Abstract
High atomic number Z, nanoparticles are able to enhance the photoelectric and Compton effects under X-Ray irradiation resulting the increase of radiation therapy efficacy. To achieve enhanced radiation therapy, Bi2S3 biocompatible particles coated with bovine serum albumin (BSA) (Bi2S3@BSA HNPs) were prepared through a BSA-mediated biomineralization procedure under green conditions. Then, to achieve improved chemo-radiation therapy against HT-29 cancer cells, curcumin (CUR) as natural anti-cancer therapy agent loaded on the Bi2S3@BSA (Bi2S3@BSA@CUR HNPs). Next, this synthesized nanodrug was evaluated for physical and chemical properties and in vitro cytotoxicity studies. Here, in vitro enhanced chemo-radiation combination therapy power was evaluated against HT-29 cell line under 2 Gy and 6 Gy X-ray irradiation doses. The Bi2S3@BSA HNPs without irradiation rarely affect cell viability which shown the non-toxicity of Bi2S3@BSA HNPs. The result of this study proved that Bi2S3@BSA@CUR HNPs can be used as both proficient vehicles for effective delivery of CUR and radiosensitizer in the treatment of cancer. In addition, the result of this study confirmed that the combination of high Z-element nanoradiosensitizer, Bi2S3@BSA HNPs, with a natural anti-cancer drug, CUR, enhanced therapeutic power against HT-29 cells.
Introduction
Despite many strategies developed for effective treatment of various cancers, global cancer statistics demonstrate that the incidence of cancer met a noticeable increase in through the world. For improving cancer therapy effectively with X-Ray irradiation, high dose irradiation is needed. Unlikely, high dose irradiation can damage normal tissues. High atomic number Z, nanoparticles can desirably enhance the photoelectric, Compton effects and radiation therapy efficacy [Citation1,Citation2]. In addition, by considering nanoparticles power, it is easy to concentrate the high atomic number particles in tumour tissues, and capable to enhance radiation therapy. To date among a lot of studies, colloidal hybrid nanoparticles (HNPs) have been considered as an significant family of multifunctional or enhanced functional nanoparticles [Citation3,Citation4]. HNPs include multiple components in a single nanosized particle. HNPs as novel important properties are attractively considered for biomedical applications. These types of biomaterials have shown abundant application in various biomedical fields, especially, as radiosensitizer [Citation5,Citation6]. Moreover, recent years bismuth compound nanomaterials have attracted huge attention in this field as well. Bismuth is a typical high-Z element with a high X-ray attenuation coefficient (5.74 cm2.kg−1 at 100 keV) [Citation6]. Obviously, the bismuth compounds have the X-ray computed tomography enhancement efficiency [Citation7]. In addition, the possibility of bismuth nanoparticles for in vivo X-ray computed tomography imaging and photothermal therapy/radiotherapy has been demonstrated very lately [Citation6].
Furthermore, bovine serum albumin (BSA) has received more attention than other biological carrier, especially in cancer treatment because of their unique properties [Citation8]. Rarely toxicity or immunogenicity; biodegradable; stable in circulation; qualifying a long half-life; does not require surfactants or polymeric materials for preparation; easy access to all tumour sites via the blood circulation; high chemical stability; and convenient to administer are likely properties to persuade researcher to use Albumin eagerly. In addition, the amino and carboxylic groups of the BSA structure can be used for surface modification as well.
Curcumin (CUR) is a biologically active component of the Indian spice which derived from the Zingiberaceae family known as turmeric (Curcumin longa). Turmeric is used to treat of various diseases in conventional medicine because of its anti-oxidative and anti-cancer properties [Citation9,Citation10]. CUR by down-regulating TNF-inducible NF-κB and AP-1 protein activity induces apoptosis in various carcinomas. Despite CUR have chemopreventive, cytotoxic and anti-metastatic properties, its severe drawback such as its instability reduces bioavailability and systemic uptake [Citation11]. Nowadays, some approaches have been successfully used to solve these drawbacks, such as encapsulating it in cyclodextrins and conjugating it with silver nanoparticles [Citation12,Citation13].
Herein, we report a BSA biomineralization facile method for achieving biocompatible BSA coated bismuth sulfide HNPs (Bi2S3@BSA HNPs). The resulting Bi2S3@BSA HNPs were characterized by ultra-small size and excellent colloidal stability. CUR, as a natural anti-cancer drug, was loaded on Bi2S3@BSA HNPs with physical encapsulation to form of Bi2S3@BSA@CUR HNPs. Then, anticancer effect of these nanoparticles was investigated, too. Furthermore, the enhanced efficiency of chemoradiation therapy confirms the radiosensitization and chemotherapeutic effect of Bi2S3@BSA@CUR HNPs.
Materials and methods
Materials
BSA, Bi (NO3)3, 3–(4, 5-dimethylthiazol-2-yl)-2, 5-diphenyl tetrazolium bromide (MTT) and CUR were achieved from Sigma Aldrich Chemicals, (St. Louis, MO, USA). All other solvents were purchased from Emertat Chimi Company (Tehran, Iran).
Preparation of bismuth sulfide hybrid nanoparticles (Bi2S3@BSA HNPs)
Bi2S3@BSA HNPs was prepared as previously process [Citation2,Citation14]. 50 mM Bi (NO3)3 in 1.0 ml of HNO3 solution (2 M) was slowly added into BSA solution (250 mg BSA in 8.0 ml deionized water) under vigorous stirring. After 30 min, NaOH (2 M) was used to adjust the solutions pH to 12, and the mixture was allowed to react at room temperature for overnight under vigorous stirring. The colour of the solution changed from colourless to dark black, and BSA stabilized Bi2S3 NPs (Bi2S3@BSA HNPs) were thus formed. The resulting Bi2S3@BSA HNPs were purified by the dialysis (12 kDa) of the solution against deionized water for 48 h to remove the excess precursors.
Loading of CUR on Bi2S3@BSA HNPs (Bi2S3@BSA@CUR HNPs)
Ten milligrams of Bi2S3@BSA HNPs was dispersed in 2.8 ml of distilled water under stirring at 400 rpm. CUR (2.5 mg) was dissolved in 200 µl of acetone and added dropwise to the above suspension. The mixture was shacked for 24 h under the dark condition at room temperature. Final formulation Bi2S3@BSA@CUR HNPs were purified by centrifugation at 18,000 rpm.
Characterization
The samples were characterized using XRD, FTIR, TEM, UV/Vis and DLS techniques.
The particle size and morphology of the Bi2S3@BSA@CUR HNPs were studied using TEM measurements. The sample was imaged by a transmission electron microscope (TEM; Cambridge 360–1990 Stereo Scan Instrument-EDS).
The ζ-potential and hydrodynamic size were measured using a nano/zetasizer (Malvern Instruments, Worcestershire, UK, model Nano ZS).
A powder X-ray diffractometer system (a Bruker AXS model D8 Advance diffractometer) was used for measurement of XRD pattern. Samples were exposed to Cu-Kα radiation at 1.542 Å. The measurements were done at 2θ from 10° to 80°.
FTIR spectra were measured using a FTIR spectrophotometer (Bruker, Tensor 27).
The absorption spectrum of the samples was documented using UV/Vis spectrophotometer (T80) to determine the components of BSA and CUR in the final formulation CUR-BSA.
In addition, stability of synthesized nanoparticles was examined using DLS and UV-vis techniques. The size of nanoparticles was monitored using DLS until 210 days after preparation. Also, the absorbance of synthesized nanoparticles was read at 261 nm (λ max of Bi2S3) at different times and compared with each other for determination of precipitation and further for determination of stability.
In vitro study
Drug release study
To evaluation the drug release behaviour, we incubated Bi2S3@BSA@CUR HNPs in PBS (pH 7.4 and 4.5) at 37 °C in a dialysis bag (molecular weight cutoff 12,000 Da) and next immersed this bag into 45 ml of PBS (2% tween). At selected time intervals, the exterior solution (2 ml) was withdrawn and analyzed spectrophotometrically at 428 nm and returned back to the medium to keep the volume of the drug release test constant.
Cell culture
The HT-29 cells were proliferated in RPMI1640 medium supplemented with 10% fetal bovine serum (FBS) and 100 mg/ml penicillin G and 100 mg/ml streptomycin (Gibco, Germany) at 37 °C in 5% CO2 atmosphere.
In vitro anti-tumour activity study
In vitro MTT cytotoxicity assay was performed to compare anti-tumour effects of Bi2S3@BSA HNPs, Bi2S3@BSA@CUR HNPs and free CUR against HT-29 cell line. Also, MTT cytotoxicity assay was further used for determination radiosensitization effect of Bi2S3 HNPs under X-Ray irradiation at 2 Gy and 6 Gy doses. The cells were seeded on a 96 well plate at a cell density of 3 × 104 cells/well. Every drug was tested in 5 wells. After incubating the cells in a logarithmic phase with medium containing free CUR, Bi2S3@BSA HNPs and Bi2S3@BSA@CUR HNPs at different concentrations for 5 h, the cells washed multiple times with PBS and further wells filled with fresh medium. Next, the cells were exposed with 2 Gy and 6 Gy doses X-Ray. After more incubation for overnight MTT dye (20 µl of 2.5 mg/ml) was added to each well. After incubation for additional 4 h, the percentage of cell viability was determined at 570 nm relative to non-treated and non- irradiated cells. In order to compare irradiated cells with non-irradiated cells, one of the plates does not irradiate with X-Ray.
Results and discussion
Synthesis of Bi2S3@BSA@CUR HNPs
The many available ways to the synthesis of Bi2S3 NPs require severe conditions or post-synthetic surface modification. For the biomedical applications, a facile aqueous synthesis under ambient conditions is required [Citation15,Citation16].
Recently, a novel BSA-mediated biomineralization method for achieving biocompatible Bi2S3 NPs was reported [Citation2,Citation14]. The synthesis process is highlighted by two steps: (i) suitable conditions for facilitating the coordination of Bi3+ cations with BSA and preventing the former from hydrolysis, (ii) pH-mediated formation of Bi2S3 HNPs upon a quick adjustment of the reaction pH up to 12. In the present synthetic method, BSA has two practical roles. The BSA not only worked as a stabilizer but also as a sulfur precursor for forming Bi2S3 HNPs with excellent colloidal stability. The BSA can be denatured to release numerous residues (e.g. 35 cysteine residues) under strong basic conditions [Citation17,Citation18], as an excellent sulfur source for forming metal sulfide NPs [Citation19,Citation20]. Moreover, Biodegradability, stability in blood circulation and a long half-life are some of advantages albumin. Next, the CUR molecules were loaded physically and led to the formation of final formulation (Bi2S3@BSA@CUR HNPs).
Characterization of Bi2S3@BSA@CUR HNPs
Optical properties of Bi2S3@BSA@CUR HNPs were examined with UV − Vis absorption spectrophotometer. As shown in the loading of CUR to the nanoparticle was examined using UV-Vis absorption. As shown in , the UV-Vis spectrum peak of the BSA and Bi2S3@BSA HNPs is around 266 nm and 264 nm, respectively. In the UV-Vis absorption spectrum of CUR, it can be seen that a characteristic absorption centred at 424 nm, which has been assigned to the π-π* transition [Citation21,Citation22]. displays the UV-Vis absorption spectrum of the Bi2S3@BSA@CUR HNPs, the peaks in the UV-Vis absorption spectrum around 255 nm and 430 nm corresponds to the BSA and CUR, respectively, successfully loading CUR to the surface of the Bi2S3@BSA HNPs giving rise to the enhanced absorption at 430 nm [Citation23].
Figure 1. (a) UV-vis Spectra of BSA, CUR, Bi2S3@BSA HNPs and Bi2S3@BSA@CUR HNPs; (b) Absorbance of Bi2S3@BSA HNPs and Bi2S3@BSA@CUR HNPs at 261 nm for monitoring the stability.
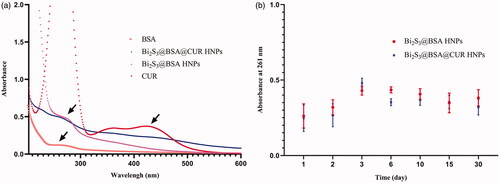
Many biomedical claims require nanoparticles to exhibit more colloidal stability. UV − vis spectrophotometer is one of the techniques used for the evaluation of colloidal stability of nanoparticles [Citation24]. For example, Frankamp et al. measured absorbance of magnetic nanoparticles at 450 nm in order to examine the stability [Citation25]. In this study, the stability of Bi2S3@BSA HNPs and Bi2S3@BSA@CUR HNPs were investigated with UV − vis absorption spectrophotometer. The absorption value of Bi2S3@BSA HNPs and Bi2S3@BSA@CUR HNPs were recorded at different times at 261 nm as shown in . As shown in the result, Bi2S3@BSA HNPs and Bi2S3@BSA@CUR HNPs are all very stable even after almost 1 month stored.
As shown in , we can be seen the unmodified Bi2S3@BSA HNPs exhibited a mean particle size around 60 nm with a PDI of 0.130. Also, the DLS result showed that Bi2S3@BSA@CUR HNPs exhibited a diameter of about 103 nm with a PDI of 0.202. The size of the Bi2S3@BSA@CUR HNPs was slightly larger than that before loading with drug. The stability of the particles is related closely to the surface charge. The same surface charge is favourable for particle stability because it could provide repulsion between the nanoparticles. The Bi2S3@BSA HNPs was negatively charged with a zeta potential of −32.61 mV, whereas the zeta potential of Bi2S3@BSA@CUR HNPs was −26.35 mV. Thus, it can be decided that the particles were negatively charged, which provided good physical stability for the as-prepared formulations [Citation26]. One of the other factors used for stability checking is size monitoring at different times. There, we used DLS technique for monitoring the size for 210 days after preparation. As shown in , the stability checking shows that there is no significant increase in size. These results confirmed the stability of synthesized nanoparticles.
Figure 2. (a) Hydrodynamic size of Bi2S3@BSA HNPs and Hydrodynamic size of Bi2S3@BSA@CUR HNPs; (b) Hydrodynamic size of Bi2S3@BSA HNPs and Bi2S3@BSA@CUR HNPs at different time intervals for monitoring the stability; (c) TEM image of Bi2S3@BSA@CUR HNPs.
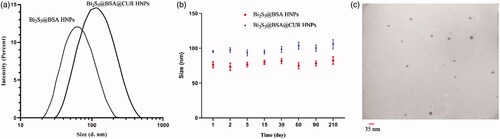
The TEM analysis was conducted to detect the morphology and size of and the results showed that Bi2S3@BSA@CUR HNPs existed in a spherical structure. The Bi2S3@BSA@CUR HNPs has an average diameter of approximately 14.59 ± 3.99 nm measured using TEM as shown in .
The FTIR technique was used for the successful synthesis of Bi2S3@BSA HNPs and Bi2S3@BSA@CUR HNPs. The comparison of BSA FTIR spectrum with Bi2S3@BSA HNPs FTIR spectrum and match of this result with previously reported results confirmed the successful synthesis of Bi2S3@BSA HNPs. Furthermore, after loading of CUR onto the Bi2S3@BSA HNPs the CUR characteristic bands appeared at Bi2S3@BSA@CUR HNPs FTIR spectrum ().
The crystal structure of Bi2S3@BSA HNPs was confirmed using XRD. As shown in , all reflection peaks of the Bi2S3@BSA HNPs is almost representative of the Bi2S3 structure (JCPDS NO. 43–1471) [Citation27,Citation28]. The BSA coating was confirmed by the appearance of the broad and moderately strong peak at the range of 20–29° which has been assigned to XRD pattern of the BSA [Citation29].
Drug loading and release study
The drug loading efficacy was also calculated according to the absorbance of CUR at 428 nm. The drug loading efficacy was 9.84 ± 1.27%.
Also, to reveal the distribution of CUR in cancer cells, the drug release from Bi2S3@BSA@CUR HNPs () were studied. Evidently, we can see that more CUR showed controlled release behaviour. This property is desirable in anticancer drug delivery, which can facilitate the drug release to suitable site.
Cytotoxicity assay
In this work, we used MTT assay to evaluate the anti-tumour effect of Bi2S3@BSA@CUR HNPs. Due to excellent properties of designed and prepared Bi2S3@BSA@CUR HNPs, these HNPs showed incomparable synergistic chemoradiation therapy effect. Because of the outstanding biocompatibility of Bi2S3@BSA HNPs, compared to control, the cell viability did not affect significantly in the presence of these HNPs.
As shown in , the cell viability decreased with the increase of CUR and Bi2S3@BSA@CUR HNPs concentrations. Particularly, CUR and Bi2S3@BSA@CUR HNPs exhibited dose-dependent cytotoxic effects.
Figure 5. Viability of HT-29 cells at different concentration with and without X-Ray irradiation at different doses. **indicates significant difference with p < .01, ***indicates significant difference with p < .001, ****indicates significant difference with p < .0001.
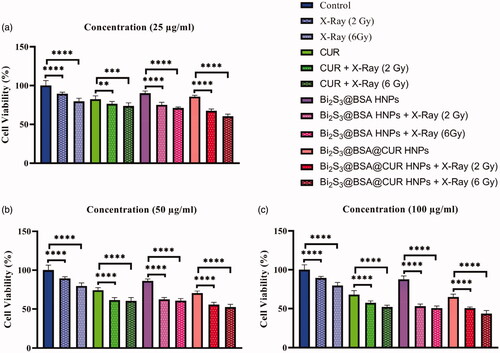
To evaluate radiosensitization power of CUR, Bi2S3@BSA HNPs and Bi2S3@BSA@CUR HNPs, the cells were treated with these particles and then were irradiated with different doses of X-Ray. Also, albeit the cell viability of HT-29 cells decreased under X-Ray irradiation, but this abate in the presence of CUR, Bi2S3@BSA HNPs was increased. Interestingly, we can see more and more decrease in cell viability in the presence of both X-Ray irradiation and Bi2S3@BSA@CUR HNPs. The groups that treated with CUR, Bi2S3@BSA HNPs and Bi2S3@BSA@CUR HNPs with the aid of X-Ray irradiation showed significantly inhabitation effect. The cell viability decreased with increasing radiation dose. These results confirmed the radiosensitization power of CUR, Bi2S3@BSA HNPs, and Bi2S3@BSA@CUR HNPs. The presence of both X-ray irradiation and HNPs showed higher anti-tumour effect which related to the radiosensitization power of high Z-elements [Citation2]. It is well known that the high Z-elements can generate ROS and further can be damage the DNA that leading cells to death [Citation30–32]. In all concentrations, the final formulation, Bi2S3@BSA@CUR HNPs, showed tiptop anticancer effect. These results are well matched with previously reported results that high Z-elements and CUR can be enhanced the therapeutic efficacy [Citation10,Citation14]. In addition, result of this study confirmed that the combination of high Z-element nanoradiosensitizer with natural anti-cancer drug, CUR, enhanced therapeutic power against HT-29 cells.
Conclusion
In conclusion, a biodegradable and stealthy nanostructure has been successfully developed through BSA-mediated biomineralization method for drug delivery in living cell. In the present synthetic method, BSA has two practical roles. The BSA not only worked as a stabilizer but also as a sulfur precursor for forming Bi2S3 HNPs with excellent colloidal stability. Also, Bi2S3@BSA@CUR HNPs exhibited attractive controlled release ability for CUR. This Bi2S3@BSA@CUR HNPs represents an essential approach for efficiently cancer therapy. In vitro cytotoxicity assay was performed to compare anti-tumour effects of Bi2S3@BSA@CUR HNPs and free CUR with and without of irradiation. The result of this study proved that Bi2S3@BSA@CUR with the aid of X-Ray irradiation increased therapeutic efficacy and can be used as a proficient vehicle for effective delivery of CUR in treatment of cancer. Compared to control group the presence of both X-ray irradiation and Bi2S3@BSA@CUR HNPs showed 40%, 47% and 57% increased of cytotoxicity towards HT-29 cells at 25 µg/ml, 50 µg/ml and 100 µg/ml concentration, respectively.
Acknowledgements
This work was supported by Zanjan University of Medical Sciences (Grant No. A-12-430-35).
Disclosure statement
No potential conflict of interest was reported by the authors.
References
- Wang H, Mu X, He H, et al. Cancer radiosensitizers. Trends in pharmacological sciences. 2018;39(1):24–48.
- Nosrati H, Charmi J, Salehiabar M, et al. Tumor targeted albumin coated bismuth sulfide nanoparticles (Bi2S3) as radiosensitizers and carriers of curcumin for enhanced chemoradiation therapy. ACS Biomater Sci Eng. 2019;5(9):4416–4424.
- Cheng K, Kothapalli S-R, Liu H, et al. Construction and validation of nano gold tripods for molecular imaging of living subjects. J Am Chem Soc. 2014;136(9):3560–3571.
- Seh ZW, Liu S, Zhang SY, et al. Anisotropic growth of titania onto various gold nanostructures: synthesis, theoretical understanding, and optimization for catalysis. Angew Chem. 2011;123(43):10322–10325.
- Cheng K, Sano M, Jenkins CH, et al. Synergistically enhancing the therapeutic effect of radiation therapy with radiation activatable and reactive oxygen species-releasing nanostructures. ACS Nano. 2018;12(5):4946–4958.
- Huang H-H, Chen J, Meng Y-Z, et al. Synthesis and characterization of Bi2S3 composite nanoparticles with high X-ray absorption. Mater Res Bull. 2013;48(10):3800–3804.
- Yang S, Li Z, Wang Y, et al. Multifunctional Bi@ PPy-PEG core–shell nanohybrids for dual-modal imaging and photothermal therapy. ACS Appl Mater Interfaces. 2018;10(2):1605–1615.
- Ding C, Xu Y, Zhao Y, et al. Fabrication of BSA@ AuNC-based nanostructures for cell fluoresce imaging and target drug delivery. ACS Appl Mater Interfaces. 2018;10(10):8947–8954.
- Esatbeyoglu T, Huebbe P, Ernst IM, et al. Curcumin—from molecule to biological function. Angew Chem Int Ed. 2012;51(22):5308–5332.
- Aggarwal BB, Harikumar KB. Potential therapeutic effects of curcumin, the anti-inflammatory agent, against neurodegenerative, cardiovascular, pulmonary, metabolic, autoimmune and neoplastic diseases. Int J Biochem Cell Biol. 2009;41(1):40–59.
- Gupta SC, Prasad S, Kim JH, et al. Multitargeting by curcumin as revealed by molecular interaction studies. Nat Prod Rep. 2011;28(12):1937–1955.
- Pochampally R, John V, Alb A, et al. Curcumin-loaded γ-cyclodextrin liposomal nanoparticles as delivery vehicles for osteosarcoma. Nanomed Cancer Pan Stanford. 2017;8:291–322.
- Yang XX, Li CM, Huang CZ. Curcumin modified silver nanoparticles for highly efficient inhibition of respiratory syncytial virus infection. Nanoscale. 2016;8(5):3040–3048.
- Wang Y, Wu Y, Liu Y, et al. BSA‐mediated synthesis of bismuth sulfide nanotheranostic agents for tumor multimodal imaging and thermoradiotherapy. Adv Funct Mater. 2016;26(29):5335–5344.
- Min Y, Caster JM, Eblan MJ, et al. Clinical translation of nanomedicine. Chem Rev. 2015;115(19):11147–11190.
- Cheng Z, Al Zaki A, Hui JZ, et al. Multifunctional nanoparticles: cost versus benefit of adding targeting and imaging capabilities. Science. 2012;338(6109):903–910.
- Wang Y, Yan X-P. Fabrication of vascular endothelial growth factor antibody bioconjugated ultrasmall near-infrared fluorescent Ag2S quantum dots for targeted cancer imaging in vivo. Chem Commun. 2013;49(32):3324–3326.
- Xie J, Zheng Y, Ying JY. Protein-directed synthesis of highly fluorescent gold nanoclusters. J Am Chem Soc. 2009;131(3):888–889.
- Chen W-T, Hsu Y-J. l-Cysteine-Assisted Growth of Core − Satellite ZnS − Au Nanoassemblies with High Photocatalytic Efficiency. Langmuir. 2010;26(8):5918–5925.
- Xiang J, Cao H, Wu Q, et al. L-cysteine-assisted synthesis and optical properties of Ag2S nanospheres. J Phys Chem C. 2008;112(10):3580–3584.
- Galasso V, Kovac B, Modelli A, et al. Spectroscopic and theoretical study of the electronic structure of curcumin and related fragment molecules. J Phys Chem A. 2008;112(11):2331–2338.
- van der Vlies AJ, Morisaki M, Neng HI, et al. Framboidal Nanoparticles Containing a Curcumin–Phenylboronic Acid Complex with Antiangiogenic and Anticancer Activities. Bioconjugate Chem. 2019;30(3):861–870.
- Singh PK, Wani K, Kaul-Ghanekar R, et al. From micron to nano-curcumin by sophorolipid co-processing: highly enhanced bioavailability, fluorescence, and anti-cancer efficacy. RSC Adv. 2014;4(104):60334–60341.
- Chen Z, Zhang Y, Xu K, et al. Stability of hydrophilic magnetic nanoparticles under biologically relevant conditions. J Nanosci Nanotech. 2008;8(12):6260–6265.
- Frankamp BL, Fischer NO, Hong R, et al. Surface modification using cubic silsesquioxane ligands. Facile synthesis of water-soluble metal oxide nanoparticles. Chem Mater. 2006;18(4):956–959.
- Ohshima H, Miyagishima A, Kurita T, et al. Freeze-dried nifedipine-lipid nanoparticles with long-term nano-dispersion stability after reconstitution. Int J Pharm. 2009;377(1–2):180–184.
- Cademartiri L, Malakooti R, O'Brien PG, et al. Large‐scale synthesis of ultrathin Bi2S3 necklace nanowires. Angew Chem Int Ed. 2008;47(20):3814–3817.
- Cademartiri L, Malakooti R, O'Brien PG, et al. Inside cover: large‐scale synthesis of ultrathin Bi2S3 necklace nanowires. Angew Chem Int Ed. 2008;47(20):3652–3652.
- Yang P, Liu Q, Liu J, et al. Bovine serum albumin-coated graphene oxide for effective adsorption of uranium (VI) from aqueous solutions. Ind Eng Chem Res. 2017;56(13):3588–3598.
- Yu N, Wang Z, Zhang J, et al. Thiol-capped Bi nanoparticles as stable and all-in-one type theranostic nanoagents for tumor imaging and thermoradiotherapy. Biomaterials. 2018;161:279–291.
- Song G, Liang C, Gong H, et al. Core–shell MnSe@ Bi2Se3 fabricated via a cation exchange method as novel nanotheranostics for multimodal imaging and synergistic thermoradiotherapy. Adv Mater. 2015;27(40):6110–6117.
- Huang P, Bao L, Zhang C, et al. Folic acid-conjugated silica-modified gold nanorods for X-ray/CT imaging-guided dual-mode radiation and photo-thermal therapy. Biomaterials. 2011;32(36):9796–9809.