Abstract
Siberian ginseng, perennial herb belongs to Araliaceae family used in traditional medicines to treat hypertension, thrombus, inflammation and cancer. In the present study, we biosynthesized goldnanoparticles using Siberian ginseng aqeous extract in a cost effective manner. The synthesized Siberian ginseng gold nanoparticle (SG-GNPs) were characterized using UV-Vis spec, HR-TEM, XRD, FTIR, SAED analysis. UV-Vis spectroscopic analysis showed a surface Plasmon resonance peak at 538 nm which does not reduce till 30 days of incubation. The results of HR-TEM, XRD and SAED confirm the spherical shape, crystalline nature and the size of the synthesized gold nanoparticles. The FTIR results prove that the biological components present in the Siberian ginseng had reduced the gold ions to synthesis gold nanoparticles. After characterization, the efficacy of SG-GNPS against the melanoma, a deadliest skin carcinoma, was assessed in vitro using B16 murine melanoma cells. The CC50 dose of SG-GNPs against B16 cells were assessed with MTT assay and the anticancer activity was evaluated using Rhodamine 123, H2DCFDA and dual staining techniques. The induction of apoptosis by SG-GNPs against melanoma cells were confirmed with q-PCR analysis. The results of staining techniques prove that SG-GNPs increase the reactive oxygen species and decreased the mitochondrial membrane potential. It is further confirmed by the results of q-PCR analysis which shows increased apoptotic Bid, Bad, Casp3, Casp 9 genes and decreased antiapoptotic Bcl2 gene expression in SG-GNPs treated cells. Our results authentically prove the biosynthesized SG-GNPs induces apoptosis in melanoma cells and it possesses anticancer property.
Introduction
Nanotechnology is a fascinating field which has led to the discovery of various vital products in diverse fields like food, agriculture, environment, medicine, diagnosis, electronics etc. Nanotechnology along with biological sciences seems to be a boon in health care systems. The possibility to design nanoparticles in specific shape, composition and dimension enhances the advantages of nanoparticles in the field of pharmaceutics and diagnostics [Citation1–3]. However, the synthesis of nanoparticles uses toxic chemicals as reducing agents which lead to environmental pollution. Hence it is the need of today, to synthesize eco-friendly nanoparticles using an organic reducing agent instead of toxic inorganic reducing agents. Therefore, various researches were focused on using plants [Citation4], algae, fungi [Citation5] and bacteria [Citation6] as a reducing agent for the cost-effective synthesis of nanoparticles [Citation7]. Biosynthesized nanoparticles are much more potent than the chemically and physically synthesized nanoparticles since no toxic chemicals, heat or pressure were applied during the synthesis process.
The usage of metal nanoparticles in the field of medicine and diagnosis of diseases are drastically increased. Among all metal naoparticles, gold nanoparticles are considered to be more potent due to its unique optical, electronic properties and biocompatible nature increased its usage in the field of biomedicine field [Citation8–10]. Gold, possess inert nature and less toxicity and hence it is used as a drug in the traditional medicine to treat various diseases. The small size increases the cell penetration and drug delivery properties of gold naoparticles [Citation11], which makes it as potent candidate for targeted anticancer therapy [Citation12,Citation13]. Due to these distinctive properties, gold nanoparticles based drugs were assessed at the level of preclinical and clinical trials to treat cancer [Citation14].
Siberian ginseng is a perennial herb, which belongs to the family of Araliaceae aromatic flowering plants. In traditional medicine, Siberian ginseng is used as a drug to treat tumour, hypertension, inflammation, thrombus, diabetes, obesity and it also used as a laxative agent [Citation15–17]. Siberian ginseng is not only used as a drug but also consumed as a diet by Japanese, Asian and Chinese peoples. Chalcones, coumarins and flavanones are the major bioactive components present in Siberian ginseng apart from these polyacetylenes, triterpenes and cycohexenones were also present [Citation18]. Chalcones present in Siberian ginseng were proven to possess antitumor property against murine melanoma [Citation19]. Melanoma, deadliest form of cancer occurs in the skin, eye and inner part of the ear due to a genetic mutation in melanocytes (1–4,5). Various therapies like chemotherapy, radiotherapy, immunotherapy are available to treat melanoma but in most of the cases, it leads to severe side effects.
Hence in the present study, we synthesized a cost-effective nanobased phytodrug to treat melanoma. Gold nanoparticels were biosynthesized with aqueous extract of Siberian ginseng as an organic reducing agent. The synthesized drug was characterized using various techniques and assessed for the anticancer property against murine melanoma B16 cells which is potent in vitro model resembles the human skin carcinoma condition.
Materials and methods
Chemicals
Tetra chloroauric (III) acid trihydrate, sodium borohydride, potassium carbonate, tri-sodium citrate dehydrate, dimethylsulfoxide, Dulbecco's Modified Eagle's Medium, fetal bovine serum, trypsin, antibiotic-antimycotic solution, methylthiazolyl dipheytetrazolium bromide and all other fine chemical were bought from Sigma Aldrich, MO, USA. All the chemicals used for the present experiment were of high-quality grade. Stains Rhodamine 123, 2′,7′-dichlorofluorescein, acridine orange and ethidium bromide were purchased from Sigma Aldrich, USA Antibodies were purchased from Santa Cruz Biotech, Santa Cruz, CA, USA.
Biosynthesis of Siberian ginseng coated gold nanoparticles (AK-GNPs)
Preparation of Siberian ginseng ethanolic extract
The fresh healthy Siberian ginseng plants were obtained from the local farm was washed nicely with double distilled water and shadow dried until the water dries completely. Then, the 30 g of leaves and the stems of Siberian ginseng were cut into pieces and blended along with 30 ml of sterile double distilled water. Then, the mixture was made up to 50 ml with double distilled water and then centrifuged to remove ungrounded particles. The clear homogenous Siberian ginseng aqueous extract was further used to prepare SG-GNPs.
Siberian ginseng coated gold nanoparticles synthesis
SG-GNPs were biosynthesized by mixing 20 ml of 4 mM HAuCl4 solution to 1.5 ml of Siberian ginseng aqueous extract to incubate at 28˚C to reduce the Au3+ ions to Au0. After the incubation time, the mixture was centrifuged at 15000 rpm for 20 min. Finally, the pellet was resuspended in double-distilled water, then it was stored and used for further analysis. The synthesis of Siberian ginseng gold nanoparticles was observed through the colour change of the solution to dark red which further confirmed with UV spectroscopic analysis.
Characterization of SG-GNPs
UV-Visible spectroscopic analysis
The synthesized SG-GNPs were subjected to UV-Vis spectroscopic analysis at the different duration of time from 24 h– 30 days to analyze the stability of the AK-GNPs and their particle size. The sample is read at the different wavelength between 300 and 700 nm using Shiamudaz UV-Vis Spectrophotomete (Shimadzu, Japan). The samples were stored at 0 °C for the entire period of experiment and the samples fully thawed to room temperature before subjecting spectroscopic analysis.
Selected area diffraction (SAED) pattern analysis
SAED pattern analysis was performed to assess the structure of the synthesized SG-GNPs. The sample was filtered with Millipore filter and then analyzed with Zetasizer Nano ZSP (Malvern, UK). The SAED pattern was observed and the distance between two planes was calculated using the formula λL Rd = (1) where λL is a constant of the microscope, R is the ring radius, and d is the interplanar distance
Transmission electron microscopic (TEM) - XRD analysis
The morphology and the nature of the biosynthesized SG-GNPs were assessed with TEM and XRD analysis. 5 µl of SG-GNPs was placed on the copper grids coated with formvar and dried. The dried samples were then scanned at a voltage of 120 kV, in which the sample is subjected to a beam of electron and the image, was captured (JEOL 1200EX, JEOL, Tokyo, Japan). The captured image was assessed with Image J softwar (NIH, USA). X ray diffraction studies were carried out using XRD – 6000/6100 model XRD instrument.
Fourier transform infrared (FTIR) spectroscopy analysis
SG-GNPs were subjected to FTIR spectroscopic analysis to assess the functional groups present. SG-GNPs were lyophilized and 2 mg of lyophilized SG-GNPs powder is mixed with 200 mg of KBr and the mixture were placed on the FTIR sample holder. The samples were assessed at the spectroscopic range of 4000–5000 cm−1 (FTIR Spectrum 2000, Perkin Elmer, Connecticut, USA). About 50 scans at resolution of 4 cm per scan was performed and the data obtained were assessed using the software WINFIRST, Mattson, USA
Culturing of murine B16 melanoma cells
Murine Melanoma cells B16 which is used as renowned model for skin carcinogenic studies were used for the present study. B16 cells were procured from American Type Culture Collection (ATCC). The cells were cultured using Dulbecco’s Modified Eagle’s Medium supplemented with 10% fetal bovine serum and 1% antibiotic-antimycotic solution. The cells were maintained at the sterile condition in a CO2 incubator at a temperature of 37 °C and 5% CO2. Once the cells obtain 80% of confluency the cells were subcultured using trypsin solution and used for further experiments.
Cytotoxicity analysis of SG-GNPs
The cytotoxic property of biosynthesized SG-GNPs against the murine melanoma B16 cells were assessed using MTT assay. The cells were cultured on a 24 well plate and the cultured cells were treated with different concentration of SG-GNPs ranging from 0.5–20 µg/ml. SG-GNPs were mixed with serum-free DMEM medium and added on to the cells. The cells were incubated for 24 h in a CO2 incubator at a temperature of 37 °C and 5% CO2. After incubation, the cells were washed with PBS and fresh serum-free DMEM medium was added. To each well 20 µl of MTT solution was added and incubated for 4 h in CO2 incubator at a temperature of of 37 °C and 5% CO2. The intact mitochondrial reductase present in live cells precipitates MTT to form blue formazon crystals. These crystals were dissolved using 100 µl of DMSO solution and the culture plates were subjected to spectroscopic analysis using microplate reader at 545 nm. The experiment was repeated thrice and the data were statistically analyzed.
Rhodamine 123 staining
SG-GNPs induced changes in mitochondrial membrane permeability of murine melanoma B16 cells were assessed using Rhodamine 123 staining technique. The cell was cultured and treated with 5 and 10 µg/ml concentrations of SG-GNPs for 24 h, the treated cell was compared with control cells. After 24 h incubation, the cells were rinsed with PBS and to each well 1mMol Rhodamine 123 stain was added and incubated for 15 min. The cells were then observed under a fluorescent microscope and the images were assessed with Image J software.
Dual AO/EtBr staining
The induction of apoptosis in murine melanoma B16 cells was assessed using dual staining technique with fluorescent dyes acridine orange and ethidium bromide. The control and SG-GNPs treated cells were stained with 1 mM acridine orange and ethidium bromide for 5 min. The stained cells were viewed under a fluorescent microscope and the images were captured, assessed with Image J software.
H2DCFDA staining
The accumulation of ROS in control and SG-GNPs treated cells were assessed using 2',7'-dichlorodihydrofluorescein diacetate staining technique. After 24 incubation of cells with different concentration of SG-GNPs, the treated and control cells were stained with 5 mM 2',7'-dichlorodihydrofluorescein diacetate for 15 min and the stained cells were observed under a fluorescent microscope.
qPCR analysis
The mRNA expression levels of apoptotic genes Caspase 3, Caspase9, Bax, Bcl2, Bid were measured using qPCR analysis. The total RNA from control and SG-GNPs treated murine melanoma B16 cells were isolated using TRI Reagent by the method of Chomczynski and Sacchi (1987) [Citation20]. 2 µg of total RNA isolated from control and treated cells were reverse transcribed to cDNA according to the manufacturer’s protocol using the Superscript III first-strand cDNAsynthesis kit (Invitrogen, Carlsbad, CA, USA). The real-time PCR reaction for the above genes was carried out in MX3000p PCR system (Stratagene, Amsterdam, The Netherlands). Using MESA green PCR master mix, the specific genes were amplified and the specificity was determined by melting curve analysis. The data were analyzed by comparative CT method and the fold change is calculated by 2 − CT method described by Schmittgen and Livak (2008) using CFX Manager Version 2.1 (Bio Rad, Hercules, CA, USA).
Statistical analysis
The data were expressed as mean ± standard deviation (SD) of three independent observations. Data were subjected to statistical analysis by performing one-way ANOVA followed by Tukey’s test using Graph Pad prism 4 statistical software (Hercules, CA,). The p values <.05 were considered significant.
Results
Characterization of SG-GNPs
UV-Vis spectroscopic and SAED analysis of SG-GNPs
The formation and stability of biosynthesized SG-GNPs were assessed using UV-Vis Spectroscopic analysis. The maximum absorption peak was observed at 538 nm which confirms the synthesis of gold nanoparticle and ruby red colour formation. Not much significant difference was observed until 15 days of incubation period whereas it is slightly decreased on 30th day analysis (. depicts the SAED pattern of SG-GNPs with the characteristic ring-shaped pattern indicating the crystalline nature of the SG-GNPs.
TEM - XRD analysis of SG-GNPs
The transmission electron microscopic image of SG-GNPs was depicted in , which resembles the spherical shape and the size of biosynthesized SG-GNPs was measured to be 200 nm. The results of TEM analysis correlates with the SAED pattern of AK-GNPs thus confirming the synthesis of gold nanoparticles. The crystallinity nature of the biosynthesized SG-GNPs was assessed using XRD and the patterns were depicted in . SG-GNPs exhibited three distinct peaks at 111, 200 and 220 which are the characteristic peaks exhibited by the gold nanocrystals.
FTIR spectroscopic analysis of SG-GNPs
signifies the FTIR spectroscopic results of SG-GNPs, the spectra were observed between the ranges of 600 and 3500 cm−1. The significant bands were obtained at 718, 1634, 2139 and 3329 cm−1. The band at 3329 cm−1 confirms the presence of polyphenolic O–H stretch and hydroxyl bonds present on the gold nanoparticles. The band at 718 is due to the presence of alkyl halides and band at 1634 cm−1 represent the presence of amide group.
Anticancer activity of SG-GNPs
Cytotoxic dose analysis of SG-GNPs
The CC50 dose of SG-GNPs against murine melanoma B16 cells was assessed using MTT analysis and the results were depicted in . The viability of melanoma cells in control and SG-GNPs treated cells were compared with the results obtained for MTT assay. There is a significant reduction in a number of viable melanoma cells were observed in SG-GNPs treated cells compared to the control cells. The reduction of viable melanoma cells was in a dose-dependent manner and the 50% reduction in viable melanoma cells were observed at the dose of 10 µg/ml SG-GNPs. Hence, to further assess the anticancer activity of SG-GNPs, the B16 melanoma cells were treated with 10 µg/ml SG-GNPs and also to check the efficacy of lower concentration, 5 µg/ml SG-GNPs was used.
Figure 4. Anticancer activity of biosynthesized Siberian ginseng gold nanoparticle. The CC50 dose of SG-GNPs against murine melanoma B16 cells were assessed by MTT assay. B16 cells were treated with different concentration of SG-GNPs ranging from 0.5 to 20 µg/ml and incubated for 24 h. The control and SG-GNPs treated cells were subjected to MTT assay and the results were statistically analyzed. Each bar represents mean ± SEM of three independent observations. p < .05 is considered as statistically significant.
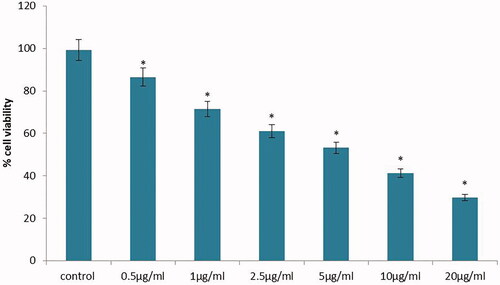
Effect of SG-GNPs on MMP of B16 cells
The initial event of apoptosis is the decrease in mitochondrial membrane potential and the release of cytochrome C, therefore in the present, we assessed the transmembrane mitochondrial membrane permeability using Rhodamine 123 staining (. Compared to control melanoma cells, the SG-GNPs treated cells showed decreased green fluorescence which indicates the reduction in mitochondrial membrane potential.
Figure 5. Anticancer activity of biosynthesized Siberian ginseng gold nanoparticle. Apoptotic effect of SG-GNPs on mitochondrial membrane premeability in murine melanoma cell line B16 was assessed using 1 mM Rhodamine 123 staining technique. B16 murine melanoma cells were treated with 5 and 10 µg/ml SG-GNPs and incubated for 24 h. The control and treated cells were then stained with Rhodamine 123 stain for 15 min. The experiments were performed in triplicates.
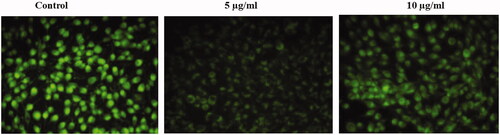
Assessment of ROS generation by SG-GNPs on B16 cells
The generation of reactive oxygen species and induction of apoptosis was assessed by dual AO/EtBr staining () and it is further confirmed with H2DCFDA Staining (. The early apoptotic cells were stained with acridine orange nuclear stain with yellowish-green colour whereas the cells at the late apoptotic stage were stained as orange colour by ethidium bromide stain. Compared to the control melanoma cells the SG-GNPs treated cells showed increased yellowish green and orange stained cells. Increased number of late apoptotic cells stained with orange colour by EtBr were observed in 10 µg/ml SG-GNPs treated cells compared to the control and 5 µg/ml AK-GNPs treated cells (.
Figure 6. Anticancer activity of biosynthesized Siberian ginseng gold nanoparticle. Apoptotic effect of SG-GNPs in murine melanoma cell line B16 was assessed using dual staining technique. B16 murine melanoma cells were treated with 5 and 10 µg/ml SG-GNPs and incubated for 24 h. The control and treated cells were then stained with 1 mM acridine orange and 1 mM Ethidium bromide for 5 min. The experiments were performed in triplicates.
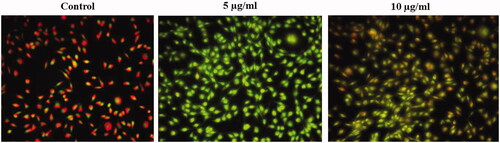
Figure 7. Anticancer activity of biosynthesized Siberian ginseng gold nanoparticle. Apoptotic effect of SG-GNPs via generation of reactive oxygen generation in murine melanoma cell line B16 was assessed using H2DCFDA staining technique. B16 murine melanoma cells were treated with 5 and 10 µg/ml SG-GNPs and incubated for 24 h. The control and treated cells were then stained with 2',7'-dichlorodihydrofluoresceindiacetate stain for 15 min. The experiments were performed in triplicates.
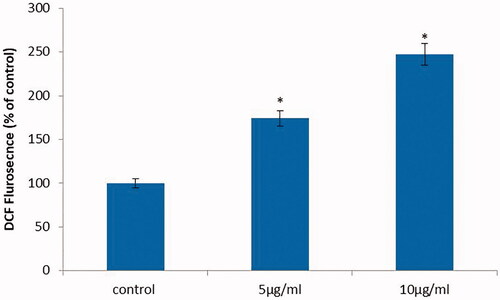
The 2',7'-dichlorodihydrofluorescein diacetate non fluorescent stain is converted to fluorescent 2',7'-dichlorofluorescein (DCF) in the presence of reactive of oxygen species which is an indicator of apoptosis induction. Compared to control melanoma cells the SG-GNPs treated cells showed increased fluorescence which confirms the generation of reactive oxygen species (.
Effect of SG-GNPs on apoptotic gene expression
depicts the results of the qPCR analysis to detect the effect of SG-GNPs on apoptotic gene expression in murine melanoma B16 cells. The apoptotic genes Caspase 3, Caspase 9, Bid and Bax were significantly increased in SG-GNPs treated cells whereas the antiapoptotic genes Bcl2 expression are significantly decreased. The apoptotic gene expression was increased in a dose-dependent manner compared to 5 µg/ml the 10 µg/ml AK-GNPs treated cells showed increased apoptotic gene expression.
Figure 8. Anticancer activity of biosynthesized Siberian ginseng gold nanoparticle. Apoptotic effect of SG-GNPs on apoptotic gene expression in B16 murine melanoma cells was assessed using qPCR analysis. B16 murine melanoma cells were treated with 5 and 10 µg/ml SG-GNPs and incubated for 24 h. After incubation, the control and treated cells were subjected to total RNA isolation. 2 µg of total RNA from control and SG-GNPs treated group were converted to cDNA and subjected to qPCR analysis with specific apoptotic genes Caspase 3, Caspase 9, Bid, Bax and antiapoptotic gene Bcl2. The experiments were performed in triplicates.
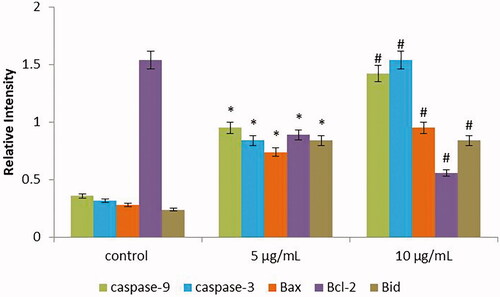
Discussion
In the last 20 years, the usage of nanotechnology in various fields has increased drastically and its application in health care industries are remarkable. Even though nanoparticles possess incredible usage, recently the toxic effects induced by these nanoparticles are taken our consideration by the researchers. The main cause for the nanoparticles induced toxicity is due to the inorganic compounds used during nanoparticle synthesizing process. Hence, current researches were focused on green synthesis of eco-friendly biocompatible nanoparticles using organic compounds.
Synthesizing nano drug using plants possessing medicinal value will be a perfect alternative for the toxic inorganic reducing chemicals. Compared to all other metallic nanoparticles, gold nanoparticles possess unique characteristics features which denote it as a potent drug in the nanomedicine field [Citation21]. Gold nanoparticles specifically target the tumour site and hence, it is used as a carrier in targeted therapies [Citation13]. Food and Drug Agency of United States have accepted gold nanoparticles that are biocompatible, nontoxic, ecofriendly and can be used in the field of pharmaceuticals [Citation22]. Therefore, in the present study, we synthesized a potent biocompatible gold nanoparticle using an aqueous extract of Siberian ginseng. The leaves and roots of Siberian ginseng were used in traditional medicine as an antidiabetic, anti-inflammatory, antihypertensive, antibacterial, anticancer drug, antiobesity [Citation18].
The ruby red colour formation during the synthesis of SG-GNPs confirms the generation of gold nanoparticles and it is further confirmed with UV-Vis spectroscopic analysis where the peak was observed at 538 nm (). The red colour formation during gold nanoparticles is due to the surface plasmon resonance property of gold which correlates with the previous study of Venkatesan et al. (2014) [Citation23]. According to the theory of Mie (1908) [Citation24], spherical-shaped nanoparticles show a strong absorption peak at 520 nm and it starts decreased after 600 nm. In the present study also the SG-GNPs showed intense absorption peak at 520 and it starts decreasing after 600 nm. The TEM image and SAED pattern of SG-GNPs also confirm the spherical shape and crystalline nature of the SG-GNPs which may be the reason for the reduction of absorption peak after 600 nm. Singh et al. (2013) [Citation7], proved the XRD patterns of biosynthesized nanoparticles are crystalline in nature and the Bragg’s reflection pattern of gold metal shows a characterstic peak at 111, 200 and 220 [Citation25], this correlates with our present XRD results of SG-GNPs confirming the synthesis of gold nanoparticles.
FTIR results illustrate intense bands were seen at 718, 1634, 2139 and 3329 cm−1 obtained during the reduction of Au(III) to Au (0). The band at 3329 cm−1 confirms the presence of polyphenolic O–H stretch and hydroxyl bonds present on the gold nanoparticles. The band at 718 is due to the presence of alkyl halides and band at 1634 cm−1 represent the presence of amide group. These characteristic bands are may be due to the carbonyl group from the protein caps on the gold nanoparticles which thereby prevents the nanoparticle agglomeration and the stabilization of gold nanoparticles in the aqueous medium are maintained by the amide linkage protein [Citation26].
The structural and characterization studies using UV-Vis Spec, SAED, HR-TEM, EDX and FTIR confirms that the biosynthesized SG-GNPs is a typical spherical gold nanoparticle, hence we further assessed the SG-GNPs anticancer property against melanoma cells. Melanoma is cancer occurs due to the mutations in the genes of melanocytes producing melanin, the colouring pigment of the skin. It affects most the dark-skinned populations of both genders and the younger adults are more affected than the older population. Even though the survival rate of melanoma patients is 90%, if diagnosed at an earlier stage, it is considered to be one of the deadliest diseases since the symptoms are mostly considered as normal skin diseases and the prognosis is pitiable [Citation27–29]. Based on the stage of cancer condition, melanoma patients were treated with chemotherapy, radiotherapy, surgery but all these therapies have an adverse effect and the efficacy of the drugs are also questionable [Citation30]. Therefore, we assessed the anticancer property of SG-GNPs against B16 murine melanoma cells, a persuasive model of human melanoma condition.
Apoptosis, the programmed cell death is one of the extensively investigated molecular mechanisms by most of the cancer researchers. Since apoptosis is a critical pathway, it controls both physiological and pathological conditions of the cell [Citation31]. The hallmark biochemical changes occurring during the apoptotic process are activation of caspases, reduction in membrane permeability of cells and the breakdown of DNA [Citation32]. Usually, apoptotic signals are triggered to scavenge the damaged DNA which may lead to carcinogenesis, whereas dysregulation in this apoptotic signaling leads to cancer [Citation33]. Hence, we assessed the induction of apoptosis by SG-GNPs against the B16 murine melanoma cells.
The different cytotoxic signaling such as reactive oxygen species, growth deprivation and death receptors induces the apoptotic signaling in cells [Citation34]. Our H2DCFDA staining results of control and SG-GNPs treated cells clearly depicts SG-GNPs increased the generation of reactive oxygen species. Rhodamine 123 staining results signify that the ROS induced by SG-GNPs had lead to the activation of apoptotic proteins thereby it increased the mitochondrial membrane permeability of the B16 cells. Increase in mitochondrial membrane permeability leads the release of proapoptotic proteins such as cytochrome C which in turn activates the apoptotic protein [Citation35]. The results of our dual staining with AO/EtBr clearly depicts that the reactive oxygen species generated by SG-GNPs had increased the mitochondrial membrane permeability, thereby induced apoptosis in B16 murine melanoma cells.
Mitochondrial mediated apoptotic pathway was regulated by the Bcl2 group of proteins, these proteins have common BH domain and they are classified into antiapoptotic proteins (Bcl2, Bclxl, Bclw) and proapoptotic proteins (Bid, Bax, Bad). Most of the anticancer drugs were designed thereby targeting the Bcl2 group of proteins and the drugs which are successfully entered clinical trials [Citation36]. In the present study, we assessed the ability of SG-GNPs to target the BCl2 group of proteins. Our results of q-PCR confirm that the SG-GNPs decreased the antiapoptotic Bcl2 gene expression whereas increased the proapoptotic gene expression, this may be due to the BH3 mimetic property of SG-GNPs which would bind to the hydrophobic groove of Bcl2 thereby induced apoptosis. Same results were observed with BH3 mimetics cancer drug ABT-737 [Citation37].
Caspases, proteases which target the cysteine aspartyl amino acids are the actual masters of the apoptotic process. Caspases cleave the cytoskeletal proteins and nuclear proteins by activating DNA degrading enzymes thereby execute apoptosis. The cytoplasmic release of cytochrome c from the damaged mitochondrial membrane leads to the activation of Caspase 3 which in turn forms an apoptosome complex with Apaf1, Cyt C and Caspase 9 to induce apoptosis [Citation38]. In our present study, SG-GNPs increased the expression of caspase 3 and caspase 9 this may be due to the reactive oxygen species generated by SG-GNPs which would have increased the mitochondrial membrane permeability thereby led to the release of CytC and activation of caspases 3 and 9 in turn escort apoptosis in B16 murine melanoma cells.
Conclusion
Our overall results signify that we synthesized a cost-effective potent biocompatible gold nanoparticle with aqueous extract of Siberian ginseng. The physicochemical of SG-GNPs analysis with UV-Spec, SAED, FTIR, XRD and TEM confirms that the synthesized gold nanoparticle satisfies all the properties of potent gold nanoparticle. The cytotoxicity study reveals that the CC50 dose of SG-GNPs against B16 murine melanoma cells is very minimal. The results of our staining techniques give a clear picture that SG-GNPs generated ROS, increased the mitochondrial membrane permeability thereby induced apoptosis. SG-GNPS acted as BH3 mimic thereby decreased antiapoptotic protein and increased the expression of proapoptotic proteins in melanoma cells. However, the accumulation of nanoparticles present in the human body needs to be studied before to use as an anticancer drug. To conclude that SG-GNPs is proven to be a potent anticancer drug in in vitro condition further in vivo analysis may lead this drug to clinical trials.
Disclosure statement
No potential conflict of interest was reported by the authors.
References
- Armendariz V, Herrera I, peralta-videa JR, et al. Size controlled gold nanoparticle formation by Avena sativa biomass. J. Nanoparticle Res. 2004;6:377–382.
- Castelvetro V, De Vita C. Nanostructured hybrid materials from aqueous polymer dispersions. Adv Colloid Interface Sci. 2004;108–109:167–185.
- Gupta AK, Gupta M. Synthesis and surface engineering of iron oxide nanoparticles for biomedical applications. Biomaterials. 2005;26:3995–4201.
- Raut R,W, Niranjan Kolekar S, Jaya Lakkakula R, Vijay Mendhulkar D, Sahebrao Kashid B. Extracellular synthesis of silver nanoparticles using dried leaves of pongamia pinnata (L) pierre. Nano-Micro Lett. 2010;2:106–113.
- Selvi VK, Sivakumar T. Antihelminthic, anticancer, antioxidant activity of silver nanoparticles isolated from f.oxysporum. Int J Cur Res Chem Pharm Sci. 2014;1:105–111.
- Priyaragini S, Sathishkumar SR, Bhaskararao KV. Biosynthesis of silver nanoparticles using actinobacteria and evaluating its antimicrobial and cytotoxicity activity. Int J Pharm Pharm Sci 2013;2:709–712.
- Singh M, Kalaivani R, Manikandan S, et al. Facile green synthesis of variable metallic gold nanoparticle using Padina gymnospora, a brown marine macroalga. Appl Nanosci. 2013;3:145–151.
- Gao D. Drug designing: open access drug design for cancer: gold nanoparticle-liposome hybrids for drug delivery and monitoring. Drug Des. 2013;2:10–11.
- Engin AB, Nikitovic D, Neagu M, et al. Mechanistic understanding of nanoparticles? Interactions with extracellular matrix: the cell and immune system. Part Fibre Toxicol 2017;14:22.
- Wu CC, Chen DH. Facile green synthesis of gold nanoparticles with gum arabic as a stabilizing agent and reducing agent. Gold Bull. 2010;43:234–239.
- Garrido C, Simpson CA, Dahl NP, et al. Gold nanoparticles to improve HIV drug delivery. Future Med Chem. 2015;7:1097–1107.
- Khan A, Rashid R, Murtaza G, et al. Gold nanoparticles: synthesis and applications in drug delivery. Trop J Pharm Res. 2014;13:1169–1177.
- Tiwari PM, Vig K, Dennis VA, et al. Functionalized gold nanoparticles and their biomedical applications. Nanomaterials 2011;1:31–63.
- Pedrosa P, Vinhas R, Fernandes A, et al. Gold nanotheranostics: proof-of-concept or clinical tool? Nanomaterials (Basel). 2015;5:1853–1879.
- Akihisa T, Tokuda H, Hasegawa D, et al. Enjo, F: Chalcones and other compounds from the exudates of Angelica keiskei and their cancer chemnopreventive effects. J Nat Prod. 2006;69:38–42.
- Enoki T, Ohnogi H, Nagamine K, et al. Antidiabetic activities of chalcones isolated from a Japanese Herb, Angelica keiskei. J Agric Food Chem. 2007;55:6013–6017.
- Kimura Y, Baba K. Antitumor and antimetastatic activities of Angelica keiskei roots, part 1: Isolation of an active substance, xanthoangelol. Int J Cancer. 2003;106:429–437.
- Caesar LK, Cech NB. A Review of the Medicinal Uses and Pharmacology of Ashitaba. Planta Med. 2016;82:1236–1245.
- Okuyama T, Takata M, Takayasu J, et al. Anti-tumor-promotion by principles obtained from Angelica keiskei . Planta Med. 1991;57:242–246.
- Chomczynski P, Sacchi N. Single-step method of RNA isolation by acid guanidinium thiocyanate-phenol-chloroform extraction. Anal Biochem. 1987;162:156–159.
- Chanda N, Kan P, Watkinson LD, et al. Radioactive gold nanoparticles in cancer therapy: therapeutic efficacy studies of GA-198AuNP nanoconstruct in prostate tumor-bearing mice. Nanomedicine. 2010;6:201–209.
- Dhar S, Reddy EM, Shiras A, et al. Natural gum reduced/stabilized gold nanoparticles for drug delivery formulations. Chemistry. 2008;14:10244–10250.
- Venkatesan J, Manivasagan P, Kim SK, et al. Marine algae-mediated synthesis of gold nanoparticles using a novel Ecklonia cava. Bioprocess Biosyst Eng. 2014;37:1591–1597.
- Mie G. Beiträge zur Optik trüber Medien, speziell kolloidaler Metallösungen. Ann Phys. 1908;25:377.
- Saxena A, Tripathi RM, Singh P. Biological Synthesis of silver nanoparticles by using onion (Allium cepa) extract and their antibacterial activity. Digest J Nanomater Biostruct. 2010;5:427–432.
- Ghodake G, Lee DS. Biological synthesis of gold nanoparticles using the aqueous extract of the brown algae Laminaria japonica. J. Nanoelectron Optoe 2011;6:1–4.
- Jiang BP, Zhang L, Guo XL, et al. Poly(N-phenylglycine)-based nanoparticles as highly effective and targeted near-infrared photothermal therapy/photodynamic therapeutic agents for malignant melanoma. Small 2017;13:1602496
- Bombelli FB, Webster CA, Moncrieff M, et al. The scope of nanoparticle therapies for future metastatic melanoma treatment. Lancet Oncol. 2014;15:e22–32.
- Ferlay J, Soerjomataram I, Dikshit R, et al. Cancer incidence and mortality worldwide: sources, methods and major patterns in GLOBOCAN 2012. Int J Cancer. 2015;136:E359–86.
- Domingues B, Lopes JM, Soares P, et al. Melanoma treatment in review. Immunotargets Ther. 2018;7:35–49.
- Wong RS. Apoptosis in cancer: from pathogenesis to treatment. J Exp Clin Cancer Res. 2011;30:87.
- Kumar V, Abbas AK, Fausto N, et al. Robins and Cotran: pathologic basis of disease. Vol. 8. Philadelphia: Saunders Elsevier; 2010. p. 25–32.
- Hassan M, Watari H, AbuAlmaaty A, et al. Apoptosis and molecular targeting therapy in cancer. Biomed Res Int. 2014;2014:1.
- Koff JL, Ramachandiran S, Bernal-Mizrachi L. A time to kill: targeting apoptosis in cancer. Int J Mol Sci. 2015;16:2942–2955.
- Danial NN, Korsmeyer SJ. Cell death: critical control points. Cell. 2004;23:205–219.
- Abou-Nassar K, Brown JR. Novel agents for the treatment of chronic lymphocytic leukaemia. Clin Adv Haematol Oncol 2010;8:886–895.
- Oltersdorf T, Elmore SW, Shoemaker AR, et al. An inhibitor of Bcl-2 family proteins induces regression of solid tumours. Nature. 2005;2:677–681.
- Kroemer G, Galluzzi L, Brenner C. Mitochondrial membrane permeabilization in cell death. Physiol Rev. 2007;87:99–163.