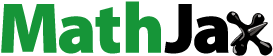
Abstract
This study investigated the impact of aggregate type on the volumechanges including drying shrinkage, autogenous shrinkage, and swelling for mortars made with different w/b ratios. The main properties including compressive and flexural strengths were also studied. For this purpose, three groups of mixes were prepared: two of them were prepared with 0.2 and 0.3 w/b ratios which were classified as high-strength mortars, and for comparison, a one group of normal-strength mortars was prepared with a 0.5 w/c ratio. Each group consisted of three mixes made with natural sand (NS), limestone aggregate (LA), or recycled concrete aggregate (RCA). Within each group, the LA mixes generally showed the lowest drying shrinkage and autogenous shrinkage regardless of the w/b ratio, while the greatest ones were observed for RCA mortars. The results also indicated that the group of mixes of the 0.2 w/b ratio gave the highest autogenous shrinkage.
PUBLIC INTEREST STATEMENT
The properties of aggregates influence the concrete performance because aggregates occupy 70−80% of the concrete volume. Aggregates have a major role in decreasing the long-term deformations of concrete. Regarding the mechanical properties, the effect of aggregate is more obvious in high-strength concrete than in normal-strength concrete. Therefore, in the present work, three types of fine aggregates were used to study the strength and volumechanges properties of normal- and high-strength mortars made with silica fume and different w/b ratios. These aggregates were natural sand, limestone, and recycled concrete aggregates. Indeed, two reasons behind the use of the recycled aggregates in concrete and mortar: first, to counterbalance the shortage of aggregates from natural sources, and second to decrease the negative effect of waste concrete on the environment.
1. Introduction
Aggregates occupy 70−80% of concrete volume. Consequently, it is expected that the characterizations of aggregates including strength, texture, shape, mineral composition, and maximum size can determine the concrete performance (Aydin, Yazici, Yardimci, & Yiğiter, Citation2010). Aggregates have an important role in reducing deformations caused by the moisture movements such as shrinkage. They make concrete more resistant to wear, which is resulted from different sources such as traffic and water (Alexander & Mindess, Citation2010). Aggregates have also a certain impact on the strength and stiffness of concrete that are essential for engineering applications.
So far, aggregates from natural source have been considered in the production of concrete. However, the need for lighter or heavier aggregates led to manufacture aggregates from industrial products (Gesoglu, Guneyisi, Ozturan, Oz, & Asaad, Citation2014, Citation2015; Kohno, Okamoto, Isikawa, Sibata, & Mori, Citation1999; Neville, Citation1995). Furthermore, the huge new constructions such as buildings, pavements, and bridges led to produce aggregates from waste concrete in order to counterbalance the shortage of natural aggregate as well as to decline the undesirable impact of the waste materials on the environment (Bui, Satomi, & Takahashi, Citation2017; Silva, Brito, & Dhir, Citation2015).
When loading concrete, cracks initiate and then propagate but thereafter they are arrested by coarse aggregates. The differences in strength between mortar and aggregate as well as the aggregate properties have an importance influence on the mechanism of crack growth (Neville, Citation1995). In ordinary strength concrete, the properties of coarse aggregate do not determine the concrete performance because their strength is significantly higher than the strength of mortar. On the other hand, in high-strength concrete, the coarse aggregate properties are important because of the improved mortar quality.
Concrete is typically classified as high-strength concrete when its compressive strength exceeds 42 MPa at 28 days (Shannag, Citation2000). Because of its better performance compared to conventional concrete, this type of concrete has been broadly used in several applications. The high level of strength can be attained by incorporating superplasticizer and pozzolanic materials such as SF, FA, and GGBFS. The industrial by-product materials help to decrease the cement content thus making the cement-based products less costly and environmental friendly.
The research work by Ozturan and Cecen (Citation1997) revealed that the concrete with ordinary strength gave comparable compressive strengths irrespective of aggregate type, while concrete of high strength gave different strengths such that the basalt aggregate gave the best strength and the gravel showed the lowest performance.
Tokyay (Citation1998) used crushed limestone, diabase, granite, and natural river to prepare high-strength concrete. Although limestone was the weakest among three crushed stone used, the highest strengths were obtained in limestone concrete. As mentioned by Wu, Chen, Yao, and Zhang (Citation2000), the characterizations of high-strength concrete may be enhanced by the use of aggregate of better strength, higher ductility, appropriate texture, and mineralogical properties. Zhou and Chen (Citation2017) pointed out that different coarse aggregate types strongly influenced the mechanical properties of the interfacial transition zone due to the significant different of their surface and shape. Compressive strength and flexural strength of recycled aggregate concrete are comparable and even higher than conventional concrete. Bui et al. (Citation2017) indicated that the recycled aggregates content influenced the compressive strength of concrete such that the greater the recycled aggregate content the poorer the strength.
As far as shrinkage behavior was concerned, Khatib (Citation2005) observed that the concrete prepared with waste concrete aggregate or crushed brick as fine aggregate showed more drying shrinkage when compared to that incorporating virgin aggregate. Andal, Shehata, and Zacarias (Citation2016) found that the mixtures containing coarse recycled concrete aggregate (RCA) (with a 20 mm maximum size) exhibited a 40–50% higher drying shrinkage than the corresponding specimens with natural aggregate. Furthermore, in a recent comprehensive review on the subject, Silva et al. (Citation2015) have pointed out that increasing recycled aggregate contents has resulted in an improvement in the drying shrinkage of concrete. However, there is inconsistent evidence about the magnitude of shrinkage increment such that it is varied between 10 and 80%. Furthermore, there is a disagreement of whether the fine or coarse RCA causes higher shrinkage.
Regarding the effects of aggregate type, the emphasis of the literature, as presented earlier, has been given to the properties of high-strength concretes rather than mortars. In the present study, therefore, the effects of three types of fine aggregates, namely natural sand (NS), limestone aggregate (LA), and waste concrete aggregates on the volumechanges and strength behavior of 30–70 MPa mortars were investigated. The investigated volumechanges were drying shrinkage, autogenous shrinkage, and expansion.
2. Experiments
2.1. Raw materials
2.1.1. Cement and silica fume
The cement used in the experiments was ordinary Portland cement with the chemical composition shown in Table . The test results showed that this cement fulfilled the requirement of Iraqi specification IQS No.5/1984 (Iraqi Organization of Standards, Citation1984). The relative density of the cement was 3.15 and its fineness was 340 m2/kg. The properties of silica fume (SF) used in this experimental work followed ASTM C1240 (Citation2015), as shown in Table .
Table 1. Chemical composition of cement and silica fume
2.1.2. Fine aggregates
Three types of fine aggregates were used: NS, LA, and RCA. The LA was brought from Karbla Cement Factory in the form of large balls. Then, it was crushed to minimize the size. The RCA was prepared by crushing concrete waste resulting from tested concrete cubes of a 30-MPa strength at the Babylon Construction Laboratory. The gradation of both LA and RCA was adjusted manually to be similar to that of NS. Figure illustrates the distribution of particle size of the aggregates and the limits of Iraqi specification IQS No.45/1984 (Iraqi Organization of Standards, Citation1984). The figure obviously shows that the used aggregates conformed this standard. The water absorption of NS, LA, and RCA were 0.68, 0.45, and 11.4%, respectively.
2.1.3. Superplasticizer
Superplasticizer in accordance with (ASTM C494/C494M, Citation2017) was used to get the required flowability at a given w/b ratio.
2.2. Mortar mix proportions
Nine mixes were designed and prepared in the present work, as shown in Table . The mixes were divided into three series. Series A consisted of the ordinary Portland cement mortars made with a 0.5 w/c ratio. Series B was produced with a 0.2 w/b ratio cementitious mortars. Series C was prepared with a 0.3 w/b ratio cementitious mortars. In each series, three types of fine aggregates were used, namely NS, LA, and RCA.
Table 2. Mix proportions of mortars (kg/m3)
2.3. Mixing procedure
The mixes of normal-strength mortar (Series A) were mixed via Hobart mixer following the procedure of ASTM C305 (Citation2012). The required water was supplied to the mixer first. Then the required amount of cement was added to water and mixed with low speed for 30 s. While the mixer was still rotating at low speed, total quantity of sand was added slowly during a period of 30 s. The mixer speed was changed to the medium speed (285 rpm) and mixed for 30 s .The mixer was stopped thereafter and the puddle was cleaned from any adhered mortar. Finally, the medium speed was also applied on the mixtures for 60 s.
The Series B and C of high-strength mixes were mixed by Hobart mixer as follows: over duration of 5 min, the aggregate and cementitious materials were mixed in the mixer at low speed. After the addition of water, the low speed was applied for additional 5 min. After adding an adequate amount of the chemical admixture, the low speed was also applied for further 5 min. Finally, a 285 rpm medium speed was applied over a period of 2 min.
2.4. Casting and curing procedure
In the laboratory, all molds were well cleaned first and their internal surfaces were lightly oiled to avoid the adhesion of hardened mortar to them. The fresh mortars were consolidated by an external vibrator to minimize the air voids and to get a well compacted mortar. The top surface of the samples was leveled. The specimens were demolded after about 1 day from casting time. Then, the samples for compressive strength and flexural strength were water cured until the testing time. All swelling samples were put in water over an entire curing period of 56 days during which the measurements were taken. Similar to Yang, Sato, and Kawai (Citation2005), the bars for autogenous shrinkage were covered by aluminum adhesive tape to avoid the loss of water and were cured in air at 23 ± 2°C and 50 ± 5% for 56 days during which different length change readings were recorded. The drying shrinkage bars were also air-cured at 23 ± 2°C and 50 ± 5% over a 56-day period. During this time period, the drying shrinkage measurements were taken frequently.
2.5. Testing fresh samples
The flowability of mortars was tested according to ASTM C230/C230M (Citation2015) and ASTM C1437 (Citation2014) by using a flow table. A 20 ± 2 cm flow was accomplished for all mortars by adding an appropriate dosage of superplasticizer.
2.6. Testing hardened mortars
2.6.1. Compressive strength
For compressive strength tests, 50 mm cubic samples were used and tested at 7 and 28 days according to ASTM C109/C109M (Citation2016a). The test was done by using a 2000 kN capacity machine.
2.6.2. Flexural strength
The flexural test was carried out on (50*50*300) mm prisms following a method outlined in ASTM C78/C78M (Citation2016). The specimens were also tested at two ages, namely 7 and 28 days.
2.6.3. volumechanges
The length change measurements were applied on ASTM C 157/C157M (Citation2017) bars of 25 × 25 × 285 mm in order to monitor the evolution of autogenous shrinkage, drying shrinkage, and swelling strains. The comparator length devise was adopted for the length measurements. For all mortar bars, the first measurement was taken directly after demolding and additional readings were taken at specified ages. The change in length was calculated by using the following equation:
where ∆Lx is the length change at an age of x, Lx is the comparator reading of sample at an age of x, (mm), Li is the first comparator reading of sample, and G is the gauge length = 250 mm.
3. Results and discussions
3.1. Compressive strength
The compressive strengths of the three groups of mortar mixes are given in Figure . As seen in the figure, the compressive strengths of mixes in Series A were comparable regardless of the testing age. In other words, the type of aggregate had no impact on the compressive strength of a 0.5 w/c ratio normal-strength mortars. At the age of 28 days, for example, the compressive strengths were 39.7, 39.4, and 39 MPa for NS, LA, and RCA mixes, respectively. However, the findings showed that the compressive strengths of both Series B and C mixes were affected by the type of fine aggregate. When the mixes of Series B were considered, which were made with SF and 0.2 w/b ratio, the greatest compressive strength was recorded for mortar mixes made with LA. The mixes made with NS generally gave the minimum compressive strength. In other words, the compressive strength of mortars made with LA or RCA was generally higher than that of mortars made with NS. As far as Series C mixes were concerned, which were produced with SF and 0.3 w/b ratio, the LA and RCA cementitious mortars also showed higher compressive strength co w/b ratio compared to NS mix such that the best strengths were observed for mortars made with LA. For Series B, the 28-day compressive strengths were 63.6, 68.6, and 61.7 MPa for NS, LA, and RCA mixes, respectively. The corresponding values were 55.8, 61, and 58.4 MPa, respectively, for the mixes of Series C.
Figure 2. Effect of aggregate type and w/b ratio on the 7- and 28-day compressive strength of the mortars.
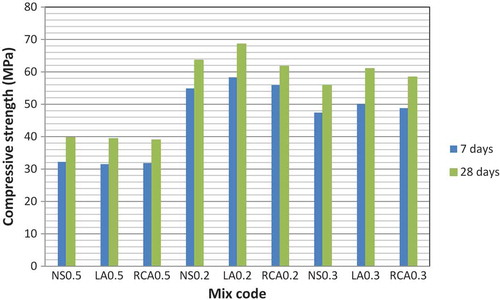
The concrete strength is affected by the properties of cement paste, aggregate, and the interface between aggregate and cement paste. In the case of normal-strength concretes, the characteristics of aggregate are not as vital as in the case of high-strength concretes. In high-strength concretes, various types of aggregates with different mineralogy, shape, strength, and texture may produce different concrete strengths. This could be related to the low w/c ratio (less than 0.4), which in turn leads to improve the paste strength and the bond at the interface considerably. Thus, the all strength of aggregate particle may be used in the case of high-strength concretes. Therefore, the compressive strength in Series B and C for LA and RCA mixes increased over that of NS mixes. The good performance of LA mixes was due to the angular shape of the crushed aggregates, which helped to enhance the bond between cement paste and aggregate. The present results of LA agreed well with the previous studies on concrete (Tokyay, Citation1998). With respect to RCA, there is inconsistent evidence in the literature regarding the effect of this type of aggregate on the strength of concrete and mortar (Ho et al., Citation2013; Topçu & Günçan, Citation1995; Xiao, Li, & Zhang, Citation2005). The relatively good strength performance of RCA in the present study may be related to three main reasons: (1) the water absorption of the RCA was high (11.4%) that lowered the effective w/c ratio thus improving the strength, (2) the shape of RCA was angular which improved bond between aggregate and cement paste, and (3) the strength of mother concrete of RCA was relatively high (30 MPa).
3.2. Flexural strength
The flexural strengths of all mixes are presented in Figure . As with the compressive strength, the modulus of rupture of normal-strength mortars (Series A) was not affected by the type of fine aggregate. At 28 days, the modulus of rupture was 5.12, 5.02, and 5.17 MPa, respectively, for the mixes made with NS, LA, and RCA, respectively. However, the fine aggregate type had an impact on the flexural strength of high-strength mortars (Series B and C). Among the three mixes of each group, the maximum modulus of rupture was recorded for mixes with LA while the lowest modulus of rupture was generally recorded for mixes with NS. In other words, replacing NS by either LA or RCA resulted in a higher modulus of rupture. At 28 days, the flexural strength increased by up to 8% and 4%, due to the use of LA and RCA, respectively, instead of NS.
Figure 3. Effect of aggregate type and w/b ratio on the 7- and 28-day flexural strength of the mortars.
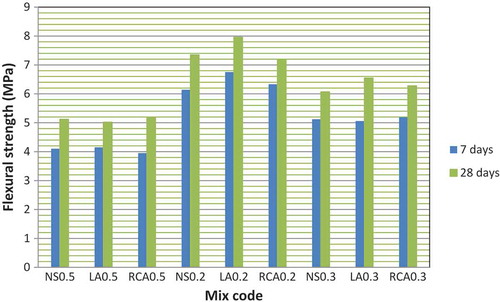
As expected, when the duration of curing increased from 7 to 28 days the modulus of rupture improved due to the progress of hydration process and consequent formation of C–S–H structure. The rule of w/c ratio was also valid herein such that the lower the w/c ratio the higher the modulus of rupture.
The tensile strength of the normal-strength cement-based composites is not much affected by the normal weight aggregate properties since the strength of aggregate is much higher than the strength of the matrix. Therefore, the failure accrues in the cement paste and/or at the interface. In high-strength cement-based composites, the matter is different. In such a case, the strength of cement paste and aggregate is comparable. This means that the aggregate plays more important rule in concretes that having high strength. In this study, the better performance of crushed limestone mixes as well as recycled aggregate mixes may be related to the improved bond between cementitious paste and crushed aggregate compared to natural aggregate mixes at a given w/b ratio.
3.3. Drying shrinkage
Drying shrinkage is the reduction in volumeof a hardened mortar or concrete which is subjected to drying as the result of the moisture loss. This shrinkage causes the tensile stress to increase, which may lead to cracking before the concrete is exposed to any type of loading. The magnitude of contraction is controlled by the characterizations of concrete as well as the relative humidity and temperature of the surrounded environment.
The development of drying shrinkage with age is shown in Figure , for Series A, B, and C, respectively. The all mixes contracted rapidly at early ages and the rate of drying shrinkage declined thereafter.
Figure 4. Drying shrinkage with age for mortars produced with (a) w/b = 0.5, (b) w/b = 0.2, and (c) w/b = 0.3.
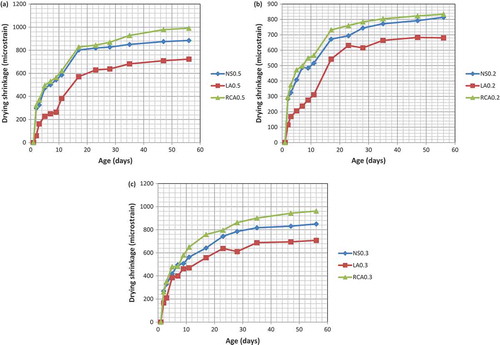
When Series A of mixes was considered, the bars with LA exhibited the lowest drying shrinkage; at 56 days, an 18% decrease in drying shrinkage was recorded as compared to the bars with NS. This observation is in agreement with the finding of others (Zhang, Zakaria, & Hama, Citation2013) showing that the incorporation of LA in mortars diminished the drying shrinkage by 22% as compared with NS and they attributed such behavior to the relatively lower water absorption of LA. On the other hand, the bars with RCA showed the highest drying shrinkage. This was because of the presence of deformable old adhered mortar which reduced the efficiency of restraint to the shrinkage (Silva et al., Citation2015). At 56 days, the drying shrinkage for NS, LA, and RCA bars was 884, 723, and 991 microstrain, respectively.
As far as Series B and C were concerned, the lowest drying shrinkage was also recorded for LA mixes. The percentage decrease in the 56- day drying shrinkage was about 16% due to the replacement of NS with LA. On the other hand, the bars prepared with RCA showed the maximum drying shrinkage.
Figure illustrates that, for a given aggregate, increasing w/b ratio resulted in an increase in the drying shrinkage because much water in the capillary pores evaporated. In the present study, the water contents for Series A (w/b = 0.5), Series B (w/b = 0.2), and Series C (w/b = 0.3) were 265, 173, and 260 kg/m3, respectively. Indeed, the drying shrinkage depends on many other factors such as contents of cement, aggregate, and paste as well as on the level of strength (Kayali, Haque, & Zhu, Citation1999). When the w/c ratio is kept constant, the drying shrinkage rises with increasing cement content as this leads to volumetric rise in the hydrated cement paste, which is responsible for contraction. In the present work, the w/c ratio was a predominant factor such that the high-strength mortars (Series B and C) showed a lower drying shrinkage compared to normal-strength mortars (Series A) though the later had a higher aggregate content and lower cement content.
3.4. Autogenous shrinkage
Autogenous shrinkage is the contraction of sealed concrete or mortar at a constant temperature and relative humidity. Chemical shrinkage caused by the cement hydration is considered as the essential developing force of this type of deformation. Though autogenous shrinkage grows uniformly through the concrete member, it may cause cracking, because it develops quickly and occurs when the cement paste is still young and has inadequate mechanical performance. Cracking may have negative impact on the strength and durability of concrete or mortar (Bentz & Jensen, Citation2004).
As shown in Figure , the autogenous shrinkage of the sealed bars developed quickly at the early ages and then gently at the later ages. The rapid development of autogenous shrinkage at the early ages was due the rapid decrease in the porosity and the pore size after setting. On the other hand, the gradual evolution of autogenous shrinkage at the later ages was related to the situation in which ettringite decomposes because of the depletion of gypsum (Yang et al., Citation2005).
Figure 6. Autogenous shrinkage with age for mortars prepared with (a) w/b = 0.5, (b) w/b = 0.2, and (c) w/b = 0.3.
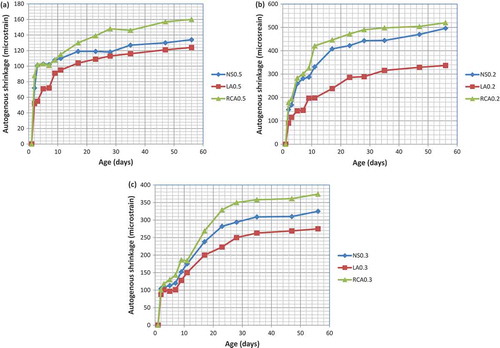
Figure illustrates the 56-autogenous shrinkage for the three groups of mortar mixtures. Obviously, Series B exhibited the highest autogenous shrinkage due to its lowest w/b ratio, 0.2 as compared to the other two groups. However, the lowest autogenous shrinkage was recorded for Series A due to its highest w/c ratio, 0.5.
Autogenous shrinkage or sometimes called self-desiccation is generated due to the additional reaction between cement and water after the growth of preliminary structure of the paste. For mortar or concrete with the w/c ratio of less than 0.4, the water in the capillary pores is inadequate to completely hydrate particles of cement; thus leading to increase the autogenous shrinkage. The self-desiccation may be further increased due to the inclusion of some supplementary cementitious materials like SF because the pozzolanic reactions speed up the consumption of water from capillary pores (Wu et al., Citation2000).
Like drying shrinkage, the mixes made with LA showed the lowest autogenous shrinkage while the RCA mixes exhibited the highest autogenous shrinkage. At 56 days, the LA mortars showed a 7–32% lower autogenous shrinkage as compared to NS mixes. However, the autogenous shrinkage of RCA mortars was higher than that of NS mortars by 5–19%.
3.5. Swelling
Figure shows the swelling of the bars with elapsed time. At the end of curing period in water, all specimens showed an increase in length except those of Series B which exhibited shrinkage in spite of immersing in water. Concrete or mortar made with normal w/c ratio and cured in moist conditions shows a net volumetric increase because of the absorption of water by the gel of cement. Moreover, the entrance of water reduces the surface tension of the cement gel, so more minor swelling occurs (Neville, Citation1995). However, because of the very low w/b ratio of the mixtures of Series B, the additional water from curing process was unable to enter the capillary pores, so contraction took place instead of expansion. Similar results were also reported by Miyazawa and Monteiro (Citation1996) for mortars prepared with river sand.
Figure 8. Swelling versus age for mortars produced with (a) w/b = 0.5, (b) w/b = 0.2, and (c) w/b = 0.3.
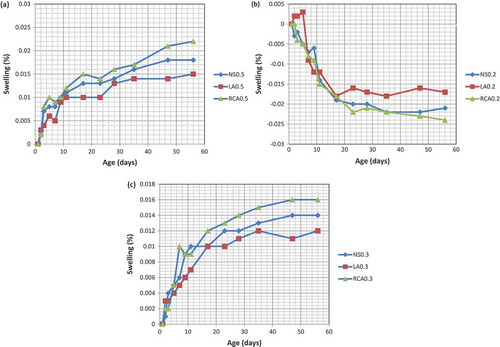
Figure also indicates that the LA bars generally showed the minimum swelling among the three mixes of Series A and C. However, the maximum expansion was observed for RCA. The expansion strains at 56 days for Series A were 0.018, 0.015, and 0.022% for mixes 0.5NS, 0.5LA, and 0.5RCA, respectively. The corresponding strains for Series C mixes were 0.014, 0.012, and 0.016%, respectively. The research work by other investigator showed that the concrete produced with RCA or crushed brick aggregates exhibited an increase in expansion strain when compared to reference concrete (Khatib, Citation2005).
4. Conclusions
Analyzing the experimental findings of the present study led to the following concluding remarks:
The mixes in Series A which were produced with different fine aggregates and the w/c ratio of 0.5 showed comparable compressive strengths regardless of the testing age.
The compressive strengths of Series B and C mixes that containing SF and were made with 0.2 and 0.3 w/b ratios, respectively, were affected by the type of fine aggregate such that the best compressive strengths were recorded for LA mixes followed by the RCA mixes and then NS mixes.
Like compressive strengths, the flexural strengths of normal-strength mortars (Series A) were not influenced by the type of fine aggregate, while the high-strength mortars (Series B and C) were affected such that the substitution of NS by LA or RCA generally increased the flexural strength of mortars.
Within each group, the LA mixes generally exhibited the minimum drying shrinkage while the RCA mixes showed the highest drying shrinkage.
When the type of fine aggregate was kept constant, increasing the w/b ratio led to improve contraction.
When the three mixes of each series were compared, the mixes made with LA gave the lowest autogenous shrinkage while the RCA mixes exhibited the highest autogenous shrinkage. At 56 days, the autogenous shrinkage diminished by 7–32% and increased by 5–19% due to the full substitution of NS by LA and RCA, respectively.
The highest autogenous shrinkage was recorded for Series B, due to its lowest w/b ratio as compared to the other two series. On the other hand, the lowest autogenous shrinkage was recorded for Series A due to its greatest w/c ratio.
It was found that Series A and C showed swelling under water while Series B showed contraction under water due to self-desiccation.
Additional information
Funding
Notes on contributors
Ali H. Nahhab
Ali H. Nahhab received his PhD in civil engineering. Currently, he is working as a lecturer at Civil Engineering Department in the University of Babylon. His research interests include high-strength concrete, high-performance concrete, self-compacting concrete, and fiber-reinforced concrete. He has published many papers on the ultra high-performance cementitious composites that made with gypsum-contaminated aggregates. His research interests also include the recycled concrete aggregates and their use in concrete and mortar along with by-product materials including silica fume and ground granulated plast furnace slag. Nowadays, he is working on the properties of the contaminated recycled aggregate concrete.
References
- Alexander, M. , & Mindess, S. (2010). Aggregates in concrete. In Modern concrete technology series (pp. 435).Taylor and Frances Group, London and New York.
- Andal, J. , Shehata, M. , & Zacarias, P. (2016). Properties of concrete containing recycled concrete aggregate of preserved quality. Construction and Building Materials , 125, 842–855. doi:10.1016/j.conbuildmat.2016.08.110
- ASTM C 1240 . (2015). Standard specification for silica fume used in cementitious mixtures . West Conshohocken, PA: American Society for Testing and Materials.
- ASTM C 157/C157M . (2017). Standard specification for length change of hardened hydraulic-cement mortar and concrete . West Conshohocken, PA: American Society for Testing and Materials.
- ASTM C109/C109M . (2016 a). Standard test method for compressive strength of hydraulic cement mortars (using 2-in. or [50-mm] cube specimens) . West Conshohocken, PA: American Society for Testing and Materials.
- ASTM C1437 . (2014). Standard test method for flow of hydraulic cement mortar . West Conshohocken, PA: American Society for Testing and Materials.
- ASTM C230/C230M . (2015). Standard specification for flow table for use in tests of hydraulic cementX . West Conshohocken, PA: American Society for Testing and Materials.
- ASTM C305 . (2012). Standard practice for mechanical mixing of hydraulic cement paste and mortars of plastic consistency . West Conshohocken, PA: American Society for Testing and Materials.
- ASTM C494/C494M . (2017). Standard specification for chemical admixtures for concrete . West Conshohocken, PA: American Society for Testing and Materials.
- ASTM C78/C78M . (2016). Standard test method for flexural strength of concrete (using simple beam with third-point loading) . West Conshohocken, PA: American Society for Testing and Materials.
- Aydin, S. , Yazici, H. , Yardimci, M. Y. , & Yiğiter, H. (2010). Effect of aggregate type on mechanical properties of reactive powder concrete. ACI Materials Journal , 107(5), 441–449.
- Bentz, D. P. , & Jensen, O. M. (2004). Mitigation strategies for autogenous shrinkage cracking. Cement and Concrete Composite , 26, 677–685. doi:10.1016/S0958-9465(03)00045-3
- Bui, N. , Satomi, T. , & Takahashi, H. (2017). Improvement of mechanical properties of recycled aggregate concrete basing on a new combination method between recycled aggregate and natural aggregate. Construction and Building Material , 148, 376–385. doi:10.1016/j.conbuildmat.2017.05.084
- Gesoglu, M. , Guneyisi, E. , Ozturan, T. , Oz, H. O. , & Asaad, D. S. (2014). Self-consolidating characteristics of concrete composites including rounded fine and coarse fly ash lightweight aggregates. Composite Part B , 60, 757–763.
- Gesoglu, M. , Guneyisi, E. , Ozturan, T. , Oz, H. O. , & Asaad, D. S. (2015). Shear thickening intensity of self-compacting concretes containing rounded lightweight aggregates. Construction and Building Materials , 79, 40–47. doi:10.1016/j.conbuildmat.2015.01.012
- Ho, N. Y. , Yang, P. K. L. , Lim, W. F. , Zayed, T. , Chew, K. C. , Low, G. L. , & Ting, S. K. (2013). Efficient utilization of recycled concrete aggregate in structural concrete. Journal of Materials in Civil Engineering , 25, 318–327. doi:10.1061/(ASCE)MT.1943-5533.0000587
- Iraqi Organization of Standards. (1984) . IQS 5/1984, for Portland Cement.
- Iraqi Organization of Standards (1984) . IQS 45/1984; for Aggregate.
- Kayali, O. , Haque, M. N. , & Zhu, B. (1999). Drying shrinkage of fibre-reinforced lightweight aggregate concrete containing fly ash. Cement and Concrete Research , 29, 1835–1840.
- Khatib, J. M. (2005). Properties of concrete incorporating fine recycled aggregate. Cement and Concrete Research , 35, 763–769. doi:10.1016/j.cemconres.2004.06.017
- Kohno, K. , Okamoto, T. , Isikawa, Y. , Sibata, T. , & Mori, H. (1999). Effects of artificial lightweight aggregate on autogenous shrinkage of concrete. Cement and Concrete Research , 29, 611–614. doi:10.1016/S0008-8846(98)00202-6
- Miyazawa, S. , & Monteiro, P. J. M. (1996). volumechange of high-strengthconcrete in moist conditions. Cement and Concrete Research , 26(4), 567–572. doi:10.1016/0008-8846(96)00033-6
- Neville, A. M. (1995). Properties of Concrete . New York: Longman.
- Ozturan, T. , & Cecen, C. (1997, January). Effect of coarse aggregate type on mechanical properties of concretes with different strengths. Cement and Concrete Research , 27(2), 165–170.
- Shannag, M. J. (2000 June). High strength concrete containing natural Pozzolan and SF. Cement and Concrete Composite , 23, 399–406. doi:10.1016/S0958-9465(00)00037-8
- Silva, R. V. , Brito, J. , & Dhir, R. K. (2015). Prediction of the shrinkage behavior of recycled aggregate concrete: A review. Construction and Building Materials , 77, 327–339. doi:10.1016/j.conbuildmat.2014.12.102
- Tokyay, M. (1998). Effect of aggregate type on mechanical properties of high strength concrete. Teknik Dergi , 9(2), 1627–1638.
- Topçu, I.B. , & Günçan, N. F. (1995). Using waste concrete as aggregate. Cement and Concrete Research , 25, 1385–1390. doi:10.1016/0008-8846(95)00131-U
- Wu, K. , Chen, B. , Yao, W. , & Zhang, D. (2000). Effect of coarse aggregate type on mechanical properties of high-performance concrete. Cement and Composite Research , 31, 1421–1425. doi:10.1016/S0008-8846(01)00588-9
- Xiao, J. Z. , Li, J. B. , & Zhang, C. (2005). Mechanical properties of recycled aggregate concrete under uniaxial loading. Cement and Concrete Research , 35, 1187–1194. doi:10.1016/j.cemconres.2004.09.020
- Yang, Y. , Sato, R. , & Kawai, K. (2005). Autogenous shrinkage of high-strength concrete containing SF under drying at early ages. Cement and Concrete Research , 35, 449–456. doi:10.1016/j.cemconres.2004.06.006
- Zhang,W. , Zakaria, M. , & Hama, Y. (2013). Influence of aggregate materials characteristics on the drying shrinkage properties of mortar and concrete. Construction and Building Materials , 49, 500–510. doi:10.1016/j.conbuildmat.2013.08.069
- Zhou, C. , & Chen, Z. (2017). Mechanical properties of recycled concrete made with different types of aggregate. Construction and Building Materials, 134, 497–506.