Abstract
Biosynthesis of silver nanoparticles (AgNPs) from the medicinal plants has been considered as a remarkable approach of several therapeutic innovations and successful drug delivery. Silver nanoparticles were biosynthesized with Salvia miltiorrhiza, Chinese medicinal herb and assessed for its anticarcinogenic property. Synthesis of AgNPs was characterized by several studies such as UV-absorbance and it shows peak values in the range of 425–445 nm. The sizes of the nanoparticles are confirmed by dynamic light scattering analysis and it shows 100 nm. Furthermore, transmission electron microscopy (TEM) and energy dispersive X-ray analysis (EDX) was to confirm the shape and Ag particles are present in the synthesized materials. FTIR analysis to find out the active biomolecules located in the surface of the synthesized particles. This AgNPs from S. miltiorrhiza inhibits the growth of Bacillus subtillis, Staphylococcus aureus, Escherichia coli, and Klebsiella pneumonia. Furthermore, the anticancer potential of AgNPs is examined in prostate adenocarcinoma (LNCaP) cell lines. In this study, we found the AgNPs effectively induces cytotoxicity, ROS and apoptosis by modulation of intrinsic apoptoic Bcl2, Bclxl, Bax and Caspase 3 protein expressions in LNCap cell lines. Based on the study, synthesis of AgNPs from S. miltiorrhiza shows eco-friendly and it exhibits antimicrobial and anticarcinogenic effects.
Introduction
Nanotechnology is the field of materials science to develop the most active nanomaterials for the therapeutic potential of numerous diseases [Citation1]. The prepared nanoparticles exhibits several physiochemical properties and characteristics such as size, morphology and distribution [Citation2]. Crystal based nanoparticles have shown tremendous applications and it detects high sensitivity biomolecules [Citation3,Citation4]. There are numerous chemicals used to develop nanoparticles for therapeutic potential. However, those materials are highly economic and it is environmentally hazardous Therefore, uses of metal nanoparticles are considered low cost and eco-friendly in nature [Citation5,Citation6]. Metal compounds such as gold and silver have strong ameliorated activities against several microbes such as fungi, bacteria, yeast and virus [Citation7]. Silver has been recognized to inhibit microbes for medical and industrial application and it has been mainly used in the medical and pharmaceutical industry for preparing topical ointments to prevent infection against burn and wounds. Moreover, silver metal has been considered as a toxic material that can cause deleterious toxicity against several types of cancer cells [Citation1]. Synthesis and characterization of silver nanoparticles from the medicinal plant or extracts are recently shown to be the greatest approach to develop selective drug delivery for cancer treatment.
Prostate cancer (PCa) is the most common in men and is shown to be a major communal health issue worldwide, especially in the United States [Citation8]. American Cancer Society has been reported that there are 164,690 new PCa cases diagnosed and among those cases, 29,430 deaths happened in the year 2018 in the United States [Citation9]. Despite recent advances in therapeutic modalities such as chemotherapy, radiotherapy and radical prostatectomy, which can control localized PCa, it will not work on metastatic PCas [Citation10]. Moreover, uses of radiotherapy and standard chemotherapeutic drugs produce pronounced and significant undesirable side effects on the patients [Citation11]. Hence, novel strategies are required for the treatment of PCa. Therefore, plant-mediated synthesis of nanoparticles have the greatest ideal approach for targeted delivery on cancer cells and prevent the undesirable side effects.
Medicinal plants are one of the most commonly used alternative therapies. There are several medicinal plants that have been used besides conventional treatment regimens [Citation12]. The preparation of nanoparticles from medicinal herbs or plant extracts are currently attracting attention as a promising chemopreventive strategy in several kinds of cancer [Citation13,Citation14]. Salvia miltiorrhiza Bunge otherwise called as Danshen herbal is the most well-known Chinese conventional herbs and it has more than 1000 years of clinical application [Citation15]. Tanshinones is the major lipid-soluble component present in S. miltiorrhiza and also having a group of abietane diterpenes. Salvia miltiorrhiza exhibits several beneficial and pharmacological activities such as cardioprotective, antioxidants, antiinflammation, antidiabetic and anticarcinogenic [Citation16]. It has been previously reported that common hemorrheologic agent promotes blood circulation and regulates blood stagnation in humans [Citation17–19]. Previous reports also implicated that S. miltiorrhiza extracts and its active components inhibit the growth of cancer cells including breast, prostate and lung cancer [Citation20–22].
Hence, in the current study, non-toxic, eco-friendly silver nanoparticles (AgNPs) were green synthesized from Chinese medicinal herb S. miltiorrhiza, characterized using various techniques and evaluated its anticarcinogenic potency against PCa cells.
Materials and methods
Chemicals, antibodies and solvents
Cell culture medium DMEM, FBS and trypsin-EDTA were purchased from Himedia, Mumbai, India. Bcl-xl, Bax, Bcl-2, caspase-3and β-actin, goat anti-mouse IgG-HRP polyclonal antibody were procured from Santacruz, CA, USA. MTT, DCFH-DA stains acridine orange and propidium iodide were obtained from Sigma Chemical Co., MO, USA. All the other fine chemicals and solvents used for the present study were of analytical grade.
Silver nanoparticles (AgNPs) synthesis and purification
Salvia miltiorrhiza leaves were used to make the aqueous extract. Fresh S. miltiorrhiza leaves weighed 25 g and washed with double distilled water. After that, it is allowed for drying and crushed into 100 m + l sterile distilled water and it was filtered by Whatman No.1 filter paper (25 μm). Silver nitrate (1 mM AgNO3) was prepared and used for the synthesis of silver nanoparticles. 10 ml of S. miltiorrhiza extract was added into 90 ml of aqueous solution of 1 mM silver nitrate for reduction into Ag + ions and kept at room temperature for 5 h.
Characterization of AgNPs synthesized from Salvia miltiorrhiza
The synthesis of AgNPs was confirmed with ultraviolet-visible spectroscopic analysis between the absorbance 300–700 nm (Shimadzu UV spectrophotomete (Shimadzu, Japan)). The size and shape of the nanoparticles were analyzed with transmission electron microscopic analysis and energy dispersive X-ray analysis. The active compounds present in nanoparticles were analyzed by subjecting the nanoparticles to FTIR analysis at the of 000 and 1000 cm−1. The surface morphology of the synthesized silver nanoparticles was assessed with dynamic light scattering and X-ray diffraction.
Antibacterial assays
The antibacterial activity assays were analyzed by using pathogens such as Bacillus subtilis, Staphylococcus aureus, Escherichia coli and Klebsiella pneumonia by the method of standard disc diffusion method. In brief, Luria Bertani (LB) broth/agar medium was used to culture the above-mentioned bacteria. Overnight cultures of inoculum (100 μl) of each culture were spread on to the LB agar plates. Sterile paper discs of 5 mm diameter (containing 50 µg/ml of AgNPs) along with four standard antibiotic containing discs were used in each plate.
Cell culture
Androgen-sensitive human prostate adenocarcinoma cells LNcap cells were procured from National Center for Cell Sciences, Pune. Cells were cultured with DMEM medium supplemented with 10% fetal bovine serum and 1% antibiotics at 37 °C in a humidified incubator at 5% CO2. The medium was changed once in four days or when yellow colour change was observed. The cells were subcultured for further analysis using 1 ml of trypsin-EDTA solution once 80% of confluency was reached by Balupillai et al. 2018 [Citation23].
Cell viability assay
The viability of LNcap cells at different concentration of synthesized silver nanoparticles treatment were assessed using MTT assay. LNCap cells were treated with different concentration of AgNP particles from S. miltiorrhiza ranging from 5 to 100 µg/ml for 24 h at 37 °C and 5% CO2. The cells were then treated with MTT reagent for 3 h and the formazon crystals formed were dissolved with DMSO. The colour intensity developed were measured at 570 nm in micro plate reader, the colour developed is inversely proportional to the percentage of dead cells.
Intracellular ROS measurement
Reactive oxygen species plays a key role in both apoptosis and cancer cell development measuring the levels of intracellular ROS levels indicate the anticarcinogenic effect induced by silver nanoparticles. Intracellular ROS levels were measured using the fluorescent probe, 2,7-diacetyl dichlorofluorescein (DCFH-DA) staining [Citation24]. LNcap cells were treated with high and low dose (25 and 50 µg/ml, respectively) of silver nanoparticles and incubated for 24 h at 37 °C and 5%CO2. The cells were then stained with 1 μl of DCFH-DA (1 mg/mL) and the fluorescent intensity was measured by spectroflourimeter with excitation and emission at 485 ± 10 and 530 ± 12.5 nm, respectively.
Fluorescent terminal deoxynucleotidyl transferase (TdT)-meditated dUTP-fluorescein nick end-labeling (TUNEL)
The apoptosis induction of AgNPs can be assessed by measuring nuclear fragmentation in the silver nanoparticle treated cells using TUNEL assay kit (Sigma, MO, USA). The procedure was followed as per the instruction of product’s manual. LNcap cells were treated with 25 and 50 µg/ml and incubated for 24 h after the incubation periods. The cell was fixed with 2% paraformaldehyde for 30 min. The fixed cells were permeabilized with 0.1% Triton X-100 for 30 min and TUNEL reaction buffer was added, incubated at 37 °C for 1 h in the dark. After incubation, the cells were washed with ice cold phosphate buffered saline twice and then stained with DAPI. The stained images were viewed and photographed using a fluorescence microscope (Nikon, Japan), the images were assessed using ImageJ software, USA.
Apoptotic studies for acridine orange/propidium iodide
Dual staining with acridine orange/propidium iodide will clearly indicate the apoptotic induction by the silver nanoparticle treatment. AO stains both live and dead cells whereas PI stains only the dead cells, therefore, we stained the silver nanoparticle treated cells with 20 µL of AO/PI staining (10 µL/mg AO and 10 µL/mg PI) solution at 37 °C for 15 min. The stained cells were then stained. Images were viewed and photographed using a fluorescence microscope (Nikon), the images were assessed using ImageJ software.
Caspase-8, 9 and 3 activity assay
Caspases are proteolytic enzymes which plays a key role in executing apoptosis, therefore, assessing the levels of caspases in control and silver nanoparticle treated cells will confirm the induction of apoptosis by silver nanoparticles. The caspases activity were assessed with the caspases 8, 9 and 3 assay kit procured form Invitrogen, USA. LNcap cells were treated with high and low dosage of silver nanoparticles and incubated at 37°C for 24 h. The control and treated cells were then subjected to estimation of caspases as per manufacturer’s guidelines. The colour intensity developed were measured using a microplate reader at 405 nm.
Western blot analysis
Apoptotic proteins Bax, Bcl-xl, Bcl-2 and caspase-3 expression in control and silver nanoparticle treated LNcap cells were assessed using Western blotting [Citation25]. The cells were treated with 25 and 50 µg/ml of synthesized silver nanoparticles and incubated at 37°C, 5% CO2 for 24 h. After the incubation period, the cells were harvested using RIPA buffer and subjected to sonication for 20 s. The sonicated cells were centrifuged at 12,000 rpm for 15 min at 4°C. Supernatant was collected and subjected to protein estimation with nanodrop instrument (Thermo Scientific, Waltham, USA). About 40 µg protein from each sample were electrophoresed in 10% SDS-PAGE for 1 h at 100 mV. The electrophoresed protein were then transferred to PVDF membrane. The PVDF membrane was further blocked with 5% blocking buffer to prevent non-specific binding of proteins. The membranes were then washed with tris-buffered saline and incubated with primary monoclonal antibodies Bax, Bcl-xl, Bcl-2 and caspase-3 overnight at 4°C. After overnight incubation, the membranes were treated with appropriated secondary HRP conjugated antibodies and the chemiluminescence was assessed using ECL kit, Promega, USA. The intensity of specific proteins were assessed using ImageJ software.
Statistical analysis
The results were statistically analyzed with one-way ANOVA followed by post hoc test SNK using Graph Pad prism softwar (San Diego, CA). p < .05 Were considered to be statistically significant and the results were expressed as the mean ± standard deviation.
Results and discussion
Synthesis nanomaterials by the method of green biosynthesis have a peculiar tendency in the current research era. Based on this method, mostly metal and semiconductor nanoparticles can be developed using different natural resources such as medicinal plants, microorganisms and fruit extracts for minimizing stabilizing agents [Citation26,Citation27]. Terpenoids present in the leaf extract of S. miltiorrhiza act as reducing agents thereby initiated the synthesis of silver nanoparticles. These kinds of nanosynthesis cost low and eco-friendly in nature and it has several pharmaceutical and biomedical applications [Citation28,Citation29]. In this current study, we have demonstrated that to prepare silver nanoparticles from S. miltiorrhiza, it was characterized by UV-visible absorbance spectrum, high resolution transmission electron microscope (HR-TEM), dynamic light scattering analysis (DLS), energy dispersive X-ray analysis (EDX) and FTIR analysis. Moreover, synthesized AgNPs exploited the anticancer potential studies by apoptotic regulation in PCa cells (LNCap).
Characterization of silver nanoparticles (AgNPs) synthesized from Salvia miltiorrhiza
The synthesis of AgNPs from S. miltiorrhiza was initially characterized by the color conversion from light yellow to dark brown (. The changes of color indicated excitation of surface plasmon resonance synthesized AgNPs. GC-MS analysis of S. miltiorrhiza leaves reports sesquiterpenoids are the major constituent of S. miltiorrhiza leaves ranging about 33.1%, among which 23.5% were hydrocarbon sesquiterpenes [Citation30]. As the S. miltiorrhiza extract was mixed with the aqueous solution of the silver ion complex, it initiated to convert the color from watery to yellowish brown due to the reduction of silver ion (); which leads to the formation of silver nanoparticles. This information was already proved by several lines of studies on silver nanoparticles synthesis [Citation31]. Furthermore, we confirmed the S. miltiorrhiza mediated synthesized AgNPs by UV visible absorbance. An increasing concentration of S. miltiorrhiza extracts that leads to increase in the intensity of absorption. The UV-visible spectra recorded after different time intervals such as of 24 h, 48 h, 72 h and 92 h from the initiation of reaction with different amount of plant extracts. It is generally identifying that UV-Vis spectroscopy might be used to observe the size and shape-controlled nanomaterials present in the aqueous suspensions [Citation32]. In this study, synthesized AgNPs exhibits maximum absorbance, which was found to be in the ranges of 435 nm at 96 h prepared solution. The highest absorbance bands occurred at different concentration of AgNPs solution at different time points (). The metal nanoparticles, particularly AgNPs, have free electrons which donate SPR absorption bands due to the combined vibration of electrons of metal nanoparticles in resonance with lightwave [Citation33].
Figure 1. (A) The synthesis of AgNPs from aqueous leaf extract of Salvia miltiorrhiz was confirmed by changes in solution color from light yellow to dark brown. (B). UV-visible absorption spectrum of synthesized AgNPs.

To analyze the size, distributions of synthesized silver nanoparticles from S. miltiorrhiza extract by dynamic light scattering (DLS) method. In this histogram, image indicated small size of the particle and size of the Ag nanoparticles from S. miltiorrhiza is 128 nm (. Moreover, there was no particle agglomeration observed. This data provides accuracy of the particle size and distribution. Furthermore, size and morphological characteristics of AgNPs synthesized from S. miltiorrhiza was studied by HR-TEM analysis (). The HR-TEM image shows that the physical structure of AgNPs which are observed in spherical, oval, hexagonal and triangular with average sizes between 80 and 12 nm. This data also supported and statistically correlated with DLS images for AgNPs synthesized from S. miltiorrhiza. The TEM data provides high-density silver nanoparticles synthesized by the S. miltiorrhiza extract, which further confirmed the development of silver nanostructures. Moreover, EDX studies were analyzed to evaluate the shape of the particles synthesized from S. miltiorrhiza extract silver metal (). In this study, strong signals were observed in the silver region and it confirmed the synthesis of AgNPs. We also observed the strong signals of Ag (1.4–2 and 2.5–3 keV) at characteristic energy were observed. The typical optical absorption for metallic crystal generally found to be 3 KeV due to surface plasmon resonance [Citation34]. XRD analysis of biosynthesized AgNP’s showed three distinct diffraction peaks at the plane of (111), (200) and (220) which are the characteristic peaks due to the crystalline nature of silver nanoparticles (.
Figure 2. Dynamic light scattering (DLS) images of silver nanoparticles synthesized from Salvia miltiorrhiza and the size of the nanoparticles is 128 nm.
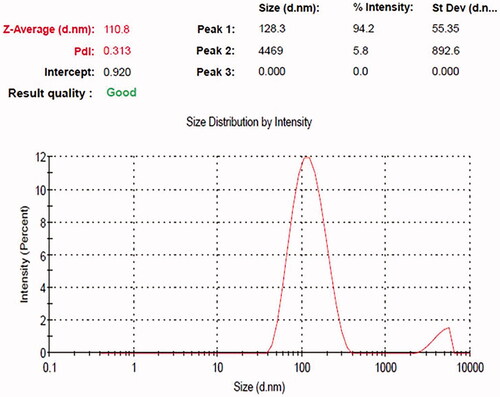
Figure 3. (A) Transmission electron microscopy (TEM) and (B) energy dispersive X-ray (EDX) and analysis silver nanoparticles synthesized from Salvia miltiorrhiza.
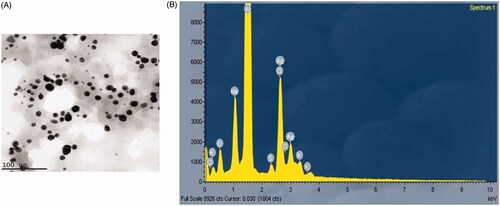
FTIR spectra are used to identify the several functional groups present in the synthesis of Ag nanoparticles from Salvia miltiorrhiz extract by observing different spectrum values. FTIR data showed that 3870 cm−1, 3563 cm−1, 3482 cm−1, 3084 cm−1, 3014 cm−1, 2725 cm−1, 2376 cm−1, 2306 cm−1, 1709 cm−1, 1619 cm−1, 1359 cm−1, 1210 cm−1, 1090 cm−1 and 753 cm−1 which indicates the carboxyl, aromatic, hydroxyl, amide and alkyl groups are present on the surface of AgNPs (). Previously, the synthesis of silver nanoparticles from different plant extracts also presented in the several functional groups [Citation28,Citation29].
Antimicrobial activity of AgNPs synthesized from Salvia miltiorrhiza extract
Salvia miltiorrhiza synthesized AgNPs was tested against MDR bacterial pathogens by disc diffusion method. It displayed greater growth inhibitory activity against all tested bacterial strains and the zone of inhibition was significantly varied according to the concentrations used. Among the tested bacterial pathogens, the S. pyogenes was highly inhibited with 10.8 ± 0.51 mm of inhibitory zone at 60 µg concentration. It was followed by S. flexneri and S. typhi with 10.5 ± 0.36 mm and 10.2 ± 0.11 mm zone of inhibition, respectively. The least activity was recorded against P. aeruginosa with 9.24 ± 0.48 mm zone of inhibition at 60 µg. The standard antibiotic ciprofloxacin (µl) was used as a positive control and exhibited greater antibacterial activity (ranged from 25 to 30 mm) than S. miltiorrhiza synthesized AgNPs () (). Previously, silver nanoparticles from papaya extracts also exhibited antibacterial activity against E. coli and P. aeruginosa [Citation35].
Table 1. Antimicrobial activity of AgNPs from salvia miltiorrhiza.
Anticancer potential of AgNPs from Salvia miltiorrhiza prostate cancer cell lines (LNcap)
The green synthesized AgNPs were tested for their anticancer activity against PCa LNcap cell lines. Therefore, the anticancer potential of AgNPs was studied by MTT based cytotoxicity assay. In this cytotoxicity assay, increasing concentration of AgNPs significantly inhibits the growth of the LNcap cells for 24 h incubation (). We found that 50 µg/ml concentrations of AgNPs exhibits pronounced cell death in LNcap cell lines. Therefore, we have chosen 50 µg/ml concentrations of AgNPs for further apoptotic studies. Green synthesized silver nanoparticles from several plant extracts are showing the remarkable anticancer activity of numerous cancer cells and also these nanoparticles are less toxic in nature [Citation36]. Overproduction of reactive oxygen species (ROS) in our system induces oxidative stress, which resulted in apoptosis [Citation24]. Hence, AgNPs mediated ROS production was analyzed by DCFH-DA fluorescent assay. In this study, we found that AgNPs synthesized from S. miltiorrhiza induces ROS generation in prostate LNcap cancer cell lines (). Overproduction ROS leads to cell organelles damage, i.e mitochondrial depolarization. Nuclear fragmentation resulted in oxidative stress-mediated apoptosis [Citation23,Citation24].
Figure 7. (A) Cytotoxic potential of AgNPs from Salvia miltiorrhiza in prostate cancer LNcap cell. (B). Effect of AgNPs from Salvia miltiorrhiza mediated ROS measurements in LNcap cells.
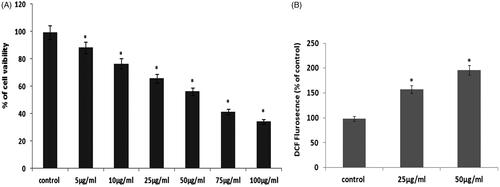
Programmed cell death called as apoptosis plays a pivotal role in cancer, since pro- and antiapoptotic proteins are concurrently activated in tumor expansion and progression [Citation37]. Therefore, apoptosis is considered as an important pathway for the treatment of cancer. AgNPs synthesized from S. miltiorrhiza induces ROS mediated apoptosis, which was analyzed by AO/PI dual staining. In this study, control cells showed acridine orange-stained green fluorescent that indicates viable cells. However, treatment with AgNPs synthesized from S. miltiorrhiza showed increased apoptotic cells by observing PI stained red fluorescent cells (). Nuclear fragmentation has been considered as a hallmark event to confirm cells going apoptosis. To assess the AgNPs synthesized from S. miltiorrhiza mediated nuclear fragmentation studied by TUNEL assay, LNcap cells were exposed with 50 µg/ml of AgNPs for 24 h. We observed the increased number of TUNEL-positive cells. The results implicated that AgNPs induces fragmentation of DNA which leads to apoptosis in PCa cells. Previously, AgNPs can stimulate apoptosis in human ovarian and triple-negative breast cancer cell lines [Citation38–40].
Figure 8. (A) AgNPs mediated apoptotic morphological studied by AO/PI staining. (B) AgNPs mediated nuclear fragmentation in LNcap cells studied by TUNEL assay. (C) DAPI staining analysis.
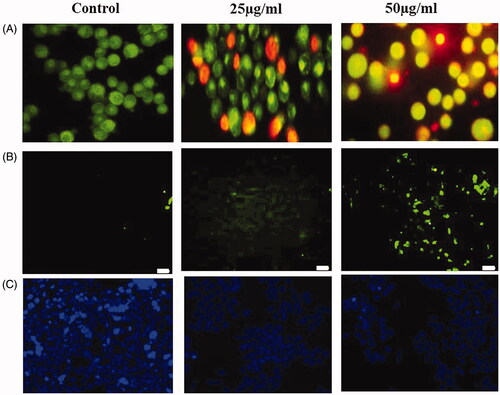
Apoptosis is the hallmark event deregulated in all the cancer conditions, therefore, in the present study assessed the potency of AgNPs on apoptosis regulating protein such as Bax, Bcl-2, Bcl-xl, Caspase-8, 9 & 3. As shown in , treatment with AgNPs synthesized from S. miltiorrhiza downregulated the expression of antiapoptotic proteins Bcl-2, Bcl-xl expression whereas the apoptotic protein Bax expression was increased significantly. The expression of executor caspases, caspase -3 was significantly increased in PCa cells (LNcap) treated with biosynthesized AgNPs compared to the control LNcap, which confirms the induction apoptosis by silver nanoparticles. The levels of caspases 8 and 9 assessed with ELISA kits were also correlated with the results of caspases protein expression observed with immunoblotting analysis. These reports are also closely related to our results obtained during acridine orange/PI staining and TUNEL assay increased the number of apoptotic cells in a dose-dependent manner, which were observed in silver nanoparticle treated LNcap cells compared to control cells. Based on these studies, AgNPs synthesized from S. miltiorrhiza effectively induces apoptosis by regulating the intrinsic apoptotic pathway.
Conclusion
In conclusion, the reduction of the Ag + ions through aqueous leaf extract of the S. miltiorrhiza leading to the formation of silver nanoparticles was demonstrated. The physiochemical characteristics of synthesized AgNPs from S. miltiorrhiza confirmed UV-absorbance, DLS, TEM, EDX and FTIR. These AgNPs from S. miltiorrhiza inhibits the growth of B. subtillis, S. aureus, E. coli and K. pneumonia. Furthermore, AgNPs inhibit cancer cell growth and it induces prostate adenocarcinoma (LNCaP) cell lines. AgNPs effectively induces cytotoxicity, ROS and apoptosis by modulation of intrinsic apoptotic gene expressions in LNCap cell lines. Based on the study, synthesis of AgNPs from S. miltiorrhiza shows it is eco-friendly and it exhibits antimicrobial and anticarcinogenic effects.
Disclosure statement
All the authors declared that there are no conflicts of interest.
References
- Bindhani BK, Panigrahi AK. Biosynthesis and characterization of silver nanoparticles (SNPs) by using leaf extracts of Ocimum Sanctum L (Tulsi) and study of its antibacterial activities. Nanomed Nanotechnol. 2015 Nov 1(S6):1, 6–10.
- Appenzeller T. The man who dared to think small. Science. 1991;254:1300–1301.
- Murray CB, Norris DJ, Bawendi MG. Synthesis and characterization of nearly monodisperse CdE (E = sulfur, selenium, tellurium) semiconductor nanocrystallites. J Am Chem Soc. 1993;115:8706–8715.
- Park J, Joo J, Kwon SG, et al. Synthesis of monodisperse spherical nanocrystals. Angew Chem Int Ed. 2007;46:4630–4660.
- Raveendran P, Fu J, Wallen SL. Am Chem Soc. 2003;125:13940–13941.
- Sharma HS, Ali SF, Hussain SM, et al. Nanosci Nanotechnol. 2009;9:5055–5072.
- Sahayaraj K, Rajesh S. Bionanoparticles: synthesis and antimicrobial applications. Vol. 23. In: Méndez-Vilas A, editor. Science against microbial pathogens: communicating current research and technological advances. Spain: Formatex Research Center; 2011. p. 228–244.
- Quinn M, Babb P. Patterns and trends in prostate cancer incidence, survival, prevalence and mortality: Part I. International comparisons. BJU Int. 2002;90:162–173.
- Centers for Disease Control and Prevention. Prostate cancer; 2014. Available from: http://www.cdc.gov/cancer/prostate/
- De Bono JS, Oudard S, Ozguroglu M, et al. Prednisone plus cabazitaxel or mitoxantrone for metastatic castration-resistant prostate cancer progressing after docetaxel treatment: a randomised open-label trial. Lancet. 2010;376:1147–1154.
- Scher HI, Fizazi K, Saad F, et al. Increased survival with enzalutamide in prostate cancer after chemotherapy. N Engl J Med. 2012;367:1187–1197.
- Calixto JB. Twenty-five years of research on medicinal plants in Latin America: a personal view. J Ethnopharmacol. 2005;100:131–134.
- Popescu M, Velea A, Lorinczi A. Biogenic production of nanoparticles. Dig J Nanomater Bios. 2010;5:1035–1040.
- Baruwati B, Polshettiwar V, Varma RS. Glutathione promoted expeditious green synthesis of silver nanoparticles in water using microwaves. Green Chem. 2009;11:926–930.
- Wang ZJ, Cui LJ, Chen CX, et al. Down regulation of cinnamoyl CoA reductase affects lignin and phenolic acids biosynthesis in Salvia miltiorrhiza Bunge plant. Plant Mol Biol Rep. 2012;30:1229–1236.
- Chen X, Guo J, Bao J, et al. The anticancer properties of Salvia Miltiorrhiza Bunge (Danshen): a systematic review. Med Res Rev. 2014;34:768–794.
- La Sala L, Cattaneo M, De Nigris V, et al. Oscillating glucose induces microRNA-185 and impairs an efficient antioxidant response in human endothelial cells. Cardiovasc Diabetol. 2016;15:71.
- Zhang X, Liu W, Wu S, et al. Calcium dobesilate for diabetic retinopathy: a systematic review and meta-analysis. Sci China Life Sci. 2015;58:101–107.
- Tao MF, WD Y. Effects of Xuefu-Zhuyu oral liquid on the auricles microcirculation in mice. Chin J Clin Pharmacol Therapeut. 2003;(1):89–91.
- Gong Y, Li Y, Lu Y, et al. Bioactive tanshinones in Salvia miltiorrhiza inhibit the growth of prostate cancer cells in vitro and in mice. Int J Cancer. 2011;129:1042–1052.
- Tung YT, Chen HL, Lee CY, et al. Active component of danshen (Salvia miltiorrhiza bunge), tanshinone I, attenuates lung tumorigenesis via inhibitions of VEGF, cyclin A, and cyclin B expressions. Evid Based Complementary Altern Med. 2013;2013:1–10.
- Wang BQ. Salvia miltiorrhiza chemical and pharmacological review of a medicinal plant. J Med Plant Res. 2010;25:2813–2820.
- Balupillai A, Nagarajan RP, Ramasamy K, et al. Caffeic acid prevents UVB radiation induced photocarcinogenesis through regulation of PTEN signaling in human dermal fibroblasts and mouse skin. Toxicol Appl Pharmacol.2018;352:87–96.
- Gunaseelan S, Balupillai A, Govindasamy K, et al. Linalool prevents oxidative stress activated protein kinases in single UVB-exposed human skin cells. PLoS One. 2017;12:e0176699.
- Agilan B, Rajendra Prasad N, Kanimozhi G, et al. Caffeic acid inhibits chronic UVB-induced cellular proliferation through JAK-STAT3 signaling in mouse skin. Photochem Photobiol. 2016;92:467–474.
- Saif S, Tahir A, Chen Y. Green synthesis of iron nanoparticles and their environmental applications and implications. Nanomaterials. 2016;6:209.
- Makarov VV, Love AJ, Sinitsyna OV, et al. Green Nanotechnologies: Synthesis of Metal Nanoparticles Using Plants. Acta Naturae 2014;6:35–44.
- Rizvi SAA, Saleh AM. Applications of nanoparticle systems in drug delivery technology. SPJ. 2018;26:64–70.
- Sarvamangala D. Kondala K, Murthy USN, Narasinga Rao B, Sivakumar N. Green synthesis of AgNPs using Alternanthera Sessilis leaf extract [A natural source for ocular therapy]. Int J Innov Res Sci Eng Technol. 2014;3:15000–15010.
- Li X, Wang Z. Chemical composition, antimicrobial and antioxidant activities of the essential oil in leaves of Salvia miltiorrhiza Bunge. J Essent Oil Res. 2009;21:476–480.
- Ponarulselvam S, Panneerselvam C, Murugan K, et al. Synthesis of silver nanoparticles using leaves of Catharanthus roseus Linn. G. Don and their antiplasmodial activities. Asian Pac J Trop Biomed. 2012;2:574–580.
- Zhang XF, Liu ZG, Shen W, et al. Silver nanoparticles: synthesis, characterization, properties, applications, and therapeutic approaches. IJMS. 2016;17:1534.
- Jayaram UC, Annadurai G. Biosynthesis and characterization of silver nanoparticles using leaf extract Abutilon indicum. Global J Biotechnol Biochem. 2018;13:7–11.
- Kaviya S, Santhanalakshmi J, Viswanathan B, et al. Biosynthesis of silver nanoparticles using Citrus sinensis peel extract and its antibacterial activity. Spectrochim Acta A Mol Biomol Spectrosc. 2011;79:594–598.
- Jain D, Daima HK, Kachhwaha S, et al. Synthesis of plant-mediated silver nanoparticles using papaya fruit extract and evaluation of their anti microbial activities. Dig J Nanomater Bios. 2009;4:557–563.
- Kummara S, Patil MB, Uriah T. Synthesis, characterization, biocompatible and anticancer activity of green and chemically synthesized silver nanoparticles – a comparative study. Biomed Pharmacother. 2016;84:10–21.
- Gunaseelan S, Balupillai A, Govindasamy K, et al. The preventive effect of linalool on acute and chronic UVB-mediated skin carcinogenesis in Swiss albino mice. Photochem Photobiol Sci. 2016;15:851–860.
- Han JW, Gurunathan S, Jeong JK, et al. Oxidative stress mediated cytotoxicity of biologically synthesized silver nanoparticles in human lung epithelial adenocarcinoma cell line. Nanoscale Res Lett. 2014;9:459.
- Gurunathan S, Han JW, Eppakayala V, et al. Cytotoxicity of biologically synthesized silver nanoparticles in MDA-MB-231 human breast cancer cells. Biomed Res Int. 2013;2013:1.
- Gurunathan S, Han JW, Park JH, et al. Reduced graphene oxide-silver nanoparticle nanocomposite: a potential anticancer nanotherapy. Int J Nanomed. 2015;10:6257–6276.