsequently, the phosphorylated FAK could promote expression of the downstream signalling mediators, including Raf and Ras, which plays a significant role in the regulation of cell adhesion, migration, cell spreading and differentiation [Citation26]. In vitro studies have shown that FAK can promote cell mineralization in the modulation of osteogenic and odontogenic differentiation [Citation9]. In addition, Yuan et al. [Citation11] demonstrated that FAK and BMP-9 could cross-talk on Wnt signal pathway and promote ADSCs osteogenesis. The current study found that BMP9 could obviously promote the cell proliferation, migration and osteogenic differentiation of SMSCs in vitro. However, siRNA-induced FAK knockdown could significantly reverse the BMP9-increased cell proliferation, migration and osteogenic differentiation, which suggested that FAK plays a significant role in BMP9-associated osteogenesis of SMSCs.
The specific mechanism of osteoblastic MSCs differentiation is intricate and FAK-mediated osteogenic differentiation of SMSCs induced by BMP9 needs further exploration. The relationship between FAK and Wnt/β-catenin signal has been largely reported in cancer cells [Citation27,Citation28]. Hyperactivation of either FAK signaling or Wnt/β-catenin was independently demonstrated to promote numerous cell types of human cancers [Citation28,Citation29]. FAK-induced Wnt3a expression has been found in regulating frog neural plate, which could be highly conserved among vertebrates [Citation30]. Nevertheless, the intrinsic relationship between FAK and osteogenic differentiation of SMSCs has not been fully reported. In the present study, we provide evidence that FAK plays a key role in mediating osteogenic differentiation following BMP9 treatment. Our findings demonstrated that the ablation of FAK leads to a decrease in osteogenesis of SMSCs associated with decreased expression of Wnt pathway such as the decreased expression of Wnt/β-catenin–mediated transcription, GSK3 phosphorylation and β-catenin level. Hence, we propose that the down-regulated Wnt/β-catenin signaling may at least partially account for the decreased SMSCs proliferation and osteogenic differentiation.
FAK/MAPK pathway plays a critical role in signal transduction during cell migration, cell proliferation and differentiation [Citation31]. Previous literature has investigated that mechanical stimulation promotes FAK expression and activates FAK-dependent MAPK pathway through phosphorylation of JNK, ERK1/2 and p38 [Citation32,Citation33]. In the current study, we provide compelling evidence that FAK knockdown could effectively inhibit the expression of MAPK pathway such as the decreased expressions of JNK, ERK1/2 and p38 pathways, which demonstrated that the declined MAPK signaling may at least partially account for the depression of SMSCs proliferation and osteogenic differentiation.
Further experiments were conducted to test the hypothesis that whether the BMP9-induced osteogenic differentiation of SMSCs could be mediated via the FAK activation in vivo. As we know, bone tissue engineering consists of three components: scaffolds, growth factors and seed cells, among which seed cells is the key to the success of therapy of bone tissue engineering [Citation34]. A tissue engineering approach was used in the current study and this system was demonstrated to be promising for regenerating bone. In vivo mineralized tissue formation following the transplantation of SMSCs has been reported [Citation14]. Surveys revealed that both the transplanted MSCs and the recruited host stem cells play a pivotal role in bone regeneration [Citation35,Citation36]. Transplanted MSCs could secrete homing factors, which promote the host stem cells recruitment and growth factors or vascular endothelial factor accumulation [Citation37]. Besides, transplanted MSCs-induced chemokines and adhesion factors are also involved in the process of bone regeneration [Citation37]. Moreover, prior research found that the activated FAK via phosphorylation could aggregate with integrins at focal adhesion complexes in response to fluid shear stress, mechanical stimulus and cell adhesion [Citation38,Citation39]. FAK has been identified as an important mediator in signal transduction during cell proliferation, migration and differentiation of osteoblasts [Citation40]. Besides, FAK knockdown in osteoblasts leads to delayed bone healing and declined bone matrix deposition in rats [Citation41]. In the present study, the in vivo implantation of BMP9 transfected SMSCs into lower limb muscle bag resulted in the enhanced bone formation. However, siRNA-induced FAK knockdown could significantly inhibit the BMP9-increased bone formation, suggesting that FAK and BMP-9 could synergistically trigger osteogenic differentiation and bone formation of SMSCs in vivo.
In summary, our study demonstrated that FAK plays a pivotal role in mediating BMP9-induced osteogenesis of SMSCs. Further studies are still eagerly awaited to investigate the mechanism of FAK involvement in osteogenic differentiation through Wnt and MAPK pathways may provide a novel approach to improve bone formation for bone regeneration engineering.
Abbreviations | ||
FAK | = | focal adhesion kinase |
BMP9 | = | bone morphogenetic protein 9 |
SMSCs | = | synovial mesenchymal stem cells |
MAPK | = | mitogen-activated protein kinase |
JNK | = | c-Jun N-terminal kinase |
Disclosure statement
The authors declare that they have no conflict of interest.
Additional information
Funding
References
- Zhao X, Guan JL. Focal adhesion kinase and its signaling pathways in cell migration and angiogenesis. Adv Drug Deliv Rev. 2011;63:610–615.
- An L, Li W, Hu X, et al. Abundant focal adhesion kinase causes aberrant neuronal migration via its phosphorylation at Tyr925. J Mol Neurosci. 2018;64:102–110
- Lin C, Li X, Luo Q, et al. RELM-beta promotes human pulmonary artery smooth muscle cell proliferation via FAK-stimulated surviving. Exp Cell Res. 2017;351:43–50.
- Lee FY, Zhen YY, Yuen CM, et al. The mTOR-FAK mechanotransduction signaling axis for focal adhesion maturation and cell proliferation. Am J Trans Res. 2017;9:1603–1617.
- Liu L, Luo Q, Sun J, et al. Decreased nuclear stiffness via FAK-ERK1/2 signaling is necessary for osteopontin-promoted migration of bone marrow-derived mesenchymal stem cells. Exp Cell Res. 2017;355:172–181.
- Sha Y, Yang L, Lv Y. ERK1/2 and Akt phosphorylation were essential for MGF E peptide regulating cell morphology and mobility but not proangiogenic capacity of BMSCs under severe hypoxia. Cell Biochem Funct. 2018;36:155–165.
- Sun J, Zhou Y. Transforming growth factor-beta1 stimulates mesenchymal stem cell proliferation by altering cell cycle through FAK-Akt-mTOR pathway. Connect Tissue Res. 2019;60:1–12.
- Lachowski D, Cortes E, Robinson B, et al. FAK controls the mechanical activation of YAP, a transcriptional regulator required for durotaxis. FASEB J. 2018;32:1099–1107.
- Sun C, Yuan H, Wang L, et al. FAK promotes osteoblast progenitor cell proliferation and differentiation by enhancing Wnt signaling. J Bone Miner Res. 2016;31:2227–2238.
- Hu JK, Du W, Shelton SJ, et al. An FAK-YAP-mTOR signaling axis regulates stem cell-based tissue renewal in mice. Cell Stem Cell. 2017;21:91–106.e6.
- Yuan C, Gou X, Deng J, et al. FAK and BMP-9 synergistically trigger osteogenic differentiation and bone formation of adipose derived stem cells through enhancing Wnt-beta-catenin signaling. Biomed Pharmacother. 2018;105:753–757.
- Wang Y, Feng Q, Ji C, et al. RUNX3 plays an important role in mediating the BMP9-induced osteogenic differentiation of mesenchymal stem cells. Int J Mol Med. 2017;40:1991–1999.
- Yan S, Zhang R, Wu K, et al. Characterization of the essential role of bone morphogenetic protein 9 (BMP9) in osteogenic differentiation of mesenchymal stem cells (MSCs) through RNA interference. Genes Dis. 2018;5:172–184.
- Zheng W, Yang M, Wu C, et al. Experimental study on osteogenesis of synovium-derived mesenchymal stem cells in vitro and in vivo. Zhongguo Xiu fu Chong Jian Wai ke za Zhi. 2016;30:102–109.
- Pengjam Y, Madhyastha H, Madhyastha R, et al. Anthraquinone glycoside aloin induces osteogenic initiation of MC3T3-E1 cells: involvement of MAPK mediated Wnt and Bmp signaling. Biomol Ther. 2016;24:123–131.
- Yang H, Guo Y, Wang D, et al. Effect of TAK1 on osteogenic differentiation of mesenchymal stem cells by regulating BMP-2 via Wnt/beta-catenin and MAPK pathway. Organogenesis. 2018;14:36–45.
- Hyung JH, Ahn CB, Je JY. Blue mussel (Mytilus edulis) protein hydrolysate promotes mouse mesenchymal stem cell differentiation into osteoblasts through up-regulation of bone morphogenetic protein. Food Chem. 2018;242:156–161.
- Liu Y, Liu Y, Zhang R, et al. All-trans retinoic acid modulates bone morphogenic protein 9-induced osteogenesis and adipogenesis of preadipocytes through BMP/Smad and Wnt/beta-catenin signaling pathways. Int J Biochem Cell Biol. 2014;47:47–56.
- Wu L, Wei Q, Lv Y, et al. Wnt/beta-catenin pathway is involved in cadmium-induced inhibition of osteoblast differentiation of bone marrow mesenchymal stem cells. Int J Mol Sci. 2019;20:1519.
- Kim HY, Park SY, Choung SY. Enhancing effects of myricetin on the osteogenic differentiation of human periodontal ligament stem cells via BMP-2/Smad and ERK/JNK/p38 mitogen-activated protein kinase signaling pathway. Eur J Pharmacol. 2018;834:84–91.
- Bae WJ, Auh QS, Kim GT, et al. Effects of sodium tri- and hexameta-phosphate in vitro osteoblastic differentiation in periodontal ligament and osteoblasts, and in vivo bone regeneration. Differentiation. 2016;92:257–269.
- Zhou N, Hao S, Huang Z, et al. MiR-7 inhibited peripheral nerve injury repair by affecting neural stem cells migration and proliferation through cdc42. Mol Pain. 2018;14:174480691876679.
- Zhou Y, Shu C. Fibronectin promotes cervical cancer tumorigenesis through activating FAK signaling pathway. J Cell Biochem. 2019. DOI:10.1002/jcb.28282
- Diaz-Rodriguez P, Erndt-Marino JD, Gharat T, et al. Toward zonally tailored scaffolds for osteochondral differentiation of synovial mesenchymal stem cells. J Biomed Mater Res B Appl Biomater. 2018. DOI:10.1002/jbm.b.34293
- Tan D, Zhang W, Tao Y, et al. PZR promotes metastasis of colorectal cancer through increasing FAK and Src phosphorylation. Acta Biochim Biophys Sin. 2019;51:356–364.
- El Sayed I, Helmy MW, El-Abhar HS. Inhibition of SRC/FAK cue: A novel pathway for the synergistic effect of rosuvastatin on the anti-cancer effect of dasatinib in hepatocellular carcinoma. Life Sci. 2018;213:248–257.
- Kolev VN, Tam WF, Wright QG, et al. Inhibition of FAK kinase activity preferentially targets cancer stem cells. Oncotarget. 2017;8:51733–51747.
- Fonar Y, Frank D. FAK and WNT signaling: the meeting of two pathways in cancer and development. Anticancer Agents Med Chem. 2011;11:600–606.
- Golubovskaya VM. Targeting FAK in human cancer: from finding to first clinical trials. Front Biosci. 2014;19:687–706.
- Fonar Y, Gutkovich YE, Root H, et al. Focal adhesion kinase protein regulates Wnt3a gene expression to control cell fate specification in the developing neural plate. MBOC. 2011;22:2409–2421.
- Young SR, Gerard-O'Riley R, Kim JB, et al. Focal adhesion kinase is important for fluid shear stress-induced mechanotransduction in osteoblasts. J Bone Min Res. 2009;24:411–424.
- Song J, Ye B, Liu H, et al. Fak-Mapk, Hippo and Wnt signalling pathway expression and regulation in distraction osteogenesis. Cell Prolif. 2018;51:e12453.
- Ding Q, Gladson CL, Wu H, et al. Focal adhesion kinase (FAK)-related non-kinase inhibits myofibroblast differentiation through differential MAPK activation in a FAK-dependent manner. J Biol Chem. 2008;283:26839–26849.
- Nakamura S, Ito T, Okamoto K, et al. Acceleration of bone regeneration of horizontal bone defect in rats using collagen-binding basic fibroblast growth factor combined with collagen scaffolds. J Periodontol. 2019. DOI:10.1002/JPER.18-0674
- He XT, Li X, Xia Y, et al. Building capacity for macrophage modulation and stem cell recruitment in high-stiffness hydrogels for complex periodontal regeneration: experimental studies in vitro and in rats. Acta Biomater. 2019;88:162–180.
- Zhou Y, Fan W, Prasadam I, et al. Implantation of osteogenic differentiated donor mesenchymal stem cells causes recruitment of host cells. J Tissue Eng Regen Med. 2015;9:118–126.
- Shafiq M, Jung Y, Kim SH. Covalent immobilization of stem cell inducing/recruiting factor and heparin on cell-free small-diameter vascular graft for accelerated in situ tissue regeneration. J Biomed Mater Res. 2016;104:1352–1371.
- Kleinschmidt EG, Schlaepfer DD. Focal adhesion kinase signaling in unexpected places. Curr Opin Cell Biol. 2017;45:24–30.
- Matsuda S, Kawamoto K, Miyamoto K, et al. PCTK3/CDK18 regulates cell migration and adhesion by negatively modulating FAK activity. Sci Rep. 2017;7:45545.
- Rajshankar D, Wang Y, McCulloch CA. Osteogenesis requires FAK-dependent collagen synthesis by fibroblasts and osteoblasts. FASEB J. 2017;31:937–953.
- Ma XY, Feng YF, Wang TS, et al. Involvement of FAK-mediated BMP-2/Smad pathway in mediating osteoblast adhesion and differentiation on nano-HA/chitosan composite coated titanium implant under diabetic conditions. Biomater Sci. 2018;6:225–238.