Abstract
The aim of this study was to evaluate the effect of multilamellar vesicles (MLVs) of 1,2-dipalmitoyl-sn-glycero-3-phosphocholine (DPPC) in co-culture with in vitro-produced bovine embryos (IVPEs). The stability of five concentrations of MLVs (1.0, 1.25, 1.5, 1.75, and 2.0 mM) produced using ultrapure water or embryonic culture medium with 24 or 48 h of incubation at 38.5 °C with 5% CO2 was assessed. In addition, the toxicity of MLVs and their modulation of the lipid profile of the plasma membrane of IVPEs were evaluated after 48 h of co-culture. Both media allowed the production of MLVs. Incubation (24 and 48 h) did not impair the MLV structure but affected the average diameter. The rate of blastocyst production was not reduced, demonstrating the nontoxicity of the MLVs even at 2.0 mmol/L. The lipid profile of the embryos was different depending on the MLV concentration. In comparison with control embryos, embryos cultured with MLVs at 2.0 mmol/L had a higher relative abundance of six lipid ions (m/z 720.6, 754.9, 759.0, 779.1, 781.2, and 797.3). This study sheds light on a new culture system in which the MLV concentration could change the lipid profile of the embryonic cell membrane in a dose-dependent manner.
Introduction
Although the use of in vitro-produced (IVP) bovine embryos has grown exponentially in the last 20 years, the number of cryopreserved IVP embryos transferred to recipients remains proportionally lower than that of in vivo counterparts [Citation1,Citation2]. Embryonic development and quality are crucial factors associated with cryotolerance and the establishment of pregnancy. Cryopreservation exposes embryos to physical, chemical, and biological processes that cause osmotic, thermal, and mechanical stresses. Therefore, procedures such as cryopreservation can damage cell structures, especially the plasma membrane [Citation1,Citation3].
The plasma membrane may rupture and undergo severe changes in volume due to the entry and exit of cryoprotectants and intracellular water. Cell membranes that exhibit higher fluidity, permeability, and thermal phase properties suffer less damage during cryopreservation [Citation4,Citation5]. The main membrane lipids are glycerophospholipids (GPs), sphingolipids, and sterols (usually cholesterol in mammals). Phospholipids (PLs) may be present as different molecular species, such as phosphatidylcholine (PC), phosphatidylethanolamine (PE), phosphatidylinositol (PI), sphingomyelin (SM), and phosphatidylserine (PS) [Citation6–8]. Depending on the level of lipid saturation, the interaction between PLs and cholesterol molecules in the cell membrane can affect bilayer organisation and biophysical [Citation7] and physicochemical [Citation9–11] properties, including phase fluidity and permeability.
The properties of lipid vesicles have been examined to understand the interactions between cells and vesicles and apply them in clinical settings as a carrier for drug delivery [Citation12–15]. Furthermore, the formation techniques for multilamellar vesicles (MLVs) have been investigated, and these vesicles have been compared in terms of efficiency with unilamellar vesicles [Citation13,Citation15]. A better understanding of the interaction between MLVs and IVP bovine embryos can provide an alternative method for lipid transfer to the embryonic plasma membrane to modify its lipid composition and provide better conditions for cryopreservation. MLVs can be used as biocompatible carriers or transport vectors. When vesicles are produced using the same substances present in the cell membrane, the vesicles can be released into the extracellular environment to fuse with the cell membrane [Citation16,Citation17].
Although lipid vesicles are a potential tool for modulating IVP embryos, there is limited information on their use and effects. In the present study, we aimed to evaluate the use of MLVs in co-culture with bovine embryos in vitro, assess the potential embryotoxicity of the vesicles, and verify vesicle stability during embryonic culture (medium and environment of incubation). The effect of 1,2-dipalmitoyl-sn-glycero-3-phosphocholine (DPPC)-produced MLVs on the lipid profile of the plasma membrane of IVP bovine embryos was also evaluated by matrix-assisted laser desorption/ionisation mass spectrometry (MALDI-MS).
Material and methods
Unless otherwise indicated, all chemicals used in this study were purchased from Sigma-Aldrich® (St. Louis, MO, USA). For this study, review and approval by the ethics committee was not necessary since the use of bovine ovaries from a commercial slaughterhouse in Brazil does not require prior approval from any committee. The samples were collected from a slaughterhouse (Assiscarnes Distribuidora de Carnes Ltda., located at Água do Cervo, Bairro Cervinho, Assis, São Paulo state, Brazil) which is privately owned, so we have obtained previously the appropriate permission from the owner for sample collection.
Experiment I: preparation, size, and stability of MLVs
The MLVs were prepared by the lipid hydration method [Citation18,Citation19]. Briefly, a DPPC chloroform solution (10−3 mol/L) was placed in a glass vial, followed by the chloroform evaporation under nitrogen flow. The MLVs were prepared by adding the appropriate aqueous solution to the DPPC film containing vial at 55 °C, stirred for 1 h before cooling down to room temperature. MLV stability was investigated by measuring the vesicle size with different media and time intervals. The film was resuspended in ultrapure water (Milli-Q, Direct-Q® 3UV) and SOFaaci medium (synthetic oviduct fluid) in the absence or presence of 2.5% foetal bovine serum (FBS; v/v), 5 mg/mL bovine serum albumin (BSA), and 13 mmol/L pyruvate. For each resuspension medium, three different lipid concentrations were used: 1.0, 1.5, or 2.0 mmol/L. In addition, the MLVs were evaluated immediately after production (refrigerated at 4 °C) and after incubation at 38.5 °C in a controlled gas atmosphere (5% O2, 5% CO2, and 90% N2) for 24 and 48 h.
Vesicle stability under in vitro culture (IVC) was evaluated by dynamic light scattering (DLS; Brookhaven Instruments Corporation, Holtsville, NY, USA) using a He-Ne laser (wavelength 633 nm) operating with a maximum adjustable power of 15 mW [Citation20]. The scattered light was detected by a photomultiplier at a scattering angle of θ = 90° measured using a goniometer. The samples were thermostated at 25 °C in a toluene bath placed at the goniometer centre. The hydrodynamic diameter (DH) was determined using the Stokes-Einstein equation [Citation20].
Experiment II: evaluation of the embryotoxicity and lipid profile of the membrane of embryos co-cultured with MLVs
Collection of cumulus-oocyte complexes (COCs)
Bovine ovaries, predominantly from Bos taurus indicus females and their crossbreeds, were obtained from a local commercial slaughterhouse and transported to the laboratory (30 min at maximum) in a thermal pool containing sterile saline solution (0.9% NaCl) at 37 °C. Follicles (3–8 mm in diameter) were aspirated with the aid of a 10 ml syringe attached to an 18 G hypodermic needle [Citation21]. The pellet was recovered after sedimentation, and COCs were recovered using a stereomicroscope. The COCs were classified as described by Seneda et al. [Citation22]. Only quality grade I and II COCs were selected.
In vitro maturation (IVM)
The selected and washed COCs were transferred to 4-well plates (Nunclon Delta 4-Well Treated IVF Dish; Nunc, Roskilde, Denmark) containing 500 µL of IVM medium (25–30 structures per well) without mineral oil and incubated for 22 h at 38.5 °C in an atmosphere of 5% O2, 5% CO2, and 90% N2 at maximum humidity.
The IVM medium was TCM-199 supplemented with 0.2 mmol/L pyruvate, 25 mmol/L sodium bicarbonate, 50 μg/mL amikacin, 0.5 μg/mL follicle-stimulating hormone (FSH; Folltropin-V; Bioniche Animal Health, Ontario, Canada), 100 IU/mL human chorionic gonadotropin (hCG; Vetecor; Hertape Calier, Juatuba, Minas Gerais, Brazil), and 10% FBS (Gibco BRL, Grand Island, NY, USA).
In vitro fertilisation (IVF)
Matured COCs were removed from the IVM medium, transferred to 90 µL of IVF medium composed of Tyrode’s albumin lactate pyruvate (TALP) containing 0.2 mmol/L pyruvate, 25 mmol/L sodium bicarbonate, 13 mmol/L of sodium lactate, 50 μg/mL amikacin, 6 mg/mL fatty acid-free BSA, 10 μg/mL heparin, 20 μmol/L penicillamine, 10 μmol/L hypotaurine, and 2 μmol/L epinephrine (TALP-IVF medium), and covered with silicone oil. For insemination, straws of cryopreserved semen from Nellore bulls (previously tested) were used. The straws were thawed at 37 °C for 30 s, and the contents were subjected to centrifugation using a Percoll discontinuous density gradient (45% and 90%; GE Healthcare, Uppsala, Sweden) at 2500 × g for 7 min at room temperature. The supernatant was discarded, and the pellet was resuspended in 500 μL of TALP medium and centrifuged again at 5000 × g for 5 min. Sperm cells (2 × 106 cells/mL) were added to a drop of the TALP-IVF medium. COCs and sperm cells were co-incubated for 18 h under the same conditions as in the maturation stage. The start of IVF was defined as day 0 (D0).
In vitro culture (IVC)
After 18 to 20 h of insemination, the presumptive zygotes were subjected to the denudation process to remove the surrounding cumulus cells. Repeated gentle pipetting of the presumptive zygotes created a vortex in the fertilisation medium, which resulted in the detachment of the cumulus cells.
For the cultivation of the presumptive zygotes, the medium used was changed to synthetic oviduct fluid (mSOF) supplemented with 0.2 mmol/L L-glutamine, 0.34 mmol/L sodium citrate, 2.8 mmol/L myo-inositol, 2% essential amino acids, 1% non-essential amino acids, 0.2 mmol/L pyruvate, 50 μg/mL amikacin, 5 mg/mL BSA fraction V, and 2.5% FBS.
The presumptive zygotes were transferred to 500 μL of the mSOF medium without mineral oil (25 to 30 structures per well) and cultured at 38.5 °C with 5% O2, 5% CO2, and 90% N2 at maximum humidity for up to 7 days. The cleavage rate was evaluated 72 h after insemination (D2). On the 5th day of cultivation (D5), feeding was performed, and the rate of morula production was evaluated. On the last day of culture (D7), the embryos were examined to verify the total blastocyst production rate and developmental kinetics. Blastocyst samples (only expanded and grade I [excellent or good quality] blastocysts according to the International Embryo Transfer Society (IETS) [Citation23]) were collected from the experimental groups.
Embryotoxicity evaluation
The embryos described above were cultured in the absence or presence of MLVs. MLVs were included during the 2nd feeding (D5) for ease of handling so that the embryos were not exposed to unnecessary temperature and O2 changes in other stages of the IVC. Furthermore, the stability of the MLVs was considered on the day of inclusion. If they were stable only for 24 h, the addition of MLVs on D5 and D6 would be necessary for their effect to last until D7. As the stability of the MLVs was verified for 24–48 h, they were added on D5, and the effect lasted until D7. The MLV-free control group (CG) was compared with the MLV-treated groups with the following MLV concentrations: 1.0 mmol/L (G1), 1.25 mmol/L (G2), 1.5 mmol/L (G3), 1.75 mmol/L (G4), and 2.0 mmol/L (G5).
Each experiment was performed with eight replicates. In each replicate, each group had between 40 and 80 COCs. The same batch of FBS was used in all experimental groups to avoid variations in the membrane lipid composition. Embryotoxicity was evaluated by comparing blastocyst production in the five treated groups (G1–G5) with that in the CG. The concentration that prevented the production of blastocysts was considered lethal and embryotoxic, which could reduce the formation of complete blastocysts [Citation24].
Analysis of the lipid profile of the plasma membrane by mass spectrometry
To determine the lipid profile of blastocysts co-cultured with MLVs, only the G1, G3, and G5 groups were used in addition to the group without MLVs (control). Blastocysts (D7; 9 BL/group) (only expanded and grade I blastocysts according to the IETS [excellent or good quality] [Citation23]) were collected for MALDI-MS analysis as described by Ferreira et al. [Citation25]. The blastocysts were randomly selected, washed three times with PBS + 0.1% PVA (to eliminate any lipids that might originate from the culture medium), transferred to microtubes containing 200 µL of methanol (grade ACS/HPLC; Burdick and Jackson, Muskegon, MI, USA), diluted in ultrapure water (Millipore, Bedford, MA, USA), and stored overnight at −20 °C.
For MALDI-MS analysis, each embryo was washed again with methanol and ultrapure water (1:1). Each embryo was placed in a given spot of the target plate under a stereomicroscope together with 1 μL of dihydroxybenzoic acid (1 mol/L) diluted in methanol. The samples were dried at room temperature until crystallisation was complete and examined under a stereomicroscope to confirm their presence and location.
MALDI-MS data were acquired in positive ion and reflectron modes using an Autoflex III MALDI time-of-flight mass spectrometer (Bruker Daltonics, Bremen, Germany) equipped with smart beam laser technology. The acquisition range for the MS data was m/z 700–1200; consecutive laser shots were averaged at a frequency of 200 until the region of interest was observed and disappeared as the sample was consumed. Argon was used as the collision gas, and the data were processed using flexAnalysis software (version 3.0; Bruker Daltonics). The most intense ions (distinct from possible technical noise arising from the exclusion of isotopic peaks) were considered for each spectrum and used as a starting point to determine m/z values that corresponded to the lipids of interest [Citation25,Citation26]. Lipid attribution of the significant ions was performed using the LIPID MAPS (http://www.lipidmaps.org) and LipidomeDB databases and was based on the results of previous studies [Citation27–31].
Statistical analysis
The normal distribution and homogeneity of variance of the data (preparation and stability of MLVs) were evaluated. Subsequently, ANOVA was performed using the statistical software JMP® version 5.0.1a (SAS Inst. Inc., Cary, NC, USA), followed by Tukey’s post-hoc test.
Embryotoxicity was also assessed using the same software (JMP®). A statistically significant decrease in the blastocyst production rate (treated groups vs. control group) was considered to indicate embryotoxicity. The data were tested for normal distribution and homogeneity of variance. If the data were normally distributed, one-way ANOVA was applied. If they were nonparametric, data transformation (log10) was applied. Each experiment was performed with eight replicates. Differences with p < .05 were considered significant. The data are presented as mean values and standard errors of the mean (SEM).
Lipid profiles were evaluated by MALDI-MS with multivariate and univariate statistical models. Data processing was performed using MetaboAnalyst 3.6. The data input consisted of a .csv file with a list of ion peaks (m/z) and intensities for each isolate analysed. The ions were realigned with 0.4 Da mass tolerance. Data filtering was performed based on the standard deviation. Normalisation among samples was performed by sum and autoscaling among the individual features. Statistical analysis was performed by partial least squares-discriminant analysis (PLS-DA). The variable importance in projection (VIP) values of PLS-DA were obtained using auto-scaled data, and for visualisation, the top 15 ions were selected. A boxplot was used to show the difference in the relative abundance of the six significant ions in the CG compared with the G5. Univariate analysis by t-test was performed to show featured ions according to the threshold (p < .05) and Fisher’s least significant difference.
Results
Preparation and stability of MLVs
DLS showed the presence of structures with a mean diameter consistent with that of MLVs in the embryonic culture medium. In addition, the incubation of the vesicles did not impair the MLV structure. However, the incubation time (24 and 48 h) affected the mean diameter of MLVs (Supplementary Table S1 and S2) in a nonlinear model (i.e. not predicted by the time of incubation or used medium). The mean DH values of the MLVs in ultrapure water and embryonic culture medium for three lipid concentrations and in three storage forms (without incubation and with 24 and 48 h of incubation) are shown in .
Table 1. Effect of different forms of storage on the average diameter of MLVs at different concentrations. Results are presented as the mean (in nm) ± SEM, as previously published [Citation32].
Embryotoxicity assessment of MLVs
Data on the kinetics of embryonic development in the presence and absence of MLVs during the culture of IVP bovine embryos are presented in . There was no difference in the kinetics of embryonic development between the treated groups with different concentrations of MLVs (G1, G2, G3, G4, and G5) and the CG (p > .05).
Table 2. Evaluation of the embryonic development rate (after 7 days of culture; D7) with or without MLVs added on the 5th day of culture (D5).
Embryonic lipid profile analysis by mass spectrometry
The results of mass spectroscopy () demonstrated that embryos treated with MLVs showed a change in the lipid profile of the plasma membrane compared with that of the non-treated embryos. The PLS-DA graphs in 2 D and 3 D () show the separation of MLV-free embryos (control group) from embryos exposed to different MLV concentrations (G1, G3, and G5). There was also a marked difference in the lipid profile between G1 and G5 () after the exclusion of the CG. In PLS-DA, a comparison of the CG with G5 demonstrated a complete separation ().
Figure 1. MALDI-MS spectra in the positive ion mode for blastocysts co-cultured with MLVs (G1, G3, and G5) or without MLVs (control; CG).
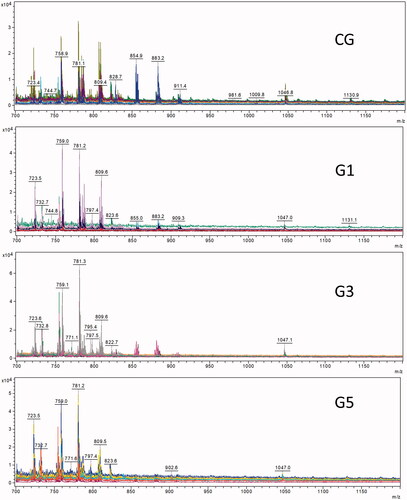
Figure 2. PLS-DA for embryos cultured without MLVs (CG) or with different concentrations of MLVs (G1, G3, and G5). (A) PLS-DA 2 D and (B) PLS-DA 3 D score plots.
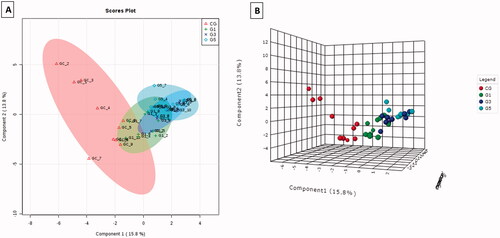
Figure 3. (A) PLS-DA 2 D for embryos cultured with MLVs (G1, G3, and G5). (B) PLS-DA 2 D for comparing embryos cultured without MLVs (CG) and with the highest concentration of MLVs (G5).
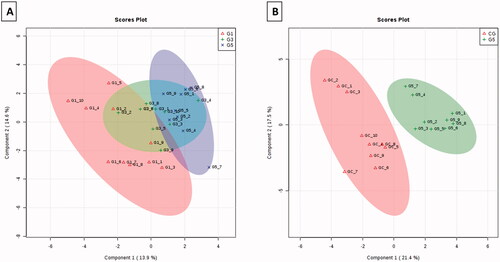
Following PLS-DA, six lipid ions were selected according to their statistical significance by comparing the extreme groups, i.e. CG and G5 (Supplementary Figure S1). The m/z values for the selected ions, lipid ions, and possible attributions are shown in .
Table 3. Tentative identification of discriminant ions in the CG and MLV-treated group (G5) based on the MALDI-MS profile.
As shown in , ions were assigned according to the smallest mass difference observed in the MALDI-MS spectra (), the calculated value in the LIPID MAPS database (http://www.lipidmaps.org), and the results of previous studies [Citation27–31]. shows the distribution of lipid ions according to the CG and G5 (). All lipid ions showed a greater relative abundance in G5 than in the CG.
Discussion
In our study, we attempted to modulate the lipid profile of the embryonic plasma membrane using MLVs. The effect of MLVs in co-culture with IVP embryos was investigated. The lipid composition of IVP embryos is relevant for physiological embryonic development and survival after cryopreservation processes [Citation34]. Molecules that alter or reduce the lipid content and profile of IVP embryos may contribute to an increase in embryonic survival rates and development similar to their physiological counterparts. Modification of the lipid profile involves the transport of molecules into cells via lipid vesicles [Citation14,Citation15].
Initially, based on DH measurements, the stability of MLVs in the Mili-Q and SOFaaci media was evaluated. The formation of MLVs was possible in both media, with the mean DH being influenced by the incubation time (24 or 48 h). MLVs were used in the present study because they can be formed simply by hydrating PL films with no extra mechanical effort (sonication or extrusion) to transform the MLVs into large or small unilamellar vesicles (LUVs or SUVs) [Citation18,Citation19]. The mean size (DH) of DPPC MLVs ranges from 0.5 to 10 µm depending on the temperature (below the melting temperature, Tm) and ionic strength. Overall, the temperature, added salt (ionic strength), vesicle size, and electrostatic potential affect the MLV structure and stability [Citation35,Citation36].
The largest MLVs were observed with 1.0 and 1.5 mmol/L DPPC (with or without incubation) in water compared with SOFaaci medium. After 24 h of incubation, MLVs with the largest diameter (approximately 1.5 µm) were obtained with 1.0 mmol/L DPPC in the culture medium. However, the in vivo use of MLVs has some limitations, such as stability in biological fluids, scale-up, and sterilisation (known as “Triple S”) [Citation37]. The embryonic culture medium contains salts and minerals (sodium chloride, calcium chloride, magnesium sulphate, and potassium), amino acids (L-glutamine and essential and non-essential amino acids), and hormones that help the embryo to develop until the blastocyst stage. In addition to these components, incubation conditions mimic the animal’s physiological state/environment such as temperature (38.5 °C) and humidity (maximum saturation in air). These factors can alter the lipid bilayer and phase transition. MLVs have been suggested to be more stable than unilamellar giant and large vesicles until they reach their Tm (in water, Tm = 41.4 °C for DPPC MLVs [Citation38]. Brooks and Moggridge [Citation39] reported that vesicles prepared at 60 °C were more stable than vesicles formed at lower temperatures. Our DLS results demonstrated that DPPC MLVs were relatively stable in different environments. Even in embryonic culture medium at a lower temperature (38.5 °C), MLV integrity was maintained up to 48 h of incubation.
Although amino acids, carbohydrates, proteins, lipids, and PLs are present in the oviduct fluid [Citation40] during embryonic development, the use of MLVs in co-culture with bovine embryos has never been assessed in terms of toxicity or lethality. Nevertheless, despite being an exogenous substance that is unusual for conventional IVC methods, they are not harmful to blastocyst production.
In the literature, there is no information on an in vitro embryonic culture method that can modify the lipid composition of the embryonic cell membrane using MLVs. More studies on the stability of MLVs in different culture environments are needed. MLVs have been useful as models for the study of cell membranes (PL bilayers) and as vectors for drug delivery to target cells [Citation41]. MLVs exhibit low toxicity when used in vivo [Citation42] or in vitro [Citation43]. Therefore, MLVs are a desirable vehicle for the delivery of cytoactive agents, and in some cases, they are more effective than co-incubation with a high concentration of the free drug [Citation44]. In addition, they have been used in cattle as a vehicle for the delivery of hormones such as FSH [Citation45].
MLVs have been the subject of several review articles; they are widely used in medicine [Citation46] as vectors for intracellular transport [Citation47] and cellular interactions [Citation48]. The vesicles have a high potential for transporting various bioactive molecules and could be used for therapeutic applications in humans and animals. However, research on animal reproduction (especially IVP embryos) and the use of lipid vesicles has not been carried out consistently.
Recent studies have indicated that embryonic quality and viability are directly associated with the intracellular lipid content. Excess intracellularly accumulated lipids can increase the sensitivity of embryos to oxidative stress and cryopreservation [Citation49]. In this study, MLVs did not affect embryo production; there was no change in embryo production in the MLV-treated groups compared with the group cultivated without MLVs. However, our results showed that the concentrations of PC, ether-linked PC (PCe), and GPs were higher in embryos cultured with MLVs at 2.0 mmol/L from day 5. Nevertheless, the changes in the embryonic lipid profile with the inclusion of MLVs did not prevent the embryos from undergoing cavitation (assessed based on the total blastocyst production rate) and hatching.
It is likely that the conditions of the co-cultivation of MLVs with embryos induced a dynamic change in the average diameter of the vesicles with the rupture of outer bilayers or the fusion of them (decreasing and increasing the diameter, respectively). MLVs with a decreased diameter (the inner core) could pass through the pores of the zona pellucida and reach the embryonic cells. In this theoretical scenario, lipid exchange could occur via direct action, i.e. by the fusion of MLVs and/or lipid bilayers with embryos [Citation50,Citation51] and/or indirect action, i.e. by inducing an endogenous change in lipid metabolism [Citation52,Citation53]. Vanroose et al. [Citation54] found a difference in the quantity and diameter of the outer pores of the zona pellucida according to the embryonic developmental stage. Although the blastocyst stage was not evaluated by Vanroose et al. [Citation54], the development of the morula (which was predicted at D5 of the IVC in our study) was analysed. At this stage (morula), the average outer pore diameter was 155 nm, and 19% of the pores were larger than 200 nm [Citation54].
The lipid content (composition and size of cytoplasmic droplets) and the morphology of the organelles present in the cells of IVP embryos are important markers for differentiation from in vivo-derived bovine embryos [Citation55–60]. Although lipids may impair in vitro production, they are extremely important in metabolic processes and are actively involved in early embryonic development [Citation28]. The plasma membrane plays a fundamental role in this scenario. More than a simple barrier, it is essential for the active functioning of cells, regulating the passage of molecules and maintaining physiologically relevant concentration gradients in addition to metabolic and biosynthetic activities [Citation61].
GPs, the main lipid constituents in mammalian cells, are important for maintaining membrane homeostasis and the physical properties of membranes, which are crucial for their functioning [Citation62]. In our study, the embryos cultured with the highest concentration of MLVs had the greatest relative abundances of GP (42:4) and GP (44:5) at m/z 779.1 and 797.3, respectively.
GPs and sphingolipids are involved in a wide range of cell signalling pathways and act as precursors for many biomolecules, such as lysophosphatidylcholines (LPCs), lysoPE (LPE), and eicosanoids [Citation63]. Recently, in a study of endogenous extracellular vesicles of bovine oviduct fluid, LPCs, PC, LPE, and SM were detected, strongly suggesting that these lipids may be an important in vivo source of PLs for embryonic cells [Citation64,Citation65]. In addition to GPs, PLs play a key role in determining the general characteristics of the embryo, including several physical properties such as the fluidity and permeability of the membrane [Citation10]. The PL composition includes several molecular species of PC, PE, PI, PCe, and ether-linked phosphatidylethanolamine (PEe), as well as the small species of PS or SM [Citation6]. In embryos cultured with MLVs, we observed higher relative concentrations of PCe (32:0), PC (32:1) or PC (34:4), PC (34:2), and PC (34:2) or PC (36:5) at m/z 720.6, 754.9, 759.0, and 781.2, respectively. Furthermore, a higher relative concentration of PC (34:2) was detected in in vivo embryos compared with IVP embryos, suggesting that it may be used as a biomarker for high quality embryos [Citation26,Citation30,Citation33].
A higher abundance of unsaturated PC might increase membrane fluidity in blastocysts and improve their resistance to possible cryopreservation methods [Citation66,Citation67]. Therefore, strategies to improve the cryotolerance of these embryos should not attempt to remove lipids but rather change their composition and content closer to those observed in in vivo embryos.
In conclusion, this study is the first to evaluate a culture system in which MLVs were co-cultured for 24 and 48 h with bovine embryos in vitro. The lack of a toxic effect on the embryos and the stability of the MLVs were observed in this system. The exposure of IVP embryos to MLVs for 48 h altered the lipid profile of the plasma membrane in a dose-dependent manner. Further in vitro and in vivo studies should be performed to assess embryonic cryotolerance and the establishment of pregnancy with this new system, including additional analysis for complete lipid identification.
Geolocation information
Assis, São Paulo, Brazil.
Author contributions
Me. Hugo de Rossi: Conceptualisation, Methodology, Formal analysis, Investigation, Writing – Original Draft; Me. Camila Bortoliero Costa: Formal analysis, Data Curation, Writing – Original Draft and, Review & Editing; Me. Luana Teixeira Rodrigues-Rossi: Methodology, Formal analysis, Investigation, Writing – Original Draft; Me. Giovana Barros Nunes: Methodology, Formal analysis, Investigation, Writing – Original Draft; Dóris Spinosa Chéles: Data Curation, Writing – Original Draft and, Review & Editing; Isabella Maran Pereira: Data Curation, Writing – Original Draft and, Review & Editing; Dr. Daniele F. O. Rocha: Methodology, Supervision, Visualisation, Writing – Review & Editing; Dr. Eloi da Silva Feitosa: Methodology, Supervision, Visualisation, Writing – Review & Editing; Dr. Ana Valéria Colnaghi Simionato: Methodology, Data Curation, Visualisation, Supervision, Writing -Original Draft and, Review & Editing; Dr. Gisele Zoccal Mingoti: Methodology, Supervision, Visualisation, Writing – Review & Editing; Dr. Pedro Henrique Benites Aoki: Methodology, Supervision, Visualisation, Writing – Review & Editing; Dr. Marcelo Fábio Gouveia Nogueira: Conceptualisation, Methodology, Supervision, Visualisation, Writing – Review & Editing.
Supplemental Material
Download MS Word (1.3 MB)Disclosure statement
The authors declare that there is no conflict of interest in this work.
Data availability statement
The authors confirm that the data that support the findings of this study are available from Marcelo Fábio Gouveia Nogueira, upon reasonable request.
Additional information
Funding
References
- Marsico TV, de Camargo J, Valente RS, et al. Embryo competence and cryosurvival: molecular and cellular features. Anim Reprod. 2019;16(3):423–439.
- Fernandes GO, de Faria OAC, Sifuentes DN, et al. Electrospray mass spectrometry analysis of blastocoel fluid as a potential tool for bovine embryo selection. J Assist Reprod Genet. 2021;38(8):2209–2217.
- Vajta G, Kuwayama M. Improving cryopreservation systems. Theriogenology. 2006;65(1):236–244.
- Seidel GE. Jr. Modifying oocytes and embryos to improve their cryopreservation. Theriogenology. 2006;65(1):228–235.
- Van Meer G, Voelker DR, Feigenson GW. Membrane lipids: where they are and how they behave. Nat Rev Mol Cell Biol. 2008;9(2):112–124.
- Bartz R, Li WH, Venables B, et al. Lipidomics reveals that adiposomes store ether lipids and mediate phospholipid traffic. J Lipid Res. 2007;48(4):837–847.
- Harayama T, Riezman H. Understanding the diversity of membrane lipid composition. Nat Rev Mol Cell Biol. 2018;19(5):281–296.
- Yamashita A, Hayashi Y, Nemoto-Sasaki Y, et al. Acyltransferases and transacylases that determine the fatty acid composition of glycerolipids and the metabolism of bioactive lipid mediators in mammalian cells and model organisms. Prog Lipid Res. 2014;53:18–81.
- Ohvo-Rekila H, Ramstedt B, Leppimaki P, et al. Cholesterol interactions with phospholipids in membranes. Prog Lipid Res. 2002;41(1):66–97.
- Edidin M. Lipids on the frontier: a century of cell-membrane bilayers. Nat Rev Mol Cell Biol. 2003;4(5):414–418.
- Marsico TV, Sales JNS, Ferreira CR, et al. Characteristic MALDI-MS lipid profiles of Gir, Holstein and crossbred (Gir x Holstein) oocytes recovered by ovum pick-up. Livest Sci. 2021;243:104380.
- Amatore C, Arbault S, Bonifas I, et al. Dynamics of full fusion during vesicular exocytotic events: release of adrenaline by chromaffin cells. ChemPhysChem. 2003;4(2):147–154.
- Morshed A, Karawdeniya BI, Bandara Y, et al. Mechanical characterization of vesicles and cells: a review. Electrophoresis. 2020;41(7-8):449–470.
- Zhang W, Böttger R, Qin Z, et al. Phospholipid-free small unilamellar vesicles for drug targeting to cells in the liver. Small. 2019;15(43):e1901782.
- Chacko IA, Ghate VM, Dsouza L, et al. Lipid vesicles: a versatile drug delivery platform for dermal and transdermal applications. Colloids Surf B Biointerfaces. 2020;195:111262.
- Pagano RE, Weinstein JN. Interactions of liposomes with mammalian cells. Annu Rev Biophys Bioeng. 1978;7:435–468.
- Boriachek K, Islam MN, Möller A, et al. Biological functions and current advances in isolation and detection strategies for exosome nanovesicles. Small. 2018;14(6):1702153.
- Seneda MM, Esper CR, Garcia JM, et al. Relationship between follicle size and ultrasound-guided transvaginal oocyte recovery. Anim Reprod Sci. 2001;67(1–2):37–43.
- Bó GA, Mapletoft RJ. Evaluation and classification of bovine embryos. Anim Reprod. 2013;10(3):344–348.
- Razza EM, Satrapa RA, Silva CF, et al. Lethal effect of high concentrations of parecoxib and flunixin meglumine on the in vitro culture of bovine embryos. Anim Reprod. 2012;9(2):80–85.
- Ferreira CR, Saraiva SA, Catharino RR, et al. Single embryo and oocyte lipid fingerprinting by mass spectrometry. J Lipid Res. 2010;51(5):1218–1227.
- Sudano MJ, Santos VG, Tata A, et al. Phosphatidylcholine and sphingomyelin profiles vary in Bos taurus indicus and Bos taurus taurus in vitro- and in vivo-produced blastocysts. Biol Reprod. 2012;87(6):130.
- Tata A, Sudano MJ, Santos VG, et al. Optimal single-embryo mass spectrometry fingerprinting. J Mass Spectrom. 2013;48(7):844–849.
- Leão BCS, Rocha-Frigoni NAS, Cabral EC, et al. Membrane lipid profile monitored by mass spectrometry detected differences between fresh and vitrified in vitro-produced bovine embryos. Zygote. 2015;23(5):732–741.
- Belaz KRA, Tata A, França MR, et al. Phospholipid profile and distribution in the receptive oviduct and uterus during early diestrus in cattle. Biol Reprod. 2016;95(6):127.
- Razza EM, Satrapa RA, Emanuelli IP, et al. Screening of biotechnical parameters for production of bovine inter-subspecies embryonic chimeras by the aggregation of tetraploid Bos indicus and diploid crossbred Bos taurus embryos. Reprod Biol. 2016;16(1):34–40.
- Lasic DD. The mechanism of vesicle formation. Biochem J. 1988;256(1):1–11.
- Stetefeld J, Mckenna SA, Patel TR. Dynamic light scattering: a practical guide and applications in biomedical sciences. Biophys Rev. 2016;8(4):409–427.
- Sudano MJ, Rascado TD, Tata A, et al. Lipidome signatures in early bovine embryo development. Theriogenology. 2016;86(2):472–484.e1.
- Ferré LB, Kjelland ME, Taiyeb AM, et al. Recent progress in bovine in vitro-derived embryo cryotolerance: impact of in vitro culture systems, advances in cryopreservation and future considerations. Reprod Domest Anim. 2020;55(6):659–676.
- Šturm L, Poklar Ulrih N. Basic methods for preparation of liposomes and studying their interactions with different compounds, with the emphasis on polyphenols. IJMS. 2021;22(12):6547.
- Botigelli RC, Razza EM, Pioltine EM, et al. Supplementing in vitro embryo production media by NPPC and sildenafil affect the cytoplasmic lipid content and gene expression of bovine cumulus-oocyte complexes and embryos. Reprod Biol. 2018;18(1):66–75.
- Lasic DD. Liposomes: from physics to applications. 1a ed. Amsterdam: Elsevier Science Publishers., chap.3, 63–90. 1993.
- Kreutzberger MA, Tejada E, Wang Y, et al. GUVs melt like LUVs: the large heat capacity of MLVs is not due to large size or small curvature. Biophys J. 2015;108(11):2619–2622.
- Brooks MS, Moggridge GD. The effect of additives and the stability of multilamellar vesicles in a commercial surfactant system. Chem Eng Res Des. 2006;84(2):139–146.
- Aguilar J, Reyley M. The uterine tubal fluid: secretion, composition and biological effects. Anim Reprod. 2005;2(2):91–105.
- Garrett FE, Goel S, Yasul J, et al. Liposomes fuse with sperm cells and induce activation by delivery of impermeant agents. Biochim Biophys Acta. 1999;1417(1):77–88.
- Hara T, Aramaki Y, Takada S, et al. Receptor-mediated transfer of pSV2CAT DNA to mouse liver cells using asialofetuin-labeled liposomes. Gene Ther. 1995;2(10):784–788.
- Boelaert JR. Mucormycosis (zygomycosis): is there news for the clinician? J Infect. 1994;28:1–6.
- Jones MN, Kaszuba M, Reboiras MD, et al. The targeting of phospholipid liposomes to bacteria. Biochim Biophys Acta. 1994;1196(1):57–64.
- Dees C, Stringfellow D, Schultz RD. Incorporation of a follicle stimulating hormone used for embryo transfer in cattle into multilamellar liposomes. Theriogenology. 1984;21(4):661–675.
- Banerjee R. Liposomes: applications in medicine. J Biomater Appl. 2001;16(1):3–21.
- Cullis PR, Fenske DB. Model membrane systems as drug delivery vehicles. Biol Skr Dan Vid Selsk. 1998;49:201–208.
- Monck JR, Fernandez JM. The fusion pore and mechanisms of biological membrane fusion. Curr Opin Cell Biol. 1996;8(4):524–533.
- Al Darwich A, Perreau C, Petit MH, et al. Effect of PUFA on embryo cryoresistence, gene expression and AMPKα phosphorylation in IVF-derived bovine embryos. Prostaglandins Other Lipid Mediat. 2010;93(1–2):30–36.
- Mondal Roy S, Sarkar M. Membrane fusion induced by small molecules and ions. J Lipids. 2011;2011:528784.
- Ragaliauskas T, Mickevicius M, Rakovska B, et al. Fast formation of low-defect-density tethered bilayers by fusion of multilamellar vesicles. Biochim Biophys Acta Biomembr. 2017;1859(5):669–678.
- Thompson JG. Defining the requirements for bovine embryo culture. Theriogenology. 1996;45(1):27–40.
- Freitas DS, Lopes GADG, Nascimento BR, et al. Conjugated linoleic acid as a potential bioactive molecule to modulates gamete and embryo cryotolerance. Ciênc. anim. bras. 2020;21:1–20.
- Vanroose G, Nauwynck H, Soom AV, et al. Structural aspects of the zona pellucida of in vitro-produced bovine embryos: a scanning electron and confocal laser scanning microscopic study. Biol Reprod. 2000;62(2):463–469.
- Leibo SP, Pollard JW, Martino A. Chilling and freezing sensitivity of reassembled in vitro-derived bovine embryos. Theriogenology. 1995;43(1):265.
- Abe H, Yamashita S, Satoh T, et al. Accumulation of cytoplasmic lipid droplets in bovine embryos and cryotolerance of embryos developed in different culture systems using serum-free or serum-containing media. Mol. Reprod. Dev. 2002;61(1):57–66.
- Camargo LSA, Boite MC, Wohlres-Viana S, et al. Osmotic challenge and expression of aquaporin 3 and Na/K ATPase genes in bovine embryos produced in vitro. Cryobiology. 2011;63(3):256–262.
- Paschoal DM, Sudano MJ, Schwarz KRL, et al. Cell apoptosis and lipid content of in vitro-produced, vitrified bovine embryos treated with forskolin. Theriogenology. 2017;87:108–114.
- López-Damián EP, Fiordelisio T, Lammoglia MA, et al. Characterization of lipid droplets in Bos indicus and Bos taurus embryos. Reprod. Fertil. Dev. 2013;25(1):226.
- López-Damián EP, Jiménez-Medina JÁ, Lammoglia MA, et al. Lipid droplets in clusters negatively affect Bos indicus embryos during cryopreservation. Anat Histol Embryol. 2018;47(5):435–443.
- Becker WM, Kleinsmith LJ, Hardin J, et al. The world of the cell. San Francisco: Pearson Benjamin Cummings. 2009.
- Kroon AIPM. Pieter JR, smet CHD. Checks and balances in membrane phospholipid class and acyl chain homeostasis, the yeast perspective. Prog Lipid Res. 2013;52(4):374–394.
- Eyster KM. The membrane and lipids as integral participants in signal transduction: lipid signal transduction for the non-lipid biochemist. Adv Physiol Educ. 2007;31(1):5–16.
- Banliat C, Dubuisson F, Corbin E, et al. Intraoviductal concentrations of steroid hormones during in vitro culture changed phospholipid profiles and cryotolerance of bovine embryos. Mol Reprod Dev. 2019;86(6):661–672.
- Banliat C, Bourhis DL, Bernardi O, et al. Oviduct fluid extracellular vesicles change the phospholipid composition of bovine embryos developed in vitro. IJMS. 2020;21(15):5326.
- Razza EM, Sudano MJ, Fontes PK, et al. Treatment with cyclic adenosine monophosphate modulators prior to in vitro maturation alters the lipid composition and transcript profile of bovine cumulus-oocyte complexes and blastocysts. Reprod Fertil Dev. 2018;30(10):1314–1328.
- Hochi S, Kimura K, Hanada A. Effect of linoleic acid-albumin in the culture medium on freezing sensitivity of in vitro-produced bovine morulae. Theriogenology. 1999;52(3):497–504.
- Morini MA, Sierra MB, Pedroni VI, et al. Influence of temperature, anions and size distribution on the zeta potential of DMPC, DPPC and DMPE lipid vesicles. Colloids Surf B Biointerfaces. 2015;131:54–58.
- Leão BCS, Rocha-Frigoni NAS, Cabral EC, et al. Improved embryonic cryosurvival observed after in vitro supplementation with conjugated linoleic acid is related to changes in the membrane lipid profile. Theriogenology. 2015;84(1):127–136.
- Armengol X, Estelrich J. Physical stability of different liposome compositions obtained by extrusion method. J. Microencapsulation. 1995;12(5):525–535.
- De Rossi H. DISSERTATION, “Evaluation of lipid composition in the cytoplasmic membrane of bovine embryos produced in vitro and co-cultured with multilamellar vesicles” São Paulo State University (UNESP); 2019. https://repositorio.unesp.br/handle/11449/183428. (183428).