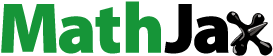
Abstract
In the current study, porous silicon (por-Si) samples were fabricated by electrochemical etching at different times (20 min, 40 min, 60 min). Scanning electron microscope (SEM) images of horizontal cross-sections of the samples showed the formation of pores. The etched samples’ porosity was determined by the gravimetric method and amounted to 59.5%, 72.7%, 83.3%, respectively. Optical characteristics such as Raman spectra and photoluminescence (PL) spectra were obtained. The current-voltage and capacitance-voltage characteristics were also measured to calculate the sensitivity of the samples. The study results show that sample, which is etched for 40 minutes has a maximum response value to ammonia (NH3) gas than others, and the sensitivity is 33.25. The results demonstrated that it is possible to develop a high sensitive sensor device based on por-Si for determining NH3 gas in concentrations below 0.1 ppm at room temperature.
PUBLIC INTEREST STATEMENT
Nowadays NH3 is widely used in chemical processes, agriculture, living environments, medical treatments and industrial applications. But, it is a toxic gas that is a severe irritant to the respiratory tract of human. Therefore, the study and fabrication of high-performance NH3 gas sensors to continuously monitor and detect the leakage of NH3 gas are essential to provide the safety of environments. The results of this work demonstrated that it is possible to develop a high sensitive NH3 sensor device based on porous silicon, which can determine NH3 gas in concentrations below 0.1 ppm at room temperature. There are also metal oxide-based sensors, however, usually these sensors have a high operating temperature, which can increase the power consumption, as well as have complex and expensive production technologies.
1. Introduction
Nowadays environmental pollution with noxious gases negatively affects both the human body and the ecosystem as a whole (Desai et al., Citation2005). Therefore, it is important to monitor the excess of harmful gases and determine how many molecules of poisonous gases are contained in the environment (Kayahan, Citation2018; Yamazoe, Citation2005). The detection of ammonia (NH3) gas is as of great interest in areas such as agriculture, industrial chemistry, environmental orientation, mechanical engineering, and medicine. Gaseous NH3 is very dangerous, flammable, poisonous gas that can damage the skin, eyes, and respiratory system even in small concentrations. At present, metal oxide semiconductors are widely used as NH3 and other gas sensors (ZnO, SnO2, TiO2, WO3, etc. (Berger et al., Citation2009; Jeevitha & Mangalaraj, Citation2019; Karunagaran et al., Citation2007; Matsubara et al., Citation2005; X. Wang et al., Citation2006)). Naderi et al. developed a high-stability sensor device capable of detecting NH3 gas up to a concentration of 212 ppb at 150°C from the V2O5/CUWO4 heterostructure, and also chemically explained sensitivity mechanism (Naderi et al., Citation2020). Bin Yang et al. showed that sensitivity and selectivity of the sensor device to NH3 gas increase at high temperatures using the ZnFe2O4 structure as sensitive material and adding gold to it (Yang et al., Citation2020). Weiwei Gong et al. found that the structure Bi0.95Ni0.05VO3.975 obtained by hydrothermal method is good at detecting NH3 gas at a high temperature of 500–600°C (Meng et al., Citation2018). Although these sensors have a high coefficient of sensitivity to NH3 gas, usually sensors based on metal oxides have a high operating temperature, which can increase the power consumption (Yuan et al., Citation2016), as well as have complex and expensive production technologies.
Due to the large surface area and the chemically active surface of the material, porous materials (Hoseinzadeh et al., Citation2019), namely, silicon (por-Si) can be used as a gas sensor (Baratto et al., Citation2000; H.J. Kim et al., Citation2010). Hee-Kyung Min et al. used por-Si for sensing the concentration of ethanol solutions (Min et al., Citation2000). S.M. Manakov et al. developed a structure based on por-Si for detecting of acetonitrile and chloroform (Manakov et al., Citation2019). In fact, por-Si can be obtained by electrochemical etching in an electrolyte with hydrofluoric (HF) acid by applying an electric current to the silicon wafer (Zhang & Braun, Citation2012). Such parameters as the current density of the electrochemical process, the etching time, and also the correctly selected concentration of liquids in the electrolyte make it possible to precisely control the structure of silicon and the thickness of the nanostructured layer (Ahmed & Mehaney, Citation2019; Zhanabaev et al., Citation2016).
The main purpose of this work is to determine the optimal parameters for preparing inexpensive sensor device based on por-Si, which is highly sensitive to ammonia gas and does not require complex technology of manufacturing, as well as to explain the reasons of changing the electrical characteristics of por-Si under the influence of the gas.
2. Experimental details
Por-Si layers were formed by electrochemical etching of <100 > p-type boron-doped silicon wafers (1015 cm−3) having resistivity of 10 Ω·cm with dimensions of 1 × 1 cm2. The electrochemical etching process was carried out in a special Teflon bath and was controlled by a special power source. The silicon wafers were immersed in HF acid for 5 seconds and then cleaned with ethanol. An aluminum plate, the silicon wafer, and the O-ring were collected horizontally in the Teflon bath in the form of a sandwich (Figure ). The electrolyte solution used was consisted of HF acid and ethanol in 1:1 volume ratio. Formation current was chosen as 5 mA and etching time was set to be 20, 40, and 60 minutes for samples 1, 2 and 3, respectively. To obtain electrical characteristics, two ohmic contacts of InGa alloy were deposited on their surface by thermal installation. The etched samples’ porosity was determined by the gravimetric method using the formula (1):
Figure 1. The electrochemical etching process (A) and obtaining the electrical characteristics of por-Si (B).
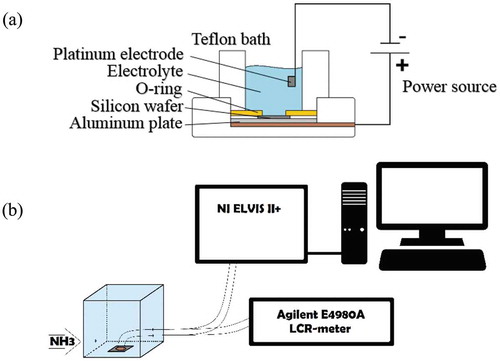
The current-voltage characteristics of por-Si samples were taken by the NI ELVIS II + module in the voltage range U = —2.5–2.5 V and the capacitance-voltage characteristics were measured using the Agilent E4980A LCR-meter at 1 MHz frequency. Photoluminescence (PL) and Raman spectra were studied on NT-MDT Solver Spectrum and excited by a laser with a wavelength of 473 nm. The morphology of por-Si was investigated from images of the scanning electron microscope (SEM) Quanta 200i 3D. To determine the sensitivity of por-Si to NH3, samples were placed in a cubic sealed box with a volume of 19x19x19 cm3 for 2 hours, and, finally, by measuring the current and capacity values at constant voltage and various gas concentrations, the sensitivity was calculated.
3. Results and discussion
SEM images were used to determine the size, the shape, and the thickness of the fabricated por-Si samples. Figure shows cross-section SEM images of por-Si samples, which were etched for 20, 40, and 60 minutes, respectively. According to the theory of pore formation, the size, the porosity, and the thickness of the pores increase with increasing the etching time (Choi et al., Citation2019). In Figure one can see that due to electrochemical etching in hydrofluoric acid, the pores were formed in the vertical direction and their thickness are 6.27 microns, 10.52 microns, 18.82 microns, respectively.
The porosity values of the samples were calculated by formula (1) and amounted to 59.5%, 72.7%, and 83.3% for three samples, respectively.
Figure shows the Raman and PL spectra of the samples. In Figure it can be noted that the Raman spectra of all three samples exhibit a peak intensity at 520 cm−1 band. This is the main combination scattering band that occurs from the action of monochromatic light with a crystal lattice. Raman spectra indicate that the size of the crystal structures of sample 3 is larger compared to the others (Kadlečíková et al., Citation2018). The small spectral hump for all samples at the band of 940 cm−1 is the result of the scattering of transverse optical phonons (Harraz et al., Citation2016).
As can be seen in Figure , sample 2 has the highest PL intensity, with a porosity value of 72.7 (625 nm). High PL intensity is associated with an increase in the total volume of nanocrystallites on the surface of por-Si (D.A. Kim et al., Citation2004).
The current-voltage and the capacitance-voltage characteristics of the samples were measured at various concentrations of NH3 gas at room temperature using the special sealed box. The desired concentration of gas in the sealed box is determined by the following formula (2):
where C (ppm) is the concentration of gas, ρ (g/cm3) is the density of NH3, T (К) is the absolute temperature, V1 (μl) is the volume of liquid (NH3), R (8,31 J/mol*К) is the universal gas constant, P (Pа) is the pressure inside the box, V2 (L) is the volume of the box, M (g/mol) is the molecular weight of the liquid (Naderi et al., Citation2020).
The sensitivity of por-Si samples at a constant voltage value (in our case U = 2 V) was calculated by the following formula (3):
where Iair and Igas are the currents of por-Si samples, measured in air and under the influence of NH3 gas, respectively (Liu et al., Citation2019). All samples showed that they can react to NH3 gas at room temperature even at concentrations below 0.1 ppm. As a result of the study, it was determined that the sensitivity of sample 2, obtained by etching for 40 minutes, the porosity of which is 72.7%, is higher compared to the other two samples, that is, the sensitivity was 33.25 for 0.1 ppm. In the reference (Zhang & Braun, Citation2012), the authors obtained similar results, and according to them, it is related to higher surface area and high enough initial resistance of the sample 2. The results are presented in Table .
Table 1. Sensitivity coefficients of por-Si samples at U = 2 V
A comparison of several reported NH3 sensors on the sensing performance towards ammonia is summarized in Table .
Table 2. Comparison of ammonia gas sensors
Figure shows the current-voltage and the capacitance-voltage characteristics of sample 2, measured after saturation of NH3 gas of various concentrations in the sealed box (0.03 ppm, 0.06 ppm, 0.1 ppm). With the increase in the concentration of NH3 gas, the resistance of the por-Si sample decreases, and the current increases. From Figure one can see that with increasıng the concentration of NH3 gas, the capacity increases. The capacity has a high value if there is no NH3 or presents in a low concentration at a low voltage, and increasing the voltage, it decreases accordingly, then at a certain voltage value, it acquires a stable value. This behavior of the capacity is explained by the absorption of NH3 molecules on the surface of por-Si, completely saturated with liquid vapors in the sealed box for 2 hours. As the concentration of NH3 gas increases, its saturation time also increases. Therefore, capacitance values are small at low voltage for 0.06 ppm and 0.1 ppm in the figure, and as the voltage increases, the values will increase. At a constant voltage of 2 V, capacitance values in the air and under the influence of the NH3 gas concentration of 0.1 ppm for all samples are shown in Table .
Figure 4. Current-voltage (A) and capacitance-voltage characteristics (B) at 1 MHz frequency of sample 2 at various gas concentrations.
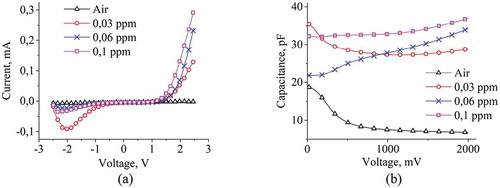
A common mechanism for determining the toxic gases of many gas sensors is a change in electrical conductivity or resistance as a result of the adsorption of gas particles (Ibraimov et al., Citation2016). When the gas sensor is in the air, oxygen molecules are adsorbed onto the surface of por-Si. Then, the adsorbed oxygen molecules are converted into oxygen ions by abstracting free electrons from the sensitive layer. The number of electrons decreases under the influence of electron abstraction in the outer region, and the number of holes increases, and a hole accumulation region is formed for sensitive p-type por-Si materials. Considering that the conductivity in semiconductors is formed by the action of holes, the resistance in the region of hole accumulation will be less than in other internal regions of the sensor.
When the por-Si-based sensor is exposed to NH3 gas particles, it is adsorbed on the surface of por-Si and the electrons located on the sensor surface are attracted to gas molecules. Accordingly, the resistance of the p-type por-Si sensor is reduced, because the number of holes is increased, forming a hole accumulation layer. In fact, as the thickness of the hole accumulation layer increases, more holes are present in the silicon conductivity and the resistance decreases. The higher the gas concentration, the more the number of attracted electrons increases, and the higher sensitivity is observed (Choi et al., Citation2019; Mirzaei et al., Citation2018).
The sensitivity, determined by the change in the capacitance and conductivity of the por-Si-based sensor, depends on several factors (Harraz et al., Citation2020; Schechter et al., Citation1995). The combination of the dipole moment and polarization can provide complete information about the overall structural interaction (Baker & Gole, Citation2016; Harraz et al., Citation2020). The dipole moment of the gas induces a local zone, thus displacing the energy levels of surface states, as a result of which the space charge zones of the por-Si structure modify. This leads to a change in the conductivity of por-Si (Harraz et al., Citation2020; Schechter et al., Citation1995).
4. Conclusion
As a summary, the high sensitive por-Si-based gas sensor device was successfully fabricated by electrochemical etching method and applied for NH3 gas detection at ambient temperature. Optical characteristics such as SEM, Raman spectra, PL spectra, and electrical characteristics were investigated. Research results revealed that a sample, which is etched for 40 min has the maximum sensitivity among all the three samples. The sensing mechanism of the por-Si-based gas sensor was proposed based on the resistance change as a result of the adsorption of gas particles. This study shows that it is possible to fabricate a high sensitive, inexpensive gas sensor device based on por-Si for determining NH3 gas in concentrations below 0.1 ppm at a room temperature. However, it is obvious that many studies are needed before application of por-Si as a NH3 sensor.
Additional information
Funding
Notes on contributors
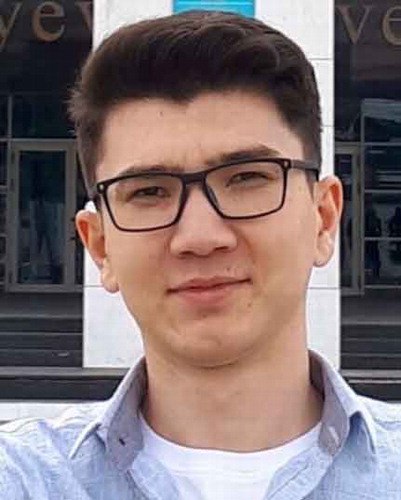
B.A. Khaniyev
Khaniyev Bakyt received the B.S. and M.S. degree in Radio engineering, Electronics, and Telecommunications from Al-Farabi Kazakh National University, Almaty, Kazakhstan, in 2016 and 2016, respectively. He is currently pursuing the Ph.D. degree in the Department of Physics and Technology at Al-Farabi Kazakh National University, Almaty, Kazakhstan. His current research interests include nanoelectronics and semiconductor gas sensors.
References
- Ahmed, A. M., & Mehaney, A. (2019). Ultra-high sensitive 1D porous silicon photonic crystal sensor based on the coupling of Tamm/Fano resonances in the mid-infrared region. Scientific Reports, 9(1), 1–8. https://doi.org/10.1038/s41598-019-43440-y
- Baker, C., & Gole, J. L. (2016). Detection of liquid organic solvents on metal oxide nanostructure decorated porous silicon interfaces. ACS Sensors, 1(3), 235–242. https://doi.org/10.1021/acssensors.5b00075
- Baratto, C., Comini, E., Faglia, G., Sberveglieri, G., Di Francia, G., De Filippo, F., La Ferrara, V., Quercia, L., & Lancellotti, L. (2000). Gas detection with a porous silicon based sensor. Sensors and Actuators. B, Chemical, 65(1–3), 257–259. https://doi.org/10.1016/S0925-4005(99)00297-X
- Berger, F., Sanchez, J. B., & Heintz, O. (2009). Detection of hydrogen fluoride using SnO2-based gas sensors: Understanding of the reactional mechanism. Sensors and Actuators. B, Chemical, 143(1), 152–157. https://doi.org/10.1016/j.snb.2009.08.054
- Chen, H. I., Hsiao, C. Y., Chen, W. C., Chang, C. H., Chou, T. C., Liu, I. P., Lin, K. W., & Liu, W. C. (2018). Characteristics of a Pt/NiO thin film-based ammonia gas sensor. Sensors and Actuators: B Chemical, 256, 962–967. https://doi.org/10.1016/j.snb.2017.10.032
- Choi, M. S., Na, H. G., Mirzaei, A., Bang, J. H., Oum, W., Han, S., Choi, S. W., Kim, M., Jin, C., Kim, S. S., & Kim, H. W. (2019). Room-temperature NO2 sensor based on electrochemically etched porous silicon. Journal of Alloys and Compounds, 811, 151975. https://doi.org/10.1016/j.jallcom.2019.151975
- Desai, R. R., Lakshminarayana, D., Patel, P. B., & Panchal, C. J. (2005). Indium sesquitelluride (In2Te3) thin film gas sensor for detection of carbon dioxide. Sensors and Actuators. B, Chemical, 107(2), 523–527. https://doi.org/10.1016/j.snb.2004.11.011
- Harraz, F. A., Ismail, A. A., Al-Sayari, S. A., Al-Hajry, A., & Al-Assiri, M. S. (2016). A highly sensitive and durable electrical sensor for liquid ethanol using thermally-oxidized mesoporous silicon. Superlattices and Microstructures, 100, 1064–1072. https://doi.org/10.1016/j.spmi.2016.10.074
- Harraz, F. A., Ismail, A. A., Faisal, M., Al-Sayari, S. A., Al-Hajry, A., & Al-Assiri, M. S. (2020). Organic analytes sensitivity in meso-porous silicon electrical sensor with front side and backside contacts. Arabian Journal of Chemistry, 13(1), 444–452. https://doi.org/10.1016/j.arabjc.2017.05.015
- Hoseinzadeh, S., Heyns, P. S., Chamkha, A. J., & Shirkani, A. (2019). Thermal analysis of porous fins enclosure with the comparison of analytical and numerical methods. Journal of Thermal Analysis and Calorimetry, 138(1), 727–735. https://doi.org/10.1007/s10973-019-08203-x
- Ibraimov, M. K., Sagidolda, Y., Rumyantsev, S. L., Zhanabaev, Z. Z., & Shur, M. S. (2016). Selective gas sensor using porous silicon. Sensor Letters, 14(6), 588–591. https://doi.org/10.1166/sl.2016.3657
- Jeevitha, G., & Mangalaraj, D. (2019). Ammonia sensing at ambient temperature using tungsten oxide (WO3) nanoparticles. Materials Today: Proceedings, 18, 1602–1609. https://doi.org/10.1016/j.matpr.2019.05.254
- Kadlečíková, M., Breza, J., Vančo, Ľ., Mikolášek, M., Hubeňák, M., Racko, J., & Greguš, J. (2018). Raman spectroscopy of porous silicon substrates. Optik, 174, 347–353. https://doi.org/10.1016/j.ijleo.2018.08.084
- Karunagaran, B., Uthirakumar, P., Chung, S. J., Velumani, S., & Suh, E. K. (2007). TiO2 thin film gas sensor for monitoring ammonia. Materials Characterization, 58(8–9), 680–684. https://doi.org/10.1016/j.matchar.2006.11.007
- Kayahan, E. (2018). Porous silicon based CO2 sensors with high sensitivity. Optik, 164, 271–276. https://doi.org/10.1016/j.ijleo.2018.03.024
- Kim, D. A., Shim, J. H., & Cho, N. H. (2004). PL and EL features of p-type porous silicon prepared by electrochemical anodic etching. Applied Surface Science, 234(1–4), 256–261. https://doi.org/10.1016/j.apsusc.2004.05.028
- Kim, H. J., Kim, Y. Y., & Lee, K. W. (2010). Multiparametric sensor based on DBR porous silicon for detection of ethanol gas. Current Applied Physics, 10(1), 181–183. https://doi.org/10.1016/j.cap.2009.04.020
- Lee, S.-K., Chang, D., & Kim, S. W. (2014). Gas sensors based on carbon nanoflake/tin oxide composites for ammonia detection. Journal of Hazardous Materials, 268, 110–114. https://doi.org/10.1016/j.jhazmat.2013.12.049
- Liu, I. P., Chang, C. H., Chou, T. C., & Lin, K. W. (2019). Ammonia sensing performance of a platinum nanoparticle-decorated tungsten trioxide gas sensor. Sensors and Actuators: B Chemical, 291, 148–154. https://doi.org/10.1016/j.snb.2019.04.046
- Manakov, S. M., Ibraimov, M. K., Sagidolda, Y., Zhumatova, S. A., & Darmenkulova, M. B. (2019). Detection of acetonitrile and chloroform using structures on the base of porous silicon. Eurasian Chemico-Technological Journal, 21(1), 89–93. https://doi.org/10.18321/ectj796
- Matsubara, I., Hosono, K., Murayama, N., Shin, W., & Izu, N. (2005). Organically hybridized SnO2 gas sensors. Sensors and Actuators. B, Chemical, 108(1–2), 143–147. https://doi.org/10.1016/j.snb.2004.10.051
- Meng, W., Wang, L., Li, Y., Dai, L., Zhu, J., Zhou, H., & He, Z. (2018). Enhanced sensing performance of mixed potential ammonia gas sensor based on Bi0.95Ni0.05VO3.975 by silver. Sensors and Actuators. B, Chemical, 259, 668–676. https://doi.org/10.1016/j.snb.2017.12.120
- Min, H.-K., Yang, H.-S., & Cho, S. M. (2000). Extremely sensitive optical sensing of ethanol using porous silicon. Sensors and Actuators. B, Chemical, 67(1–2), 199–202. https://doi.org/10.1016/S0925-4005(00)00399-3
- Mirzaei, A., Kang, S. Y., Choi, S. W., Kwon, Y. J., Choi, M. S., Bang, J. H., Kim, S. S., & Kim, H. W. (2018). Fabrication and gas sensing properties of vertically aligned Si nanowires. Applied Surface Science, 427, 215–226. https://doi.org/10.1016/j.apsusc.2017.08.182
- Naderi, H., Hajati, S., Ghaedi, M., Dashtian, K., & Sabzehmeidani, M. M. (2020). Sensitive, selective and rapid ammonia-sensing by gold nanoparticle-sensitized V2O5/CuWO4 heterojunctions for exhaled breath analysis. Applied Surface Science, 501, 144270. https://doi.org/10.1016/j.apsusc.2019.144270
- Schechter, I., Ben-Chorin, M., & Kux, A. (1995). Gas sensing properties of porous silicon. Analytical Chemistry, 67(20), 3727–3732. https://doi.org/10.1021/ac00116a018
- Wang, X., Zhang, J., & Zhu, Z. (2006). Ammonia sensing characteristics of ZnO nanowires studied by quartz crystal microbalance. Applied Surface Science, 252(6), 2404–2411. https://doi.org/10.1016/j.apsusc.2005.04.047
- Wang, Y., Jia, W., Strout, T., Schempf, A., Zhang, H., Li, B., Cui, J., & Lei, Y. (2009). Ammonia gas sensor using polypyrrole-coated TiO2/ZnO nanofibers. Electroanalysis: An International Journal Devoted to Fundamental and Practical Aspects of Electroanalysis, 21(12), 1432–1438. https://doi.org/10.1002/elan.200904584
- Yamazoe, N. (2005). Toward innovations of gas sensor technology. Sensors and Actuators. B, Chemical, 108(1–2), 2–14. https://doi.org/10.1016/j.snb.2004.12.075
- Yang, B., Wang, C., Xiao, R., Yu, H., Wang, J., Liu, H., Xia, F., & Xiao, J. (2020). High sensitivity and fast response sensor based on sputtering Au tuned ZnFe2O4-SE for low concentration NH3 detection. Materials Chemistry and Physics, 239, 122302. https://doi.org/10.1016/j.matchemphys.2019.122302
- Yuan, L., Hu, M., Wei, Y., & Ma, W. (2016). Enhanced NO2 sensing characteristics of Au modified porous silicon/thorn-sphere-like tungsten oxide composites. Applied Surface Science, 389, 824–834. https://doi.org/10.1016/j.apsusc.2016.07.068
- Zhanabaev, Z., Grevtseva, T., & Ibraimov, M. K. (2016). Morphology and electrical properties of silicon films with vertical nanowires. Journal of Computational and Theoretical Nanoscience, 13(1), 615–618. https://doi.org/10.1166/jctn.2016.4849
- Zhang, H., & Braun, P. V. (2012). Three-dimensional metal scaffold supported bicontinuous silicon battery anodes. Nano Letters, 12(6), 2778–2783. https://doi.org/10.1021/nl204551m