Abstract
Osteoarthritis (OA) is a major public health concern for which a reliable non-invasive treatment option has yet to be developed. In the present study, we investigated the effects of saxagliptin, a novel dipeptidyl peptidase IV (DPP-4) inhibitor, on several important aspects of the pathophysiology of OA using primary human chondrocytes. The results of real-time PCR and ELISA analyses show that saxagliptin treatment significantly decreased mRNA and protein expression of three key cartilage degrading enzymes: matrix metalloproteinase (MMP)-1, MMP-3, and MMP-13. The results of western blot confirmed that this decrease in MMP-1, -3, and -13 expression prevented degradation of type II collagen. We also found that saxagliptin significantly inhibited expression of a disintegrin and metalloproteinase with thrombospondin motif (ADAMTS)-4 and ADAMTS-5, which was reflected by markedly decreased degradation of aggrecan. Inhibition of DPP-4 by saxagliptin also reduced oxidative stress in human primary chondrocytes as evidenced by decreased production of reactive oxygen species (ROS) and increased glutathione (GSH) levels. Additionally, the results of western blot analysis show that the effects of saxagliptin are mediated through the p38/IκBα/NF-κB pathway, which is considered an important treatment target for OA. These findings suggest a potential role for saxagliptin as a novel treatment against OA.
Introduction
As medical therapies continue to advance, the average age of the global population increases at a phenomenal rate. However, there are still many factors of ageing and age-related diseases of which we have limited understanding. One of the most prominent of these is osteoarthritis (OA). While many factors contribute to the development and progression of OA, such as mechanical loading, injury, and other inflammatory diseases, age is recognized as the main risk factor [Citation1]. In part, this is due to the accumulation of advanced glycation end products (AGEs) in cartilage tissue. AGEs are the result of non-enzymatic protein glycation and are extremely resilient to degradation. Chondrocytes have been shown to express the receptor for AGEs (RAGE), indicating the involvement of AGEs in chondrocyte regeneration and cartilage remodelling [Citation2]. However, increased activation of RAGE on chondrocytes by AGEs has been shown to induce cartilage degradation by shifting cartilage metabolism and increasing catabolic activity [Citation3]. Accumulation of AGEs has also been shown to promote chronic inflammation by triggering the production of reactive oxygen species (ROS), expression of tumour necrosis factor-α (TNF-α) and other cytokines, and activation of nuclear factor-κ B (NF-κB) [Citation4]. While production of ROS occurs in normal physiology, AGEs directly influence the generation of ROS via catalytic sites in their molecular structure [Citation5]. Excessive production of ROS disrupts the balance between oxidative and antioxidative factors, thereby disturbing cartilage homeostasis and promoting excessive degradation of type II collagen and aggrecan, the main constituents of the articular extracellular matrix (ECM). In OA, production of ROS and proinflammatory cytokines induced by AGEs creates a positive feedback loop in which oxidative stress induced by increased ROS leads to further generation of ROS and expression of cytokines, thereby creating a sustained oxidative stress environment, disrupting cellular signalling, and driving disease progress [Citation6]. Furthermore, AGE-induced generation of ROS and proinflammatory cytokines contribute to the development of the senescence-associated secretory phenotype (SASP) of chondrocytes, which has been shown to play a role in numerous OA-related events, including recruitment of immune cells, alteration of the tissue microenvironment, and induction of malignant phenotypes [Citation7].
Type II collagen is rigid in nature and has a very slow rate of turnover in healthy tissue, while aggrecan, which undergoes degradation and regeneration at a more rapid pace, provides cartilage with its shock-absorptive property [Citation8]. Excessive degradation of type II collagen and aggrecan by collagenases (matrix metalloproteinases (MMPs)) and aggrecanases (a disintegrin and metalloproteinase with thrombospondin type I motif (ADAMTS)), is recognized as a pivotal event in the development of OA [Citation9]. In particular, MMP-1, MMP-3 and MMP-13 have been shown to significantly induce degradation of type II collagen, both in vitro and in in vivo animal model experiments [Citation10–12]. Among the members of the ADAMTS family, ADAMTS-4 and ADAMTS-5 have been shown to greatly induce degradation of aggrecan [Citation13,Citation14]. Previous studies have shown that accumulation of AGEs can lead to increased expression of MMPs and ADAMTS [Citation15,Citation16]. Inhibition of MMPs and ADAMTS has been considered as a strategy for preventing degradation of type II collagen and aggrecan in the articular ECM. However, the exact mechanisms through which the expression of MMPs and ADAMTS is upregulated in OA remain to be fully elucidated. Another potential strategy involves mediating activation of proinflammatory cellular signalling pathways. Expression of proinflammatory cytokines has been shown to be enhanced via phosphorylation of MAPK p38 and activation of the NF-κB pathway through RAGE [Citation17].
In the present study, we investigated the effects of saxagliptin, a dipeptidyl peptidase-4 (DPP-4) inhibitor approved by the U.S. Food and Drug Administration (FDA) for the treatment of type II diabetes, on degradation of type II collagen and aggrecan in human primary chondrocytes [Citation18]. While concern has been raised regarding a possible connection between the use of DPP-4 inhibitors and the development of arthralgia, the chemical structures of drugs in this class vary widely and there is still no solid evidence regarding if or how inhibition of DPP-4 might negatively influence cartilage integrity [Citation19]. Additionally, recent studies have suggested that glucagon-like peptide-1 (GLP-1), which is degraded by DPP-4, may exert beneficial effects by influencing bone formation and remodelling, preventing bone resorption, and guiding osteoblast differentiation, thus implying that preservation of endogenous GLP-1 via inhibition of DPP-4 may play a role in attenuating the effects of OA [Citation20–22]. Therefore, we endeavoured to elucidate the effects of the novel DPP-4 inhibitor saxagliptin in OA by demonstrating its influence on AGE-induced oxidative stress, degradation of articular cartilage matrix by MMPs and ADAMTS, and activation of the p38/MAPK/NF-κB signalling pathway. Our results indicate that saxagliptin may indeed have promise as a safe and effective therapy against the development and progression of OA.
Materials and methods
Cell isolation, culture and treatment
Human samples were used in accordance with the World Medical Association Declaration of Helsinki Ethical Principles for Medical Research Involving Human Subjects. Written informed consent was signed by all participants. Primary chondrocytes were isolated from specimens of cartilage explants as described previously [Citation23]. Isolated primary chondrocytes were maintained in DMEM supplemented with 10% of FBS and 1% of penicillin/streptomycin. Cells were treated with 100 μg/ml AGEs in the presence or absence of 0.5 and 1 μM saxagliptin for various times.
Quantitative real-time polymerase chain reaction (PCR)
RNA was extracted from chondrocytes using TRIzol reagent (Invitrogen Life Technologies, USA). Reverse transcription PCR (RT-PCR) was applied to synthesize cDNA using purified RNA (1 μg), random primers (5 μM), dNTP (1 mM), and AMV reverse transcriptase (20 units) in reaction buffer (Promega, USA). Real-time PCR experiments were performed in a 20 μL reaction system containing cDNA (1 μL), upstream and downstream primers (1 μL, respectively), Power SYBR Green PCR Master Mix (10 μL), and PCR grade water (7 μL) on a StepOne Plus Real-time PCR system (Applied Biosystems, USA). The relative levels of target genes were calculated by normalizing to β-actin using the 2(−ΔΔCT) method.
Western blot analysis
To prepare protein extracts, the cells were lysed using cell lysis buffer supplemented with protease inhibitor cocktail. Protein concentrations were determined using a commercial bicinchoninic acid (BCA) kit (Thermo Fisher Scientific, USA). Equal amounts of samples in loading buffer were subjected to 8–12% polyacrylamide gel electrophoresis. Separated samples were then transferred to polyvinylidene difluoride (PVDF) membranes. After blocking with 5% skim milk at room temperature (RT) for 1 h, membranes were sequentially incubated with primary antibodies overnight in a cold room and HRP-conjugated secondary antibodies for 2 h at RT. After washing three times with TBST, blots were visualized using an enhanced chemiluminescence reagent kit (Thermo Fisher Scientific, USA).
Extraction of nuclear protein
To determine nuclear translocation of p65, nuclear extracts were prepared using a nuclear and cytoplasmic extraction kit (Thermo Scientific, USA) in accordance with the manufacturer’s instructions. The levels of nuclear p65 were assessed using western blot analysis with lamin B as an internal control.
Measurement of intracellular ROS
Total ROS in chondrocytes were assessed using a DCFH-DA assay. Chondrocytes were treated with 100 μg/ml AGEs in the presence or absence of 0.5 and 1 μM saxagliptin for 24 h. After washing three times with PBS, cells were loaded with 10 μM DCFH-DA in serum-free medium and incubated for 1 h at 37 °C. Fluorescent signals were then visualized using a fluorescence microscope.
ELISA assay
Human primary chondrocytes (HPCs) were seeded into 96-well plates at a density of 1 × 104 cells per well. After 12 h, cells were treated with 100 μg/ml AGEs in the presence or absence of 0.5 and 1 μM saxagliptin for 24 h. Cell supernatants were collected and used for measuring MMP-1, MMP-3, and MMP-13 by ELISA assay using commercial kits from Invitrogen (human MMP-1, Cat# EHMMP1; human MMP-3, Cat# BMS2014-3, human MMP-13, Cat# EHMMP13) in accordance with the manufacturer’s instructions.
Determination of intracellular reduced glutathione (GSH)
Human primary chondrocytes were treated with 100 μg/ml AGEs in the presence or absence of 0.5 and 1 μM saxagliptin for 24 h. Then, cells were collected with ice cold 5% metaphosphoric acid (MPA) (Sigma-Aldrich, USA). After centrifugation at 12,000 rpm for 5 min, supernatant was harvested and incubated with OPAME in methanol and potassium tetraborate borate buffer for 15 min at RT. At the end of the reaction, the results were read on a SpectraMax M2e instrument (Molecular Devices, USA) with excitation at a wavelength of 350 nm.
Luciferase reporter assay
The transcriptional activity of NF-κB was assessed using a luciferase reporter assay. Briefly, 0.5 mg NF-κB promoter luciferase plasmid and an equal amount of renilla-luciferase plasmid were transfected into cells using lipofectamine 2000 (Invitrogen, USA). At 24 h after transfection, cells were treated with 100 μg/ml AGEs in the presence or absence of 0.5 and 1 μM saxagliptin for 24 h. Cells were then lysed and centrifugated at 500×g. The supernatant was collected and used to analyze the firefly and renilla luciferase activities using the Dual-luciferase Reporter Assay System (Promega) in a luminometer.
Statistical analysis
All data are shown as the mean ± standard deviation (SD). Statistical analysis was performed with GraphPad Prism (Version 5.01). ANOVA was used to analyze data. P values less than 0.05 are regarded as statistically significant.
Results
AGE-induced expression of MMP-1/3/13 and degradation of type II collagen
First, we determined the effects of treatment with saxagliptin on the expression of MMPs and subsequent degradation of type II collagen in chondrocytes exposed to AGEs. As shown in , 100 µg/ml AGEs significantly upregulated expression of MMP-1, MMP-3 and MMP-13 at both the mRNA and protein levels (, respectively). However, 0.5 and 1 µM saxagliptin treatment successfully suppressed AGE-induced increased expression of MMP-1, MMP-3, and MMP-13 in a dose-dependent manner. Importantly, western blot analysis revealed that saxagliptin treatment successfully rescued AGE-induced degradation of type II collagen in chondrocytes ().
Figure 1. Saxagliptin inhibited expression of MMP-1, MMP-3, MMP-13. Primary human chondrocytes were treated with 100 μg/ml AGEs in the presence or absence of 0.5 and 1 μM saxagliptin for 24 h. (A) Gene expressions of MMP-1, MMP-3, and MMP-13 were determined by real-time PCR analysis; (B) Protein expressions of MMP-1, MMP-3, and MMP-13 was determined by ELISA (*, #, $, p < .01 vs. previous column group).
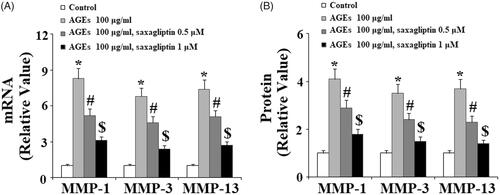
AGE-induced expression of ADAMTS-4/5 and degradation of aggrecan
Next, we determined the effects of saxagliptin treatment on ADAMTS-mediated AGE-induced degradation of aggrecan in HPCs. As demonstrated by the results of real-time PCR and western blot analysis, 100 µg/ml AGEs significantly upregulated expression of ADAMTS-4 and ADAMTS-5 at both the mRNA and protein levels (, respectively). Using β-actin as a control, we found that 0.5 and 1 µM saxagliptin successfully rescued AGE-induced increased expression of ADMATS-4 and ADAMTS-5 in a dose-dependent manner. Concordantly, we also confirmed that 0.5 and 1 µM saxagliptin ameliorated degradation of aggrecan in HPCs induced by exposure to 100 µg/ml AGEs ().
Figure 3. Saxagliptin inhibited expression of ADAMTS-4 and ADAMTS-5. Primary human chondrocytes were treated with 100 μg/ml AGEs in the presence or absence of 0.5 and 1 μM saxagliptin for 24 h. (A) mRNA expression of ADAMTS-4 and ADAMTS-5 determined by real-time PCR; (B) Protein expression of ADAMTS-4 and ADAMTS-5 determined by western blot analysis (*, #, $, p < .01 vs. previous column group).
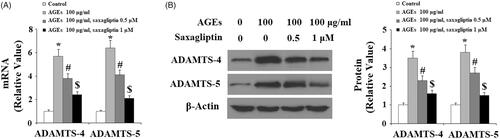
AGE-induced production of ROS
To investigate the effects of DPP-4 inhibition on AGE-induced oxidative stress, we first confirmed via DCFH-DA staining that treatment with 100 µg/ml AGEs led to a significant increase in the level of ROS in HPCs. This increase in ROS was ameliorated by 0.5 and 1 µM saxagliptin in a dose-dependent manner (). We also investigated the effects of saxagliptin on the level of the antioxidant glutathione (GSH) in HPCs exposed to 100 µg/ml AGEs. As shown in , exposure to AGEs decreased the level of GSH by half, which was rescued by treatment with 0.5 and 100 µM saxagliptin in a dose-dependent manner. These results demonstrate that saxagliptin may be beneficial for restoring the balance between oxidants and anti-oxidants in patients with OA.
AGE-induced activation of p38, IκBα, and NF-κB
Finally, we determined the effects of saxagliptin on phosphorylation of p38 in HPCs exposed to AGEs. As shown by the results of western blot analysis in , the two doses of saxagliptin successfully rescued the increase in phosphorylation of p38 induced by AGEs in a dose-dependent manner, while total p38 remained constant. To determine the effects of saxagliptin on phosphorylation of IκBα, using β-actin as a control, we exposed HPCs to 100 µg/ml AGEs in the presence or absence of saxagliptin for 6 h. As shown in , exposure to AGEs caused a significant increase in total IκBα and a significant decrease in phosphorylated IκBα, both of which were ameliorated by 0.5 and 1 µM saxagliptin in a dose-dependent manner. To investigate the effects of AGEs on activation of NF-κB, we measured nuclear translocation of p65 and luciferase activity of NF-κB in HPCs exposed to 100 µg/ml AGEs for 24 h in the presence or absence of saxagliptin. Concordant with our finding that AGEs increased phosphorylation of IκBα, the results in show that exposure to AGEs significantly increased nuclear translocation of p65 and subsequent activation of NF-κB, which was ameliorated by treatment with saxagliptin in a dose-dependent manner.
Figure 6. Saxagliptin inhibited phosphorylation of p38. Primary human chondrocytes were treated with 100 μg/ml AGEs in the presence or absence of 0.5 and 1 μM saxagliptin for 2 h. Phosphorylated and total p38 were determined by western blot analysis (*, #, $, p < .01 vs. previous column group).
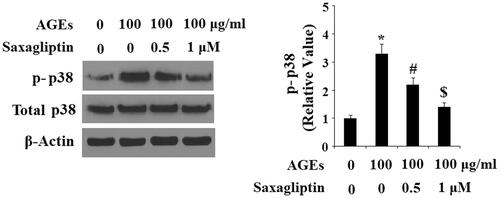
Figure 7. Saxagliptin inhibited phosphorylation and degradation of IκBα. Primary human chondrocytes were treated with 100 μg/ml AGEs in the presence or absence of 0.5 and 1 μM saxagliptin for 6 h. Phosphorylated and total IκBα were determined by western blot analysis (*, #, $, p < .01 vs. previous column group).
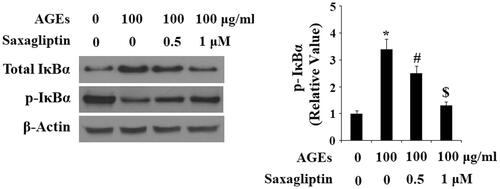
Discussion
As one of the greatest immediate threats to the health of the ageing global population, OA has been receiving increasing attention in recent decades. However, a safe and effective therapy against the development and progression of the disease has remained elusive. In the present study, we employed the specific DPP-4 inhibitor saxagliptin in the treatment of primary articular chondrocytes exposed to insult from AGEs to investigate the involvement of DPP-4 inhibition in the pathological processes driving excessive degradation of type II collagen and aggrecan in the articular ECM. While there are numerous causative factors behind the development of OA, including excessive mechanical loading, injury, and concurrent disease, ageing is regarded as the main risk factor [Citation24]. In part, this is due to the accumulation of AGEs in cartilage. AGEs are highly resilient to degradation and come to exist in the body both from external sources, such as through diet and as the result of the internal process of non-enzymatic glycation [Citation25]. While the effects of AGEs in type II diabetes have been studied somewhat extensively, the role of AGEs in OA has not been explored as thoroughly. As demonstrated by the results of this study, assault from treatment with 100 µg/ml AGEs exerted deleterious effects on the integrity of the articular ECM via upregulation of degradative enzymes that target type II collagen and aggrecan (MMPs and ADAMTS, respectively), production of ROS, and activation of proinflammatory cellular signaling pathways including the MAPK p38/NF-κB pathway. Remarkably, treatment with saxagliptin significantly ameliorated these effects in a dose-dependent manner.
Type II collagen and aggrecan, the two main components of the ECM, are degraded by MMPs and ADAMTS, respectively. Of these, MMP-1 (collagenase-1), MMP-3 (stromelysin), and MMP-13 (collagenase-3) have been shown to play key roles in OA, diabetes, and other chronic inflammatory diseases. MMP-1, which cleaves various types of matrix proteins, is activated by MMP-3 [Citation11,Citation24,Citation25]. ADAMTS-4 and ADAMTS-5 have been shown to be the major catabolic enzymes that cleave bonds at the Glu373, Glu1545, Glu1714, Glu1819 and Glu1919 sites in aggrecan. Cleavage of these sites, along with degradation of type II collagen, significantly compromises the structural integrity of the ECM in cartilage, thereby triggering irreversible joint destruction [Citation26,Citation27]. However, the exact mechanisms through which this process occurs is complicated and remains poorly understood. As demonstrated in the present study, accumulation of AGEs in cartilage tissue induces increased expression of MMPs and ADAMTS, followed by excessive degradation of type II collagen and aggrecan in the articular ECM. Inhibition of DPP-4 by saxagliptin was able to prevent AGE-induced upregulation of MMPs and ADAMTS as well as degradation of type II collagen and aggrecan, thereby implying the potential of DPP-4 as a therapeutic target in OA ().
Oxidative stress is well-recognized as a major event in the development of OA and other diseases. Under normal physiological conditions, homeostasis requires a balance between oxidants, such as ROS, and anti-oxidants, including GSH, to maintain a suitable rate of cell turnover [Citation28]. In OA, however, this balance is disturbed, resulting in sustained overproduction of ROS. As shown in this study and others, the accumulation of AGEs leads to excessive generation of ROS and a decrease in expression of GSH. This causes an oxidative stress environment to develop, which in turn further upregulates the production of ROS [Citation29]. Here, we found that while exposure to AGEs significantly upregulated generation of ROS and suppressed GSH levels, these effects were rescued by treatment with saxagliptin in a dose-dependent manner, implying the potential benefit of inhibition of DPP-4 by saxagliptin in the treatment of OA as well as other inflammatory diseases ().
Activation of the p38 MAPK signalling pathway through phosphorylation of p38 is a key event in the inflammatory response [Citation30]. The p38 signalling pathway has been shown to inhibit the kinase activity of protein kinase Cζ, which then activates NF-κB via nuclear translocation of p65 and leads to subsequent expression of MMPs, ADAMTS, and generation of ROS. The NF-κB transcription factor, which is normally sequestered by IκBα, is a major regulator of inflammatory cytokine production and cell survival. Phosphorylation and subsequent degradation of IκBα lead to activation of NF-κB, which has been implicated in the pathological process of OA [Citation31]. This inflammatory process has been implicated in a wide array of chronic inflammatory diseases including OA [Citation32–34]. Under normal physiological conditions, activation of NF-κB is inhibited by IκBα. However, as demonstrated by our findings, exposure to AGEs induces activation of the p38 MAPK signalling pathway and increases degradation of IκBα, thereby upregulating activation of NF-κB and promoting the inflammatory response in OA. Notably, saxagliptin treatment successfully prevented this pro-inflammatory process in a dose-dependent manner, thereby demonstrating the value of DPP-4 inhibition as a potential method to mediate activation of NF-κB ().
Importantly, inhibition of DPP-4 by saxagliptin can ameliorate the effects of accumulation of AGEs including upregulation of expression of MMPs and ADAMTS, increased degradation of type II collagen and aggrecan, generation of ROS, activation of the p38 MAPK signalling pathway, and downregulation of inhibition of NF-κB signalling by IκBα. Thus, treatment with saxagliptin may be able to restore the balance between oxidants and antioxidants, and suppress the inflammatory response, thereby mediating the harmful processes behind the development and progression of OA. Additional research is required to further elucidate the potential role of inhibition of DPP-4 in OA and other inflammatory diseases.
Disclosure statement
No potential conflict of interest was reported by the authors.
Additional information
Funding
References
- Fernandes JC, Martel-Pelletier J, Pelletier JP. The role of cytokines in osteoarthritis pathophysiology. Biorheology. 2002;39:237–246.
- Qu H, Li J, Wu L, et al. Trichostatin A increases the TIMP-1/MMP ratio to protect against osteoarthritis in an animal model of the disease. Mol Med Rep.2016;14: 2423–2430.
- Steenvoorden MM, Huizinga TW, Verzijl N, et al. Activation of receptor for advanced glycation end products in osteoarthritis leads to increased stimulation of chondrocytes and synoviocytes. Arthritis Rheum. 2006;54:253–263.
- Sun ZJ, Xia YY. Autophagy plays a protective role in advanced glycation end products-induced apoptosis of chondrocytes via regulation of tumor necrosis factor-α, nuclear factor-κ B and reactive oxygen species. Asian Pac J Trop Med. 2018;11:73.
- Forbes JM, Cooper ME, Oldfield MD, et al. 2003. Role of advanced glycation end products in diabetic nephropathy. J Am Soc Nephrol. 2003;14:S254–S258.
- Collins JA, Wood ST, Poole L, et al. The level of reactive oxygen species differentially regulates peroxiredoxin oxidation and mitogen-activated protein kinase signaling in human chondrocytes. Osteoarthritis Cartilage. 2016;24:S179–S180.
- Tchkonia T, Zhu Y, Van Deursen J, et al. Cellular senescence and the senescent secretory phenotype: therapeutic opportunities. J Clin Invest. 2013;123:966–972.
- Huang CY, Lai KY, Hung LF, et al. Advanced glycation end products cause collagen II reduction by activating Janus kinase/signal transducer and activator of transcription 3 pathway in porcine chondrocytes. Rheumatology (Oxford). 2011;50:1379–1389.
- Kapoor M, Martel-Pelletier J, Lajeunesse D, et al. Role of proinflammatory cytokines in the pathophysiology of osteoarthritis. Nat Rev Rheumatol. 2011;7:33–42.
- Poole AR, Kobayashi M, Yasuda T, et al. Type II collagen degradation and its regulation in articular cartilage in osteoarthritis. Ann Rheum Dis. 2002;61:ii78–ii81.
- Vincenti MP, Brinckerhoff CE. Transcriptional regulation of collagenase (MMP-1, MMP-13) genes in arthritis: integration of complex signaling pathways for the recruitment of gene-specific transcription factors. Arthritis Res. 2002;4:157–164.
- Neuhold LA, Killar L, Zhao W, et al. Postnatal expression in hyaline cartilage of constitutively active human collagenase-3 (MMP-13) induces osteoarthritis in mice. J CLIN Invest. 2001;107:35–44.
- Echtermeyer F, Bertrand J, Dreier R, et al. Syndecan-4 regulates ADAMTS-5 activation and cartilage breakdown in osteoarthritis. Nat Med. 2009;15:1072–1076.
- Verma P, Dalal K. ADAMTS-4 and ADAMTS-5: key enzymes in osteoarthritis. J Cell Biochem. 2011;112:3507–3514.
- Sourris KC, Forbes JM. Interactions between advanced glycation end-products (AGE) and their receptors in the development and progression of diabetic nephropathy-are these receptors valid therapeutic targets. CDT. 2009;10:42–50.
- Leong DJ, Sun HB. Events in articular chondrocytes with aging. Curr Osteoporos Rep. 2011;9:196.
- Qin YH, Dai SM, Tang GS, et al. HMGB1 enhances the proinflammatory activity of lipopolysaccharide by promoting the phosphorylation of MAPK p38 through receptor for advanced glycation end products. J Immunol. 2009;183:6244–6250.
- Traynor K. FDA approves saxagliptin for type 2 diabetes. Am J Health Syst Pharm. 2009;66:1513.
- Mascolo A, Rafaniello C, Sportiello L, et al. Dipeptidyl peptidase (DPP)-4 inhibitor-induced arthritis/arthralgia: a review of clinical cases. Drug Saf. 2016;39:401–407.
- Iepsen EW, Lundgren JR, Hartmann B, et al. GLP-1 receptor agonist treatment increases bone formation and prevents bone loss in weight-reduced obese women. J Clin Endocrinol Metab. 2015;100:2909–2917.
- Roth JD, Erickson MR, Chen S, et al. GLP-1R and amylin agonism in metabolic disease: complementary mechanisms and future opportunities. Br J Pharmacol. 2012;166:121–136.
- Chen J, Xie JJ, Shi KS, et al. Glucagon-like peptide-1 receptor regulates endoplasmic reticulum stress-induced apoptosis and the associated inflammatory response in chondrocytes and the progression of osteoarthritis in rat. Cell Death Dis. 2018;9:212.
- Capsoni F, Ongari AM, Lonati C, et al. α-Melanocyte-stimulating-hormone (α-MSH) modulates human chondrocyte activation induced by proinflammatory cytokines. BMC Musculoskelet Disord. 2015;16:154.
- Mahmoud RK, El-Ansary AK, El-Eishi HH. Matrix metalloproteinases MMP-3 and MMP-1 levels in sera and synovial fluids in patients with rheumatoid arthritis and osteoarthritis. Ital J Biochem 2005;54:248–257.
- Goldring MB, Otero M, Plumb DA, et al. Roles of inflammatory and anabolic cytokines in cartilage metabolism: signals and multiple effectors converge upon MMP-13 regulation in osteoarthritis. ECM. 2011;21:202–220.
- Nowotny K, Jung T, Höhn A, et al. Advanced glycation end products and oxidative stress in type 2 diabetes mellitus. Biomolecules. 2015;5:194–222.
- Song RH, Tortorella MD, Malfait AM, et al. Aggrecan degradation in human articular cartilage explants is mediated by both ADAMTS-4 and ADAMTS-5. Arthritis Rheum. 2007;56:575–585.
- Ott C, Jacobs K, Haucke E, et al. Role of advanced glycation end products in cellular signaling. Redox Biol. 2014;2:411–429.
- Yan SF, Ramasamy R, Schmidt AM. Mechanisms of disease: advanced glycation end-products and their receptor in inflammation and diabetes complications. Nat Rev Endocrinol. 2008;4:285–293.
- Cuenda A, Rousseau S. p38 MAP-kinases pathway regulation, function and role in human diseases. Biochim Biophys Acta. 2007;1773:1358–1375.
- Olivotto E, Otero M, Marcu KB, et al. Pathophysiology of osteoarthritis: canonical NF-κB/IKKβ-dependent and kinase-independent effects of IKKα in cartilage degradation and chondrocyte differentiation. RMD Open. 2015;1:e000061.
- Mohetaer M, Li G, Wang Y, et al. Protective effects of gemigliptin against type II collagen degradation in human chondrocytes. Biomed Pharmacother. 2018;104:590–594.
- Mengshol JA, Vincenti MP, Coon CI, et al. Interleukin-1 induction of collagenase 3 (matrix metalloproteinase 13) gene expression in chondrocytes requires p38, c-jun N-terminal kinase, and nuclear factor κB: Differential regulation of collagenase 1 and collagenase 3. Arthritis Rheum. 2000;43:801–811.
- Zandi E, Rothwarf DM, Delhase M, et al. The IkappaB kinase complex (IKK) contains two kinase subunits, IKKalpha and IKKbeta, necessary for IkappaB phosphorylation and NF-kappaB activation. Cell. 1997;91:243–252.