Abstract
Arbutin (ARB) has been widely used in skin pigmentation disorders. Nevertheless, the involvements of ARB in diabetic nephropathy (DN) are still unknown. We investigated the functions of ARB in high glucose (HG)-induced cell apoptosis and autophagy in HK-2 cells. Cell viability was examined through CCK-8 in HK-2 cells after disposal with 45 mM glucose and ARB (10-50 μM). Flow cytometry and western blot tested cell apoptosis and the related protein levels in HK-2 cells after 45 mM glucose and 50 μM ARB administration. RT-qPCR delved microRNA (miR)-27a expression in HG and ARB co-treated HK-2 cells. Effect of miR-27a on ARB affected cell apoptosis and autophagy was investigated after miR-27a inhibitor transfection. JNK and mTOR pathways were finally assessed by western blot. ARB alleviated HG-induced cell apoptosis, autophagy and regulated the related protein levels in HK-2 cells. MiR-27a expression was reduced in HG-treated cells, but was accelerated in HG and ARB co-treated HK-2 cells with the increased concentration. Inhibition of miR-27a apparently abolished the outcomes of ARB in HG-induced HK-2 cells apoptosis and autophagy. Besides, ARB blocked JNK and mTOR pathways by regulating miR-27a. The findings demonstrated that ARB alleviated apoptosis and autophagy in HG-treated HK-2 cells by regulating miR-27a/JNK/mTOR axis.
Introduction
Diabetic nephropathy (DN) is the material complication of type-I or type-II diabetes mellitus, has become one of the main pathogenesis of end-stage kidney disease [Citation1,Citation2]. Classically, DN is mainly associated with metabolic disorders, hemodynamic disorders, oxidative stress, inflammation and genetic factors [Citation3,Citation4]. The signs and symptoms of DN often present at 5–10 years after beginning of the disease, which include nocturia, tiredness, headaches, nausea, vomiting, inappetence, itchy skin and leg swelling [Citation5]. Recently, the therapeutic strategies of DN include controlling blood sugar, blood pressure, regulating blood lipids, diet intervention and multifactorial intervention [Citation3,Citation6]. Although the disease has been delayed and controlled, the long-term prognosis is still unsatisfactory. Tubular injury is an important pathological feature of DN. Therefore, to study the underlying mechanism of tubular injury under high glucose (HG) environment possesses great sense for seeking the novel targets and effective agents for DN.
Apoptosis and autophagy are important biological processes, which joins in mediating the pathogenesis of DN. Apoptosis is one of the mechanisms of inducing renal cell progressive loss, thereby resulting in glomerular sclerosis, tubular atrophy and renal interstitial fibrosis [Citation7]. The factors of p53 and Bcl-2 are proverbially studied proteins related to apoptosis, which can regulate cell apoptosis through activating its downstream pathways [Citation8]. Caspase-3 is an important member of Caspase family, which occupies a central position in regulating cell apoptosis process [Citation9]. Cell autophagy plays a momentous role in maintaining the stable structure and function of normal glomerular podocytes and proximal renal tubular epithelial cells [Citation10]. Beclin-1 is a homologous gene of yeast autophagy gene Atg6/Vps30, which is one of the essential molecules of the formation of autophagosome [Citation11]. LC3 is involved in the formation of autophagy, has been proved to be one of the common autophagosome marker proteins in mammalian cells [Citation11]. JNK and mTOR pathways have been testified to join in regulating cell apoptosis and autophagy in diverse diseases and also present the vital roles in the pathophysiology of DN [Citation12,Citation13]. These researches corroborated the involvements of cell apoptosis, autophagy, as well as JNK/mTOR pathway in the pathophysiology of DN.
Arbutin (ARB, molecular formula: C12H16O7, molecular weight: 272.25) is a natural hydroquinone glycoside, which exists in many plant foods, and is often used in various pigmented skin diseases [Citation14,Citation15]. In previous studies, ARB is closely linked to the pigmentation of human melanocytes and is also associated with the differentiation of melanocytes [Citation16,Citation17]. Recently, Jurica et al. investigated the role of ARB in human peripheral blood lymphocytes and found that ARB could inhibit lymphocyte proliferation [Citation18]. Additionally, the bactericidal, anti-inflammatory and anti-glycation activities in vitro of ARB have also been confirmed in the existing researches [Citation19–21]. Farzanegi et al. found the protective effect of ARB on cardiac tissue against diabetes-induced oxidative stress via the antioxidant capacity in diabetic rats [Citation22]. Moreover, the anti-oxidant activity of ARB might improve oxidative stress defence, thereby declining the risk of T2DM [Citation23,Citation24]. On the basis of these researches, we attempt to disclose whether ARB can affect the pathogenesis of DN.
Growing evidences confirm that miRNAs can promote renal fibrosis, inhibit mesangial cell proliferation and affect epithelial mesenchymal transition (EMT) process, thereby affecting the pathogenesis of DN [Citation25–27]. These evidences indicated that miRNAs might be potential molecular biomarkers for the early diagnosis and treatment of DN in clinic. MiR-27a is a member of miR-27 family, which is a neoteric regulator in the physiological process of atherosclerosis [Citation28] and cardiovascular disease [Citation29]. The abnormally expressed miR-27a has been discovered in the dissimilar diseases, such as metabolic syndrome [Citation30], diabetes [Citation31], obesity [Citation12] and nonalcoholic fatty liver disease [Citation13]. It was worth noting that miR-27a expression was up-regulated in the patients with type I and type II diabetes [Citation30,Citation32]. However, the role and specific mechanism of miR-27a in DN are still indistinct. Therefore, we attempt to uncover whether miR-27a is a crucial regulator in DN.
In this paper, we tried to ascertain the regulatory effect of ARB on cell apoptosis and autophagy induced by HG in vitro. Our research confirmed the protective effect of ARB on apoptosis and autophagy which were evoked by HG in HK-2 cells by mediating miR-27a/JNK/mTOR axis.
Materials and methods
Cell culture and treatment
HK-2 cells (ATCC® CRL-2190™, Rockville, MD, USA) were cultured in Keratinocyte Serum Free Medium (K-SFM, Invitrogen, Carlsbad, CA, USA), which was supplied with 0.05 mg/mL bovine pituitary extract (BPE) and 5 ng/mL epidermal growth factor (EGF, Invitrogen). The cells were placed in a CO2 incubator at 37 °C. Cells in logarithmic phase were taken for the subsequent experiment. For HG administration, HK-2 cells were subjected to 5.5 mM glucose as normal treatment or 45 mM glucose as HG treatment. ARB (>98%, Sigma-Aldrich Chemical Co., St. Louis, MO, USA) was diluted and adjusted the concentrations to 10, 30 and 50 μM. These different concentrations of ARB were employed to pre-treat HK-2 cells for 6 h at 37 °C.
Cell viability assay
HK-2 cells were inoculated into 96-well plate with 5 × 103 cell/well and treated with normal glucose (5.5 mM), HG (45 mM) or a series of ARB (10, 30 and 50 μM). After incubation, 10 μL Cell Counting Kit-8 (CCK-8) solution (Dojindo Laboratories, Kyushu, Japan) was supplemented to culture plate and incubated for another 1 h at 37 °C. A Microplate Reader (Multiscan EX; Labsystems, Helsinki, Finland) was executed to examine the optical density of each well at 450 nm wavelength (OD450).
Apoptosis assay
The common used apoptosis kit (Solarbio, Beijing, China) was performed for apoptosis assessment. HK-2 cells (5 × 105) well-grown in logarithmic phase were cultured in 6-well plates, as well as subjected to normal glucose (5.5 mM), HG (45 mM) or a series of ARB (10, 30 and 50 μM). After this, cells were collected and transferred to a centrifuge tube. After 5 min centrifugation, cells were washed and re-suspended in 100 μL 1× Binding Buffer, simultaneously supplemented 5 μL Annexin V-FITC dye solution and incubated at room temperature for 15 min in the absence of light. Then, cells were washed again and re-suspended in 200 μL 1× Binding Buffer, as well as added 5 μL PI dye solution. After reaction, the ratio of apoptotic cells was assessed by flow cytometry within 1–2 h.
Cell transfection
According to the previous experience of liposome transfection, miR-27a inhibitor, its control (GenePharma Co., Shanghai, China) and lipofectamine 3000 reagent (Invitrogen) were diluted into 150 μL serum-free medium. Afterward, the dilutions of plasmids and lipofectamine 3000 reagent were mixed and rest for 20 min at room temperature to make the plasmid was fully encapsulated by liposomes. After transfection for 48 h, HK-2 cells were harvested and were utilized for the next RT-qPCR and western blot assays.
Reverse transcription-quantitative polymerase chain reaction (RT-qPCR)
Trizol reagent (Life Technologies Corporation, Carlsbad, CA, USA) was utilized to extract the total RNA from HK-2 cells, which were treated with HG and ARB or transfected with miR-27a inhibitor and NC. Preparation of 10 μL reverse transcription system according to the specifications of the PrimeScript® RT reagent kit (Takara Bio, Inc., Tokyo, Japan). The obtained cDNA product was diluted in RNA-free water at a ratio of 1:10 and used to confect the RT-qRCR system according to the Kit instruction of Taqman MicroRNA Reverse Transcription Kit and Taqman Universal Master Mix II (Applied Biosystems, Foster City, CA). U6 served as an internal reference for normalizing miR-27a expression. Data in this experiment were measured by a classical 2-ΔΔCt method [Citation33].
Western blot assay
After administration or transfection, HK-2 cell extracts were prepared as Zhong et al. described [Citation34]. BCA™ Protein Assay Kit (Thermo Scientific, Rockford, IL, USA) was utilized for the assessment the content of protein samples. Equivalent samples were loaded on SDS-PAGE and then transferred to the polyvinylidene difluoride (PVDF) membranes (GE Healthcare, Piscataway, NJ, USA). After sealing with 5% BSA, the PVDF membranes were washed and co-incubated with the diluted antibodies (1:1,000) at 4 °C overnight. The used antibodies included p53 (ab32389), Bcl-2 (ab32124), Pro-Caspase-3 (ab32499), Cleaved-Caspase-3 (ab2320), Beclin-1 (ab62557), p62 (ab109012), LC3-II (ab48394), JNK (ab199380), p-JNK (ab47337), mTOR (ab134930), p-mTOR (ab109268), S6K (ab32359), p-S6K (ab59208) and β-actin (ab227387, Abcam, Cambridge, MA). Subsequently, the second antibody (ab205718, 1:2000, Abcam) was incubated with the membrane for 1 h at room temperature. The enhanced chemiluminescence (ECL) reagents (GE Healthcare) was prepared and dropped uniformly on PVDF membranes. After reaction, the X-film was scanned, and the protein bands and the Gary values of corresponding internal parameters were analysed by Image Lab™ Software (Bio-Rad).
Statistical analysis
SPSS 19.0 statistical software (SPSS Inc., Chicago, IL) was executed to analyse the data of different groups, and the results were expressed by mean ± standard deviation (SD). ANOVA followed by post hoc Tukey test and Student’s t-test were exploited to analyse significance differences. p < .05 was deemed to have statistical significance.
Results
ARB alleviated HG-induced HK-2 cell apoptosis
The molecular structure of ARB was shown in . HK-2 cells disposed with 45 mM glucose served as HG group, and cells treated with 5.5 mM glucose was regarded as a control group. CCK-8 assay determined cell viability displayed that HG treatment suppressed the viability of HK-2 cells as compared with control group (p < .01, ). HK-2 cells were then treated with a series of ARB (10, 30 and 50 μM). Under normal glucose condition, ARB had no influence on cell viability in HK-2 cells (). However, after treatment with HG, ARB evidently promoted cell viability (p < .05 or p < .01) with the increased concentrations in HK-2 cells (). In the rest experiments, 50 μM of ARB was utilized to treat HK-2 cells. The results in revealed that cell apoptosis was induced by HG (p < .001); however, ARB notably inhibited HG-induced cell apoptosis (p < .05) in HK-2 cells. Besides, western blot assay showed that HG up-regulated p53 and Cleaved-Caspase-3 expression, synchronously down-regulated Bcl-2 expression (p < .001). ARB also reversed these results in HK-2 cells accompanied by HG treatment (p < .001, ). Data from above experiments indicated that ARB alleviated HG-induced cell apoptosis in HK-2 cells.
Figure 2. ARB alleviated HG-induced HK-2 cells apoptosis. After administration with (A) high glucose (HG, 45 mM), (B) normal glucose (5.5 mM) and a series of ARB (10, 30 and 50 μM), (C) high glucose (HG, 45 mM) and ARB (10, 30 and 50 μM), cell viability of HK-2 cells by CCK-8 assay assessment. After disposing with high glucose (HG, 45 mM) and 50 μM ARB, (D) cell apoptosis, (E and F) p53, Bcl-2 and Pro/Cleaved-Caspase-3 by flow cytometry and western blot detection. HG vs Control: **p< .01, ***p < .001; HG + ARB vs HG: #p < .05, ##p < .01, ###p < .001; ns: no significant difference.
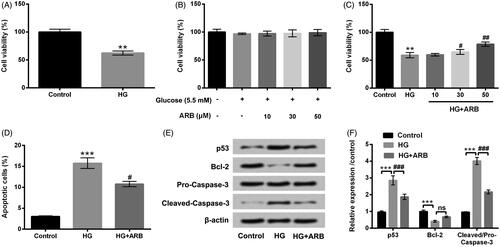
ARB inhibited HG-induced HK-2 cell autophagy
Western blot assay was carried out to assess Beclin-1, p62 and LC3-II protein levels to uncover the influence of ARB in cell autophagy. The results in revealed that HG significantly up-regulated Beclin-1 and LC3-II levels, meanwhile down-regulated p62 level (p < .001) in HK-2 cells. However, ARB observably reversed the effect of HG on Beclin-1, p62 and LC3-II expression (p < .001). These data hinted that ARB could inhibit HG-induced HK-2 cell autophagy.
Figure 3. ARB inhibited HG-induced HK-2 cells autophagy. HK-2 cells were disposed with high glucose (HG, 45 mM) and 50 μM ARB, (A) autophagy associated proteins by western blot assessment following with (B) the relative density analysis in HK-2 cells. HG vs Control: ***p < .001; HG + ARB vs HG: ###p < .001; ns: no significant difference.
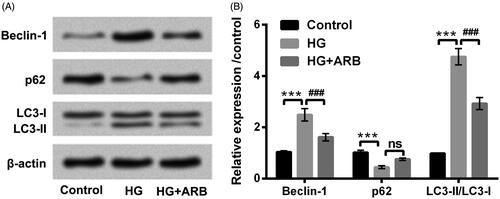
ARB up-regulated miR-27a expression in HG-treated cells
After administration with HG alone, or co-management with HG and ARB (10, 30 and 50 μM), miR-27a expression level in treated HK-2 cells were explored through RT-qPCR assay. results obviously exhibited the inhibition of miR-27a in HK-2 cells along with HG treatment compared with untreated cells (p < .001). However, ARB significantly up-regulated miR-27a expression with the increased concentrations in HK-2 cells followed by HG treatment (p < .001, ). Collectively, ARB up-regulated miR-27a expression in HG-treated cells, indicating that miR-27a might be a key regulator in ARB affected HK-2 cells apoptosis and autophagy.
Figure 4. ARB up-regulated miR-27a expression in HG-treated cells. HK-2 cells were stimulated with 5.5 mM or 45 mM glucose as control or HG group, meanwhile treated with ARB (10, 30 and 50 μM). MiR-27a expression by RT-qPCR determination in these treated cells. HG vs Control: ***p < .001; HG + ARB vs HG: ###p < .001; ns: no significant difference.
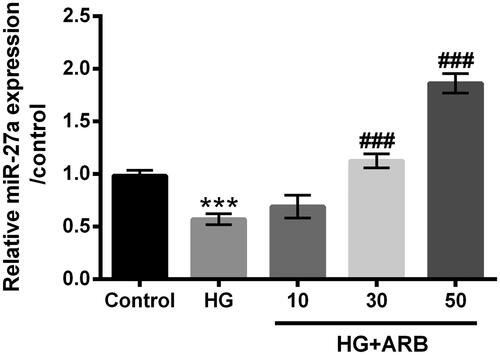
ARB weakened HG-induced HK-2 cells apoptosis and autophagy by mediating miR-27a
To further explore the involvement of miR-27a in cell apoptosis and autophagy induced by HG, miR-27a inhibitor and its control were respectively transfected into HK-2 cells. After 48 h transfection, RT-qPCR assay verified that miR-27a expression was evidently repressed in HK-2 cells after miR-27a inhibitor transfection (p < .001, ). The transfected cells were then co-treated with HG and ARB, cell viability, apoptosis, and autophagy were then investigated. revealed that miR-27a inhibition prominently suppressed cell viability as well as induced apoptosis in HG and ARB-treated cells (p < .001). p53 and Cleaved-caspase-3 expression were up-regulated, and Bcl-2 expression was decreased by miR-27a inhibition in HG and ARB-treated cells (p < .001, ). Additionally, Beclin-1 and LC3-II were notably increased, and p62 was repressed by miR-27a inhibition in HG and ARB-treated cells (p < .001, ). All above data suggested that miR-27a inhibition abolished the effect of ARB on cell apoptosis and autophagy induced by HG treatment in HK-2 cells.
Figure 5. ARB relieved HG-induced HK-2 cells apoptosis and autophagy by mediating miR-27a. (A) MiR-27a inhibitor and NC were respectively transfected into HK-2 cells, miR-27a expression by RT-qPCR assessment in these cells; The transfected cells were disposed with normal glucose (5.5 mM), high glucose (HG, 45 mM), as well as ARB (50 μM). (B) Cell viability, (C) apoptosis, (D and E) p53, Bcl-3, Pro/Cleaved-Caspase-3 expression and (F and G) Beclin-1, p62, LC3-I and LC3-II expression by CCK-8, flow cytometry and western blot assays detection, respectively. MiR-27a inhibitor vs NC or HG vs Control: **p < .01, ***p < .001; HG + ARB vs HG: ###p < .001; HG + ARB + miR-27a inhibitor vs HG + ARB + NC: &&&p < .001; ns: no significant difference.
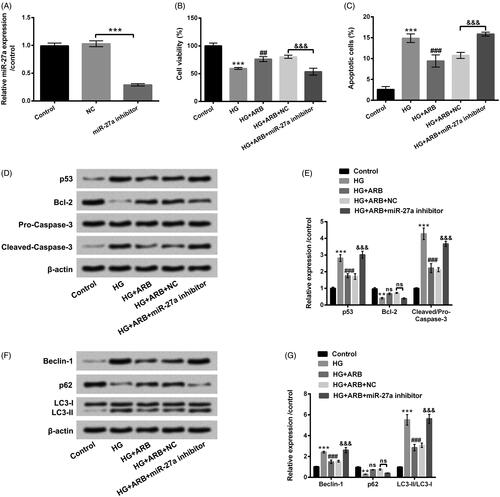
ARB regulated JNK and mTOR-signalling pathways by mediating miR-27a
Effects of ARB on JNK and mTOR pathways were examined in HG-treated HK-2 cells. The results in revealed that p-JNK, p-mTOR and p-S6K protein levels were all increased by HG (p < .01 or p < .001), as well as were repressed after treatment with ARB (p < .05 or p < .001). However, the inhibitory effect of ARB on JNK and mTOR signalling pathways was notably abolished in HK-2 cells after transfection with miR-27a inhibitor (p < .05 or p < .001). JNK, mTOR and S6K remained unchanged in different treated cells. These data indicated that ARB blocked JNK and mTOR-signalling pathways via mediating miR-27a in HG-stimulated HK-2 cells.
Figure 6. ARB regulated JNK and mTOR-signalling pathways by mediating miR-27a. MiR-27a inhibitor and NC were respectively transfected into HK-2 cells, and these transfected cells were administrated with normal glucose (5.5 mM), high glucose (HG, 45 mM), and ARB (50 μM). (A and B) JNK and p-JNK as well as (C and D) mTOR, p-mTOR, S6K and p-S6K protein levels were assessed by western blot. HG vs Control: **p < .01, ***p < .001; HG + ARB vs HG: #p < .05, ###p < .001; HG + ARB + miR-27a inhibitor vs HG + ARB + NC: &p < .05, &&&p < .001.
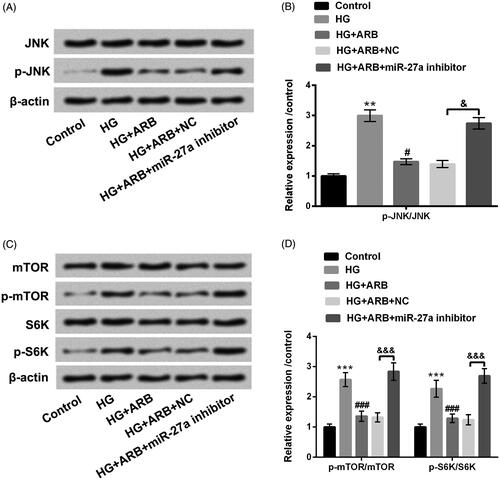
Discussion
DN is a serious complication of diabetes mellitus, which not only increases the mortality of diabetic patients, but also affects the patients’ life quality [Citation35]. Recently, the use of Chinese herbs and their extracts in the treatment of DN has achieved good results [Citation36]. Interestingly, Li et al. explored the functions of Astilbin in DN through performing in vivo and in vitro experiments. The results proven the renoprotection of Astilbin in STZ-induced DN model, as well as showed the inhibitory effect of Astilbin on the expression levels of TGF-β1 and CTGF in HK-2 cells accompanied by HG treatment, which are the initial evidences of Astilbin as a novel candidate agent for DN [Citation37]. However, whether ARB is a potential drug for the treatment of DN has not been uncovered. The study probed the effect of ARB on apoptosis and autophagy in HK-2 cells along with HG management, concurrently uncovered the regulatory mechanism of ARB in the pathogenesis of DN.
Autophagy is a pivotal biological phenomenon, participating in the development and growth of organisms [Citation38]. Increasing evidences demonstrate that autophagy is linked to the pathogenesis of kidney disease [Citation39,Citation40]. Study from Chen et al. displayed that Astilbin could suppress HG-induced HK-2 cells autophagy and apoptosis by regulating PI3K/AKT pathway [Citation41]. The interesting observation in our study demonstrated that ARB distinctly inhibited HG-induced HK-2 cells apoptosis and down-regulated p53 and Cleaved-Caspase-3, up-regulated Bcl-2 expression level. Additionally, ARB down-regulated Beclin-1, LC3-II expression, but up-regulated p62 expression in HK-2 cells along with HG treatment. These data indicated that ARB could alleviate HG-induced HK-2 cells apoptosis and autophagy. These data indicated the involvement of ARB in the pathogenesis of DN.
The role of miRNA in the pathogenesis of DN has been extensively reviewed by mediating growth factors and fibrosis in DN [Citation42]. Recent research demonstrated that up-regulated miRNAs, such as miR-192, miR-216a and miR-217 contribute to the pathogenesis of DN via targeting EF1, SIP1, ZEB1/2 and PTEN genes [Citation43]. MiR-22 has been discovered to promote renal tubulointerstitial fibrosis by targeting PTEN and suppressing autophagy in DN [Citation44]. In terms of miR-27a, increased miR-27a has been discovered in different cancers, such as pancreatic cancer [Citation45], hepatocellular carcinoma [Citation46] and breast cancer [Citation47], which confirms as an oncogene in these cancers. In addition, Hou et al. displayed that miR-27a could promote renal tubulointerstitial fibrosis by blocking PPARγ pathway in DN [Citation48]. Wu et al., miR-27a has reported to induce mesangial cell injury, and its knockdown could prevent the development of DN [Citation49]. Nevertheless, whether miR-27a is a key participator in HG-induced HK-2 cells apoptosis and autophagy remains unclear. Our research exhibited the reduction of miR-27a in HG-treated HK-2 cells, but the down-regulatory effect of HG on miR-27a expression was notably reversed by ARB. Further study demonstrated that miR-27a inhibition apparently abolished the protective effect of ARB on HK-2 cells apoptosis and autophagy evoked by HG, suggesting that ARB alleviated HG-induced HK-2 cells apoptosis and autophagy might via mediating miR-27a expression.
Mounting evidences indicate that various pathways are associated with the pathogenesis of DN, which contain JNK and mTOR pathways [Citation50–52]. Recent study demonstrated that novel curcumin analogue C66 could prevent DN by regulating JNK-signalling pathway [Citation53]. Moreover, JNK pathway participated in the process of HG evoked renal mesangial cell proliferation and fibronectin expression [Citation54]. Furthermore, highly activated mTOR pathway has been found in DN, and mTOR inhibition can prevent progressive DN [Citation55,Citation56]. Herein, the regulatory effect of ARB on JNK and mTOR pathways in HG-treated HK-2 cells was delved. Data manifested that ARB restrained JNK and mTOR pathway activations in HG-stimulated HK-2 cells. Recent research revealed that long non-coding RNA CASC2 could improve the progression of DN via prohibiting JNK pathway [Citation57]. Additionally, miR-148b-3p has been testified to suppress mTORC1-dependent apoptosis in diabetes by repressing TNFR2 in proximal tubular cells [Citation58]. However, the underlying molecule mechanism of miR-27a regulating JNK/mTOR pathway to participate in the pathogenesis of DN is still ambiguous. In this research, we discovered that the inhibitory effect of ARB on JNK and mTOR pathways was reversed by miR-27a inhibition. These data suggested that ARB functioned to HG-induced HK-2 cells apoptosis and autophagy possibly through modulating JNK and mTOR pathways. Moreover, miR-27a might be a vital regulator in this process. Further researches are still necessary for uncovering the probable molecule mechanism of miR-27a and JNK/mTOR pathways in DN.
Taken together, the study uncovered that ARB mitigated HG-induced HK-2 cells apoptosis and autophagy by mediation of JNK and mTOR-signalling pathways by up-regulating miR-27a. These findings provided novel evidences that ARB might be a novel agent for DN. More applications of ARB in DN merit further research.
Statement
Jining No.1 People's Hospital, Jining Medical University and Jiaxiang County Medicine Hospital did not provide our authors the institutional email addresses. So we can only use our personal email address to submit the paper. We confirm that they are not fake email addresses. The authors can be reached at their email addresses.
Disclosure statement
The authors declare no conflict of interest.
References
- Ma ZJ, Sun P, Guo G, et al. Association of the HLA-DQA1 and HLA-DQB1 alleles in type 2 diabetes mellitus and diabetic nephropathy in the han ethnicity of China. J Diabetes Res. 2013;2013:1–452537.
- Senthilkumar GP, Anithalekshmi MS, Yasir M, et al. Role of omentin 1 and IL-6 in type 2 diabetes mellitus patients with diabetic nephropathy. Diabetes Metab Syndr. 2018;12:23–26.
- Lv M, Chen Z, Hu G, et al. Therapeutic strategies of diabetic nephropathy: recent progress and future perspectives. Drug Discov Today. 2015;20:332–346.
- Duran-Salgado MB, Rubio GA. Diabetic nephropathy and inflammation. WJD. 2014;5:393–398.
- Afkarian M, Zelnick LR, Hall YN, et al. Clinical manifestations of kidney disease among US adults with diabetes, 1988–2014. JAMA. 2016;316:602–610.
- Lim AK. Diabetic nephropathy - complications and treatment. Int J Nephrol Renovascul Dis. 2014;7:361–381.
- Zhu Y, Cui H, Xia Y, et al. RIPK3-mediated necroptosis and apoptosis contributes to renal tubular cell progressive loss and chronic kidney disease progression in rats. PLoS One. 2016;11:e0156729.
- Kim EM, Jung CH, Kim J, et al. The p53/p21 complex regulates cancer cell invasion and apoptosis by targeting Bcl-2 family proteins. Cancer Res. 2017;77:3092–3100.
- Rogers C, Fernandes-Alnemri T, Mayes L, et al. Cleavage of DFNA5 by caspase-3 during apoptosis mediates progression to secondary necrotic/pyroptotic cell death. Nat Commun. 2017;8:14128.
- Alghamdi TA, Majumder S, Thieme K, et al. Janus kinase 2 regulates transcription factor EB expression and autophagy completion in glomerular podocytes. J Am Soc Nephrol. 2017;28:2641–2653.
- Hamurcu Z, Delibaşı N, Geçene S, et al. Targeting LC3 and Beclin-1 autophagy genes suppresses proliferation, survival, migration and invasion by inhibition of Cyclin-D1 and uPAR/Integrin β1/ Src signaling in triple negative breast cancer cells. J Cancer Res Clin Oncol. 2018;144:415–430.
- Xie H, Sun L, Lodish HF. Targeting microRNAs in obesity. Expert Opin Therap Targets. 2009;13:1227–1238.
- Alisi A, Da Sacco L, Bruscalupi G, et al. Mirnome analysis reveals novel molecular determinants in the pathogenesis of diet-induced nonalcoholic fatty liver disease. Lab Invest. 2011;91:283–293.
- Tai A, Ohno A, Ito H. Isolation and characterization of the 2,2'-azinobis(3-ethylbenzothiazoline-6-sulfonic acid) (ABTS) radical cation-scavenging reaction products of arbutin. J Agric Food Chem. 2016;64:7285–7290.
- Ertam I, Mutlu B, Unal I, et al. Efficiency of ellagic acid and arbutin in melasma: a randomized, prospective, open-label study. J Dermatol. 2008;35:570–574.
- Inoue Y, Hasegawa S, Yamada T, et al. Analysis of the effects of hydroquinone and arbutin on the differentiation of melanocytes. Biol Pharm Bull. 2013;36:1722–1730.
- Lim YJ, Lee EH, Kang TH, et al. Inhibitory effects of arbutin on melanin biosynthesis of alpha-melanocyte stimulating hormone-induced hyperpigmentation in cultured brownish guinea pig skin tissues. Arch Pharm Res. 2009;32:367–373.
- Jurica K, Karačonji IB, Mikolić A, et al. In vitro safety assessment of the strawberry tree (Arbutus unedo L.) water leaf extract and arbutin in human peripheral blood lymphocytes. Cytotechnology. 2018;70:1261–1278.
- Jedsadayanmata A. In vitro antiglycation activity of arbutin. NU J Sci Tech. 2013;13(2):35–41.
- Jurica K, Gobin I, Kremer D, et al. Arbutin and its metabolite hydroquinone as the main factors in the antimicrobial effect of strawberry tree (Arbutus unedo L.) leaves. J Herbal Med. 2017;8:17–23.
- Zhang B, Zeng M, Li B, et al. Inhibition of oxidative stress and autophagy by arbutin in lipopolysaccharide-induced myocardial injury. Phcog Mag. 2019;15:507.
- Farzanegi P, Habibian M, Anvari S. Effect of swimming training and arbutin supplement on cardiac antioxidant enzymes and oxidative stress in diabetic rats. J Gorgan University of Med Sci. 2015;17:39–44.
- Bang SH, Han SJ, Kim DH. Hydrolysis of arbutin to hydroquinone by human skin bacteria and its effect on antioxidant activity. J Cosmetic Dermatol. 2008;7:189–193.
- Guo XF, Yang B, Tang J, et al. Apple and pear consumption and type 2 diabetes mellitus risk: a meta-analysis of prospective cohort studies. Food Funct. 2017;8:927–934.
- He F, Peng F, Xia X, et al. MiR-135a promotes renal fibrosis in diabetic nephropathy by regulating TRPC1. Diabetologia. 2014;57:1726–1736.
- Zhang Z, Peng H, Chen J, et al. MicroRNA-21 protects from mesangial cell proliferation induced by diabetic nephropathy in db/db mice. FEBS Lett. 2009;583:2009–2014.
- Srivastava SP, Koya D, Kanasaki K. MicroRNAs in kidney fibrosis and diabetic nephropathy: roles on EMT and EndMT. BioMed Res Int. 2013;2013:1.
- Chen WJ, Yin K, Zhao GJ, et al. The magic and mystery of microRNA-27 in atherosclerosis. Atherosclerosis. 2012;222:314–323.
- Yu K, Ji Y, Wang H, et al. Association of miR-196a2, miR-27a, and miR-499 polymorphisms with isolated congenital heart disease in a Chinese population. Genet Mol Res. 2016;15:1–14. gmr15048929.
- Karolina DS, Tavintharan S, Armugam A, et al. Circulating miRNA profiles in patients with metabolic syndrome. J Clin Endocrinol Metab. 2012;97:E2271–E2276.
- Wang TT, Chen YJ, Sun LL, et al. Affection of single-nucleotide polymorphisms in miR-27a, miR-124a, and miR-146a on susceptibility to type 2 diabetes mellitus in Chinese Han people. Chinese Med J. 2015;128:533–539.
- Herrera BM, Lockstone HE, Taylor JM, et al. Global microRNA expression profiles in insulin target tissues in a spontaneous rat model of type 2 diabetes. Diabetologia. 2010;53:1099–1109.
- Livak KJ, Schmittgen TD. Analysis of relative gene expression data using real-time quantitative PCR and the 2(-Delta Delta C(T)) method. Methods. 2001;25:402–408.
- Zhong ZF, Hoi PM, Wu GS, et al. Anti-angiogenic effect of furanodiene on HUVECs in vitro and on zebrafish in vivo. J Ethnopharmacol. 2012;141:721–727.
- Hamed AT, Taha MM, Nasser LM. Renoprotective effect of aliskiren monotherapy and aliskiren − pentoxifylline combination in hypertensive-diabetic type 2 patients with diabetic nephropathy. Bull Fac Pharm Cairo University. 2013;51:221–227.
- Zhang H, Li P, Burczynski FJ, et al. Attenuation of diabetic nephropathy in Otsuka Long-Evans Tokushima Fatty (OLETF) rats with a combination of Chinese herbs (Tangshen formula). Evid-Based Complementary Alternat Med. 2011;2011:1–613737.
- Li GS, Jiang WL, Yue XD, et al. Effect of astilbin on experimental diabetic nephropathy in vivo and in vitro. Planta Med. 2009;75:1470–1475.
- Mizushima N, Komatsu M. Autophagy: renovation of cells and tissues. Cell. 2011;147:728–741.
- Hartleben B, Godel M, Meyer-Schwesinger C, et al. Autophagy influences glomerular disease susceptibility and maintains podocyte homeostasis in aging ing mice. J Clin Invest. 2010;120:1084–1096.
- Kimura T, Takabatake Y, Takahashi A, et al. Autophagy protects the proximal tubule from degeneration and acute ischemic injury. JASN. 2011;22:902–913.
- Chen F, Sun Z, Zhu X, et al. Astilbin inhibits high glucose-induced autophagy and apoptosis through the PI3K/Akt pathway in human proximal tubular epithelial cells. Biomed Pharmacother. 2018;106:1175–1181.
- Hagiwara S, McClelland A, Kantharidis P. MicroRNA in diabetic nephropathy: renin angiotensin, aGE/RAGE, and oxidative stress pathway. J Diabetes Res. 2013;2013:1–173783.
- Wu H, Kong L, Zhou S, et al. The role of microRNAs in diabetic nephropathy. J Diabetes Res. 2014;2014:920134
- Zhang Y, Zhao S, Wu D, et al. MicroRNA-22 promotes renal tubulointerstitial fibrosis by targeting PTEN and suppressing autophagy in diabetic nephropathy. J Diabetes Res. 2018;2018:1–4728645.
- Ma Y, Yu S, Zhao W, et al. miR-27a regulates the growth, colony formation and migration of pancreatic cancer cells by targeting Sprouty2. Cancer Lett. 2010;298:150.
- Huang S, He X, Ding J, et al. Upregulation of miR-23a approximately 27a approximately 24 decreases transforming growth factor-beta-induced tumor-suppressive activities in human hepatocellular carcinoma cells. Int J Cancer. 2008;123:972–978.
- Guttilla IK, White BA. Coordinate regulation of FOXO1 by miR-27a, miR-96, and miR-182 in breast cancer cells. J Biol Chem. 2009;284:23204–23216.
- Hou X, Tian J, Geng J, et al. MicroRNA-27a promotes renal tubulointerstitial fibrosis via suppressing PPARgamma pathway in diabetic nephropathy. Oncotarget. 2016;7:26–47760.
- Wu L, Wang Q, Guo F, et al. MicroRNA-27a induces mesangial cell injury by targeting of PPARgamma, and its in vivo knockdown prevents progression of diabetic nephropathy. Sci Rep. 2016;6:17–26072.
- Xie X, Peng J, Chang X, et al. Activation of RhoA/ROCK regulates NF-kappaB signaling pathway in experimental diabetic nephropathy. Mol Cell Endocrinol. 2013;369:86–97.
- Zhang H, Liu X, Zhou S, et al. SP600125 suppresses Keap1 expression and results in NRF2-mediated prevention of diabetic nephropathy. J Mol Endocrinol. 2018;60:145–157.
- Lu Q, Zuo WZ, Ji XJ, et al. Ethanolic Ginkgo biloba leaf extract prevents renal fibrosis through Akt/mTOR signaling in diabetic nephropathy. Phytomedicine. 2015;22:1071–1078.
- Wang Y, Wang Y, Luo M, et al. Novel curcumin analog C66 prevents diabetic nephropathy via JNK pathway with the involvement of p300/CBP-mediated histone acetylation. Biochim Biophys Acta. 2015;1852:34–46.
- Zhang L, Pang S, Deng B, et al. High glucose induces renal mesangial cell proliferation and fibronectin expression through JNK/NF-κB/NADPH oxidase/ROS pathway, which is inhibited by resveratrol. Int J Biochem Cell Biol. 2012;44:629–638.
- Gödel M, Hartleben B, Herbach N, et al. Role of mTOR in podocyte function and diabetic nephropathy in humans and mice. J Clin Invest. 2011;121:2197–2209.
- Mori H, Inoki K, Masutani K, et al. The mTOR pathway is highly activated in diabetic nephropathy and rapamycin has a strong therapeutic potential. Biochem Biophys Res Commun. 2009;384:471–475.
- Yang H, Kan QE, Su Y, et al. Long non-coding RNA CASC2 improves diabetic nephropathy by inhibiting JNK pathway. Exp Clin Endocrinol Diabetes. 2018;2018. doi:10.1055/a-0629-9958
- Kuwagata S, Kume S, Chin-Kanasaki M, et al. MicroRNA148b-3p inhibits mTORC1-dependent apoptosis in diabetes by repressing TNFR2 in proximal tubular cells. Kidney Int. 2016;90:1211–1225.