Abstract
We aimed to determine the roles and possible mechanism of long non-coding RNA SDPR-AS in the processes of non-small cell lung cancer (NSCLC). The expressions of SDPR-AS and SDPR in different subtypes of lung cancer (AC, SCC, LCC and SCLC) tissues and cells were determined. Three NSCLC cells were infused with pcDNA-SDPR-AS, pcDNA3.1, sh-SDPR-AS, sh-NC, si-SDPR and si-NC. The effects of SDPR-AS dysregulation on cell behaviors (including cell viability, colony forming ability, migration, invasion and apoptosis) were assessed. Moreover, the combined effects of SDPR-AS overexpression and SDPR-AS knockdown on H522 cell behaviors and the levels of p-p38 and p-ERK were investigated. SDPR-AS was lowly expressed in NSCLC tissues and cells, but had no changes in SCLC tissues and cells. Down-regulation of SDPR-AS enhanced the proliferation, migration and invasion and inhibited apoptosis of NSCLC cells (H522, H661 and H520). Overexpression of SDPR-AS exhibited opposite effects. Moreover, SDPR was positively regulated by SDPR-AS and effects of SDPR-AS on the cell biological processes of NSCLC cells were through regulation of SDPR. Besides, the levels of p-p38 and p-ERK were significantly decreased after SDPR-AS overexpression, which were dramatically changeover by SDPR knockdownsimultaneously. Our findings indicate that SDPR-AS was lowly expressed in NSCLC cells and down-regulation of SDPR-AS may promote the malignant behaviors of NSCLC cells possible through regulating SDPR expression and involving in p38 MAPK/ERK signaling pathway. SDPR-AS may serve as a prospective target for NSCLC diagnosis and therapy.
Introduction
Lung cancer remains to be a major cause of cancer-relevant mortality globally [Citation1], of which non-small-cell lung cancer (NSCLC) is the main type with an estimated percentage of 85% [Citation2]. NSCLC can be separated into three histologic subtypes, including adenocarcinoma, squamous cell carcinoma, and large-cell lung carcinoma [Citation3]. Moreover, the overall 5-year survival rate of NSCLC is estimated to be only 11% [Citation4,Citation5]. Therefore, elucidation of the key molecular mechanism underlying NSCLC development will facilitate to greatly improve the clinical outcomes.
Long non-coding RNAs (lncRNAs) are evolutionarily conserved RNA transcripts longer than 200 bps in length with no protein-coding ability [Citation6]. LncRNAs emerge as an essential regulator in almost every aspect of biology; moreover, aberrant expression and function of lncRNAs have been emphasized in several cancers [Citation7]. In NSCLC, lncRNA urothelial carcinoma-associated 1 (UCA1) can exert oncogenic functions by promoting proliferation and colony formation of NSCLC cells [Citation8]; higher expression of lncRNA PVT1 is correlated with lymph node metastasis and poorer overall survival and its increased expression can promote tumorigenesis [Citation9,Citation10]; and lncRNA colon cancer-associated transcript 2 (CCAT2) promotes the proliferation and invasion of NSCLC cells [Citation11]. Recently, lncRNA antisense (AS) transcript of SDPR (SDPR-AS), existed at chromosome 2q32.3, has been found to be correlated to the prognosis of renal cell carcinoma (RCC) [Citation12]. However, whether SDPR-AS functions a key regulator in NSCLC remaining to be grabbed.
In the present research, we detected whether lncRNA SDPR-AS was dysregulated in different subtypes of lung cancer (AC, SCC, LCC and SCLC) and then evaluated the effects of SDPR-AS dysregulation on cell behaviors, including cell viability, colony forming ability, migration, invasion and apoptosis. Moreover, it is reported that the lncRNAs are always relevant to their neighbouring protein-coding genes before they running their functions [Citation13]. Whether SDPR-AS was pivotal to NSCLC via regulating serum deprivation protein response (SDPR) was also investigated. Furthermore, the regulatory relationship between SDPR-AS and p38 MAPK/ERK signaling pathway was investigated because of the key roles of p38 MAPK/ERK signaling pathway in mediating the development of lung cancer [Citation14,Citation15]. All efforts of this research were to explore the roles and the possible principle of lncRNA SDPR-AS in the development of NSCLC, thus providing a new insight for NSCLC diagnosis and therapy.
Materials and methods
Patients
A total of 103 NSCLC and 3 SCLC patients who were hospitalized in our hospital were recruited in this study. Anonymous surgically resected NSCLC samples were collected as shown in . Following the World Health Organization classification of tumors [Citation16], the histological diagnosis for tumor specimens was confirmed by hematoxylin-eosin (HE) staining. Tumor tissues and paired normal lung tissues at an adjacent area were acquired from the same patients. Local ethics approval and patients' informed consent were obtained before research.
Table 1. Demographic characteristics and clinical features of lung cancer patients.
Cell culture
The human AC cell line H522, LCC cell line H661, SCC cell line H520, SCLC cell line NCI-H446 and normal lung epithelial cell line of BEAS-2B, were gained from the Cell Bank of the Chinese Academy of Sciences (Shanghai, China). These cell lines were cultured in RPMI-1640 medium (Hyclone, Logan, UT, USA) which was prepared with 10% fetal bovine serum (FBS) (Sangon Biotech, Shanghai, China) in a 37 °C incubator.
Cell transfection
The SDPR-AS coding sequence was seeded into the universally available pcDNA3.1 vector to construct SDPR-AS overexpression vector, which was named to pcDNA3.1-SDPR-AS. Small interfering RNAs targeting SDPR-AS (sh-SDPR-AS) or SDPR (si-SDPR-AS) and their negative-control shRNAs (sh-NC or si-NC) were purchased from GenePharma (Shanghai, China). Prior to transfection, approximately 50% of the cells were seeded into 12-well plates and cultured for 24 h. Following the manufacturer’s instructions, cells were then transfected with pcDNA-SDPR-AS, pcDNA3.1, sh-SDPR-AS, sh-NC, si-SDPR-AS and si-NC using Lipofectamine 2000 (Invitrogen, Carlsbad, CA, USA). After 48 h, cells were obtained for subsequent assays.
RNA extraction and qRT-PCR
We isolated the total RNA both from tissues and from cells using Trizol reagent (Invitrogen). NanoDrop ND2000 (Thermo Scientific Inc., Santa Clara, CA, USA) was selected to determine the purity and concentration of isolated RNA. Reverse transcription was carried out using A First Strand cDNA Synthesis Kit (TakaRa, Dalian, China). In an Applied Biosystems 7500 fluorescent quantitative PCR system (BD Biosciences), qRT-PCR was performed for detecting the expression of targets using the SYBR Prime-Script RT-PCR kit (TakaRa) in accordance with the manufacturer’s instructions. β-actin was selected as the control and 2−ΔΔCT method was selected for measuring the relative gene levels.
Cell proliferation test
CCK8 and colony-formation tests were used for measuring the viability and colony formation capacity, respectively. For CCK8 assay, cells that seeded into 96-well plates were incubated with the CCK8 reagent for 1 h at 37 °C. The cell density which was reflected by wavelength at 470 nm was evaluated by Tecan infinite M200 multimode microplate reader (Olympus, Japan). For colony formation capacity, cells were plated into the 60-mm dishes in triplicate parallel after 48 h of transfection and continued to be incubated for 14 days. Afterwards, cells were fixed with 4% paraformaldehyde, followed by staining with 1% crystal violet (TakaRa). The number of cells were countered under macroscopic condition.
Cell apoptosis detection
Cell apoptosis was detected by flow cytometry. At 48 h after transfection, lung cancer cells were harvested, and then double stained with FITC-Annexin V and Propidium iodide (PI) using the FITC-Annexin V Apoptosis Detection Kit (BD Bioscience, NY, USA) following the manufacturer's protocols. Apoptotic cells were then analyzed with a flow cytometry (FACScan; BD Biosciences) equipped with a CellQuest software (BD Biosciences).
Transwell test
We chose Transwell assay to evaluate the lung cancer cell migration and invasion capacities. Firstly, the invasive properties of cells were assessed using the Invasion Chambers of 8 mm pore size mixed with Matrigel (Millipore, Billerica, MA, USA). After 48 h, cells were plated into the above chamber containing serum-free fresh medium, while RPMI-1640 containing 10% FBS that was used as a chemoattractant was filled into the nether chamber. After 24–48 h of incubation, cells that did not invade were wiped off from the upper chambers, whereas the invaded cells were fixed in 95% methyl aldehyde and stained with 0.5% crystal violet solution. For migrated capacity evaluation, the upper chamber was disposed free of Matrigel. The images of invaded cells per well were counted in random fields using a microscope.
Western blot
We isolated protein samples from cells after cells were disposed in cell lysis buffer (Sangon Biotech). The isolated protein samples (50 μg/lane) were disposed for separation on 12% SDS-polyacrylamide gels, followed by transferring onto polyvinylidene fluoride (PVDF) membranes (Millipore). Afterwards, membranes were blocked with 5% non-fat milk, immune-blotting of the PVDF membranes was conducted with the primary rabbit monoclonal antibodies to Bcl-2, Bax, pro-caspase3, cleaved caspase3, SDPR, p38, p-p38, ERK, p-ERK, and β-actin (1:1000 dilutions; Abcam, Cambridge, UK) overnight at 4 °C, respectively. β-actin was selected as the control. The protein signals were revealed after incubation with the appropriated secondary antibodies (1:5000; Abcam) using enhanced chemiluminescence (ECL) analysis system (Santa Cruz, USA).
Statistical analyses
Each experiment mentioned above was conducted in triplicate and data were displayed as mean ± standard deviation. Significant differences between 2 groups were analyzed with two-tailed t-test. Statistical analyses were carried out with SPSS 18.0 (SPSS, Chicago, IL, USA). p < .05 was chosen as statistically significant.
Results
LncRNA SDPR-AS is under-expressed in NSCLC tissues and cells
To grab the potential impact of lncRNA SDPR-AS in lung cancer, we evaluated the level of SDPR-AS in different subtypes of lung cancer tissues and cells. The levels of SDPR-AS in AC, SCC and LCC tissues and cells were dramatically lower relative to those in non-rumor tissues and in BEAS-2B cells (p < .05, ). However, there was no apparent difference in SDPR-AS expression between SCLC and adjacent non-rumor tissues, as well as SCLC NCI-H446 and BEAS-2B cells.
Figure 1. LncRNA SDPR-AS was down-regulated NSCLC tissues (A) and cells (B). NSCLC: non-small cell lung cancer AC: adenocarcinoma; SCC: squamous cell carcinoma; LCC: large cell carcinoma; SCLC: small cell lung cancer. Data were presented as mean ± standard deviation. *p < .05, **p < .01 and ***p < .01 compared with corresponding controls.
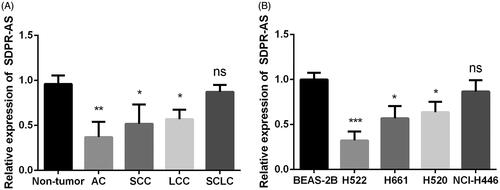
Under-expression of SDPR-AS promotes NSCLC cell proliferation and inhibites cell apoptosis
To further grab the impact of SDPR-AS in NSCLC cells, H522, H661 and H520 cells were transfected with the following plenty of vectors including pcDNA-SDPR-AS, sh-SDPR-AS or their corresponding controls. SDPR-AS was confirmed to be successfully overexpressed and knocked down in H522, H661 and H520 cells, respectively (). The highly expressed SDPR-AS significantly inhibited H522 cell viability and colony forming ability and promoted cell apoptosis (p < .05), whereas depression of SDPR-AS exhibited opposite effects (). Consistent results about the effects of SDPR-AS dysregulation on cell viability, colony forming ability and apoptosis were obtained in H661 and H520 cells ().
Figure 2. Down-regulation of SDPR-AS promoted NSCLC cell proliferation and inhibited cell apoptosis. NSCLC cell lines H522, H661 and H520 were transfected with pcDNA-SDPR-AS, sh-SDPR-AS or their corresponding controls. (A) SDPR-AS was confirmed to be successfully overexpressed and knocked down in H522, H661 and H520 cells. (B) Effects of SDPR-AS dysregulation on H522 cell viability, colony forming ability and apoptosis. (C) Effects of SDPR-AS dysregulation on H661 cell viability, colony forming ability and apoptosis. (D) Effects of SDPR-AS dysregulation on H520 cell viability, colony forming ability and apoptosis. Data were presented as mean ± standard deviation. *p<.05, **p<.01 and ***p<.01 compared with corresponding controls.
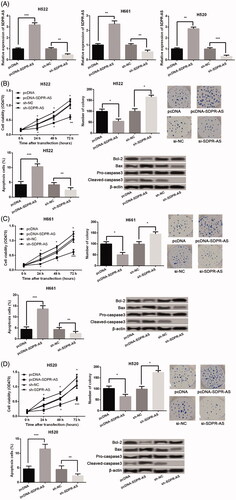
Under-expression of SDPR-AS promotes NSCLC cell migration and invasion
The effects of SDPR-AS dysregulation on NSCLC cell migration and invasion were also observed. The results uncovered that high level of SDPR-AS significantly depressed the migration and invasion of H522 (), H661 () and H520 () cells (p < .05), whereas depression of SDPR-AS exhibited opposite effects in these cells.
SDPR is positively regulated by SDPR-AS
It is reported that SDPR-AS and SDPR are positively associated with cell process of renal cell carcinoma cells [Citation12]. We thus detected the expression levels of SDPR in different subtypes of lung cancer tissues and cells. The results showed that, compared with adjacent non-rumor tissues and normal lung epithelial of BEAS-2B cells, the SDPR expressions were also significantly down-regulated in AC, SCC and LCC tissues and cells (p < .05), but not exhibited significant changes in SCLC tissues and cells (). Moreover, SDPR expression was also significantly up-regulated in pcDNA-SDPR-AS transfected H522, H661 and H520 cells and markedly down-regulated in sh-SDPR-AS transfected (p < .05, ). We thereby deducted that SDPR expression was positively correlated with SDPR-AS expression in NSCLC cells.
Figure 4. The expression of SDPR was positively regulated by SDPR-AS. (A) The expression of SDPR in AC, SCC, LCC, SCLC and non-tumor tissues. (B) The expression of SDPR in the human AC cell line H522, LCC cell line H661, SCC cell line H520, SCLC cell line NCI-H446 and normal lung epithelial cell line BEAS-2B. (C) The expression of SDPR in NSCLC cell lines H522, H661 and H520 that were transfected with pcDNA-SDPR-AS, sh-SDPR-AS or their corresponding controls. Data were presented as mean ± standard deviation. *p < .05, **p < .01 and ***p < .01 compared with corresponding controls.
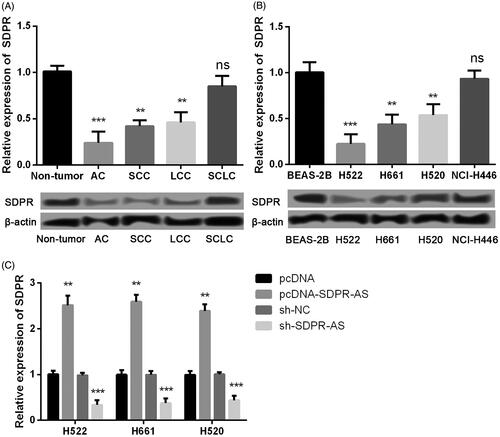
Impacts of SDPR-AS on NSCLC cells is through regulation of SDPR
To further investigated whether effects of SDPR-AS on the cell biological processes of NSCLC cells were through regulation of SDPR, SDPR was suppression in H522 cells by cell transfection. The transfection efficiency of SDPR knockdown was confirmed by qRT-PCR (p < .001, ). H522 cells were then cotransfection with si-SDPR and pcDNA-SDPR-AS. The results uncovered that depression of SDPR could significantly reverse the effects of SDPR-AS overexpression on H522 cell viability, colony forming ability, apoptosis, migration and invasion (). These findings implying that impacts of SDPR-AS on the malignant behaviors of NSCLC cells may be through regulation of SDPR.
Figure 5. Effects of SDPR-AS on NSCLC cells were through regulation of SDPR. (A) The transfection efficiency of SDPR knockdown in H522 cells that were transfected with si-SDPR and si-NC. (B–F) The combined effects of SDPR-AS overexpression and SDPR knockdown on H522 cell viability, colony forming ability, apoptosis, migration and invasion. (G) The expression of p38 MAPK/ERK signaling pathway-related proteins in H522 cells that were transfected with pcDNA-SDPR-AS, pcDNA, si-NC and/or si-SDPR. Data were presented as mean ± standard deviation. *p < .05, **p < .01 and ***p < .01 compared with corresponding controls.
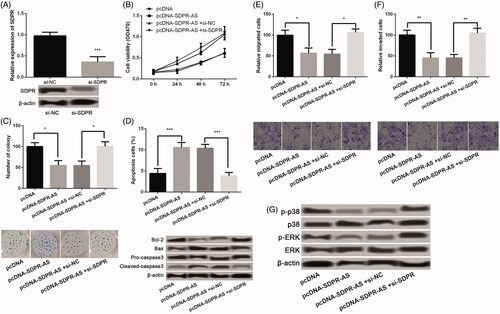
Role of SDPR-AS in NSCLC might be mediated by p38 MAPK/ERK signaling pathway
Previous studies have highlighted the key roles of p38 MAPK/ERK signaling pathway in the development of lung cancer [Citation14,Citation15]. The regulatory relationship between SDPR-AS and p38 MAPK/ERK signaling pathway was then investigated. Data displayed that the levels of p-p38 and p-ERK were dramatically depressed in the pc-SDPR-AS group relative to the pcDNA3.1 group, which were markedly reversed in H522 cells cotransfected with pc-SDPR-AS and si-SDPR (). These data indicated that SDPR-AS might play a pivotal impact in NSCLC through regulating p38 MAPK/ERK signaling pathway.
Discussion
The present research explored the possible impact of SDPR-AS in different subtypes of lung cancer. The results uncovered that SDPR-AS was under-expressed in NSCLC tissues and cells (H522, H661 and H520), but had no changes in SCLC tissues and cells. Down-regulation of SDPR-AS accelerated the proliferation, migration and invasion NSCLC cells, but inhibited the apoptosis of these cells. Overexpression of SDPR-AS exhibited opposite effects. Moreover, SDPR expression was positively regulated by SDPR-AS and effects of SDPR-AS on the malignant behaviors of NSCLC cellswas through regulation of SDPR. Besides, the levels of p-p38 and p-ERK were significantly decreased after SDPR-AS overexpression, which was markedly changeover by SDPR suppression simultaneously, indicating that p38 MAPK/ERK signaling pathway may be a key mechanism to mediate the impact of SDPR-AS in NSCLC development.
In previous studies, lncRNAs have been pointed out to act as certain critical regulators in the process of lung cancer [Citation17,Citation18]. For instance, lncRNA ANRIL knockdown can inhibit NSCLC cell proliferation and promote cell apoptosis by regulating KLF2 and P21 [Citation19]; lncRNA MEG3 can impair NSCLC cells proliferation and enhance apoptosis by altering p53 [Citation20]; and lncRNA GAS5 functions as a tumor depressor in NSCLC by declining tumor cell growth arrest and inducing apoptosis [Citation21]. In our study, lncRNA SDPR-AS was lowly expressed in NSCLC tissues and cells and depression of SDPR-AS promoted NSCLC cell proliferation, migration and invasion and inhibited apoptosis. Consistent with the findings that overexpression of SDPR-AS can inhibit RCC cell migration and invasion [Citation12], we speculate that SDPR-AS may function as a tumor depressor in NSCLC.
Furthermore, our results showed that SDPR expression was positively regulated by SDPR-AS. SDPR has been found to be expressed in plenty of cellular types and was under-expressed in several cancers, such as prostate, breast, kidney, gastric, and oral cancer [Citation22,Citation23]. In breast cancer, SDPR is found to function as a metastasis suppressor through promoting apoptosis [Citation24]. Moreover, SDPR levels are related to tumor differentiation and TNM stage in hepatocellular carcinoma [Citation25]. In our study, knockdown of SDPR could significantly reverse the effects of SDPR-AS overexpression on H522 cell viability, colony forming ability, apoptosis, migration and invasion. Considering the key impact of SDPR in cancer development, we speculate that the impacts of SDPR-AS on the cell biological processes of NSCLC cells may be through regulation of SDPR.
Furthermore, MAPK/ERK signaling pathway is pointed out to regulate cell proliferation, migration, and apoptosis [Citation26]. ERK/p38 MAPK proteins are found to be involved in CCL22 induced cell migration and invasion in lung cancer [Citation27]. In addition, ETS1-Associated Protein II (EAPII) is shown to play a tumor-enhanced impact in regulating lung cancer development through activating the MAPK–ERK pathway [Citation28]. Matrine can induce caspase-dependent apoptosis in NSCLC cells by promoting ROS generation to activate p38 [Citation29]. Moreover, it is reported the ERK signal can be attenuated frequently in SDPR-overexpressed oral squamous cell carcinomas [Citation23]. Our data uncovered that the levels of p-p38 and p-ERK were significantly decreased after SDPR-AS overexpression, which was dramatically changeover by si-SDPR simultaneously. Hence, we deducted that SDPR-AS may be crucial in NSCLC development via regulating SDPR to activate p38 MAPK/ERK signaling pathway.
Taken together, our data indicate that SDPR-AS was lowly expressed in NSCLC cells and down-regulation of SDPR-AS may promote the malignant behaviors of NSCLC cells possible through regulating SDPR expression and involving in p38 MAPK/ERK signaling pathway. SDPR-AS may serve as a promising target for NSCLC diagnosis and therapy. However, this research only grabbed the correlations among lncRNA SDPR-AS vs. SDPR vs. p38 MAPK/ERK in NSCLC in cell lines, our research team plan to deeply explore the comprehensive performances of the above interactions both in animal and in the clinical level in the future.
Disclosure statement
No potential conflict of interest was reported by the authors.
References
- Meaney CL, Zingone A, Brown D, et al. Identification of serum inflammatory markers as classifiers of lung cancer mortality for stage I adenocarcinoma. Oncotarget. 2017;8:40946–40957.
- Sève P, Reiman T, Dumontet C. The role of betaIII tubulin in predicting chemoresistance in non-small cell lung cancer. Lung Cancer. 2010;67:136–143.
- Herbst RS, Heymach JV, Lippman SM. Lung cancer. N Engl J Med. 2008;359:1367–1380.
- Sun M, Liu XH, Lu KH, et al. EZH2-mediated epigenetic suppression of long noncoding RNA SPRY4-IT1 promotes NSCLC cell proliferation and metastasis by affecting the epithelial-mesenchymal transition. Cell Death Dis. 2014;5:e1298–e1298.
- Thomson CS, Forman D. Cancer survival in England and the influence of early diagnosis: what can we learn from recent EUROCARE results? Br J Cancer. 2009;101:2S102–9.
- Mercer TR, Dinger ME, Mattick JS. Long non-coding RNAs: insights into functions. Nat Rev Genet. 2009;10:155
- Yang G, Lu X, Yuan L. LncRNA: a link between RNA and cancer. Biochim Biophys Acta. 2014;1839:1097–1109.
- Nie W, Ge H-j, Yang X-q, et al. LncRNA-UCA1 exerts oncogenic functions in non-small cell lung cancer by targeting miR-193a-3p. Cancer Lett. 2016;371:99–106.
- Yang Y-R, Zang S-Z, Zhong C-L, et al. Increased expression of the lncRNA PVT1 promotes tumorigenesis in non-small cell lung cancer. Int J Clin Exp Pathol. 2014;7:6929.
- Wan L, Sun M, Liu G-J, et al. Long noncoding RNA PVT1 promotes non–small cell lung cancer cell proliferation through epigenetically regulating LATS2 expression. Mol Cancer Ther. 2016;15:1082–1094.
- Qiu M, Xu Y, Yang X, et al. CCAT2 is a lung adenocarcinoma-specific long non-coding RNA and promotes invasion of non-small cell lung cancer. Tumor Biol. 2014;35:5375–5380.
- Ni W, Song E, Gong M, et al. Downregulation of lncRNA SDPR-AS is associated with poor prognosis in renal cell carcinoma. Onco Targets Ther. 2017;10:3039–3047.
- Preker P, Almvig K, Christensen MS, et al. PROMoter uPstream transcripts share characteristics with mRNAs and are produced upstream of all three major types of mammalian promoters. Nucleic Acids Research. 2011;39:7179–7193.
- Chung LY, Tang SJ, Sun GH, et al. Galectin-1 promotes lung cancer progression and chemoresistance by upregulating p38 MAPK, ERK, and cyclooxygenase-2. Clin Cancer Res. 2012;18:4037.
- Chen KH, Weng MS, Lin JK. Tangeretin suppresses IL-1beta-induced cyclooxygenase (COX)-2 expression through inhibition of p38 MAPK, JNK, and AKT activation in human lung carcinoma cells. Biochem Pharmacol. 2007;73:215.
- Kleihues P, Cavenee W. World Health Organization classification of tumours. Pathology and genetics of tumours of the nervous system. Lyon: IARC; 2000.
- Yang J, Lin J, Liu T, et al. Analysis of lncRNA expression profiles in non-small cell lung cancers (NSCLC) and their clinical subtypes. Lung Cancer. 2014;85:110–115.
- Hu X, Bao J, Wang Z, et al. The plasma lncRNA acting as fingerprint in non-small-cell lung cancer. Tumour Biol. 2016;37:3497–3504.
- Nie F-q, Sun M, Yang J-s, et al. Long noncoding RNA ANRIL promotes non–small cell lung cancer cell proliferation and inhibits apoptosis by silencing KLF2 and P21 expression. Mol Cancer Ther. 2015;14:268–277.
- Lu K-h, Li W, Liu X-h, et al. Long non-coding RNA MEG3 inhibits NSCLC cells proliferation and induces apoptosis by affecting p53 expression. BMC Cancer. 2013;13:461.
- Shi X, Sun M, Liu H, et al. A critical role for the long non‐coding RNA GAS5 in proliferation and apoptosis in non‐small‐cell lung cancer. Mol Carcinog. 2015;54:E1.
- Li X, Jia Z, Shen Y, et al. Coordinate suppression of Sdpr and Fhl1 expression in tumors of the breast, kidney, and prostate. Cancer Sci. 2008;99:1326–1333.
- Unozawa M, Kasamatsu A, Higo M, et al. Cavin‐2 in oral cancer: a potential predictor for tumor progression. Mol Carcinog. 2016;55:1037–1047.
- Ozturk S, Papageorgis P, Wong CK, et al. SDPR functions as a metastasis suppressor in breast cancer by promoting apoptosis. Proc Natl Acad Sci USA. 2016;113:638–643.
- Jing W, Luo P, Zhu M, et al. Prognostic and diagnostic significance of SDPR-Cavin-2 in hepatocellular carcinoma. Cell Physiol Biochem. 2016;39:950–960.
- Sun Y, Liu W-Z, Liu T, et al. Signaling pathway of MAPK/ERK in cell proliferation, differentiation, migration, senescence and apoptosis. J Recept Signal Transduct. 2015;35:600–604.
- Guan C, Lou Y, Zhao K. Express of ERK/p38MAPK protein in lung cancer cell migration and invasion induced by chemokine CCL22. Zhejiang Med J. 2014;
- Li C, Fan S, Owonikoko T, et al. Oncogenic role of EAPII in lung cancer development and its activation of the MAPK-ERK pathway. Oncogene. 2011;30(35):3802–3812.
- Tan C, Qian X, Jia R, et al. Matrine induction of reactive oxygen species activates p38 leading to caspase-dependent cell apoptosis in non-small cell lung cancer cells. Oncol Rep. 2013;30:2529–2535.