Abstract
Lung cancer is a kind of malignant tumour characterized as uncontrolled cell growth in lung. These malignant cell growth can spread beyond the lung by process of metastasis into other tissues or parts of the body. In this study, we developed dequalinium (DQA) modified paclitaxel plus ligustrazine micelles to destroy vasculogenic mimicry (VM) channels and inhibit tumour metastasis. In vitro assays showed that the targeting micelles with centralized particle size distribution showed not only vigoroso cytotoxicity on A549 cells but also strong inhibition on VM channels and tumour metastasis. Mechanism studies indicated that the DQA modified paclitaxel plus ligustrazine micelles could down-regulate the expressions of VEGF, MMP2, TGF-β1 and E-cadherin in A549 cells. In vivo assays indicated that the targeting drug-loaded micelles could enhance the accumulation of chemotherapeutic drugs at tumour sites and exhibit strong tumour inhibitory activity with negligible toxicity. Hence, the DQA modified paclitaxel plus ligustrazine micelles developed in this study may provide a potential strategy for treatment of NSCLC.
Introduction
Lung cancer is one type of malignant tumour with the highest morbidity and mortality. It has become the most prevalent cause of cancer-related deaths in men and the second most common in women. Lots of data show that the inheritance of cancer genes, long-term heavy smoking and serious air pollution are considered to be related to the occurrence of lung cancer [Citation1,Citation2]. Non-small-cell lung cancer (NSCLC), a kind of frequently occurring lung cancer, is characterized by short proliferative cycle, strong metastatic activity and high fatality rate. It accounts for over 80% of all lung cancers, and 90% of NSCLC patients have metastasis before definite diagnosis [Citation3]. It has established the irreplaceable position of surgical resection, radiotherapy and chemotherapy in treatment of lung cancer [Citation4]. However, surgical resection is only appropriate for the patients who are diagnosed with early stage of NSCLC and the cancer cells have not yet metastasized beyond nearby lymph nodes [Citation5]. The treatment effect of conventional chemotherapy is also not satisfactory for the poor targeting of chemotherapeutic drugs and the high metastasis of cancer cells.
Paclitaxel is a tetracyclic diterpene compound and is often used as a first-line anticancer drug in the clinical chemotherapy of NSCLC. The obvious tumour cytotoxicity of paclitaxel is caused by destroying the dynamic balance of microtubule proteins in cancer cells, inducing the aggregation of microtubule proteins and stabilizing microtubules. And the stable microtubules can suppress cancer cells at the stage of mitosis G2/M, so as to effectively inhibit the proliferation and eliminate cancer cells in the body [Citation6,Citation7]. However, the solubility of paclitaxel in water is extremely low (about 5 μg/mL), and this has become the primary difficulty for paclitaxel in clinical chemotherapy. The commonly used paclitaxel injection has serious biotoxicity and neurotoxicity for the adding of polyoxyethylene castor oil to improve the solubility of paclitaxel. Uncontrollable allergic reactions will be induced after long-term use [Citation8,Citation9]. From this perspective, it is necessary to exploit a suitable drug delivery system to avoid drawbacks of conventional paclitaxel injection. Fortunately, this problem could be solved by polymeric micelles prepared in our previous study [Citation10].
Polymeric micelles, an easy formulation, is one of the new-style targeting drug-delivery systems. When the amount of surfactants increases to the critical micelle concentration (CMC), the hydrophobic segments attract each other and self-assemble to form the polymeric micelle [Citation11]. The solubility of hydrophobic chemotherapeutic drugs can be greatly increased by means of this kind of polymeric micelle with core-shell structure. Soluplus, an emerging synthetic material for polymeric micelles, is polyvinyl caprolactam-polyvinyl acetate-polyethylene glycol graft copolymer [Citation12]. It can easily form micelle at a concentration of 0.76 × 1 0−3% (w/v). In recent years, some articles have reported that soluplus has successfully solved the problem of low solubility of hydrophobic chemotherapy drugs, such as cyclosporine A, doxorubicin and paclitaxel [Citation13,Citation14].
In fact, 90% of NSCLC patients died from tumour metastasis. Tumour metastasis is that the tumour tissues with a certain volume gain ability of invasion and the adhesion to extracellular matrix is increased, so as to penetrate the basement membrane into the blood vessels or lymph nodes and form new tumour foci on the distal tissue. Ligustrazine is a well-known bioactive component isolated from the rhizoma of ligusticum. As a common alkaloid regulator for chemotherapy, ligustrazine exhibits multiple biological activities, including anti-platelet, anti-inflammatory and anticancer effect [Citation15,Citation16]. The powerful anticancer mechanism of ligustrazine is due to inhibit invasion and metastasis of cancer cells, thereby restraining the self-healing, angiogenesis and metastasis of malignant tumours [Citation17,Citation18].
In this study, the easy formulation encapsulated paclitaxel and ligustrazine was produced by membrane hydration to solve the problem of low solubility of paclitaxel, increase the active targeting of paclitaxel and inhibit invasion and metastasis of NSCLC. In the polymeric micelles, dequalinium lipid derivative (DSPE-PEG2000-DQA) was synthesized as targeting carries according to our reports. Furthermore, the physical characterization, the effects on treatment of NSCLC both in vitro and in vivo, and mechanism of anticancer of the polymeric micelles were evaluated in detail.
Material and methods
Reagents and cells
Soluplus was obtained by Feng li Jing qiu Pharmaceutical Co., Ltd. (Beijing, China). DQA was obtained from Sigma-Aldrich (Saint Louis, MO, USA). Paclitaxel and ligustrazine were obtained from Meilun Biotechnology Co., Ltd. (Dalian, China). RPMI-1640 medium was obtained from GIBCO (Billings, MT, USA). A549 cells were obtained from the Institute of Basic Medical Science, Chinese Academy of Medical Science (Beijing, China), and incubated in RPMI-1640 medium with 10% fetal bovine serum (FBS) (Zhejiang Tianhang Biological Technology Co., Ltd. Hangzhou, China) under 5% CO2 in a humidified incubator at 37 °C. Chemicals were analytical or high performance liquid chromatography (HPLC) grade. BALB/c nude mice (18–20 g) were obtained from Peking University Experimental Animal Center (Beijing, China). All procedures were carried out according to guidelines of the Institutional Authority for Laboratory Animal Care of Peking University.
Preparation of polymeric micelles
DSPE-PEG2000-DQA was synthesized according to our previous study and polymeric micelles were prepared by membrane hydration method [Citation10,Citation19]. Briefly, the methanol solution of soluplus, paclitaxel, ligustrazine and DSPE-PEG2000-DQA were blended and stirred in a round-bottom flask with a molar ratio of 100:1:5:6. The rotary evaporator was used to remove the methanol under vacuum at 40 °C and a film was formed on the inner wall of the flask. Then the film was dissolved with PBS buffer (pH 7.4) and the flask was shaken in the 25 °C water bath for 30 min. Finally, the micelles were passed through microporous membranes with pore size of 100 nm for three times. Then, DQA modified paclitaxel plus ligustrazine polymeric micelles were developed.
In addition, blank micelles, paclitaxel micelles, DQA modified paclitaxel micelles and paclitaxel plus ligustrazine micelles were prepared according to the same methods. Specifically, the respective compositions were soluplus/DSPE-PEG2000-DQA (50:3, molar ratio), soluplus/paclitaxel (100:1, molar ratio), soluplus/DSPE-PEG2000-DQA/paclitaxel (100:6:1, molar ratio) and soluplus/paclitaxel/ligustrazine (100:1:5, molar ratio), respectively. As fluorescence probes, coumarin micelles, coumarin plus ligustrazine micelles and DQA modified coumarin plus ligustrazine micelles (lipids/coumarin = 100:1, w/w), DiR micelles and DQA modified DiR plus ligustrazine micelles (lipids/DiR = 200:1, w/w) were accordingly prepared to measure the distribution of polymeric micelles.
Characterization of the polymeric micelles
Transmission electron microscope (TEM; Tecnai G2 20ST; FEI Co., Tokyo, Japan) was used to measure the morphology of DQA modified paclitaxel plus ligustrazine polymeric micelles at 120 kV. To further characterize the micelles, the newly prepared micelles were added to PBS and diluted to 1 ml. After the mixture was evenly mixed, the particle size, polydispersity index (PDI) and zeta potential were determined using a Nano Series Zen 4003 Zetasizer (Malvern Instruments Ltd., Malvern, UK). Instrument laser beam was set at a wavelength of 633 nm and the angle of incident light and scattering light was 90°. Encapsulation efficiencies (EE) of polymeric micelles were analyzed using HPLC equipped with a UV detector (Shimadzu, LC-2010 AHT, Japan). In short, micelles were centrifuged at 10,000 rpm for 10 min and demulsified by methanol at 1:9 volume. 20 μL of supernatant was absorbed into the HPLC. The EE% was calculated by the formula: (Q1/Q0) × 100%, where Q1 and Q0 referred to the quality of drug successfully loaded in micelles and added in the micellar suspensions, respectively.
Cytotoxicity on A549 cells
To evaluate the cytotoxicity of polymeric micelles on A549 cells, sulforhodamine B (SRB) proliferation assay was employed in this study [Citation20]. Briefly, A549 cells were seeded in 96 well culture plates (8 × 103 cells/well) and placed in cell incubator for 24 h. Then, medium was removed and culture plates were gently washed for three times with PBS buffer. In the group of free drugs, paclitaxel and ligustrazine were dissolved in DMSO solutions. DMSO was diluted less than 10% by serum-free culture medium to prepare free paclitaxel, free ligustrazine, free paclitaxel and ligustrazine mixture solution (1:1, 1:3 and 1:5, mole ratio). 20 μL of free drugs were added per well and the final concentrations of paclitaxel were in the range of 0.01–5 μM and DMSO is less than 1%. The same amount of culture medium was added as control. Then, 96 well culture plates were placed in cell incubator for 48 h. In the micellar groups, A549 cells were added with blank polymeric micelles, paclitaxel polymeric micelles, DQA modified paclitaxel polymeric micelles, paclitaxel plus ligustrazine polymeric micelles and DQA modified paclitaxel plus ligustrazine polymeric micelles, respectively. The final concentrations of paclitaxel were in the range of 0.01–10 μM and mole ratios of paclitaxel and ligustrazine were 1:5. After the incubation, samples were fixed with 10% trichloroacetic acid solution (TCA, 10%) and stained with 0.4% SRB. Tris base solution (pH = 10.5, 10 mM) was used to dissolve the combined dye after full dyeing. Finally, the optical density was measured at 540 nm with microplate reader (HBS-1096A, DeTie, Nanjing, China). The survival rates of A549 cells were calculated by the formula: survival % = (AD/AB) ×100%, where AD and AB were the absorbance value of drug groups and blank control. Survival curves were drawn and IC50 values were calculated using GraphPad Prism.
Cellular uptake in A549 cells
As fluorescent probe, coumarin was loaded in varying polymeric micelles to evaluate the active targeting in A549 cells. Briefly, A549 cells were seeded in six-well culture plates at a density of 3 × 105 cells per well and placed in cell incubator for 24 h. Then the varying micellar formulations were added in six well culture plates. The final concentration of coumarin was 2 μM. Blank micelles were used as blank control. After incubation for 3 h, A549 cells were trypsinized and harvested in PBS buffer. A FACScan flow cytometer (BD Biosciences, NJ, USA) was used to analyze the mean fluorescence intensity of coumarin. Each assay was done in triplicate.
Destructive effects on VM channels
The three-dimensional culture of Matrigel was used to simulate the formation of VM channels of lung cancer in vitro. Briefly, the Matrigel low growth factor matrix in 4 °C was melted into liquid. 50 μL Matrigel was added per well in the pre-cooled 96 well cell culture plate. This part of operation was carried out on the ice box placed in advance on the super clean platform. Then, the culture plate was placed at 37 °C for 30 min to curdle the Matrigel. After solidification, 3 × 104 cells/well were inoculated into the 96 well culture plate and the varying polymeric micelles were added. Final concentration of paclitaxel was 10 μM. Blank micelles were used as the control group. Then the cell culture plate was placed in the incubator and incubated for 4 h. The damage effects of micelles on the VM channels of lung cancer were observed by an inverted microscope (Chongqing Photoelectric Co., Ltd., Chongqing, China).
Inhibitory effects on A549 cells invasion
A 12-well Transwell chamber was used to carry out the invasion assay. Briefly, A549 cells were added into upper chamber in a density of 3 × 104 cells/well. The cells were then treated with the varying polymeric micelles at a concentration of 10 μM paclitaxel. Culture medium was added into each lower chamber as the chemoattractant. Incubation for 8 h, the cells which did not go through the membrane were washed by a cotton swab and the invasive cells were fixed with cold 4% formaldehyde for 20 min. Subsequently, cells were stained with 0.1% crystal violet for 20 min. The amount of invasive A549 cells was observed using the inverted microscopy.
Wound healing assay on A549 cells
Wound healings were measured as the distance migrated by the leading edge of the wound at each time point. A549 cells were digested and added into the six-well culture plate in a density of 1 × 106 cells per well. After the cells spread to about 80% confluence, small scratches were made at center of the culture plate by a pipette tip. The culture plate was washed by cold PBS buffer to remove the debris. The varying polymeric micelles with a concentration of 10 μM paclitaxel were added into culture plate. Blank micelles were used as blank control. The inverted microscopy was used to photograph the scratch area at 0, 12 and 24 h.
Adhesion rate of A549 cells to extracellular matrix
In order to avoid the bias induced by cytotoxicity of paclitaxel, an SRB assay was used to evaluate the cytotoxicity on A549 cells after treatment with the varying micelles for only 12 h with the final concentration of 10 μM paclitaxel, and all the experimental procedures were same as the part of “Cytotoxicity on A549 cells”. Subsequently, 30 μL Matrigel was added into the 96 well culture plate at room temperature. A549 cells were seeded in six-well culture plate and cultured for 24 h. Then, the cells were treated with the varying polymeric micelles with the final concentration of 10 μM paclitaxel for another 12 h. Group treated with blank micelles was regarded as the control. After incubation for 12 h, the living cells were trypsinized, resuspended in culture medium and then seeded on the top of the Matrigel in a final volume of 100 μL. Cells were incubated at 37 °C under 5% CO2 for 2 h. The SRB staining method was used to evaluate the inhibition on adhesion according to our previous report. The adhesion rate was calculated by the formula: rate % = (At for treated groups/Ac for control group) ×100%, where At and Ac represented the absorbance value measured at 540 nm for treated group and control group, respectively. Each assay was carried out in triplicate.
Regulating metastasis-related proteins and apoptotic enzymes
Enzyme-linked immunosorbent assay kits (Cusabio Biotech Co. Ltd., Beijing, China) were used to evaluate the activities of vascular endothelial growth factor (VEGF), matrix metalloproteinase 2 (MMP 2), transforming growth factor-β1 (TGF-β1) and E-cadherin in A549 cells. Briefly, A549 cells about 80% confluence were treated with the varying micellar formulation at a concentration of 10 μM paclitaxel. The group treated with blank micelles was the control. After incubation for 12 h, A549 cells were trypsinized, harvested and lysed. The acquisition was centrifuged (10,000 rpm) at 4 °C for 10 min. The total protein was analyzed by a bicinchoninic acid kit at 540 nm. The Cell lysates were operated according to the manufacturer’s instructions of the kits and analyzed by using a microplate reader at 450 nm. The protein activity ratio was calculated by the following formula: activity ratio % = (At 450 nm/At 540 nm)/(Ac 450 nm/Ac 540 nm), where At and Ac were the absorbance values for treated group and control group, respectively. Each assay was done in triplicate.
To investigate the apoptotic mechanism, apoptotic enzymes were measured after A549 cells were treated with varying formulations. Briefly, the A549 cells were grown to 50% confluence in 96-well plates before treatment with the varying micellar formulation at a concentration of 10 μM paclitaxel. Blank micelles were used as a blank control. After incubation for 6 h, the cells were fixed with 4% formaldehyde for 10 min. Then, the cells were permeabilized with PBS containing 0.5% Triton X-100 and 0.3 M glycine for 15 min and blocked with PBS containing 5% goat serum for 2 h. The cells were then incubated with primary antibodies (anti-Caspase-3, anti-Caspase-8 and anti-Caspase-9). All the primary antibodies were diluted to 2 μg/ml and incubated at 4 °C overnight. The culture wells were added with secondary antibody conjugated with Alexafluor-488 (1:500), and further incubated for 2 h at room temperature. Cell nuclei in above culture wells were then counterstained with Hoechst 33258 (1 μg/ml). The fluorescence intensity of each well was subsequently measured by a fluorescence microscope.
In vivo imaging and pharmacodynamics study in tumour-bearing mice
The tumour-bearing mice model was established to reveal the in vivo targeting property of the polymeric micelles and fluorescent DiR dye was encapsulated into the polymeric micelles as a fluorescence probe. Briefly, 6 × 106 A549 cells were suspended in 200 μL RPMI-1640 medium and implanted into BALB/c nude mice by right antebrachial oxter injection. The mice were fed under standard condition for 2 weeks and used for subsequent experiments. The tumour-bearing mice were randomly divided into four groups and treated with blank micelles, free DiR, DiR micelles and DQA modified DiR plus ligustrazine micelles (200 μg/kg) via the tail vein (3 per group). The fluorescent images and X-ray images of the tumour-bearing mice were scan by an in vivo image system (Carestream FX PRO; Carestream Health Inc., Rochester, NY, USA) at 1, 3, 6, 12, 24 and 48 h.
Tumour-bearing mice model was established as mentioned above. After two weeks feeding, mice were randomly distributed into five groups and treated with physiological saline (blank control), free paclitaxel, paclitaxel micelles, DQA modified paclitaxel micelles, paclitaxel plus ligustrazine micelles and DQA modified paclitaxel plus ligustrazine micelles via tail intravenous injection, respectively. The dosage was 3 mg/kg paclitaxel, and the operation was conducted every other day for four times in succession. To evaluate the pharmacodynamics of the varying polymeric micelles, a vernier caliper was used to measure the tumour size and tumour volume ratio was calculated by the following formula: tumour volume ratio = Vn/V0, where Vn and V0 refer to tumour volume measured at different time point and tumour volume measured at day 0. After treatment evaluation, the mice were sacrificed by dislocation. Tumours were collected were fixed in paraformaldehyde for subsequent evaluation. TUNEL assay was then applied according to the kit instructions to detect apoptosis and the nuclei were stained with DAPI. The fluorescence was observed with a fluorescence microscope.
Statistical analysis
Data were presented as the mean ± standard deviation (SD). Analyses were carried out using the SPSS 17.0 software. Analysis of variance was used to determine the significance among groups, and p < .05 was considered to be significant.
Results
Characterization of the polymeric micelles
Particle size, EE%, PDI and zeta potential of the varying polymeric micelles are shown in . For all the micellar formulations, EE% of paclitaxel and ligustrazine was higher than 89% and 88%, respectively. Particle sizes of the varying polymeric micelles were approximately in range of 50–60 nm with a centralized polydispersity indexes (PDI ≤ 0.20). Zeta potentials were 0.32 ± 0.01 mV for paclitaxel micelles, 7.73 ± 0.21 mV for DQA modified paclitaxel micelles, 0.18 ± 0.02 mV for paclitaxel plus ligustrazine micelles and 6.78 ± 1.92 mV for DQA modified paclitaxel plus ligustrazine micelles, respectively. As shown in , the polymeric micelles were mainly composed of soluplus. Both paclitaxel and ligustrazine were entrapped simultaneously into the hydrophobic core of the micelles, and DSPE-PEG2000-DQA was inserted on the surface of polymeric micelles to increase the active targeting. The TEM image in showed that the morphology of DQA modified paclitaxel plus ligustrazine micelles was basically spherical. attested the positive zeta potential and centralized size distribution of DQA modified paclitaxel plus ligustrazine micelles.
Figure 1. Characterization of DQA modified paclitaxel plus ligustrazine micelles. Notes: (A) A schematic representative of DQA modified paclitaxel plus ligustrazine micelles. (B) TEM image of DQA modified paclitaxel plus ligustrazine micelles. (C) Zeta potential of DQA modified paclitaxel plus ligustrazine micelles. (D) Partical size of DQA modified paclitaxel plus ligustrazine micelles.
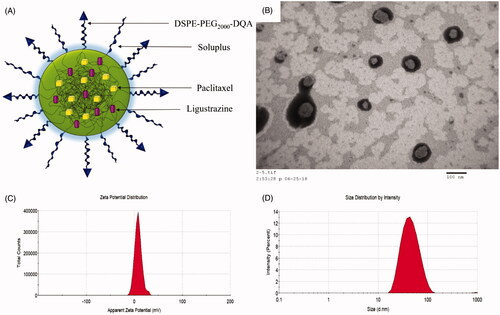
Table 1. Characterization of the varying micelles.
Cytotoxicity on A549 cells
shows the cytotoxicity on A549 cells after treatments with the varying formulations. According to , ligustrazine alone had negligible cytotoxicity on A549 cells, and the cytotoxicity was strongest under the combine of paclitaxel and ligustrazine in a molar ratio of 1:5. showed that the rank of cytotoxicity on A549 cells was DQA modified paclitaxel plus ligustrazine micelles > paclitaxel plus ligustrazine micelles > DQA modified paclitaxel micelles > paclitaxel micelles > blank micelles. The IC50 values were 1.83 ± 0.21 μM for paclitaxel micelles, 1.49 ± 0.12 μM for DQA modified paclitaxel micelles, 0.60 ± 0.08 μM for paclitaxel plus ligustrazine micelles and 0.42 ± 0.08 μM for DQA modified paclitaxel plus ligustrazine micelles, respectively.
Figure 2. Cytotoxicity on A549 cells after treatments with the varying formulations. Notes: (A) Cytotoxicity of free drugs, I, vs. free ligustrazine; II, vs. free paclitaxel; III, vs. free paclitaxel: free ligustrazine =1:1; IV, vs. free paclitaxel: free ligustrazine = 1:3. (B) Cytotoxicity of the varying micellar formulations. 1, vs. Blank micelles; 2, vs. Paclitaxel micelles; 3, vs. DQA modified paclitaxel micelles; 4, vs. Paclitaxel plus ligustrazine micelles. Data are presented as mean ± SD (n = 3). p < .05.
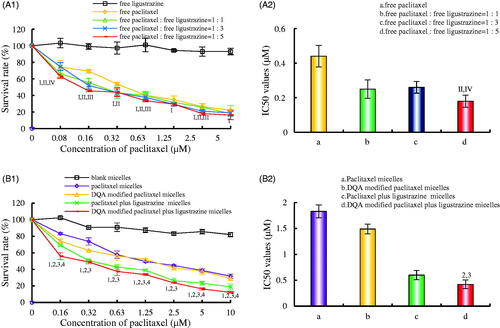
Cellular uptake in A549 cells
Cellular uptake of the polymeric micelles was measured by flow cytometry (). The rank of mean fluorescent intensity values of A549 cells were 507.65 ± 41.43 for DQA modified paclitaxel plus ligustrazine micelles, 412.76 ± 26.76 for paclitaxel plus ligustrazine micelles, 367.98 ± 21.33 for DQA modified paclitaxel micelles, 249.54 ± 16.64 for paclitaxel micelles and 3.56 ± 0.85 for blank micelles, respectively.
Figure 3. Cellular uptake and targeting effects after incubation with the varying formulations. Notes: (A) Cellular uptake of A549 cells. (B) Fluorescence intensity of coumarin 6 in A549 cells. a. Blank micelles; b. Coumarin micelles; c. DQA modified coumarin micelles; d. Coumarin plus ligustrazine micelles; e. DQA modified coumarin plus ligustrazine micelles. p < .05, 1, vs. a; 2, vs. b; 3, vs. c; 4, vs. d.
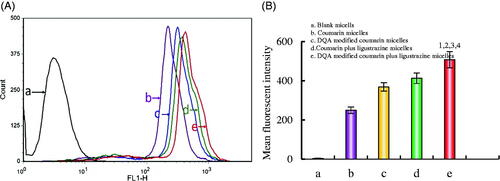
Destructive effects on VM channels
shows the destructive effects on VM channels in vitro after incubation with the varying formulations. There were apparent vessel-like loops, channels, and networks after treatment with blank micelles. Both DQA modified paclitaxel plus ligustrazine micelles and paclitaxel plus ligustrazine micelles exhibited destructive effects on VM channels, and negligible destructions were observed after treatment with paclitaxel micelles or DQA modified paclitaxel micelles.
Figure 4. Destructive effects on VM channels in vitro after incubation with the varying formulations. Notes: a. Blank micelles; b. Paclitaxel micelles; c. DQA modified paclitaxel micelles; d. Paclitaxel plus ligustrazine micelles; e. DQA modified paclitaxel plus ligustrazine micelles; f. VM channel correlation index ratio. p < .05, 1 vs a, 2 vs b, 3 vs c, 4 vs d.
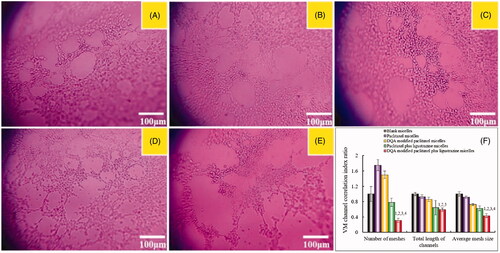
Inhibitory effects on A549 cells invasion
exhibits the inhibitory effects on A549 cells invasion after treatment with the varying formulations. The invasive cells to the other side of the insert were dyed to rich purple by crystal violet, and the inhibitory effects on A549 cells invasion were evaluated by the intensity of purple signal. Results demonstrated that the rank of inhibitory effects on A549 cells invasion was DQA modified paclitaxel plus ligustrazine micelles > paclitaxel plus ligustrazine micelles > DQA modified paclitaxel micelles > paclitaxel micelles > blank micelles.
Figure 5. Inhibiting invasion on A549 cells in vitro after incubation with the varying formulations. Notes: a. Blank micelles; b. Paclitaxel micelles; c. DQA modified paclitaxel micelles; d. Paclitaxel plus ligustrazine micelles; e. DQA modified paclitaxel plus ligustrazine micelles; f. Cell invasion rate. p < .05, 1 vs a, 2 vs b, 3 vs c, 4 vs d.

Wound healing assay on A549 cells
The inhibitory effects on wound healing after treatment with the varying polymeric micelles are shown in . Results attested that the extent of recovery of damaged areas was high after treatment with blank micelles, paclitaxel micelles and DQA modified paclitaxel micelles compared with the initial wound. However, DQA modified paclitaxel plus ligustrazine micelles and paclitaxel plus ligustrazine micelles showed the strongest inhibitory effects on wound healing.
Figure 6. Blocking wound healing effects on A549 cells in vitro after incubation with the varying formulations. Notes: (A) Blocking wound healing effects; (B) Wound healing rate (%). a. Blank micelles; b. Paclitaxel micelles; c. DQA modified paclitaxel micelles; d. Paclitaxel plus ligustrazine micelles; e. DQA modified paclitaxel plus ligustrazine micelles; f. Cell invasion rate. p < .05, 1 vs Blank micelles, 2 vs Paclitaxel micelles, 3 vs DQA modified paclitaxel micelles, 4 vs Paclitaxel plus ligustrazine micelles.
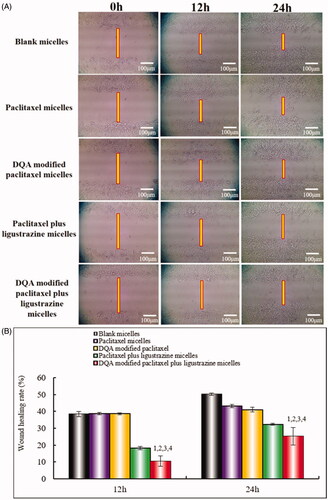
Adhesion rate of A549 cells to extracellular matrix
shows the adhesion rates of A549 cells on Matrigel. The survival rates of A549 cells were evaluated to avoid the cytotoxic influence (). Results illustrated that all polymeric micelles had negligible cytotoxic effects on A549 cells at a concentration of 10 μM paclitaxel at 12 h. In , The adhesion rates of A549 cells after treatment with paclitaxel micelles, DQA modified paclitaxel micelles, paclitaxel plus ligustrazine micelles and DQA modified paclitaxel plus ligustrazine micelles were 95.77 ± 2.12%, 95.91 ± 2.98%, 72.53 ± 2.60% and 73.63 ± 6.11%, respectively. The adhesion rates of A549 cells treated with paclitaxel plus ligustrazine micelles and DQA modified paclitaxel plus ligustrazine micelles were significantly reduced compared with other groups.
Regulating effects on metastasis-related proteins and apoptotic enzymes
illustrates the relative expressions of VEGF, MMP2, TGF-β1 and E-cadherin after treatment with the varying polymeric micelles. Results showed that all the micellar formulations could evidently down-regulated the expression of VEGF, MMP2, TGF-β1 and E-cadherin in A549 cells. The activity ratio of VEGF, MMP2, TGF-β1 and E-cadherin after treatment with DQA modified paclitaxel plus ligustrazine micelles were reduced to 0.62 ± 0.06, 0.49 ± 0.05, 0.36 ± 0.02 and 0.72 ± 0.07, respectively.
Figure 8. Regulating effects on metastasis-related proteins in A549 cells after treatments with the varying formulations. Notes: a. Blank micelles; b. Paclitaxel micelles; c. DQA modified paclitaxel micelles; d. Paclitaxel plus ligustrazine micelles; e. DQA modified paclitaxel plus ligustrazine micelles. p < .05, 1, vs. a; 2, vs. b; 3, vs. c. Data are presented as mean ± SD (n = 4).
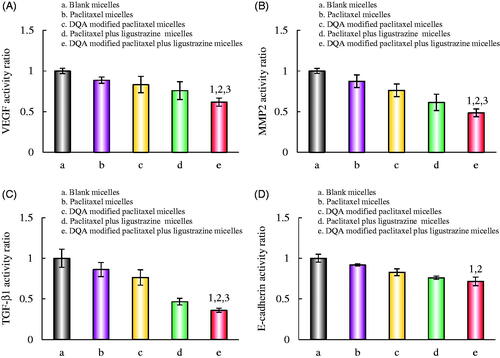
shows that the nuclei of A549 cells were stained as the blue colour by Hoechst 33258 while activated apoptotic enzymes or proteins were dyed as the green colour by specific monoclonal antibodies, respectively. Expressions of Caspase-3, Caspase-8 and Caspase-9 were significantly increased after treatment with varying formulations, in which, DQA modified paclitaxel plus ligustrazine micelles demonstrated the strongest activating effect on the apoptotic enzymes.
In vivo imaging and pharmacodynamics study in tumour-bearing mice
shows the in vivo real-time imaging in tumour-bearing mice after treatment with the varying formulations. Results depicted the strong fluorescent signal in tumour location after the administration of DQA modified DiR plus ligustrazine micelles and the signal was maintained up to 24 h. The rank of DiR fluorescent signal in tumour site at varying time-point was DQA modified DiR plus ligustrazine micelles > DiR micelles > free DiR > blank micelles. In the assay of weight change (), results showed that weight of tumour-bearing mice in treatment groups exhibited no obvious change compared with the group of normal saline, indicating that the varying drug-loaded formulation had negligible toxicity on the mice's own health. shows the tumour volume changes. Results showed that tumour volume ratios were 26.47% ± 8.23 for blank control, 21.43% ± 9.45 for free paclitaxel, 19.54% ± 7.84 for paclitaxel micelles, 18.54% ± 9.24 for DQA modified paclitaxel micelles, 17.65% ± 7.56 for paclitaxel plus ligustrazine micelles and 14.65% ± 8.13 for DQA modified paclitaxel plus ligustrazine micelles, respectively.
Figure 10. In vivo real-time imaging observation after intravenous administration of the varying formulations.
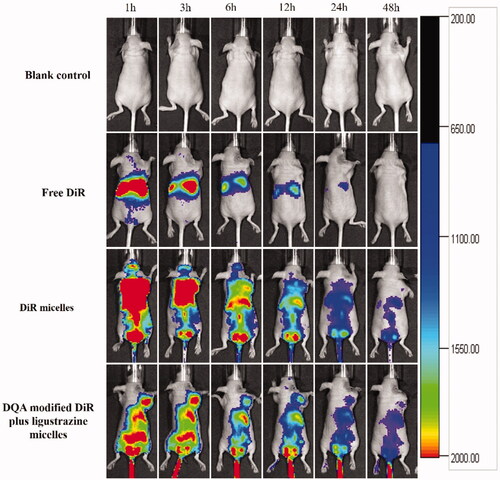
Figure 11. Anticancer effects in A549 cells xenografts mice after treatment with the varying formulations. Notes: (A) Body weight changes. (B) Tumour volume changes. (C) Representative images of tumour cells apoptosis were detected by TUNEL, Scale bar = 100 μm. Where a. blank control, b. free paclitaxel, c. paclitaxel micelles, d. DQA modified paclitaxel micelles, e. paclitaxel plus ligustrazine micelles and f. DQA modified paclitaxel plus ligustrazine micelles.
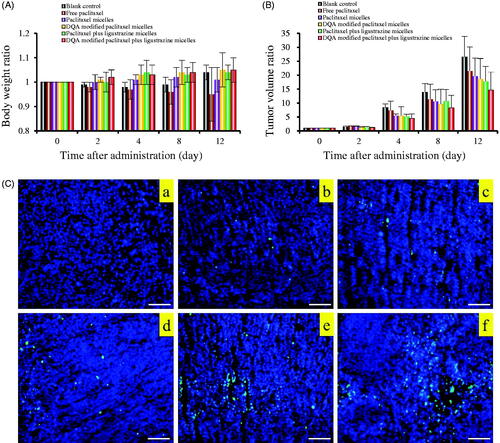
Results of TUNEL assay for detection of apoptosis in tumour tissues are shown in . Blue fluorescence represented the nuclei and green fluorescence represented apoptotic cells. The number of cells expressing green fluorescence in DQA modified paclitaxel plus ligustrazine micelles was the highest among all the experimental groups which indicated the most apoptotic cells in treatment group of DQA modified paclitaxel plus ligustrazine micelles.
Discussion
Lung cancer, a considerable threat to human health, is a type of malignant tumour with the highest morbidity and mortality. NSCLC is characterized by short proliferative cycle, strong metastatic activity and high fatality rate. Traditional anticancer measures are often restricted by poor targeting and poor solubility of chemotherapeutic drugs, tumour metastasis, tumour recurrence and bad prognosis [Citation21–23]. Polymeric micelles, a novel targeting drug delivery system, can faultlessly overcome these limitations by improving the solubility of hydrophobic drugs and enhancing the drug accumulation on focus [Citation24]. In this study, a kind of DQA modified paclitaxel plus ligustrazine polymeric micelles were prepared to increase solubility of paclitaxel, improve drug active targeting, induce apoptosis of cancer cells and inhibit tumour metastasis.
DQA modified paclitaxel plus ligustrazine polymeric micelles had the following outstanding physicochemical properties, smooth sphere in morphology (), positive zeta potential (), centralized particle size distribution () and high encapsulation efficiencies (). In addition, the particle size of polymeric micelles was approximately 60 nm, which was conducive to the passive transport of paclitaxel to tumour tissue through the leaky vasculature.
In vitro cytotoxicity on A549 cells after treatment with the varying formulations was evaluated by determining survival rate using SRB method. In , results showed that the combined application of paclitaxel and ligustrazine exhibited stronger inhibitory effect on A549 cells compared with paclitaxel alone, and ligustrazine alone had negligible cytotoxicity on A549 cells, which demonstrated that ligustrazine was helpful for improving therapeutic effect of paclitaxel. According to , DQA modified paclitaxel plus ligustrazine polymeric micelles had a vigoroso inhibitory effect on A549 cells. Compared with other formulations, the IC50 value of DQA modified paclitaxel plus ligustrazine polymeric micelles was significantly decreased. In addition, blank micelles showed the negligible inhibitory effect, which eliminated the deviation of micellar material. The drug-loaded micelles exhibited the cytotoxic effect in inducing apoptosis by a cascade of reactions via activating initiator Caspase-8 and Caspase-9 to effector Caspase-3 signalling pathway ().
In the cellular uptake, coumarin was encapsulated in polymeric micelles instead of paclitaxel and used as a fluorescent probe due to non-fluorescence of paclitaxel. According to the results measured by FACScan flow cytometer, the fluorescent intensity of coumarin was the highest after treatment with DQA modified coumarin plus ligustrazine polymeric micelles, which showed that the targeting micelles could improve cellular uptake of chemotherapeutic drugs in A549 cells ().
Metastasis is the leading cause of death in cancer patients, and approximately 90% of cancer deaths arise from the metastatic spread [Citation25]. In fact, tumour metastasis refers to not only the translocation of tumour, but the tumour migration and the establishment of a new tumour environment after arriving at the distal tissue. During the metastatic cascade, tumour cells which grow to a certain extent are separated from the original tissue and are combined with extracellular matrix by morphological and structural changes. Then, tumour cells acquire the ability of invasion and enter into blood or lymph by degrading the extracellular matrix. When the cells invade into the other normal organs, they attach to the extracellular matrix again by adhesion. Subsequently, VM channels are formed by tumour cells to provide nutrition for the rapid growth of tumour cells before the formation of new blood vessels [Citation26–29]. Hence, the adhesiveness of tumour cells to extracellular matrix, the migration ability of tumour cells and the VM channels are the main factors that affect the tumour metastasis.
Under the condition of hypoxia, VM channels could be formed by tumour cells, thus facilitating tumour metastasis without the involvement of endothelial cells [Citation30]. Hence, it is necessary to evaluate the destroying effect on VM channels after treatment with the varying polymeric micelles. In this study, the VM channel model was established by A549 cells in three-dimensional Matrigel (). Results indicated that both paclitaxel plus ligustrazine micelles and DQA modified paclitaxel plus ligustrazine micelles showed a strong damage on the VM channels, which illustrated that the addition of ligustrazine could effectively destroy the formation of VM channels.
Invasion is the pivotal factor for tumour metastasis. In the process of tumour metastasis, tumour cells invasion to basement membranes which is essential to enter into blood or lymph system for tumour cells. In this study, the transwell invasion model was established to evaluate the inhibitory effect on A549 cells after treatment with the varying formulations. As shown in , compared with blank control, paclitaxel plus ligustrazine micelles and DQA modified paclitaxel plus ligustrazine micelles exhibited the formidable ability of inhibiting tumour invasion. To further evaluate inhibitory effects of the varying micellar formulations on A549 cells invasion, the wound healing assay was also carried out in vitro. As showed in , the wound healing ability of A549 cells without drugs was obvious. In contrast, the strong inhibitory effects were observed after treatment with ligustrazine loaded micelles, which illustrated that ligustrazine played a significant role in the process of inhibiting invasion of A549 cells.
Inhibiting tumour cells adhesion to basement membrane is an important strategy to inhibit tumour metastasis. In this study, the inhibitory effects on the adhesion of A549 cells were evaluated using Matrigel to imitate extracellular matrix. Results showed that ligustrazine loaded micelles could inhibit the adhesion of A549 cells to matrix with a negligible cytotoxicity to tumour cells. Among all the micellar formulations, DQA modified paclitaxel plus ligustrazine micelles exhibited the strongest inhibitory effects on adhesion, which attested that the targeting micelles could suppress effectively the process of tumour metastasis.
VEGF is a kind of multi-functional cell regulatory factor, and VEGF could affect the formation and growth of VM channels which is closely related to the tumour invasion and metastasis [Citation31,Citation32]. MMP2 is a protein connected with the ability of tumour metastasis, it can accelerate tumour invasion by degrading the extracellular matrix and the basement membrane [Citation33]. TGF-β1 is a well-known chemokine that plays an important role in cancer progression and contributes to disease deterioration, and it is also involved in regulation of cell proliferation, differentiation and invasion [Citation34,Citation35]. E-cadherin is epithelial markers of epithelial mesenchymal transition (EMT) which is involved in embryonic development, wound healing, tissue remodelling, and malignant tumour metastasis. The down-regulated expression of E-cadherin can markedly suppress tumour metastasis [Citation36]. As shown in , the activity ratios of VEGF, MMP2, TGF-β1 and E-cadherin after treatment with DQA modified paclitaxel plus ligustrazine micelles were down-regulated obviously, which indicated that the targeting micelles could distinctly inhibit tumour metastasis via down-regulating related protein.
Tumour-bearing mice were established to evaluate targeting ability and therapeutic effects of the varying polymeric micelles. The body real-time imaging observation was measured by an in vivo image system. Results showed that DQA modified DiR and ligustrazine micelles had a long circulating time in the body and had obvious concentration in tumour compared with free DiR (). The high tumour-targeting ability of DQA modified DiR and ligustrazine micelles may be connected with the combination of the EPR effect and DQA-mediated cellular uptake. The pharmacodynamic assay was carried out on tumour-bearing mice to observe the anticancer effect of the varying polymeric micelles. Bodyweight ratios showed that there was no obvious indication of acute toxicity after treatment with DQA modified paclitaxel plus ligustrazine micelles (). Results from tumour volume changes showed that the tumour-bearing mice in group of DQA modified paclitaxel plus ligustrazine micelles exhibited the stronger tumour inhibitory activity compared with other control groups (). TUNEL assay suggested the anti-cancer mechanism of DQA modified paclitaxel plus ligustrazine micelles may be related to the inhibition of tumour cell proliferation and the promotion of tumour cell apoptosis, which was consistent with those results of in vitro evalutions.
Conclusion
In this study, the DQA modified paclitaxel plus ligustrazine polymeric micelles were prepared and evaluated from physicochemical characteristics, targeting effect and ability of inhibit tumour metastasis. The experimental results illustrated that the polymeric micelles prepared in this study were associated with the following ideal characteristics: (i) the centralized particle size (50–60 nm) and positive zeta potential were more convenient for accumulation in tumour tissue; (ii) the addition of paclitaxel is highly lethal to A549 cells and the modification of DQA on the surface improved the cellular uptake of polymeric micelles; (iii) the addition of ligustrazine inhibited the metastasis and VM channels of A549 cells by down-regulating the expressions of a variety of metastasis-related proteins. DQA modified paclitaxel plus ligustrazine polymeric micelles prepared in this study provided a potential treatment strategy for NSCLC.
Disclosure statement
No potential conflict of interest was reported by the authors.
Additional information
Funding
References
- Torre LA, Siegel RL, Jemal A. Lung cancer statistics. Adv Exp Med Biol. 2016;893:1–19.
- Dubey P, Gidwani B, Pandey R. In vitro and in vivo evaluation of PEGylated nanoparticles of bendamustine for treatment of lung cancer. Artif Cells Nanomed Biotechnol. 2016;44:1491–1497.
- Dearing KR, Sangal A, Weiss GJ. Maintaining clarity: review of maintenance therapy in non-small cell lung cancer. WJCO. 2014;5:103–113.
- Graham EA, Singer JJ. Successful removal of an entire lung for carcinoma of the bronchus. CA Cancer J Clin. 1974;24:238–242.
- Zhu Y, Zhu R, Wang M, et al. Anti-metastatic and anti-angiogenic activities of core-shell SiO2@LDH loaded with etoposide in non-Small cell lung cancer. Adv Sci (Weinh). 2016;3:1600229.
- Zhang L, Liu Z, Yang K, et al. Tumor progression of non-small cell lung cancer controlled by albumin and micellar nanoparticles of itraconazole, a multitarget angiogenesis inhibitor. Mol Pharmaceutics.. 2017;14:4705–4713.
- Chen X, Sun X, Guan J, et al. Rsf-1 influences the sensitivity of non-small cell lung cancer to paclitaxel by regulating NF-κB pathway and its downstream proteins. Cell Physiol Biochem. 2017;44:2322–2336.
- Jain MM, Gupte SU, Patil SG, et al. Paclitaxel injection concentrate for nanodispersion versus nab-paclitaxel in women with metastatic breast cancer: a multicenter, randomized, comparative phase II/III study. Breast Cancer Res Treat. 2016;156:125–134.
- Wang HY, Zhang XR. Comparison of efficacy and safety between liposome-paclitaxel injection plus carboplatin and paclitaxel plus carboplatin as first line treatment in advanced non-small cell lung cancer. Zhongguo Yi Xue Ke Xue Yuan Xue Bao. 2014;36:305–308.
- Wang X, Cheng L, Xie HJ, et al. Functional paclitaxel plus honokiol micelles destroying tumour metastasis in treatment of non-small-cell lung cancer. Artif Cells Nanomed Biotechnol. 2018;46:1154–1169.
- Parent LR, Bakalis E, Ramírez-Hernández A, et al. Directly observing micelle fusion and growth in solution by liquid-cell transmission electron microscopy. J Am Chem Soc. 2017;139:17140–17151.
- Zhao J, Xu Y, Wang C, et al. Soluplus/TPGS mixed micelles for dioscin delivery in cancer therapy. Drug Dev Ind Pharm. 2017;43:1197–1204.
- Yu H, Xia D, Zhu Q, et al. Supersaturated polymeric micelles for oral cyclosporine A delivery. Eur J Pharm Biopharm. 2013;85:1325–1336.
- Jin X, Zhou B, Xue L, et al. Soluplus(®) micelles as a potential drug delivery system for reversal of resistant tumor. Biomed Pharmacother. 2015;69:388–395.
- Zou Y, Zhao D, Yan C, et al. Novel ligustrazine-based analogs of piperlongumine potently suppress proliferation and metastasis of colorectal cancer cells in vitro and in vivo. J Med Chem. 2018;61:1821–1832.
- Shen J, Zeng L, Pan L, et al. Tetramethylpyrazine regulates breast cancer cell viability, migration, invasion and apoptosis by affecting the activity of Akt and caspase-3. Oncol Lett. 2018;15:4557–4563.
- Zheng CY, Xiao W, Zhu MX, et al. Inhibition of cyclooxygenase-2 by tetramethylpyrazine and its effects on A549 cell invasion and invasion and metastasis. Int J Oncol. 2012;40:2029–2037.
- Yu K, Chen Z, Pan X, et al. Tetramethylpyrazine-mediated suppression of C6 gliomas involves inhibition of chemokine receptor CXCR4 expression. Oncol Rep. 2012;28:955–960.
- Szymusiak M, Kalkowski J, Luo H, et al. Core-shell structure and aggregation number of micelles composed of amphiphilic block copolymers and amphiphilic heterografted polymer brushes determined by small-angle x-ray scattering. ACS Macro Lett. 2017;6:1005–1012.
- Li XT, Zhou ZY, Jiang Y, et al. PEGylated VRB plus quinacrine cationic liposomes for treating non-small cell lung cancer. J Drug Target. 2015;23:232–243.
- Zhang C, Yu G, Shen Y. The naturally occurring xanthone α-mangostin induces ROS-mediated cytotoxicity in non-small scale lung cancer cells. Saudi J Biol Sci. 2018;25:1090–1095.
- Yang D, Dai R, Zhang Q, et al. Apatinib for heavily treated patients with non-small cell lung cancer: report of a case series and literature review. Saudi J Biol Sci. 2018;25:888–894.
- Abraham C, Garsa A, Badiyan SN, et al. Internal dose escalation is associated with increased local control for non-small cell lung cancer (NSCLC) brain metastases treated with stereotactic radiosurgery (SRS). Adv Radiat Oncol. 2018;3:146–153.
- Liu G, Li K, Wang H. Polymeric micelles based on PEGylated chitosan-g-lipoic acid as carrier for efficient intracellular drug delivery. J Biomater Appl. 2017;31:1039–1048.
- Liu Z-L, Wang C, Chen H-J, et al. Bone metastasis from lung cancer identified by genetic profiling. Oncol Lett. 2017;13:847–850.
- Sun YW, Xu J, Zhou J, et al. Targeted drugs for systemic therapy of lung cancer with brain metastases. Oncotarget. 2018;9:5459–5472.
- Thakur C, Rapp UR, Rudel T. Cysts mark the early stage of metastatic tumor development in non-small cell lung cancer. Oncotarget. 2017;9:6518–6535.
- Glinsky GV. Activation of endogenous human stem cell-associated retroviruses (SCARs) and therapy-resistant phenotypes of malignant tumors. Cancer Lett. 2016;376:347–359.
- Schnegg CI, Yang MH, Ghosh SK, et al. Induction of vasculogenic mimicry overrides VEGF-A silencing and enriches stem-like cancer cells in melanoma. Cancer Res. 2015;75:1682–1690.
- Ju RJ, Cheng L, Qiu X, et al. Hyaluronic acid modified daunorubicin plus honokiol cationic liposomes for the treatment of breast cancer along with the elimination vasculogenic mimicry channels. J Drug Target. 2018;26:793–805.
- Fu N, Li H, Sun J, et al. Aqueous extract enhances the mobilization of endothelial progenitor cells and up-regulates the expression of VEGF, eNOS, NO, and MMP-9 in acute myocardial ischemic rats. Front Physiol. 2018;17:1132.
- Rivera-Pérez J, Monter-Vera MDR, Barrientos-Alvarado C, et al. Evaluation of VEGF and PEDF in prostate cancer: a preliminary study in serum and biopsies. Oncol Lett. 2018;15:1072–1078.
- Lu S, Zhang Z, Chen M, et al. Silibinin inhibits the migration and invasion of human gastric cancer SGC7901 cells by downregulating MMP-2 and MMP-9 expression via the p38MAPK signaling pathway. Oncol Lett. 2017;14:7577–7582.
- Tao MZ, Gao X, Zhou TJ, et al. Effects of TGF-β1 on the proliferation and apoptosis of human cervical cancer hela cells in vitro. Cell Biochem Biophys. 2015;73:737–741.
- Gao J, Zhu Y, Nilsson M, et al. TGF-β isoforms induce EMT independent migration of ovarian cancer cells. Cancer Cell Int. 2014;14:72.
- Rötzer V, Hartlieb E, Vielmuth F, et al. E-cadherin and Src associate with extradesmosomal Dsg3 and modulate desmosome assembly and adhesion. Cell Mol Life Sci. 2015;72:4885–4897.