Abstract
Diabetic retinopathy (DR) is one of the most common diabetic complications and remains the leading cause of vision loss among adults. C1q/TNF-related protein 3 (CTRP3) is a member of CTRP family that has been found to be involved in the progression of diabetes mellitus and diabetic complications. However, the role of CTRP3 in DR has not been fully understood. In the present study, the results showed that CTRP3 expression was significantly decreased in DR patients compared with controls. In vitro investigations proved that overexpression of CTRP3 improved cell viability of ARPE-19 cells in response to high glucose (HG) stimulation. CTRP3 also attenuated HG-induced oxidative stress in ARPE-19 cells with decreased levels of reactive oxygen species (ROS) and malondialdehyde (MDA), and increased superoxide dismutase (SOD) activity. Apoptotic rate was significantly decreased in CTRP3 overexpressing ARPE-19 cells. Besides, bcl-2 expression was increased, while bax expression was decreased by CTRP3 overexpression. Moreover, overexpression of CTRP3 enhanced the activation of nuclear factor erythroid 2-related factor 2 (Nrf2)/hemeoxygenase-1 (HO-1) pathway in HG-stimulated ARPE-19 cells, and Nrf2 knockdown reversed CTRP3-mediated oxidative stress and apoptosis. These findings suggested that CTRP3 attenuated HG-stimulated oxidative stress and apoptosis in ARPE-19 cells, which were mediated by activation of Nrf2/HO-1 pathway.
Introduction
Diabetic retinopathy (DR) is one of the most common diabetic complications, representing the leading cause of vision loss among adults [Citation1]. Metabolic abnormalities induced by high glucose (HG) levels in diabetes can result in the excessive production of reactive oxygen species (ROS) [Citation2,Citation3]. The imbalance between ROS production and the antioxidant defence system activates several oxidative stress-related mechanisms, thereby promoting the pathogenesis of DR [Citation2,Citation4]. Therefore, alleviating the ROS production and oxidative damage will prevent and postpone the progression of DR.
The nuclear factor, erythroid 2 like 2- (NFE2L2-) related pathway (Nrf2), is a redox-sensitive factor that has the capacity to regulate the transcription of many antioxidant genes [Citation5,Citation6]. In recent years, Nrf2 signalling has attracted increasing attention since it has been found to act as a protective factor in response to oxidative stress and is associated with the scavenging of ROS. Moreover, Nrf2 was reported to play crucial roles in the development of DR and might be a new therapeutic strategy [Citation7,Citation8].
C1q/TNF-related protein 3 (CTRP3), a member of CTRP family, has been documented to possess a variety of roles in cellular processes. For instance, CTRP3 promotes cellular differentiation and proliferation, increases hepatic lipid oxidation and adipokine secretion, as well attenuates inflammation response [Citation9]. Dysregulation of CTRP3 has been found to be involved in the progression of diabetes mellitus. Elsaid et al. [Citation10] reported that reduction of CTRP3 concentrations is likely to bring a concomitant increase in risk of diabetes, indicating that CTRP3 may have an essential role in the pathophysiology of diabetes. In addition, CTRP3 serves as a novel diagnostic biomarker and independent predictor for DR [Citation11]. However, the molecular mechanism remains unclear. The aim of the present study was to investigate the effects of CTRP3 on oxidative stress and apoptosis in ARPE-19 cells cultured under HG condition.
Materials and methods
Patient samples collection
The study was approved by the Ethics Committee of the Shaanxi Provincial People’s Hospital (Xi’an, China). Informed consent was obtained from all participants involved in this study. Vitreous samples were collected from patients with DR (n = 13) and those with cataract (control group; n = 13). All the clinical samples were collected from May 2017 to March 2018 at Shaanxi Provincial People’s Hospital.
Cell culture and treatment
ARPE-19 cells were obtained from American Type Culture Collection (ATCC, Manassas, VA). Cells were grown in standard DMEM/F12 medium (Hyclone, Logan, UT) supplemented with 10% FBS (Hyclone, Logan, UT), 100 mg/ml streptomycin and 100 U/ml penicillin (Sigma-Aldrich, St. Louis, MO) at 37 °C in a humidified atmosphere with 5% CO2. Cells in control group were cultured in 5.5 mmol/l d-glucose, while cells in HG induction group were maintained in 25 mmol/l d-glucose.
Cell transfection
Recombinant plasmid expressing CTRP3 (pcDNA3.1-CTRP3) and control plasmid (pcDNA3.1) were obtained from GenePhama (Shanghai, China). ARPE-19 cells were transfected with pcDNA3.1-CTRP3 or pcDNA3.1 using Lipofectamine 2000 (Invitrogen, Carlsbad, CA). After 24 h, the CTRP3 levels were measured using quantitative real-time polymerase chain reaction (qRT-PCR) and western blot analysis.
The siRNA targeting Nrf2 (siRNA-Nrf2) and its negative control (siRNA-NC) were purchased from Santa Cruz Biotechnology (Santa Cruz, CA) and transfected into cells as per the manufacturer’s instructions.
qRT-PCR analysis
Total RNA was isolated from tissues and ARPE-19 cells using TRIzol reagent (Invitrogen, Carlsbad, CA) according to the manufacturer’s instructions. Then, the cDNA was produced from obtained total RNA using Primescript RT Reagent Kit (TaKaRa, Otsu, Japan). Subsequently, PCR was performed with a SYBR Green qPCR Master Mix kit (Applied Biosystems, Foster City, CA). The relative mRNA expression levels of CTRP3 were calculated using the 2–ΔΔCT method. Primers used in the study were listed as follows: CTRP3, forward: 5′-TTGCTCCTCGTAGCTTCT-3′, reverse: 5′-CGTATGTCTCTTGGGGTTACG-3′; β-actin, forward: 5′-GATCATTGCTCCTCCTGAGC-3′ and reverse: 5′-ACTCCTGCTTGCTGATCCAC-3′.
Cell viability assay
ARPE-19 cells were seeded in 96-well plate at the density of 1 × 104 cells/well, followed by an incubation for 24 h. To assess the cell viability, MTT was added to ARPE-19 cells and incubated for 4 h for the formation of formazan crystals. Then, DMSO was added to dissolve the crystals. The absorbance was measured at the wavelength of 490 nm using multi-well reader (SpectraMax Plus, Molecular Devices, Sunnyvale, CA).
Western blot analysis
ARPE-19 cells were lysed in RIPA buffer (150 mM NaCl, 50 mM Tris–HCl, 0.1% Triton-X 100, 0.1% SDS, 0.5% sodium deoxycholate) with protease and phosphatase inhibitor cocktail (Sigma-Aldrich, St. Louis, MO). The protein concentration was determined by BCA assay kit (Thermo Fisher Scientific, Waltham, MA). Totally, 40 μg proteins were separated in 10% SDS-PAGE, and then transferred to PVDF membrane (Thermo, Waltham, MA). Then, 5% nonfat milk in TBST was used for blocking the unspecific bands. The membranes were incubated overnight with primary antibodies against CTRP3 (1:1500; Abcam, Cambridge, MA), bax (1:2000; Santa Cruz Biotechnology, Santa Cruz, CA), bcl-2 (1:2500; Santa Cruz), nuclear Nrf2 (1:1000; Abcam, Cambridge, MA), hemeoxygenase-1 (HO-1; 1:1500; Abcam, Cambridge, MA) and β-actin (1:2500; Santa Cruz). After washing for three times to remove the unbound primary antibodies, the membranes were incubated with appropriate secondary antibody (1:1500; Abcam, Cambridge, MA) for 2 h. Bands were detected using enhanced chemiluminescence (ECL) prime reagent (GE Healthcare, Piscataway, NJ). Intensity of bands was assessed using Image-J software (National Institutes of Health, Bethesda, MD).
Detection of ROS, malondialdehyde and superoxide dismutase
The ROS production was measured with an ROS assay kit (Beyotime Biotechnology, Shanghai, China) using DCFH-DA as a fluorescence probe. The malondialdehyde (MDA) level and superoxide dismutase (SOD) activity were determined using commercial kits obtained from Beyotime according to the manufacturer’s protocol.
Cell apoptosis assay
Cell apoptosis of ARPE-19 cells was quantified by flow cytometry using an Annexin V-FITC/PI double staining assay kit (Sigma, St. Louis, MO). ARPE-19 cells were harvested and resuspended in binding buffer, after which Annexin V-FITC and PI were added to the cells and incubated for 15 min in the dark. The ARPE-19 cells were analysed by FACSCalibur flow cytometer (BD Biosciences, Franklin Lakes, NJ).
Statistical analysis
The data are expressed as mean ± SD and analysed using SPSS 22.0 software (IBM Corp., Armonk, NY). Comparisons among different groups were examined by Student’s t-test or one-way ANOVA. p Value less than .05 was considered statistically significant.
Results
CTRP3 expression was decreased in vitreous samples from DR patients
To evaluate the role of CTRP3 in DR, the expression levels of CTRP3 in vitreous samples collected from patients with DR or cataract were measured using qRT-PCR. As shown in , the CTRP3 expression was significantly lower in vitreous samples from DR patients than control group.
CTRP3 was lowly expressed in HG-induced ARPE-19 cells
To explore the function of CTRP3 in vitro, the ARPE-19 cells were induced by HG for 24 h, and the expression levels of CTRP3 were determined using qRT-PCR and western blot. As illustrated in , HG stimulation caused a significant decrease in the mRNA expression level of CTRP3 in ARPE-19 cells. Besides, the protein level of CTRP3 was also decreased after HG stimulation ().
Figure 2. CTRP3 expression in ARPE-19 cells under HG condition. ARPE-19 cells were cultured in normal glucose condition (5.5 mmol/l d-glucose) or HG condition (25 mmol/l d-glucose) for 24 h. The mRNA and protein levels of CTRP3 were determined using qRT-PCR (A) and western blot (B), respectively. *p < .05 vs. control.
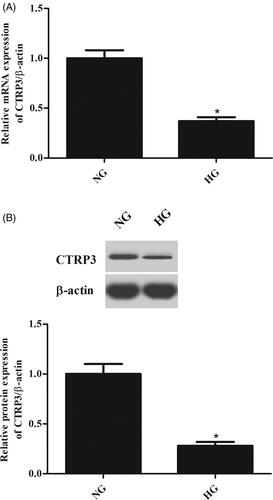
Overexpression of CTRP3 reversed the decreased cell viability in HG-induced ARPE-19 cells
Then, pcDNA3.1-CTRP3 or control plasmid pcDNA3.1 was transfected into ARPE-19 cells. The results showed that the CTRP3 expression was markedly increased after transfection with pcDNA3.1-CTRP3 (). MTT assay proved that overexpression of CTRP3 reversed the HG-caused reduction in cell viability of ARPE-19 cells ().
Figure 3. Effect of CTRP3 overexpression on cell viability in HG-induced ARPE-19 cells. ARPE-19 cells were transfected with pcDNA3.1-CTRP3 or control plasmid pcDNA3.1 for 24 h and then subjected to HG condition (25 mmol/l d-glucose) for 24 h. (A,B) The expression levels of CTRP3 were determined using qRT-PCR and western blot. *p < .05 vs. cells transfected with pcDNA3.1. (C) MTT assay was performed to evaluate cell viability. *p < .05 vs. control, #p < .05 vs. HG + pcDNA3.1 group.
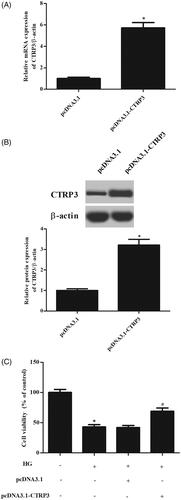
Overexpression of CTRP3 suppressed the HG-induced oxidative stress
To determine the effect of CTRP3 on HG-induced oxidative stress, the ROS generation was assessed. The results showed that HG significantly induced the ROS generation, while CTRP3 overexpression attenuated the increase in ROS generation (). Besides, the MDA production was significantly induced by HG stimulation; however, CTRP3 overexpression suppressed the production of MDA in HG-induced ARPE-19 cells (). In addition, the HG-induced inhibitory effect on SOD activity was mitigated by CTRP3 ().
Figure 4. Effect CTRP3 overexpression on HG-induced oxidative stress in ARPE-19 cells. ARPE-19 cells were transfected with pcDNA3.1-CTRP3 or control plasmid pcDNA3.1 for 24 h and then subjected to HG condition (25 mmol/l d-glucose) for 24 h. ROS generation (A), MDA production (B) and SOD activity (C) were measured to assess oxidative stress in ARPE-19 cells. *p < .05 vs. control, #p<.05 vs. HG + pcDNA3.1 group.
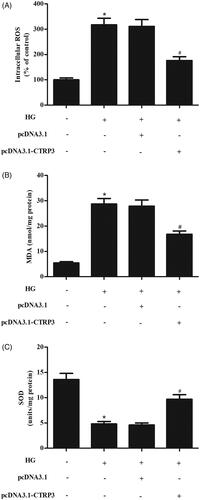
CTRP3-reduced cell apoptosis induced by HG in ARPE-19 cells
Next, flow cytometry was applied to examine apoptotic rate of ARPE-19 cells. As illustrated in , CTRP3 overexpression alleviated HG-stimulated cell apoptosis in ARPE-19 cells. Besides, western blot was used for the semi-quantitative assessment of bax and bcl-2. Overexpression of CTRP3 resulted in significant increase in bcl-2 expression and decrease in bax expression in HG-stimulated ARPE-19 cells ().
Figure 5. Effect of CTRP3 overexpression on HG-induced cell apoptosis in ARPE-19 cells. ARPE-19 cells were transfected with pcDNA3.1-CTRP3 or control plasmid pcDNA3.1 for 24 h and then subjected to HG condition (25 mmol/l d-glucose) for 24 h. (A) Flow cytometry was applied to examine apoptotic rate of ARPE-19 cells. (B) Western blot was used for the semi-quantitative assessment of the expressions of bax and bcl-2. *p < .05 vs. control, #p < .05 vs. HG + pcDNA3.1 group.
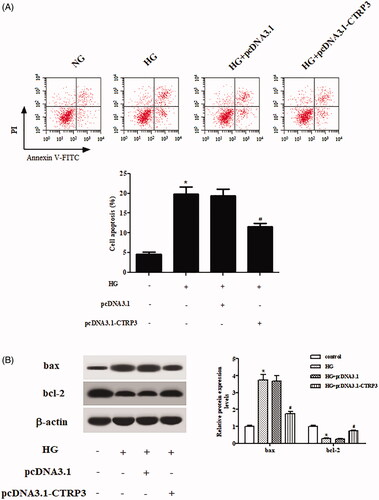
CTRP3 enhanced the activation of Nrf2/HO-1 pathway in HG-stimulated ARPE-19 cells
It is well known that Nrf2/HO-1 pathway plays crucial role in response to oxidative stress. The results revealed that HG stimulation caused slight increase in the expressions of nuclear Nrf2 and HO-1 in ARPE-19 cells; however, the induction was enhanced by CTRP3 overexpression ().
Figure 6. Effect of CTRP3 overexpression on Nrf2/HO-1 pathway in HG-stimulated ARPE-19 cells. (A) Western blot was used for the semi-quantitative assessment of the expressions of nuclear Nrf2 and HO-1. (B, C) The relative intensities of nuclear Nrf2 and HO-1 were determined. *p < .05 vs. HG + pcDNA3.1 group.
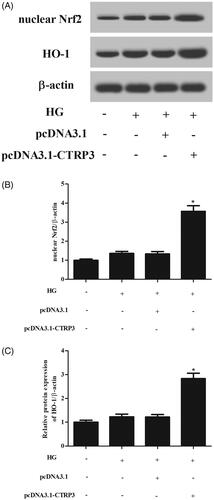
Nrf2 knockdown reversed CTRP3-mediated oxidative stress and apoptosis
To investigate whether CTRP3 inhibits HG-induced injury through activation of Nrf2, we examined the effect of siRNA-Nrf2 on CTRP3-mediated oxidative stress and apoptosis in HG-stimulated ARPE-19 cells. As shown in , knockdown of Nrf2 significantly reversed the inhibitory effects of CTRP3 overexpression on cell viability, ROS production and apoptosis ().
Figure 7. Nrf2 knockdown reversed CTRP3-mediated oxidative stress and apoptosis. ARPE-19 cells were co-transfected with pcDNA3.1-CTRP3 and siRNA-Nrf2 for 24 h and then subjected to HG condition (25 mmol/l d-glucose) for 24 h. (A) MTT assay was performed to evaluate cell viability. (B) ROS production was measured using DCFH-DA. (C) Cell apoptosis was quantified by flow cytometry using an Annexin V-FITC/PI double staining assay kit. *p < .05.
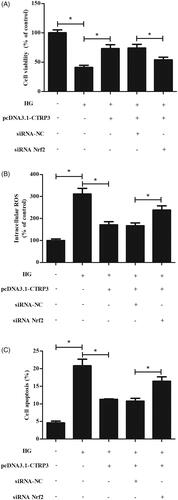
Discussion
Diabetes associated hyperglycaemia can cause dysfunction of mitochondrial electron transport chain, leading to a rise in generation of ROS, which is considered as a major causative factor involved in DR development [Citation3,Citation12]. Elevated level of ROS activates nuclear factor-κB (NF-κB) transcription factor, thereby up-regulates the production of nitric oxide (NO) and various pro-inflammatory cytokines, culminating in oxidative stress, cellular injury and apoptosis [Citation13]. In addition to the overproduction of ROS, hyperglycaemic conditions also contribute to the impairment of antioxidant enzyme activity, such as SOD, glutathione reductase, glutathione peroxidase and catalase [Citation13]. The decreased activities of antioxidant enzymes and excessive ROS production may lead to oxidative stress. In the current study, the ARPE-19 cells were cultured in HG condition to mimic hyperglycaemia in vitro. The results showed that HG caused decrease in cell viability and increase in cell apoptosis. Besides, HG stimulation resulted in significant increase in ROS production, MDA level as decrease in SOD activity in ARPE-19 cells. The results indicated that HG stimulation caused oxidative damage in ARPE-19 cells.
CTRP3 has been reported to possess diverse metabolic effects, especially on glucose metabolism. CTRP3 is implicated in diabetic complications, such as diabetic nephropathy and diabetic cardiomyopathy. Moradi et al. [Citation14] reported that serum levels of CTRP3 are significantly lower in patients with type 2 diabetes mellitus and diabetic nephropathy compared to controls. The decreased CTRP3 level is association with pathologic mechanism in these patients. Hu et al. [Citation15] demonstrated that CTRP3 overexpression suppresses cell proliferation, ROS production and extracellular matrix (ECM) accumulation in human glomerular mesangial cells (MCs) in response to HG stimulation, which suggest a possible role for CTRP3 in pathogenesis of diabetic nephropathy. Additionally, CTRP3 expression is significantly decreased in heart tissues of streptozotocin (STZ)-induced diabetic rats [Citation16]. In vitro investigations showed that knockdown of CTRP3 in cardiomyocytes results in increased oxidative injury, inflammation and apoptosis. Besides, overexpression of CTRP3 suppresses oxidative stress and inflammation, and attenuates myocyte death and improves cardiac function in diabetic rats, indicating CTRP3 is implicated in diabetic cardiomyopathy [Citation16]. In addition, CTRP3 level in serum is markedly decreased in patients with type 2 diabetes mellitus and serves as novel biomarker for DR severity. CTRP3 deficiency is associated with proliferative diabetic retinopathy (PDR) [Citation11]. However, the mechanism of the CTRP3 in DR has not been investigated. In the present study, we found that CTRP3 expression was markedly decreased in vitreous samples from DR patients and lowly expressed in HG-induced ARPE-19 cells. Overexpression of CTRP3 improved cell viability, attenuated oxidative stress and cell apoptosis in HG-induced ARPE-19 cells.
Nrf2 is a redox sensitive transcription factor that is kept in a latent state by interacting with Kelch-like ECH-associated protein 1 (Keap1) under basal conditions [Citation17]. In response to increased free radicals, Keap1 is oxidized or covalently modified, and then, Nrf2 dissociates from Keap1 [Citation18]. Subsequently, Nrf2 translocates into nucleus and binds to the antioxidant response element (ARE), which initiates the transcription of antioxidant genes, such as HO-1 and nicotinamide adenine dinucleotide/nicotinamide adenine dinucleotide phosphate (NADH/NADPH) quinine oxidoreductase 1 (NQO1) [Citation19]. These enzymes are important in detoxification and elimination of ROS and protect cells from oxidative damage [Citation19]. Previous studies have demonstrated that activation of Nrf2 signalling is a potential therapeutic approach to attenuate DR [Citation7,Citation20,Citation21]. Deliyanti et al. [Citation8] reported that a novel Nrf2 activator, dh404, elevates the antioxidant capacity of the retina with an increase in expressions of Nrf2, HO-1 and NQO1. Furthermore, dh404 treatment attenuates the diabetes-induced increase in oxidative stress. The results indicate that Nrf2 activation protects the retina against diabetes-induced damage and potentially prevents vision loss. In the present study, we found that CTRP3 overexpression enhanced the HG-stimulated activation of Nrf2/HO-1 pathway in ARPE-19 cells, and Nrf2 knockdown reversed CTRP3-mediated oxidative stress and apoptosis, which suggested that protective effects of CTRP3 on HG-stimulated oxidative damage might be mediated by activation of Nrf2/HO-1 pathway.
In conclusion, our findings suggested that CTRP3 attenuated HG-stimulated oxidative stress and apoptosis in ARPE-19 cells. The protective effects of CTRP3 were mediated by activation of Nrf2/HO-1 pathway. These findings provided evidence for understanding the role of CTRP3 in DR.
Disclosure statement
No potential conflict of interest was reported by the authors.
References
- Hendrick AM, Gibson MV, Kulshreshtha A. Diabetic retinopathy. Prim Care. 2015;42(3):451–464.
- Hammes HP. Diabetic retinopathy: hyperglycaemia, oxidative stress and beyond. Diabetologia. 2018;61(1):29–38.
- Li C, Miao X, Li F, et al. Oxidative stress-related mechanisms and antioxidant therapy in diabetic retinopathy. Oxid Med Cell Longev. 2017;2017:9702820.
- Lechner J, O'Leary OE, Stitt AW. The pathology associated with diabetic retinopathy. Vision Res. 2017;139:7–14.
- Bellezza I, Giambanco I, Minelli A, et al. Nrf2-keap1 signaling in oxidative and reductive stress. Biochim Biophys Acta Mol Cell Res. 2018;1865(5):721–733.
- Tonelli C, Chio IIC, Tuveson DA. Transcriptional regulation by nrf2. Antioxid Redox Signal. 2018;29(17):1727–1745.
- Kowluru RA, Mishra M. Epigenetic regulation of redox signaling in diabetic retinopathy: role of nrf2. Free Radic Biol Med. 2017;103:155–164.
- Deliyanti D, Alrashdi SF, Tan SM, et al. Nrf2 activation is a potential therapeutic approach to attenuate diabetic retinopathy. Invest Ophthalmol Vis Sci. 2018;59(2):815–825.
- Li Y, Wright GL, Peterson JM. C1q/TNF-related protein 3 (ctrp3) function and regulation. Compr Physiol. 2017;7(3):863–878.
- Elsaid HH, Elgohary MN, Elshabrawy AM. Complement c1q tumor necrosis factor-related protein 3 a novel adipokine, protect against diabetes mellitus in young adult Egyptians. Diabetes Metab Syndr. 2019;13(1):434–438.
- Yan Z, Zhao J, Gan L, et al. Ctrp3 is a novel biomarker for diabetic retinopathy and inhibits hghl-induced vcam-1 expression in an ampk-dependent manner. PLoS ONE. 2017;12(6):e0178253.
- Calderon GD, Juarez OH, Hernandez GE, et al. Oxidative stress and diabetic retinopathy: development and treatment. Eye (Lond). 2017;31(8):1122–1130.
- Dehdashtian E, Mehrzadi S, Yousefi B, et al. Diabetic retinopathy pathogenesis and the ameliorating effects of melatonin; involvement of autophagy, inflammation and oxidative stress. Life Sci. 2018;193:20–33.
- Moradi N, Fadaei R, Khamseh ME, et al. Serum levels of ctrp3 in diabetic nephropathy and its relationship with insulin resistance and kidney function. PLoS ONE. 2019;14(4):e0215617.
- Hu TY, Li LM, Pan YZ. Ctrp3 inhibits high glucose-induced human glomerular mesangial cell dysfunction. J Cell Biochem. 2019;120(4):5729–5736.
- Ma ZG, Yuan YP, Xu SC, et al. Ctrp3 attenuates cardiac dysfunction, inflammation, oxidative stress and cell death in diabetic cardiomyopathy in rats. Diabetologia. 2017;430(6):1–12.
- Deshmukh P, Unni S, Krishnappa G, et al. The keap1–nrf2 pathway: promising therapeutic target to counteract ros-mediated damage in cancers and neurodegenerative diseases. Biophys Rev. 2017;9(1):41–56.
- Taguchi K, Motohashi H, Yamamoto M. Molecular mechanisms of the keap1-nrf2 pathway in stress response and cancer evolution. Genes Cells. 2011;16(2):123–140.
- Sajadimajd S, Khazaei M. Oxidative stress and cancer: the role of nrf2. Curr Cancer Drug Targets. 2018;18(6):538–557.
- Nabavi SF, Barber AJ, Spagnuolo C, et al. Nrf2 as molecular target for polyphenols: a novel therapeutic strategy in diabetic retinopathy. Crit Rev Clin Lab Sci. 2016;53(5):293–312.
- Xu Z, Wei Y, Gong J, et al. Nrf2 plays a protective role in diabetic retinopathy in mice. Diabetologia. 2014;57(1):204–213.