Abstract
AMP-activated alpha 1 catalytic subunit (PRKAA1) is one of the subunits of the mammalian 5′-AMP-activated protein kinase (AMPK) playing an important role in maintaining intracellular energy metabolism and associating with the risk of gastric cancer (GC). This paper aims to uncover the influences of PRKAA1 on the tumorigenesis of GC, as well as the underlying mechanisms. We found that Helicobacter pylori (H. pylori) infection markedly increased p-NF-κBp50 and NF-κBp50 expression, along with the PRKAA1 expression, which was inhibited by NF-κBp50 knockdown. NF-κBp50 and PRKAA1 expression were lower in non-tumor gastric tissues compared with that in GC tumor tissues. Up-regulation of PRKAA1 expression was correlated with poor survival in GC patients. MKN-45 and BGC-823 cells stably knockdown of PRKAA1 were transplanted into nude mice and observed the decreased cell metastasis in the lungs. PRKAA1 knockdown in GC cells showed significant decreases in the cell invasion and migration and inhibited MMP-2 expression and NF-κB activation, whereas PRKAA1 involved in NF-κBp50 mediated GC cell invasion and migration. In conclusion, our findings suggest the involvement of NF-κBp50 in the regulation of PRKAA1 in GC tumorigenesis.
Introduction
Gastric cancer (GC) is the fourth most common tumor in the world and the second cause of cancer-related death [Citation1]. Approximately 42% of the new cases of GC worldwide are occurring in East Asia, especially in China [Citation2]. The incidence and mortality rate of GC in China rank first in all malignant tumors, with the mortality rate 0.04% in male and 0.02% in female, which is 4 and 5 times as high as that of developed countries in Europe and America, respectively [Citation3]. In recent years, although the incidence of GC is decreasing and surgery, intervention, chemoradiotherapy and targeted therapy have made great progress in the comprehensive treatment of GC, the prognosis of GC is poor [Citation4]. Studies have shown that heterogeneity is present in the process of metastasis and progression of GC, finally resulting in the difference in invasion, metastasis and drug resistance between metastatic tumors and primary tumors [Citation5,Citation6]. Although the etiology and pathogenesis of GC are very complex and have not yet been fully elucidated, genetic background [Citation7], behavioral factors (drinking, smoking, diet) [Citation8], and infectious factors (Helicobacter pylori) [Citation9] are associated with the risk of GC. Several studies have shown that H. pylori seropositivity is associated with a significantly increased risk of gastric cancer, and the longer the time interval between H. pylori detection and gastric cancer diagnosis, the higher the risk of developing cancer [Citation10]. Therefore, exploring the interaction between environmental factors and genetic factors in the occurrence and development of GC is of great importance for the early prevention, treatment and pathogenesis of GC.
AMP-activated alpha 1 catalytic subunit (PRKAA1) is the encoding gene of AMPK α subunit. AMPK is an energy receptor that maintains intracellular energy metabolism and is activated when the ratio of AMP/ATP increases. Activated AMPK is closely related to apoptosis, cell cycle, neovascularization, tumor growth and proliferation, invasion and metastasis [Citation11–13]. Epidemiological investigation showed that metformin, an AMPK activator, can reduce the incidence and mortality of digestive cancer [Citation14]. 5-Aminoimidazole-4-carboxamide ribonucleoside (AICAR), to activate AMPK, suppressed migration and invasion in human prostate cancer cell lines PC3 and PC3M [Citation15]. AMPK inactivation has been reported to mediate resistin-induced increase in the MMP-2 expression and chondrosarcoma migration [Citation16]. Risk of GC is associated with PRKAA1 SNP rs13361707 [Citation17]. There are two possible biological mechanisms of PRKAA1 in the development of GC. First, activated AMPK can phosphorylate p53 to induce cell cycle arrest at G1/S phase [Citation18]. Second, activated AMPK can reverse the inflammatory effect of macrophages [Citation19]. However, the specific mechanisms of PRKAA1 and the functions downstream PRKAA1 in GC migration and invasion still leave an open question.
As a transcription factor, nuclear factor-κB (NF-κB) can control cancer progression and cancer treatment resistance by regulating a series of genes related to cell proliferation and cell death [Citation20]. Transcription factor NF-κB family is composed of NF-κB1 (p105/p50), NF-κB2 (p100/p52), REL, REL-A (NF-κBp65) and REL-B. NF-κBp50 and NF-κBp65 are the two most important factors in NF-κB family in human and the two mostly in the form of dimers are present in the activated cytoplasm or nucleus and associate with cell invasion and migration. NF-κBp50 is overexpressed in gastric, thyroid, colorectal and hepatocellular carcinoma, suggesting an important role of NF-κBp50 in tumorigenesis [Citation21–23]. H. pylori infection promotes GC cell invasion through enhancing nuclear NF-κB activity [Citation24]. NF-κB is activated by various signals through degrading IκBs and then enters into the nucleus to bind to DNA. Another level of regulation of NF-κB activation is via AMPK [Citation25]. Combined with the latest ENCODE database (www.genome.ucsc.edu/ENCODE) and bioinformatics analysis, PRKAA1 SNP rs461404 and rs10035235 are found to locate in the binding domain of NF-κBp50. Besides, knockdown of PRKAA1 results in the marked suppression of the expression of NF-κB-dependent genes and associates with an expression of anti-apoptotic genes regulated by NF-κB activity [Citation26]. These findings suggest the interaction between PRKAA1 and NF-κB signaling.
The aims of our study were to examine the effect of PRKAA1 on GC cell migration and invasion in vitro and in vivo. Furthermore, the NF-κB signaling pathway involved in the pro-migratory and pro-invasive effect of PRKAA1 on GC cells was also examined.
Materials and methods
Tissue samples
Thirty three cases of non-tumor gastric tissues and 249 cases of GC tissues were collected from TCGA database. 60 cases of GC tissues and their paired non-tumor gastric tissues were collected from GC patients in Xuzhou Central Hospital recruited from May 2004 to Feb 2009. The study was approved by the Medical Ethical Committee of Xuzhou Central Hospital. All patients gave their informed consent for this study.
Immunohistochemistry (IHC)
The non-tumor gastric and GC tissues samples (1.5 cm × 1.5 cm × 0.3 cm) were fixed in 10% formalin for 10 min, dehydrated in a gradient of ethanol for 2 h, and then transparent, paraffin and embedded. GC and non-tumor gastric tissue slides (4–7 μm) were deparaffinized, rehydration and antigen-retrieved, after which slides were blocked by 3% H2O2 for 10 min and incubated with anti-PRKAA1 antibody (Abcam) at 25 °C for 1 h and then stained with horseradish peroxidase (HRP)-labeled IgG (Jackson Laboratories, Suffolk, UK) for 20–30 min at 25 °C. The section was subsequently with diaminobenzidine (DAB) staining, hematoxylin staining, dehydration and sealing. Cells with positive stain more than 25% were defined as PRKAA1 higher expression groups and that less than 25% were defined as PRKAA1 lower expression groups.
Cell culture and infection
Human GC cell lines BGC-823, MKN-45 and MGC-803 were obtained from the American Type Culture Collection (ATCC) in May 2016. All cell lines were authenticated by short tandem repeat PCR profiling. No cross-contamination of other human cells was observed. The last time that the cell lines were tested was June 2018. MGC-803 and MKN-45 cells were cultured in RPMI-1640 medium containing 10% fetal bovine serum (FBS; JRH Bioscience, USA) and 1% streptomycin/penicillin (Solarbio, USA). BGC-823 cells were grown in DMEM medium plus 10 mM glucose containing 10% FBS (JRH Bioscience) and 1% streptomycin/penicillin. All the cells were maintained in 95% air and 5% carbon dioxide at 37 °C. BGC-823 and MKN-45 cells were infected with the H. pylori (strain 43504, ATCC) at a multiplicity of infection (MOI) of 25, 50 and 100.
Transfection
Three shRNAs targeting human PRKAA1 (point 619–637 shRNA-1, 5′-GGTAGATATATGGAGCAGT-3′; point 1320–1338 shRNA-2, 5′-ACCCATATTATTTGCGTGT-3′; point 1376–1394 shRNA-3, 5′-ATGAGTCTACAGTTATACC-3′) were inserted into the pLKO.1 lentivirus and PRKAA1 or NF-κBp50 coding sequences were inserted into pLVX-Puro lentivirus. 293 T cells were seeded in 6-well plate and transfected with recombinant lentiviral vectors using Lipofectamine 2000 (Invitrogen, Carlsbad, CA, USA) in accordance with the instruction of the manufacturer. 48 h after transfection recombined pLKO.1-PRAKK1-shRNA, pLVX-Puro-NF-κBp50 or pLVX-Puro-PRKAA1 lentivirus was collected and transduced BGC-823, MKN-45 or MGC-803 cells. Additionally, three siRNAs targeting NF-κBp50 (point 471–491 siRNA-1, 5′-GCAGAAGATGATCCATATTTT-3′; point 2126–2146 siRNA-2, 5′-CCACCTTCATTCTCAACTTTT-3′; point 2852–2872 siRNA-3, 5′-GCCAGAGTTTACATCTGATTT-3′) were also produced and transfected into the BGC-823 and MKN-45 cells using Lipofectamine 2000. Cells transduced with blank pLVX-Puro or pLKO.1-scramble shRNA and that transfected with scramble siRNA were used as a negative control.
Animal experiments
A total of 5 × 106 BGC-823 or MKN-45 cells transduced with lentivirus silencing PRKAA1 were trypsinized, resuspended in PBS, and then injected into the tail vein of 4–5 week-old BALB/c male nude mice (6 per group). Mice were sacrificed at day 21 after the injection, and the lung tissues were collected and monitored by H&E staining as previously described [Citation27]. Animal experiments were approved by the Xuzhou Central Hospital institutional ethical committee and performed according to the legal requirements.
Cell migration and invasion
After pLVX-Puro-PRKAA1, pLVX-Puro-NF-κBp50, pLKO.1-PRKAA1-shRNA transduction or NF-κBp50-siRNA transfection, cells were serum-starved in the free-serum medium for one day and plated in the upper Transwell inserts (Millipore) containing polycarbonate filters coated with (invasion assay) or without Matrigel (Becton Dickinson, Bedford, MA, USA) (migration assay). The medium containing 10% FBS was added into the lower chamber. After 24 h incubation, cells were fixed with 4% formaldehyde, stained with 0.5% crystal violet, photographed and counted under a microscope (Olympus Corporation, Japan).
Quantitative real-time PCR
Whole RNA was extracted from GC tissues and cell lines by using RNeasy Plus Mini Kit (Qiagen, Germany) and stored at −80 °C in RNA secure RNase Inactivation Reagent (Thermo Fisher Scientific). cDNA was synthesized using a PrimeScript reagent kit (TaKaRa Biotechnology Co, Ltd, Dalian, China) in accordance with protocols of the manufacturer. Quantitative Real-time PCR was performed using SYBR Green chemistry (Thermo Fisher Scientific, Rockford, IL) and the ABI 7,300 instrument (Applied Biosystem). The primers used to amplify the coding region were as follows: PRAKK1-F, 5′-TATGGCAGAAGTATGTAGAG-3′ and PRAKK1-R, 5′-ACGGAAATCCAGTAGATAAG-3′; NF-κBp50-F, 5′-GCACAAGGAGACATGAAACAG-3′ and NF-κBp50-R, 5′-CAGCCGGAAGGCATTATTAAG-3′; GAPDH-F, 5′-AATCCCATCACCATCTTC-3′ and GAPDH-R, 5′-AGGCTGTTGTCATACTTC-3′. Data were analyzed by 2−ΔΔCt method to indicate the relative quantity of mRNA and the internal control were β-actin.
Western blotting
Total protein collected from GC tissues and cell lines were lysed using RIPA lysis buffer and then centrifuged at 12,000 × g for 20 min at 4 °C. 15 μl of proteins were separated by 10% SDS-PAGE, followed by being transferred to nitrocellulose membrane (Shanghai Haoran Biological Technology Co., China). The blots were then incubated with anti-PRAKK1 (Abcam), anti-MMP-2 (Abcam), anti-p-NF-κBp65 (CST), anti-NF-κBp65 (CST), anti-p-NF-κBp50 (Abcam), anti-NF-κBp50 (CST), anti-GAPDH (CST) and HRP-conjugated secondary antibodies (Beyotime Biotechnology, Shanghai, China). An enhanced chemiluminescence substrate kit (Amersham Biosciences; Piscataway, NJ, USA) was utilized to quantify the content of protein expression.
Plasmid construction and dual luciferase assay
BGC-823 and MKN-45 cells were transfected with pGL3-basic plasmid containing the 5′-promoter region of PRKAA1 in the absence or presence of NF-κBp50 siRNA or pLVX-Puro-NF-κBp50 along with pRL-TK plasmid using Lipofectamine 2000 (Invitrogen) following the manufacturer's protocol at 37 °C for 6 h. Then the Luciferase assay system (Promega) was carried out to measure the luciferase activity according to the manufacturer’s protocol.
Chromatin immunoprecipitation (ChIP)
ChIP analysis was performed as previously described [Citation28]. The antibody used for the ChIP against NF-κBp50 (Abcam) was used. Purified ChIP DNA was confirmed by PCR with primers for PRKAA1 (PRAKK1-F, 5′-AGCGGGAAACTCCATCACAG-3′ and PRAKK1-R, 5′-CGCAGAAGACCATCCAGAGG-3′).
Statistical analysis
All experiments were performed in triplicate. All data were expressed as mean ± SD and carried out by GraphPad prism 5.0 (GraphPad Software, USA). The differences between groups were analyzed using one-way analysis of variance (ANOVA) when more than two groups or a Student t-test when only two groups were compared. p Values of less than .05 were considered to show a significant difference between the two groups.
Results
H. pylori promotes p-NF-κBp50, NF-κBp50 and PRKAA1 expression in GC cells
Helicobacter pylori (H. pylori) infection promotes GC cell invasion through enhancing nuclear NF-κB activity [Citation24], indicating an important role of H. pylori in GC tumorigenesis. Our results found that H. pylori (25, 50 and 100 MOI) infection significantly increased the p-NF-κBp50 and NF-κBp50 protein expression in both BGC-823 and MKN-45 cells (). Moreover, H. pylori (25, 50 and 100 MOI) infection also significantly increased the PRKAA1 mRNA expression in both BGC-823 and MKN-45 cells (), along with the increased PRKAA1 protein expression (. However, the increased expression of NF-κBp50 and PRKAA1 were not observed in normal gastric cell line GES-1 following H. pylori infection (data not shown). These results suggest that NF-κBp50 and PRKAA1 may associate with the tumorigenesis of GC.
Figure 1. Helicobacter pylori infection in GC cells promotes PRKAA1 expression through targeting NF-κBp50. BGC-823 and MKN-45 cells were infected with H. pylori (25, 50, and 100 MOI), and the protein expression of p-NF-κBp50 and NF-κBp50 (A, B) as well as the PRKAA1 expression (C, D) was measured by Quantitative Real-time PCR or Western blot assay, respectively. **p < .01 compared with BGC-823 or MKN-45 cells without H. pylori infection. (E, F) BGC-823 and MKN-45 cells were transfected with NF-κBp50 siRNA in the absence or presence of H. pylori infection and the PRKAA1 expression was measured by Quantitative Real-time PCR and Western blotting assay, respectively. **p < .01 compared with siNC. ##p < .01 compared with H. pylori + siNC. (G) BGC-823 and MKN-45 cells were transfected with siRNA-NF-κBp50 or transduced with pLVX-Puro-NF-κBp50 and the transcription activity of PRKAA1 was measured by dual-luciferase analysis. **p < .01 compared with control. (H) The ChIP assay showed that NF-κBp50 bound to PRKAA1 promoter. Top: Schematic diagram of primers for ChIP analysis. 3′UTR served as a negative control (NC). Bottom: ChIP assays were carried out in BGC-823 and MKN-45 cells. BS: binding site. **p < .01 compared with control or IgG.
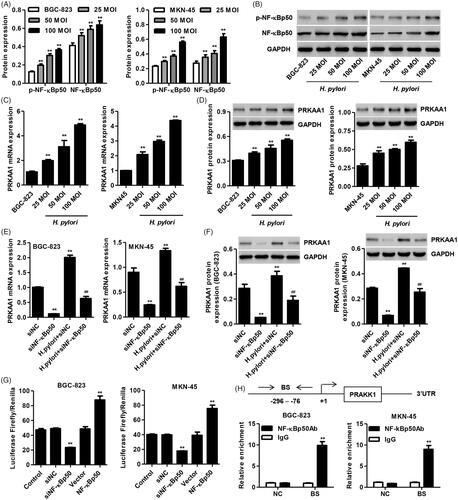
NF-κBp50 regulates PRKAA1 expression through transactivation of PRKAA1 promoter
To confirm our hypothesis that NF-κBp50 is associated with the regulation of PRKAA1 expression, three siRNAs targeting NF-κBp50 was transfected into both MKN-45 and BGC-823 cells, respectively. siRNA-1, -2 and -3 markedly inhibited the mRNA expression of NF-κBp50 by 75.5, 46.7 and 30.9% in BGC-823 cells and by 82.9, 49.3 and 43.5% in MKN-45 cells compared with siNC group (Figure S1A and B). Western blotting also showed similar results that highest knockdown efficiency detected in MKN-45 and BGC-823 cells with siRNA-1 transfection (Figure S1C and D). siRNA-1 was therefore used in our following experiments. Furthermore, NF-κBp50 knockdown significantly suppressed the PRKAA1 expression in both MKN-45 and BGC-823 cells in the absence or presence of H. pylori infection (). Our findings suggest that H. pylori infection promote PRAKK1 expression through increasing NF-κBp50 expression.
Based on this general concept, dual-luciferase and ChIP analysis were further performed in both BGC-823 and MKN-45 cells to verify our hypothesis that the PRAKK1 transcription is mediated by NF-κBp50. NF-κBp50 overexpression significantly increased the NF-κBp50 mRNA expression by 1.65-fold in BGC-823 cells and by 1.77-fold in MKN-45 cells compared with vector group (Figure S1E and F). Western blotting also showed similar results (Figure S1G and H). Importantly, we also found that NF-κBp50 knockdown markedly suppressed while NF-κBp50 overexpression markedly promoted the PRKAA1 transcription in BGC-823 and MKN-45 cells compared with the siNC or vector groups (. Using PROMO analysis, a NF-κBp50 binding site was predicted in the PRAKK1 promoter (-147 to -136) and we confirmed that NF-κBp50 directly bound to the PRKAA1 promoter (-296 to -76), but not to the 3′UTR (.
Expression pattern and clinical value of PRKAA1 in GC
To examine the role of PRKAA1 as well as NF-κBp50 in the tumorigenesis of GC, the expression of PRKAA1 as well as NF-κBp50 was measured firstly. PRKAA1 and NF-κBp50 mRNA expression in GC tissues from Hospital was higher than that in their paired non-tumor gastric tissues (). NF-κBp50 mRNA level was positively correlated with that of PRKAA1 in GC tissues (. PRKAA1 expression between corresponding non-tumor gastric tissues and GC tissues collected from two independent data, including TCGA database and Hospital, was significantly different. PRKAA1 mRNA levels in TCGA database were significant increase in 249 cases of GC tissues compared with that in 33 cases of non-tumor gastric tissues (. PRKAA1 protein expression measured by western blotting was significantly up-regulated in randomize 12 cases of GC tissues from Hospital compared with their paired non-tumor gastric tissues (). The PRKAA1 protein expression in GC tissues and corresponding non-tumor gastric tissues measured by IHC analysis was consistent with western blot assay that PRKAA1 was significant increase in the GC samples compared with the non-tumor gastric samples, which >25% positively stained PRKAA1 was observed respectively in 60.0% (36/60) of the GC tissues (. Furthermore, Kaplan-Meier analysis demonstrated that the patients with PRKAA1 higher expression possessed a shorter overall survival time compared with PRKAA1 lower expression (. PRKAA1 expression was significantly correlated with TNM stage, pathologic grade, distant metastasis, and H. pylori infection (). In addition, a Cox regression model was established to analyze the effect of each variable on overall survival. In terms of overall survival, univariate analysis revealed that PRKAA1 expression, distant metastasis, pathologic grade and TNM stage were prognostic factors. In multivariate Cox regression analysis, PRKAA1 expression, distant metastasis and TNM stage were independent predictors of poor prognosis ().
Figure 2. PRKAA1 is up-regulated in GC tissues. (A, B) NF-κBp50 and PRKAA1 mRNA expression in non-tumor gastric (n = 60) and GC tissues (n = 60) from Hospital data was measured. (C) Linear regression showed that the mRNA expression of NF-κBp50 was positively correlated with PRKAA1 mRNA expression in GC tissues (n = 60) from Hospital. (D) PRKAA1 mRNA expression in non-tumor gastric and GC tissues from TCGA database was measured. PRKAA1 protein expression in non-tumor gastric and GC tissues from Hospital data was measured by Western blot (E, F) and IHC (G) analysis, respectively. Scale bars: 100 μm. (H) Survival analysis of patients with GC. **p < .01 compared with non-tumor gastric tissues.
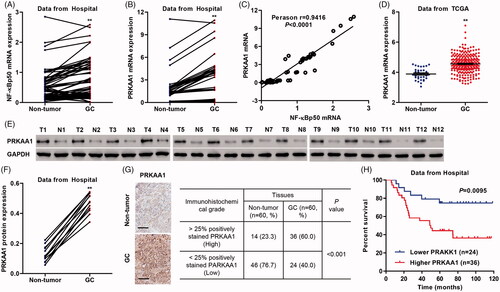
Table 1. Correlation of the expression of PRKAA1 with clinicopathologic features.
Table 2. Univariate and multivariate analysis of overall survival in patients with GC.
PRKAA1 knockdown inhibits GC cell invasion and migration in vitro
In view of the GSEA data that PRKAA1 expression is correlated with numerous metastasis signaling pathways (), and thus the biological role of PRAKK1 in regulating cell migration and invasion in vitro as well as metastasis in vivo was measured. To determine the role of PRKAA1 in the invasion and migration of GC cells in vitro, PRKAA1 was knockdown in BGC-823 and MKN-45 GC cells. shRNA-1, -2 and -3 targeting PRKAA1 markedly inhibited the PRKAA1 protein expression by 92.4, 41.2 and 60.5% in BGC-823 cells and by 79.2, 38.2 and 80.5% in MKN-45 cells compared with shNC group, respectively (). Therefore, shRNA-1 was used in our following experiments. In vitro Transwell assaydemonstrated that PRKAA1 down-regulation significantly suppressed the migration of MKN-45 and BGC-823 cells by 52.3 and 60.1% compared with shNC group, respectively (). PRKAA1 knockdown also markedly suppressed the invasion of BGC-823 and MKN-45 cells by 35.4 and 47.2% compared with shNC group, respectively (). Our results also revealed that PRKAA1 knockdown significantly inhibited the MMP-2 expression and the activation of NF-κB in both MKN-45 and BGC-823 cells ().
Figure 3. PRKAA1 knockdown inhibits the GC cell migration and invasion in vitro. (A) GSEA demonstrated the genes of metastasis were correlated with GC patients with PRKAA1 low vs. PRKAA1 high. (B, C) shRNA targeted PRKAA1 in both MKN-45 and BGC-823 cells significantly reduced PRKAA1 protein expression. MKN-45 and BGC-823 cells were transduced with shRNA targeted PRKAA1, and the migration (D, E) and invasion (F, G) were measured by Transwell assay. Scale bars: 100 μm. BGC-823 (H) and MKN-45 (I) cells were transduced with shRNA targeting PRKAA1 and the protein expression of MMP-2, p-NF-κBp65 and NF-κBp65 was measured by Western blotting. **p<.01 compared with shNC.
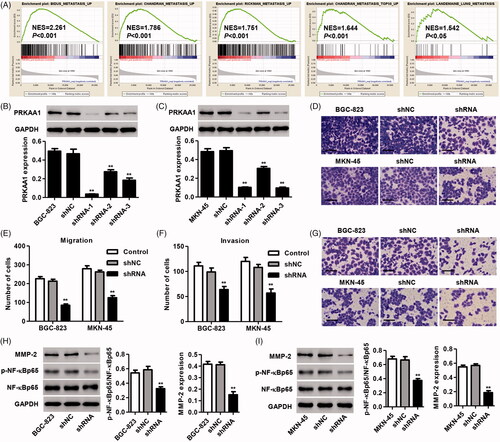
PRKAA1 knockdown inhibits GC cell metastasis in vivo
We further examined the effects of PRKAA1 on tumor metastasis in vivo. BGC-823 and MKN-45 cells stably transfecting PRKAA1 shRNA were injected into the tail vein of nude mice to observe the metastatic cells in the lungs. HE staining demonstrated that PRKAA1 knockdown significantly inhibited the lung metastasis of BGC-823 and MKN-45 cells compared with shNC group in vivo (). Moreover, the expression of p-NF-kBp65, NF-kBp65 and MMP-2 in the metastatic nodes was also examined. As shown in , PRKAA1 knockdown significantly inhibited MMP-2 expression and NF-κB activation.
Figure 4. PRKAA1 knockdown inhibits the lung metastasis of GC cells in vivo. MKN-45 and BGC-823 cells with PRKAA1 silencing were injected into the tail vein of the nude mice. Lung metastasis of MKN-45 and BGC-823 cells and expression of MMP-2, p-NF-κBp65 and NF-κBp65 in nude mice was measured by HE staining (A, B) and western blot (C, D). Scale bars: 100 μm. **p < .01 compared with shNC.
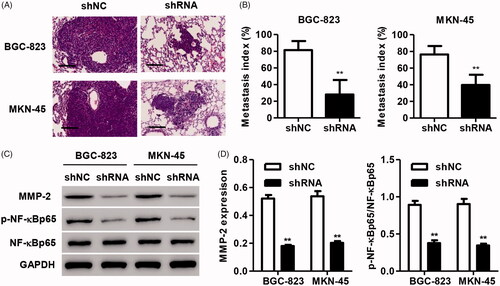
PRKAA1 involves in NF-κBp50-mediated GC cell migration and invasion
Having documented significant inhibition in the migration and invasion of GC cells transduced with PRKAA1 shRNA and the regulation of PRKAA1 by NF-κBp50, we tested the hypotheses that PRKAA1 would regulate NF-κBp50-mediated GC cell invasion and migration. As shown in , NF-κBp50 knockdown significantly decreased the NF-κBp50 protein expression by 80.6% while PRKAA1 overexpression significantly increased the PRKAA1 protein expression by 2.99-fold compared with siNC or vector group in MGC-803 cells. Transwell assay showed that NF-κBp50 knockdown significant decrease in the ability of migration and invasion of MGC-803 cells, respectively (. Furthermore, PRKAA1 overexpression inhibited NF-κBp50 knockdown-induced significant decrease in the ability of invasion and migration of MGC-803 cells, respectively (. NF-κBp50 knockdown induced the decreased MMP-2 expression and NF-κB activation was also inhibited by PRKAA1 overexpression (. Moreover, NF-κBp50 overexpression significantly increased the NF-κBp50 protein expression by 87.7% while PRKAA1 knockdown significantly decreased the PRKAA1 protein expression by 64.2% compared with vector or shNC group in MGC-803 cells (Figure S2A-D). In addition, NF-κBp50 overexpression-induced the increased cell migration, invasion, MMP-2 expression, and NF-κB activation were also inhibited by PRKAA1 knockdown (Figure S2E and F). These results indicate that PRKAA1 is involved in NF-κBp50-induced GC cell invasion and migration.
Figure 5. PRKAA1 overexpression inhibits NF-κBp50 knockdown-mediated GC cell migration and invasion. (A, B) NF-κBp50 knockdown in MGC-803 cells significantly decreased the NF-κBp50 protein expression. (C, D) PRKAA1 overexpression in MGC-803 cells significantly increased the PRKAA1 protein expression. MGC-803 cells were transfected with NF-κBp50 siRNA in the absence or presence of PRKAA1 overexpression. Transwell assay was performed for examining the cell invasion and migration (E), and the MMP-2, p-NF-κBp65 and NF-κBp65 expression was measured by Western blotting (F). Scale bars: 100 μm. **p < .01 compared with corresponding Vector or siNC. #p < .05, ##p < .01 compared with siNF-κBp50.
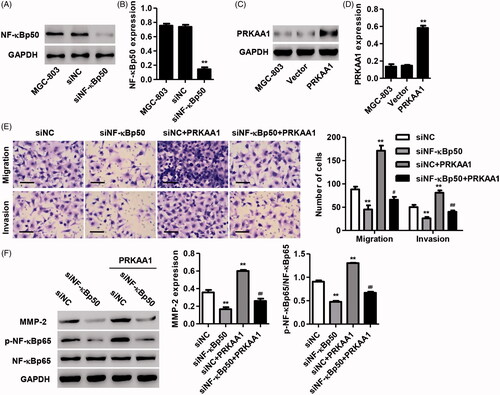
Discussion
Although H. pylori infection has been considered as an important risk in the development of peptic ulcers and chronic active gastritis, the relationship between H. pylori infection and GC progression remains unclear [Citation29,Citation30]. Helicobacter pylori infection promoted GC cell invasion, which was inhibited by NF-κB inhibitor, suggesting the involvement of NF-κB in H pylori-induced GC cell invasion [Citation24]. Helicobacter pylori infection was also verified to be positive interaction with PRKAA1 polymorphisms in GC [Citation31]. In the present study, H. pylori infection in GC cells significantly increased the p-NF-κBp50, NF-κBp50 and PRKAA1 expression. In contrast, H. pylori induced AMPK activation in human gastric epithelial cells (GECs) through increasing phosphorylation level of PRKAA1, but had no effect on the protein expression of PRKAA1 [Citation32]. These suggest that the regulation of H. pylori in PRKAA1 expression is dependent on the cell type. Besides, previous studies have reported that NF-κBp50 is associated with the regulation of miR-210 and Bcl-2 transcription [Citation33,Citation34], and NF-κBp50 significant enhancement on the COX-2 promoter activity and the interaction between them was increased by H. pylori infection [Citation24]. NF-κBp50 knockdown significantly decreased PRKAA1 expression through inhibiting the transactivation of PRKAA1 promoter. These findings suggest that H. pylori infection may increase PRKAA1 expression through enhancing NF-κBp50 expression and thus associate with GC cell migration and invasion.
Cell biological activities such as proliferation, migration, and invasion are necessary processes for the occurrence and development of malignant tumors, involving multiple genes and signaling pathways. PRKAA1 has been shown to be suppressed in primary human bladder cancers and T/B-cell acute lymphoblastic leukemia compared with non-tumor tissues [Citation35,Citation36]. However, Zhao et al. reported that PRKAA1 was overexpressed in human pancreatic, cervical and prostate cancer tissues [Citation37–39]. We demonstrated that the mRNA expression of PRKAA1 was overexpressed in 249 cases of GC tissues in TCGA database and the mRNA and protein expression levels of PRKAA1 were simultaneously overexpressed in GC tissues in Hospital, compared with that in their corresponding non-tumor gastric tissues. Linear regression showed that mRNA expression of NF-κBp50 was positively correlated with PRKAA1 mRNA expression in GC tissues, which further supported the positive regulation of PRKAA1 by NF-κBp50 in GC cell lines. These data suggest an oncogenic role of PRKAA1 in GC progression. Although the tremendous efforts have been made, the molecule mechanisms underlying the migration and invasion of GC remain largely unclear. In this study, we demonstrated that PRKAA1 expression was significantly correlated with TNM stage, pathologic grade, distant metastasis, H. pylori infection, and prognosis. To our knowledge, this is the first study to reveal the role and clinical relevance of PRKAA1 in GC.
Increasing evidence have been shown that PRKAA1 is associated with cellular energy metabolism. However, the role of PRKAA1 in cancer progression is controversial. Down-regulation of PRKAA1 inhibited pancreatic cancer cell growth in vitro and in vivo, along with induced cell apoptosis, cell cycle arrest, and inhibited invasion [Citation37]. PRKAA1 siRNA reversed oleic acid-induced the increase in cell growth and migration of HGC-27 and MDA-MB-231 cells [Citation40] and PRKAA1 overexpression induced the chondrosarcoma cancer cell migration [Citation41]. Similarly, PRKAA1 knockdown by siRNA transfection significantly reduced the inhibition of cell invasion of melanoma induced by berberine [Citation42]. In consistent with the previous studies, our results showed that PRKAA1 knockdown significantly inhibited GC cell migration and invasion in vitro and the lung metastasis of GC cells in vivo. Meanwhile, PRKAA1 overexpression obviously strengthened GC cell invasion and migration.
Matrix metalloproteinase is an important factor in the degradation and remodeling of the extracellular matrix. MMP-2 is an important member of the family, regulated by NF-κB signaling and closely related to the occurrence and metastasis of malignant tumors [Citation43,Citation44]. Inhibiting NF-κB signaling pathway significantly abolished the cell invasion and up-regulation of MMP-2 in GC cells [Citation45]. Our study showed that PRKAA1 knockdown significantly inhibited NF-κB activation and MMP-2 expression. These data suggest that PRKAA1 may regulate cell migration and invasion through NF-κB/MMP2 signaling pathway. In line with our findings that PRKAA1 knockdown significantly attenuated AICAR-induced NF-κB activation in human leukemic cell line THP-1 [Citation46]. Inhibiting NF-κB signaling and expression of MMP2 markedly suppressed the invasion and migration of B16F-10 melanoma cells and AGS gastric adenocarcinoma cells in vitro [Citation47,Citation48]. Our rescue experiments found that PRKAA1 overexpression significantly inhibited NF-κBp50 knockdown-mediated the decrease of GC cell invasion and migration while PRKAA1 knockdown significantly inhibited NF-κBp50 overexpression-induced the increase of GC cell invasion and migration, which indicated that PRKAA1 may associate with NF-κBp50-induced the invasion and migration of GC cells.
The recent study documenting the effect of PRKAA1 transcription regulated by NF-κBp50 on GC cell invasion and migration suggests that PRKAA1 is of great importance in gastric carcinogenesis and is an excellent therapeutic target for GC therapy.
Supplementary_information.docx
Download ()Disclosure statement
The authors declare that they have no competing interests.
Additional information
Funding
References
- Kamangar F, Dores GM, Anderson WF. Patterns of cancer incidence, mortality, and prevalence across five continents: defining priorities to reduce cancer disparities in different geographic regions of the world. JCO. 2006;24:2137–2150.
- Jemal A, Bray F, Center MM, et al. Global cancer statistics. CA Cancer J Clin. 2011;61:69–90.
- Ajani JA, D'Amico TA, Almhanna K, et al. Gastric cancer, version 3.2016, NCCN Clinical Practice Guidelines in Oncology. J Natl Compr Canc Netw. 2016;14:1286–1312.
- Sun M, Jin FY, Xia R, et al. Decreased expression of long noncoding RNA GAS5 indicates a poor prognosis and promotes cell proliferation in gastric cancer. BMC Cancer. 2014;14:319.
- Kim JH, Kim MA, Lee HS, et al. Comparative analysis of protein expressions in primary and metastatic gastric carcinomas. Human pathol. 2009;40:314–322.
- Guan-Zhen Y, Ying C, Can-Rong N, et al. Reduced protein expression of metastasis. Int J Exp Pathol. 2007;88:175–183.
- Mocellin S, Verdi D, Pooley KA, et al. Genetic variation and gastric cancer risk: a field synopsis and meta-analysis. Gut. 2015;64:1209–1219.
- Buckland G, Travier N, Huerta JM, et al. Healthy lifestyle index and risk of gastric adenocarcinoma in the EPIC cohort study. Int J Cancer. 2015;137:598–606.
- Chaturvedi R, de Sablet T, Asim M, et al. Increased Helicobacter pylori-associated gastric cancer risk in the Andean region of Colombia is mediated by spermine oxidase. Oncogene. 2015;34:3429–3440.
- Peek RM, Jr, Blaser MJ. Helicobacter pylori and gastrointestinal tract adenocarcinomas. Nat Rev Cancer. 2002;2:28–37.
- Jansen M, Ten Klooster JP, Offerhaus GJ, et al. LKB1 and AMPK family signaling: the intimate link between cell polarity and energy metabolism. Physiol Rev. 2009;89:777–798.
- Nakano A, Kato H, Watanabe T, et al. AMPK controls the speed of microtubule polymerization and directional cell migration through CLIP-170 phosphorylation. Nat Cell Biol. 2010;12:583–590.
- Marcus AI, Zhou W. LKB1 regulated pathways in lung cancer invasion and metastasis. J Thorac Oncol. 2010;5:1883–1886.
- Baur DM, Klotsche J, Hamnvik OP, et al. Type 2 diabetes mellitus and medications for type 2 diabetes mellitus are associated with risk for and mortality from cancer in a German primary care cohort. Metabolism. 2011;60:1363–1371.
- Choudhury Y, Yang Z, Ahmad I, et al. AMP-activated protein kinase (AMPK) as a potential therapeutic target independent of PI3K/Akt signaling in prostate cancer. Oncoscience. 2014;1:446–456.
- Tsai CH, Tsai HC, Huang HN, et al. Resistin promotes tumor metastasis by down-regulation of miR-519d through the AMPK/p38 signaling pathway in human chondrosarcoma cells. Oncotarget. 2015;6:258–270.
- Shi Y, Hu Z, Wu C, et al. A genome-wide association study identifies new susceptibility loci for non-cardia gastric cancer at 3q13.31 and 5p13.1. Nat Genet. 2011;43:1215–1218.
- Jones RG, Plas DR, Kubek S, et al. AMP-activated protein kinase induces a p53-dependent metabolic checkpoint. Mol Cell. 2005;18:283–293.
- Sag D, Carling D, Stout RD, et al. Adenosine 5'-monophosphate-activated protein kinase promotes macrophage polarization to an anti-inflammatory functional phenotype. J Immunol. 2008;181:8633–8641.
- Baldwin AS. Control of oncogenesis and cancer therapy resistance by the transcription factor NF-kappaB. J Clin Invest. 2001;107:241–246.
- Jiang W, Wang Z, Li X, et al. Reduced high-mobility group box 1 expression induced by RNA interference inhibits the bioactivity of hepatocellular carcinoma cell line HCCLM3. Dig Dis Sci. 2012;57:92–98.
- Yao X, Zhao G, Yang H, et al. Overexpression of high-mobility group box 1 correlates with tumor progression and poor prognosis in human colorectal carcinoma. J Cancer Res Clin Oncol. 2010;136:677–684.
- Stanojevic B, Dzodic R, Saenko V, et al. Mutational and clinico-pathological analysis of papillary thyroid carcinoma in Serbia. Endocr J. 2011;58:381–393.
- Wu CY, Wang CJ, Tseng CC, et al. Helicobacter pylori promote gastric cancer cells invasion through a NF-kappaB and COX-2-mediated pathway. WJG. 2005;11:3197–3203.
- Lee GR, Jang SH, Kim CJ, et al. Capsaicin suppresses the migration of cholangiocarcinoma cells by down-regulating matrix metalloproteinase-9 expression via the AMPK-NF-kappaB signaling pathway. Clin Exp Metastasis. 2014;31:897–907.
- Kim SY, Jeong S, Jung E, et al. AMP-activated protein kinase-alpha1 as an activating kinase of TGF-beta-activated kinase 1 has a key role in inflammatory signals. Cell death Dis. 2012;3:e357.
- Luo DW, Zheng Z, Wang H, et al. UPP mediated diabetic retinopathy via ROS/PARP and NF-kappaB inflammatory factor pathways. CMM. 2015;15:790–799.
- Lin B, Lee H, Yoon JG, et al. Global analysis of H3K4me3 and H3K27me3 profiles in glioblastoma stem cells and identification of SLC17A7 as a bivalent tumor suppressor gene. Oncotarget. 2015;6:5369–5381.
- Hoge S, Chaturvedi R, Fang Y, et al. Helicobacter pylori-induced epidermal growth factor receptor phosphorylation upregulates inducible nitric oxide synthase expression and nitric oxide production in macrophages. Gastroenterology. 2011;140:S-310.
- Cavaleiro-Pinto M, Peleteiro B, Lunet N, et al. Helicobacter pylori infection and gastric cardia cancer: systematic review and meta-analysis. Cancer Causes Control. 2011;22:375–387.
- Eom SY, Hong SM, Yim DH, et al. Additive interactions between PRKAA1 polymorphisms and Helicobacter pylori CagA infection associated with gastric cancer risk in Koreans. Cancer Med. 2016;5:3236–3335.
- Lv G, Zhu H, Zhou F, et al. AMP-activated protein kinase activation protects gastric epithelial cells from Helicobacter pylori-induced apoptosis. Biochem Biophys Res Commun. 2014;453:13–18.
- Kopriva SE, Chiasson VL, Mitchell BM, et al. TLR3-induced placental miR-210 down-regulates the STAT6/interleukin-4 pathway. PLoS One. 2013;8:e67760.
- Cheng EH, Wei MC, Weiler S, et al. BCL-2, BCL-X(L) sequester BH3 domain-only molecules preventing BAX- and BAK-mediated mitochondrial apoptosis. Mol Cell. 2001;8:705–711.
- Kopsiaftis S, Hegde P, Taylor JA, et al. AMPKalpha is suppressed in bladder cancer through macrophage-mediated mechanisms. Transl Oncol. 2016;9:606–616.
- Yi Y, Gao L, Wu M, et al. Metformin sensitizes leukemia cells to vincristine via activation of AMP-activated protein kinase. J Cancer. 2017;8:2636–2642.
- Zhao G, Zhang JG, Liu Y, et al. miR-148b functions as a tumor suppressor in pancreatic cancer by targeting AMPKalpha1. Mol Cancer Therap. 2013;12:83–93.
- Huang FY, Chiu PM, Tam KF, et al. Semi-quantitative fluorescent PCR analysis identifies PRKAA1 on chromosome 5 as a potential candidate cancer gene of cervical cancer. Gynecol Oncol. 2006;103:219–225.
- Park HU, Suy S, Danner M, et al. AMP-activated protein kinase promotes human prostate cancer cell growth and survival. Mol Cancer Therap. 2009;8:733–741.
- Li S, Zhou T, Li C, et al. High metastaticgastric and breast cancer cells consume oleic acid in an AMPK dependent manner. PLoS one. 2014;9:e97330.
- Chiu YC, Shieh DC, Tong KM, et al. Involvement of AdipoR receptor in adiponectin-induced motility and alpha2beta1 integrin upregulation in human chondrosarcoma cells. Carcinogenesis. 2009;30:1651–1659.
- Kim HS, Kim MJ, Kim EJ, et al. Berberine-induced AMPK activation inhibits the metastatic potential of melanoma cells via reduction of ERK activity and COX-2 protein expression. Biochem Pharmacol. 2012;83:385–394.
- Li J, Lau GK, Chen L, et al. Interleukin 17A promotes hepatocellular carcinoma metastasis via NF-kB induced matrix metalloproteinases 2 and 9 expression. PLoS One. 2011;6:e21816.
- Zeng Q, Li S, Zhou Y, et al. Interleukin-32 contributes to invasion and metastasis of primary lung adenocarcinoma via NF-kappaB induced matrix metalloproteinases 2 and 9 expression. Cytokine. 2014;65:24–32.
- Wang Y, Wu H, Wu X, et al. Interleukin 17A promotes gastric cancer invasiveness via NF-kappaB mediated matrix metalloproteinases 2 and 9 expression. PLoS one. 2014;9:e96678.
- Wang W, Chen J, Li XG, et al. Anti-inflammatory activities of fenoterol through beta-arrestin-2 and inhibition of AMPK and NF-kappaB activation in AICAR-induced THP-1 cells. Biomed Pharmacother. 2016;84:185–190.
- Hamsa TP, Kuttan G. Inhibition of invasion and experimental metastasis of murine melanoma cells by Ipomoea obscura (L.) is mediated through the down-regulation of inflammatory mediators and matrix-metalloproteinases. J Exp Therap Oncol. 2011;9:139–151.
- Ho HH, Chang CS, Ho WC, et al. Anti-metastasis effects of gallic acid on gastric cancer cells involves inhibition of NF-kappaB activity and downregulation of PI3K/AKT/small GTPase signals. Food Chemical Toxicol. 2010;48:2508–2516.