Abstract
Matrine derivative MASM (M19) is a quinolizine alkaloid with diverse pharmacological effects including preventing postmenopausal osteoporosis. In the current study, we observed that pretreatment with M19 inhibited cell apoptosis of murine osteoblastic MC3T3-E1 and primary osteoblasts induced by 1 μM dexamethasone in a dose-dependent manner. Our study also showed that pretreatment with M19 significantly prevented the upregulation of p53 that is caused by dexamethasone but had no effect on the p53 mRNA expression levels. Further immunoprecipitation (IP) experiments showed that M19 treatment increases the ubiquitination of p53 in dexamethasone-treated MC3T3-E1 cells. The expression of USP14, a deubiquitinating enzyme, increased with dexamethasone and decreased with M19 pretreatment. Co-IP experiments demonstrated the interaction between USP14 and p53, and the induced effect of a USP14 inhibitor (IU1) on p53 ubiquitination, which indicated that USP14 is a potential deubiquitinase for p53. Furthermore, pretreatment with IU1 or a p53 inhibitor (PFT-α) partially blocked dexamethasone-induced apoptosis of MC3T3-E1 cells. The overexpression of USP14 or p53 reversed the antiapoptotic effect of M19 in dexamethasone-treated MC3T3-E1 cells. In addition, PFT-α treatment remarkably blocked the effects of USP14 overexpression. In summary, our findings suggest that M19 exerts protective effects on dexamethasone-treated MC3T3-E1 cells by regulating USP14/p53.
Introduction
Glucocorticoids (GCs) are commonly used in the treatment of various chronic diseases due to their anti-inflammatory and immunosuppressive activities [Citation1]. However, long-term GC therapy and/or excessive GCs (i.e. dexamethasone) is one of the common causes of osteonecrosis and osteoporosis [Citation2–4]. Accumulated evidence from in vitro and in vivo experiments has strongly suggested that the proapoptotic effect of GCs on osteoblasts results in the inhibition of bone growth and formation [Citation5–8]. Li et al. have reported that the role of dexamethasone is mainly mediated by the activation of the cytosolic glucocorticoid receptor (GR), which, in turn, causes p53 activation, thus inducing the apoptosis of osteoblastic MC3T3-E1 cells [Citation9]. Numerous studies have been conducted to explore the mechanisms of p53 activation [Citation10]. It is widely accepted that the ubiquitin–proteasome pathway plays a major role in controlling the degradation of p53 [Citation11]. Moreover, various deubiquitinating enzymes such as USP5 [Citation12], USP7 [Citation13], USP10 [Citation14] and USP13 [Citation14], have been found to increase the stability of p53 by removing ubiquitin from p53. However, whether deubiquitinating enzymes are involved in the regulation of p53 in GC-treated osteoblasts remains unclear.
Matrine (C15H24N2O), a quinolizine alkaloid, is widely found in the roots of traditional Chinese herbs such as Sophora flavescens (Kushen), Sophora tonkinensis and Sophora alopecuroides (Kudouzi) [Citation15]. Matrine exhibits a variety of pharmacological effects, such as anti-viral [Citation16], anti-inflammatory [Citation17,Citation18], analgesic [Citation19], anti-fibrotic [Citation20], antitumor [Citation21–23] and anti-osteoporotic [Citation24]. Compared with matrine, the derivative M19 (6aS,10S,11aR,11bR,11cS-10-methylamino-dodecahydro-3a,7a-diazabenzo[de]anthracene-8-thione, C16N3H27S) presents a superior inhibitory effect on the Akt and nuclear factor-κB (NF-κB) pathways and thus has been suggested as a promising agent in the treatment of postmenopausal osteoporosis [Citation25–27]. However, the role of M19 in dexamethasone-induced apoptosis of osteoblasts has not been explored to date.
In the current study, we aimed to explore whether M19 protects osteoblastic MC3T3-E1 cells against dexamethasone-induced apoptosis and to investigate the underlying mechanism. We found that USP14 may function as a deubiquitinase for p53, and that M19 exerts antiapoptotic effects by inhibiting USP14 in dexamethasone-treated osteoblasts.
Materials and methods
Cell culture and treatment
MC3T3-E1 cells, which were obtained from the Shanghai Institute of Biochemistry and Cell Biology (Shanghai, China), were grown in Dulbecco’s modified Eagle medium (Hyclone, Logan, UT) containing 10% fetal bovine serum (FBS, Gibco, Carlsbad, CA), 100 µg/mL streptomycin, and 100 U/m: penicillin (Solarbio, Beijing, China). The cells were maintained at 37 °C and in a humidified atmosphere containing 5% CO2.
Primary osteoblasts were isolated from the calvariae of C57BL/6 mice (3-day-old) as previously described [Citation28]. Briefly, calvariae were excised and digested in 2 mg/mL collagenase A (Sigma-Aldrich, St. Louis, MO) and 0.25% trypsin for 20, 40 and 90 min at 37 °C. Cells from the third digest were collected and grown in minimal essential medium (Hyclone) supplemented with 10% FBS for subsequent experiments. The animal experiments were approved by Animal Care Committee of Naval Military Medical University (Shanghai, China).
M19 was synthesized as previously described by Prof. Zhao, QJ from the School of Pharmacy, Second Military Medical University (Shanghai, China). MC3T3-E1 and primary osteoblasts were pretreated with increasing concentrations of M19 (0, 1, 2 and 4 µM) for 1 h, and then exposed to 1 µM dexamethasone (D4902; Sigma-Aldrich) to explore the cytoprotective effects of M19. Then, a USP14 inhibitor, IU1 (S7134, Selleck Chemicals, Houston, TX) or a p53 inhibitor, PFT-α (S2929, Selleck Chemicals) were used to elucidate the underlying mechanism. Finally, the MC3T3-E1 cells were exposed to 4 µM M19 with USP14 or p53 overexpression in the presence of 1 µM dexamethasone. The MC3T3-E1 cells treated with vehicle (DMSO) and used as control.
Plasmid construction
Full-length murine USP14 and p53 were amplified using polymerase chain reaction (PCR) with the following primers: USP14, forward 5′-CGGAATTCATGCCACTCTACTCTGTTACAG-3′ and reverse 5′-CGGGATCCTTACTGTTCACTTTCTTCTTCC-3′; p53, forward 5′-CGGAATTCATGACTGCCATGGAGGAGTC-3′ and reverse 5′-CGGGATCCTCAGTCTGAGTCAGGCCCCAC-3′. Following digestion with EcoRI and BamHI, the fragments were cloned into pLVX-puro (Addgene, Cambridge, MA). The vector pLVX-puro was used as negative control.
Lentiviral production and transduction
Lentiviruses were produced using 293T cells. Briefly, the 293T cells were co-transfected with lentiviral plasmids, psPAX2 and pMD2G (Addgene), using Lipofectamine® 2000 (Invitrogen, Carlsbad, CA), following the manufacturer’s protocol. At 48–72 h post-transfection, the culture media containing the lentiviruses was collected and used to infect MC3T3-E1 cells.
Cell apoptosis assay
Cell apoptosis was assessed by double staining with Annexin V-fluorescein isothiocyanate (FITC) and propidium iodide (PI) followed by flow cytometry analysis. The MC3T3-E1 cells were plated in six-well plates at a density of 3 × 105 cells/well and treated as indicated. The cells were digested with trypsin, pelleted, and stained with Annexin V- FITC (Beyotime, Haimen, China) and PI for 10 min in the dark at room temperature according to the manufacturer’s instructions. Subsequently, the cells were subjected to flow cytometric analysis (BD Accuri C6; BD Biosciences, Franklin Lakes, NJ).
Real-time PCR assay
Total RNA was isolated using TRIzol® reagent (Invitrogen), and reverse-transcribed to first-strand cDNA with a cDNA synthesis kit (Thermo Fisher Scientific, Rockford, IL) according to manufacturers’ protocols. Real-time PCR was conducted with SYBR Green (Thermo Fisher Scientific) using an ABI 7500 (Applied Biosystems; Foster City, CA). GAPDH was simultaneously amplified as internal control. The primer sequences are listed in .
Table 1. Primers for real-time PCR.
Western blot analysis
MC3T3-E1 cells were lysed in ice-cold cell lysis solution (Solarbio, Beijing, China). A bicinchoninic acid protein assay kit (Thermo Fisher Scientific) was used to determine protein concentrations. Equal amounts (30 μg) of protein from each sample were resolved by SDS-12% PAGE, and then electrotransferred onto nitrocellulose membranes. After blocking nonspecific binding with 5% fat-free milk in TBST (10 mM Tris, pH 7.5; 500 mM NaCl; 0.05% Tween 20), the membranes were incubated with primary antibodies specific to USP14 (1:1000; ab137432; Abcam, Cambridge, MA), p53 (1:1000; ab131442; Abcam), Bax (1:2000; ab32503; Abcam), Bcl-2 (1:2000; ab182858; Abcam), ubiquitin (1:1000; ab7780; Abcam), and GAPDH (1:2000; #9743; Cell Signaling Technology, Danvers, MA) overnight at 4 °C. After three washes with TBST, the membranes were incubated with horseradish peroxidase-conjugated secondary antibodies (1:1000; A0208; Beyotime) for 1 h at room temperature. Then, the membranes were washed thrice with TBST, and staining was visualized using an enhanced chemiluminescence kit (Millipore, Billerica, MA). The band density was quantified by Image J software (ImageJ, Bethesda, MD) and normalized to that of GAPDH.
Immunoprecipitation
Cell lysates were precleared with protein A/G PLUS-Sepharose beads (Santa Cruz, Santa Cruz, CA) at 4 °C for 1 h, incubated with anti-USP14 (ab137433, Abcam) or anti-p53 (ab31333, Abcam) or IgG (Santa Cruz) at 4 °C overnight, and then with protein A/G PLUS-Sepharose beads (Santa Cruz) at 4 °C for 2 h. The precipitates were washed with TBST thrice to eliminate nonspecific binding, boiled in Laemmli sample buffer for 5 min, and then subjected to western blotting analysis.
Statistical analysis
All data are presented as the mean ± standard deviation (SD). Statistical analyses were conducted with GraphPad Prism 5 software (GraphPad Software, La Jolla, CA). One-way ANOVA with Sidak’s multiple comparison test was used to compare data from various groups. Statistical significance was set at p < .05.
Results
M19 improves dexamethasone-induced apoptosis of osteobalsts
From our preliminary experiments, we found that dexamethasone at a dose of 1 μM inhibited the proliferation of MC3T3-E1 cells by inducing cell apoptosis (data are not shown). To elucidate the potential cytoprotective effects of M19, MC3T3-E1 cells were pretreated with 0, 1, 2 and 4 µM of M19 for 1 h and exposed to 1 µM of dexamethasone for 48 h. As measured by Annexin V/PI staining and flow cytometry, 1 μM dexamethasone led to a marked increase in apoptotic rate, and pretreatment with M19 at 1, 2 and 4 µM significantly reduced the apoptotic rate in dexamethasone-exposed MC3T3-E1 cells (). Similar results were achieved in murine primary osteoblasts. These findings suggest that M19 possesses anti-apoptotic activity in osteoblasts exposed to dexamethasone.
Figure 1. M19 improves dexamethasone-induced apoptosis of osteobalsts. MC3T3-E1 cells (A) and primary osteoblasts (B) were pretreated with different doses of M19 (1, 2 and 4 µM) or vehicle (Veh, DMSO) for 1 h, and then exposed to 1 µM dexamethasone (DEX) for 48 h. Cell apoptosis was assessed by Annexin V/PI staining and flow cytometry analysis. Cells treated with vehicle (Veh, DMSO) only were used as negative control. All data are presented as the mean ± standard deviation. n = 3 independent experiments. ***p < .001 vs. Veh; ###p < .001 vs. Veh + DEX; +++p < .001 vs. 1 µM M19 + DEX; -p < .05 vs. 2 µM M19 + DEX.
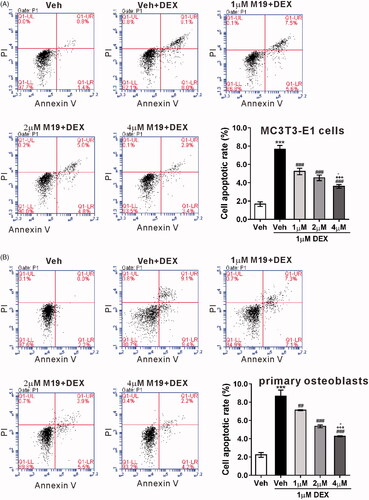
M19 induces p53 ubiquitination in dexamethasone-treated MC3T3-E1 cells
Evidence has shown that dexamethasone can upregulate the expression and transcriptional activity of p53, thus inducing osteoblasts apoptosis [Citation9]. The protein and mRNA levels of p53 were detected. The western blotting analysis showed that treatment with 1 µM dexamethasone for 48 h obviously elevated the protein expression of p53, which was noticeably attenuated by pretreatment with 1, 2 and 4 µM M19 for 1 h (). qRT-PCR analysis showed that the mRNA expression of p53 did not significantly vary (), which indicated that the alteration of p53 expression was at the post-translational level. As shown in , the ubiquitination of p53 was markedly enhanced by M19 pretreatment (4 µM) in MC3T3-E1 cells with 1 µM dexamethasone. These data imply that M19 may reduce p53 stabilization via increased ubiquitination in dexamethasone-treated MC3T3-E1 cells.
Figure 2. M19 induces p53 ubiquitination in dexamethasone-treated MC3T3-E1 cells. (A, B) MC3T3-E1 cells were pretreated with different doses of M19 (1, 2 and 4 µM) or vehicle (Veh, DMSO) for 1 h, and then exposed to 1 µM dexamethasone (DEX) for 48 h. Cells treated with vehicle (Veh, DMSO) alone were used as negative control. p53 protein (A) and mRNA expression (B) were evaluated by western blotting and real-time PCR, respectively. Representative blots from three experiments are shown. All data are presented as the mean ± standard deviation. n = 3 independent experiments. ***p < .001 vs. Veh; #p < .05, ###p < .001 vs. Veh + DEX; +p < .05, +++p < .001 vs. 1 µM M19 + DEX; -p < .05 vs. 2 µM M19 + DEX. (C) MC3T3-E1 cells were pretreated with 4 µM M19 or vehicle (Veh, DMSO) for 1 h, and then exposed to 1 µM DEX for 48 h. p53 ubiquitination was analyzed by immunoprecipitation (IP) with an anti-p53 antibody followed by immunoblotting using anti-Ub.
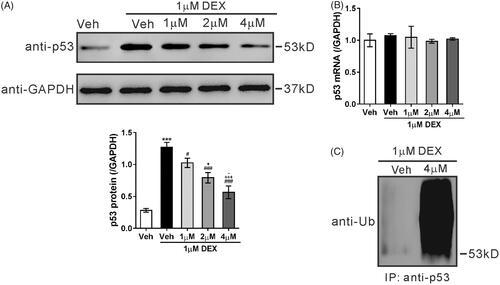
USP14 is a potential deubiquitinase for p53
USP family proteins are capable of removing ubiquitin from p53 and increase the stability of p53 [Citation12,Citation13,Citation14]. To find the possible deubiquitinase for p53, the mRNA levels of several USPs were then detected in MC3T3-E1 cells treated with 1 µM dexamethasone. The most significant increase was observed in USP14 (). Subsequently, the protein levels of USP14 in M19 and dexamethasone-treated ME3T3-E1 cells were detected. As illustrated in , USP14 protein expression was also increased by dexamethasone, and dose-dependently decreased by pretreatment with M19. Co-IP experiments confirmed the interaction of p53 and USP14 (). The inhibitor of USP14, IU1, significantly elevated the ubiquitination of p53 (). These data suggest that USP14 is a potential deubiquitinase for p53 and that USP14 mediates the response of MC3TC-E1 cells to dexamethasone and M19.
Figure 3. USP14 is a potential deubiquitinase for p53. (A) MC3T3-E1 cells were exposed to 1 µM dexamethasone (DEX) or vehicle (Veh, DMSO) for 48 h. mRNA levels of USP family proteins were detected by real-time PCR. n = 3 independent experiments. *p < .05, **p < .01 vs. Veh. (B) MC3T3-E1 cells were pretreated with different doses of M19 (1, 2 and 4 µM) or vehicle (Veh, DMSO) for 1 h, and then exposed to 1 µM DEX for 48 h. Cells treated with vehicle (Veh, DMSO) only were used as negative control. USP14 protein expression was detected by western blotting. Representative blots from three experiments are shown. n = 3 independent experiments. ***p < .001 vs. Veh; ##p < .01, ###p < .001 vs. Veh + DEX; (C) MC3T3-E1 cell lysate was immunoprecipitated (IP) with anti-USP14 (upper panel) and anti-p53 (lower panel), and immunoblotted with anti-USP14 and anti-p53. IgG was used as a negative control (D) MC3T3-E1 cells were treated with 50 μM IU1 or vehicle (Veh, DMSO) for 48 h. Cell lysates were prepared, IP with anti-p53 or IgG and the immunoblotted with anti-Ub.
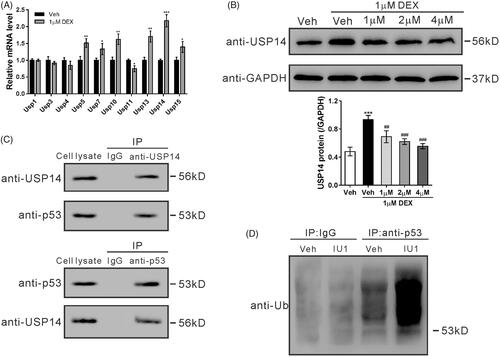
Upregulation of USP14 and p53 contributes to dexamethasone-induced apoptosis of MC3T3-E1 cells
To investigate the role of USP14 and p53 in dexamethasone-treated MC3T3-E1 cells, IU1 (25 and 50 μM) and a p53 inhibitor, pFT-α, were applied. As shown in , treatment with IU1 (50 μM) or PFT-α partially blocked dexamethasone-induced apoptosis of MC3T3-E1 cells. Simultaneously, treatment with IU1 or PFT-α also repressed the dexamethasone-induced protein expression of the antiapoptotic factor Bcl-2, and the dexamethasone-decreased protein expression of p53 and pro-apoptotic factor Bax in MC3T3-E1 cells ().
Figure 4. Upregulation of USP14 and p53 contributes to dexamethasone-induced apoptosis of MC3T3-E1 cells. MC3T3-E1 cells were pretreated with vehicle (Veh, DMSO), 25 μM IU1, 50 μM IU1, or 20 μM PFT-α for 1 h, and then with 1 µM dexamethasone (DEX) for 48 h. (A) Cell apoptosis was analyzed by flow cytometry. (B) Protein levels of p53, Bcl-2 and Bax were detected by western blotting. Representative blots from three experiments are shown. n = 3 independent experiments. ***p < .001 vs. Veh; #p < .05, ##p < .01, ###p < .001 vs. Veh + DEX.
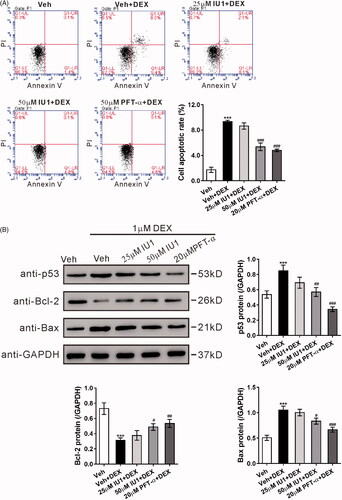
Overexpression of USP14 attenuates the protective effect of M19 in dexamethasone-treated MC3T3-E1 cells by regulating p53
To further explore the mechanism underlying the cytoprotective effect of M19, lentivirus overexpressing USP14, overexpressing p53, or vector were constructed to infect MC3T3-E1 cells, and the overexpression of USP14 () and p53 () was confirmed by western blotting.
Figure 5. Overexpression of USP14 attenuates the protective effect of M19 in dexamethasone-treated MC3T3-E1 cells by regulating p53. (A, B) MC3T3-E1 cells were transduced with lentivirus overexpressing USP14 (A), p53 (B) or vector for 48 h. The overexpression of USP14 and p53 was confirmed by western blotting. (C, D) MC3T3-E1 cells were transduced with lentivirus overexpressing USP14, p53 or vector for 24 h, treated with vehicle (Veh, DMSO) or 4 µM M19 or 4 µM M19 plus 20 μM PFT-α for 1 h, and then exposed to 1 µM dexamethasone (DEX) for 48 h. Cell apoptosis (C) was analyzed by flow cytometry. Protein levels of p53, Bcl-2 and Bax (D) were detected by western blotting. Representative blots from three experiments are shown. Three independent experiments were conducted. ***p < .001 vs. Veh; ##p < .01, ###p < .001 vs. Vector + Veh + DEX; +p < .05, +++p < .001 vs. Vector + M19 + DEX; --p < .01, ---p < .001 vs. USP14 + M19 + DEX. (E) Schematic representation of the regulation of MC3T3-E1 apoptosis by dexamethasone.
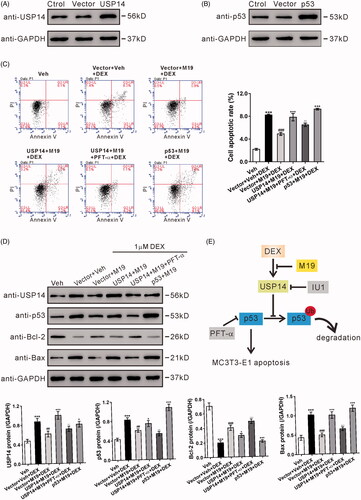
MC3T3-E1 cells were infected with lentivirus overexpressing USP14, overexpressing p53 or vector, pretreated with 4 µM M19 and then with 1 μM dexamethasone. shows that the antiapoptotic effect of M19 on dexamethasone-treated MC3T3-E1 cells was reversed by the overexpression of USP14 or p53 (). Moreover, PFT-α treatment remarkably blocked the effects of USP14 overexpression. These findings further suggest that M19 imparts a protective effect on dexamethasone-treated MC3T3-E1 cells through USP14/p53. Further, the protein expressions of USP14, p53, Bcl-2 and Bax () were also detected, and the results were consistent with the flow cytometry data.
Discussion
Approximately 30% of the patients receiving long-term GC therapy suffer from osteoporosis [Citation2]. Increased osteoblast apoptosis and a reduced number of osteoblasts have been observed in the histological images of these patients [Citation2,Citation4]. Matrine and its derivate have been shown to prevent postmenopausal osteoporosis [Citation24,Citation25]. In the present study, we show that M19 could protect osteoblastic MC3T3-E1 cells from dexamethasone-induced apoptosis, and this antiapoptotic effect involves the regulation of USP14/p53.
The rate of bone formation and resorption is highly dependent on the number of osteoblasts and osteoclasts in the basic multicellular units. Besides the production of mature cells, the number of bone cells is controlled by their apoptosis. Osteoblast apoptosis could significantly affect the number of osteoblasts [Citation29]. Accumulated evidence has supported the proapoptotic effects of GCs on osteoblasts [Citation5–8]. Previous studies have shown the proapoptotic effects of matrine on various cancer cells by regulating caspase, Bcl-2 family proteins, or JAK2/STAT3 signaling [Citation30–34]. On the contrary, matrine was found to inhibit the apoptosis of neurons [Citation35] and cardiomyocytes [Citation36], thus attenuating cerebral ischemic injury in a mouse model and heart failure in a rat model. Here, as a derivative of matrine, M19 dose-dependently inhibited dexamethasone-induced MC3T3-E1 cell apoptosis (), suggesting its role in bone growth and formation. The previous findings and our data suggest that the effects of M19 on cell apoptosis are dependent on the activation or regulation of different key proteins.
Dexamethasone is reported to activate GR, which, in turn, causes p53 activation, thus inducing the apoptosis of osteoblastic MC3T3-E1 cells [Citation9]. Recent studies have demonstrated how p53 promotes cell apoptosis [Citation37]. It is well-known that the ubiquitin–proteasome pathway is involved in the control of degradation and activity of p53 [Citation11]. Here, pretreatment with M19 significantly reduced the induced effect of dexamethasone on p53 protein expression (), whereas p53 mRNA expression was not affected by dexamethasone or M19 (). These data suggest that p53 is regulated at the post-translational level in this system, which is consistent with the previous studies that the regulation of p53 was still dominated by changes at the protein level, including post-translational modifications, protein stability regulation, and protein–protein interactions [Citation38,Citation39]. Moreover, M19 treatment increased the ubiquitination of p53 (). We then tried to explore the molecules responsible for the regulation of p53 ubiquitination in our system. USP family proteins have been found to increase p53 stabilization by removing ubiquitin from p53. Here, a member of USPs, USP14, was found to be most significantly upregulated in response to dexamethasone exposure (). Further IP experiments demonstrated the interaction between USP14 and p53 (), and the induced effect of USP14 inhibitor on p53 ubiquitination (). These data partially demonstrated that USP14 is a potential deubiquitinase for p53, although further experiments are needed to confirm the observation.
The involvement of p53 and USP14 in dexamethasone-induced apoptosis of MC3T3-E1 cells was explored by pre-exposure to a p53 inhibitor (PFT-α) or a USP14 inhibitor (IU1). Pretreatment with 20-μM PFT-α partially blocked the dexamethasone-induced apoptosis of MC3T3-E1 cells (), which was consistent with a previous study [Citation40]. A similar effect was observed in cells pretreated with 50 μM IU1. The proapoptotic factor Bax and the antiapoptotic factor Bcl-2 have been identified as p53-regulated genes [Citation41]. The expression of p53, Bax and Bcl-2 was in line with the results of flow cytometry analysis (). These findings indicate that the upregulation of USP14 and p53 contributes to dexamethasone-induced apoptosis of MC3T3-E1 cells.
The involvement of USP14 and p53 in the protective effect of M19 on dexamethasone-treated osteoblastic cells was also investigated (. The antiapoptotic effect of M19 on dexamethasone-treated MC3T3-E1 cells was reversed by the overexpression of USP14 or p53. In addition, p53 inhibitor treatment remarkably blocked the effects of USP14 overexpression, further suggesting that M19 possesses a protective effect on dexamethasone-treated MC3T3-E1 cells through USP14/p53.
In conclusion, it was observed that dexamethasone induced the apoptosis of MC3T3-E1 cells by increasing the expression of USP14, which is a potential deubiquitinase for p53, and stabilized p53 (). We also illustrated that M19 could abolish the effects of dexamethasone on MC3T3-E1 cells, although the mechanisms underlying how M19 regulates USP14 expression are yet to be explored. The next step is to use animal model to investigate the direct effects of M19 on the apoptosis of osteoblasts and further explore the therapeutic effects of M19 on osteoporosis.
Abbreviations | ||
GC | = | glucocorticoids |
GR | = | glucocorticoid receptor |
NF-κB | = | nuclear factor-κB |
FITC | = | fluorescein isothiocyanate |
PI | = | propidium iodide |
IP | = | immunoprecipitation |
SD | = | standard deviation |
Disclosure statement
No conflicts of interest, financial or otherwise, are declared by the authors.
Additional information
Funding
References
- Schäcke H, Döcke W-D, Asadullah K. Mechanisms involved in the side effects of glucocorticoids. Pharmacol Ther. 2002;96(1):23–43.
- den Uyl D, Bultink IE, Lems WF. Advances in glucocorticoid-induced osteoporosis. Curr Rheumatol Rep. 2011;13(3):233–240.
- Civitelli R, Ziambaras K. Epidemiology of glucocorticoid-induced osteoporosis. J Endocrinol Investig. 2008;31(7):2–6.
- Weinstein RS. Clinical practice. Glucocorticoid-induced bone disease. N Engl J Med. 2011;365(1):62–70.
- Weinstein RS, Jilka RL, Parfitt AM, et al. Inhibition of osteoblastogenesis and promotion of apoptosis of osteoblasts and osteocytes by glucocorticoids. Potential mechanisms of their deleterious effects on bone. J Clin Invest. 1998;102(2):274–282.
- Gohel A, McCarthy M-B, Gronowicz G. Estrogen prevents glucocorticoid-induced apoptosis in osteoblasts in vivo and in vitro. Endocrinology. 1999;140(11):5339–5347.
- Canalis E. Mechanisms of glucocorticoid action in bone. Curr Osteoporos Rep. 2005;3(3):98–102.
- O’brien CA, Jia D, Plotkin LI, et al. Glucocorticoids act directly on osteoblasts and osteocytes to induce their apoptosis and reduce bone formation and strength. Endocrinology. 2004;145(4):1835–1841.
- Li H, Qian W, Weng X, et al. Glucocorticoid receptor and sequential P53 activation by dexamethasone mediates apoptosis and cell cycle arrest of osteoblastic MC3T3-E1 cells. PLoS One. 2012;7(6):e37030.
- Dai C, Gu W. p53 post-translational modification: deregulated in tumorigenesis. Trends in Mol Med. 2010;16(11):528–536.
- Lee J, Gu W. The multiple levels of regulation by p53 ubiquitination. Cell Death Differ. 2010;17(1):86.
- Dayal S, Sparks A, Jacob J, et al. Suppression of the deubiquitinating enzyme USP5 causes the accumulation of unanchored polyubiquitin and the activation of p53. J Biol Chem. 2009;284(8):5030–5041.
- Li M, Chen D, Shiloh A, et al. Deubiquitination of p53 by HAUSP is an important pathway for p53 stabilization. Nature. 2002;416(6881):648.
- Liu J, Xia H, Kim M, et al. Beclin1 controls the levels of p53 by regulating the deubiquitination activity of USP10 and USP13. Cell. 2011;147(1):223–234.
- Huang J, Xu H. Matrine: bioactivities and structural modifications. Curr Top Med Chem. 2016;16(28):3365–3378.
- Yang Y, Xiu J, Zhang X, et al. Antiviral effect of matrine against human enterovirus 71. Molecules. 2012;17(9):10370–10376.
- Sun D, Wang J, Yang N, et al. Matrine suppresses airway inflammation by downregulating SOCS3 expression via inhibition of NF-κB signaling in airway epithelial cells and asthmatic mice. Biochem Biophys Res Commun. 2016;477(1):83–90.
- Kan Q-C, Zhu L, Liu N, et al. Matrine suppresses expression of adhesion molecules and chemokines as a mechanism underlying its therapeutic effect in CNS autoimmunity. Immunol Res. 2013;56(1):189–196.
- Xu X, Yan Y, Pan J, et al. Exploring antipruritic and analgesic effects of matrine by allergic contact dermatitis mouse model through behavioral analysis. J Pharma Biomed Sci. 2017;7(4):77–81.
- Liu Z, Zhang Y, Tang Z, et al. Matrine attenuates cardiac fibrosis by affecting ATF6 signaling pathway in diabetic cardiomyopathy. Eur J Pharmacol. 2017;804:21–30.
- Li H, Tan G, Jiang X, et al. Therapeutic effects of matrine on primary and metastatic breast cancer. Am J Chin Med. 2010;38(06):1115–1130.
- Hu MJ, Zeng H, Wu YL, et al. Synergistic effects of matrine and 5‐fluorouracil on tumor growth of the implanted gastric cancer in nude mice. Chin Dig Dis. 2005;6(2):68–71.
- Cho Y, Lee JH, Kim JH, et al. Matrine suppresses KRAS‐driven pancreatic cancer growth by inhibiting autophagy‐mediated energy metabolism. Mol Oncol. 2018;12(7):1203.
- Chen X, Zhi X, Pan P, et al. Matrine prevents bone loss in ovariectomized mice by inhibiting RANKL-induced osteoclastogenesis. The FASEB J. 2017;31(11):4855–4865.
- Chen X, Zhi X, Cao L, et al. Matrine derivate MASM uncovers a novel function for ribosomal protein S5 in osteoclastogenesis and postmenopausal osteoporosis. Cell death Dis. 2017;8(9):e3037.
- Yu B, Chang J, Liu Y, et al. Wnt4 signaling prevents skeletal aging and inflammation by inhibiting nuclear factor-kappaB. Nat Med. 2014;20(9):1009–1017.
- Liu Y, Qi Y, Bai Z-h, et al. A novel matrine derivate inhibits differentiated human hepatoma cells and hepatic cancer stem-like cells by suppressing PI3K/AKT signaling pathways. Acta Pharmacol Sin. 2017;38(1):120.
- Kaneshiro S, Ebina K, Shi K, et al. IL-6 negatively regulates osteoblast differentiation through the SHP2/MEK2 and SHP2/Akt2 pathways in vitro. J Bone Min Metabol. 2014;32(4):378–392.
- Manolagas SC. Birth and death of bone cells: basic regulatory mechanisms and implications for the pathogenesis and treatment of osteoporosis. Endocrine Rev. 2000;21(2):115–137.
- Liu X-S, Jiang J, Jiao X-Y, et al. Matrine-induced apoptosis in leukemia U937 cells: involvement of caspases activation and MAPK-independent pathways. Planta med. 2006;72(6):501–506.
- Luo C, Zhu Y, Jiang T, et al. Matrine induced gastric cancer MKN45 cells apoptosis via increasing pro-apoptotic molecules of Bcl-2 family. Toxicology. 2007;229(3):245–252.
- Liu T, Song Y, Chen H, et al. Matrine inhibits proliferation and induces apoptosis of pancreatic cancer cells in vitro and in vivo. Biol Pharma Bull. 2010;33(10):1740–1745.
- Liang CZ, Zhang JK, Shi Z, et al. Matrine induces caspase-dependent apoptosis in human osteosarcoma cells in vitro and in vivo through the upregulation of Bax and Fas/FasL and downregulation of Bcl-2. Cancer Chemother Pharmacol. 2012;69(2):317–331.
- Yang N, Han F, Cui H, et al. Matrine suppresses proliferation and induces apoptosis in human cholangiocarcinoma cells through suppression of JAK2/STAT3 signaling. Pharmacol Rep. 2015;67(2):388–393.
- Zhao P, Zhou R, Zhu X-Y, et al. Matrine attenuates focal cerebral ischemic injury by improving antioxidant activity and inhibiting apoptosis in mice. Int J Mol Med. 2015;36(3):633–644.
- Yu J, Yang S, Wang X, et al. Matrine improved the function of heart failure in rats via inhibiting apoptosis and blocking beta3adrenoreceptor/endothelial nitric oxide synthase pathway. Mol Med Rep. 2014;10(6):3199–3204.
- Amaral JD, Xavier JM, Steer CJ, et al. The role of p53 in apoptosis. Discov Med. 2010;9(45):145–152.
- Woods DB, Vousden KH. Regulation of p53 function. Exper Cell Res. 2001;264(1):56–66.
- Kwon S-K, Saindane M, Baek K-H. p53 stability is regulated by diverse deubiquitinating enzymes. Biochimica et Biophysica Acta (BBA)-Reviews on Cancer. 2017;1868(2):404–411.
- Li S, Jiang H, Gu X. Echinacoside suppresses dexamethasone-induced growth inhibition and apoptosis in osteoblastic MC3T3-E1 cells. Exp Ther Med. 2018;16(2):643–648.
- Yip K, Reed J. Bcl-2 family proteins and cancer. Oncogene. 2008;27(50):6398.