Abstract
Oil-in-water emulsions can be stabilized by proteins, and is widely used for delivery of hydrophobic bioactive compounds in food and pharmaceutical applications. But emulsions stabilized by proteins such as whey protein or soy protein isolates (SPI) may lose their stability under gastric conditions due to changes of temperature, pH and the presence of digestive enzymes. However, the additions of soy soluble polysaccharide (SSP) to protein-stabilized emulsions are reported to improve the stability against low pH, thermal treatment and under-simulated gastric conditions. This review emphasizes the importance of incorporating of SSP into SPI stabilized-emulsions and dispersion and encapsulated products to improve the stability against low pH, thermal treatment and stimulated peptic and tryptic digestions. The mechanism responsible for the stabilization of the SPI emulsion by SSP at acidic pH can be due to a balance between electrostatic interactions of SSP with SPI and interfacial adsorption of SSP.
PUBLIC INTEREST STATEMENT
In the food and pharmaceutical application oil-in-water or water-in-oil emulsions are very much needed for good quality products. Emulsions stabilized by proteins may lose their stability under gastric conditions due to changes in temperature, pH and the presence of digestive enzymes. Soy soluble polysaccharides are reported to have good emulsifying properties and it can be used to stabilize emulsions against gastric conditions, encapsulation of linoleic acid, β-carotene-chitosan, iron-lactoferrin, curcumin and denatured soy whey proteins in the emulsions. Soy soluble polysaccharide-Maillard reaction products showed better stability against simulated gastric fluids. It could be suggested that soy soluble polysaccharide can be used as an emulsifier and for encapsulation in the food and pharmaceutical industries particularly for targeted and sustained release of drug in the intestines.
Competing interests
The authors declare no competing interests.
1. Introduction
Soy soluble polysaccharides (SSPs) are biopolymers similar to pectin extracted from soy okara. SSP consists of β-1,4 galactan and α-1,3 or α-1,5 arabinan chains and homogalacturonan forming covalent bond with rhamnogalacturonan backbone with ~ 50 kD protein moiety, important for surface activity. SSP exhibits emulsifying properties due to its peptidoglycans (CitationNakamura et al., Citation2004a, 2006a). SSPs are highly water soluble, pH stable and show low bulk viscosity. SSP has the ability to generate interfacial films (17–30 nm thick) which can stabilize emulsions via steric repulsion (CitationNakamura et al., Citation2004b, 2006b). Interaction between negatively charged polysaccharide and positively charged proteins may develop a thick layer at the interface to inhibit coalescence (Gancz et al., Citation2005; Gu et al., Citation2004). SSP is a water-soluble polysaccharide contained galacturonic acids, which are about 18% of total sugar at SSP (Nakamura et al., Citation2001). SSP also holds a small amount of protein, contributing in the interfacial activity of the polysaccharides (Samant et al., Citation1993).
When two biopolymers, such as protein and polysaccharides, are mixed together at low concentrations in an aqueous solution, there is a homogenous solution formed. In the dispersion, the resulting protein and polysaccharides are distributed randomly. However, when protein and polysaccharide are mixed at high concentrations, there is a depletion interaction, and separation into two phases (Tolstoguzov, Citation1991). Interaction between protein and polysaccharide in the oil-in-water (o/w) emulsion and bulk aqueous phase may affect the interfacial activity of the protein-stabilized emulsions (McClements, Citation2006). The behaviour of protein and polysaccharide in an aqueous environment, at high concentrations, show either thermodynamic incompatibility or compatibility. These behaviours mainly depend on the charges of both protein and polysaccharide. At high concentrations and high ionic strengths thermodynamic incompatibility also known as segregative phase separation, is observed. If protein and polysaccharides contain similar electrical charges, this results in an electrostatic repulsion between protein and polysaccharides molecules and directing to the development of either protein-rich or polysaccharide-rich phases (Doublier et al., Citation2000; Kasapis, Citation2008; Tolstoguzov, Citation2003). In contrast, if protein and polysaccharide contain opposite charges, it leads to thermodynamic compatibility (known as associative phase separation or complex coacervation). This type of phase separation could occur at low concentrations (<3 to 4% w/v) and low ionic strengths (<M) of both molecules (Tolstoguzov, Citation2007) and pH between the isoelectric point of proteins and the pKa of the polysaccharides (Tolstoguzov, Citation1997). The above conditions lead both the molecules to attract each other and form two phases, namely, i) protein-polysaccharide rich phase and ii) a solvent-rich phase that is exhausted of the biopolymers. The electrostatic interaction between protein and polysaccharide takes place at a pH lower than the isolectric point. The binding sites should be negatively charged carboxylate, sulfate, pyruvate and acetate groups of polysaccharides and positively charged ε-amino group of lysyl residue, guanidium group of guanidine and an imidazole group of tryptophan of the proteins (Samant et al., Citation1993). Cross linking between amino groups of protein and carbonyl groups of polysaccharide could form protein-polysaccharide conjugates via Maillard reaction (Kato et al., Citation1990). These protein-polysaccharide Maillard reaction products were reported to stabilize emulsion systems, especially upon protein–polysaccharide Maillard reaction products were found to be effective in stabilizing emulsion systems, particularly during heating and under acidic condition (Akhtar & Dickinson, Citation2003; Dickinson & Semenova, Citation1992; Kasran et al., Citation2013). By using Maillard-type protein and polysaccharide complexes, release of emulsified bioactive in the gastrointestinal tract could be easily controlled (Lesmes & McClements, Citation2012; Oliver et al., Citation2009). These Maillard reaction products are reported to enhance the stability of o/w emulsions having citral. Citral is the key component of lemon essential oils with a strong lemon flavour, generally used for flavouring of food products. Basically, citral is a mono-terpene aldehyde composed of two geometrical isomers, neral and geranial at a ratio of 2:3 (Kimura et al., Citation1981).
Citral is proposed as an alternative to antibiotics in animal feed for the inhibition of bacterial infections. Delivery of citral to the animal intestines is likely to enhance its antibacterial efficacy as the intestine is a major reservoir of many foodborne pathogens. Now-a-days, citral is also used as natural preservatives in food formulations. But, the use of citral as an antimicrobial agent in the food or feed industry is limited by many factors, namely, chemical instability, high hydrophobicity and volatility. However, research suggests that the chemical stability of citral might be improved by emulsification. Citral showed a greater resistance to chemical transformation when present in emulsion droplets than in aqueous phase (Cho & McClements, Citation2009; Choi et al., Citation2010a, Citation2010b; Yang et al., Citation2011b; Zhao et al., Citation2013).
The fast-growing functional food markets indicate that beverages based on milk could be an ideal media for bioactive ingredients. Several bioactive ingredients have potential opportunities and challenges for development of functional dairy beverages (Sharma, Citation2005). However, the potential challenges in the formulation include poor solubility, unstable suspension, sedimentation, aggregation and uneven particle size distribution, which might be prevented by using soluble polysaccharides. Okara, which contains high protein and fibre content (Liu, Citation1997; Na Nakornpanom et al., Citation2010), could be used in human food. Dietary fibre is essential for maintaining human health, to alter the food matrix and increasing the bioavailability of nutrients. Dietary fibre could alter the time required to release oil from the digesta (Nakao et al., Citation2002; Na Nakornpanom et al., Citation2010). Bolus viscosity in the stomach and small intestine could be increased by dietary fibre. It also interacts with lipase enzyme and binds with bile acid to reduce enzyme activity (Drzikova et al., Citation2005; Pasquier et al., Citation1996; Thongngam & McClements, Citation2005). The objectives of this review article are to give outlines about the ability of soy soluble polysaccharide to stabilize the emulsion and dispersion against low pH and thermal treatment and to improve emulsion stability against peptic and tryptic digestions. The emulsification capacity of conjugated SPI-SSP in the gastrointestinal environments is also evaluated.
2. Effect of SSP in the emulsions and dispersions to improve the stability against peptic and tryptic digestions
2.1. Preparation of mixture of soy protein-polysaccharide (SPP) and soy soluble polysaccharide—Maillard reaction products (SSPMRP)
Mixtures of SPI—soy soluble polysaccharide (SSP) and soy soluble polysaccharide-Maillard reaction products (SSPMRP) were prepared according to the modified method of Yang et al. (Citation2014). 10% (w/w) of both SPI and SSP were mixed with water for 4 h. Dispersion pH was adjusted to 7.0 and stored at 4°C overnight. Soluble SPI and SSP solutions were collected by centrifuging of SPI and SSP separately at 35°C for 18,000 g for 40 min and collecting the supernatants. SSP-SPI were mixed at the ratio of 4:9, 1:2, 2.5:5, 4:5, respectively (dry basis) and freeze-dried followed by grinding to fine powders (particle size <0.13 mm) to generate SPP (soy protein-polysaccharide mixture). Maillard reaction was initiated by keeping SPP in saturated NaCl solution (RH = 80%) and thermally treated at 60, 65 and 70°C, respectively, for 36–96 h. The mixture was stirred for 4 h and centrifuged at 18,000 g for 40 min. Supernatant was collected and freeze-dried to collect SSPMRP. Citral-loaded emulsions stabilized by SPI, SSP and SSPMRP were prepared by the following modified method of Yang et al. (Citation2014). SSPMRP emulsions were prepared using 10% (w/w) citral and 2.5, 3.0, 3.3 and 5% of SSPMRP, respectively, which corresponded to citral and SSPMRP ratio of 5:1, 4:1, 3:1, 2:1. From emulsions, an aliquot of the emulsion was adjusted pH 3.0 with 2 M HCl with stirring at 30°C at 250 rpm for 30 min. On the other hand, SSP or SPI-stabilized citral rich emulsion were prepared with 10% citral and citral to SSP or SPI ratio at 3:1 only.
2.2. Emulsifying capacity of conjugated SPI, SSP and SSPMRP in the gastrointestinal environments
Emulsifying capability of individual soy protein isolate (SPI), soy soluble polysaccharide (SSP) and soy soluble polysaccharide Maillard reaction products (SSPMRP) were determining by the modifying method of Yang et al. (Citation2015) and evaluated/verified the results by comparing it with Yang et al. (Citation2015, Citation2015a)).
In the case of emulsions stabilized by SSPMRP, the droplet size was unimodal and did not increase during the entire 180 min. However, the droplet size became a multimodal distribution, if the emulsions were stabilized by SSP or SPI. The mean droplet size also significantly increased, even at the early stage of incubation under-simulated gastric conditions (SGC) for 25–60 min. These results are in agreement with previous studies showing the monomodal distribution of soy protein-polysaccharide Maillard reaction product (SPPMP)-stabilized (Yang et al., Citation2015). They also found that emulsion stabilized by SPP or SPI showed multi-modal distribution and an increase in the mean droplet size even at an early stage of incubation. We found that at pH 2.5 the droplets of SSPMRP-stabilized emulsion-exposed electro neutral surface charges (very low magnitude of surface charges) suggesting that the steric barrier created by electoral neutral complexes played an important role in stabilizing the emulsions in simulated gastric conditions. The steric barrier exhibited by the electro-neural complexes played a crucial role in stabilizing the emulsions in SGC. The citral release rate form SPI-emulsion, SSP-emulsion and SSPMRP-emulsion prior to incubation in the simulated gastrointestinal fluids (SGF) was in the following order of SPI>SSP>SSPMRP. This result is also in agreement with previous studies showing that the release rate of citral prior to incubation in the SGF was in order of SPI>soy protein-polysaccharide (SPP)>SPPMP (Yang et al., Citation2015). Our research suggested that lower rates of citral release was observed in both SSP and SSPMRP-stabilized emulsions in SGF than simulated intestinal fluids (SIF), which was a characteristic in favour of intestinal delivery. In emulsions stabilized by SPI, more than 65% of the citral was released from the emulsion droplets after incubation in SGF for 3 h, whereas SSP-emulsion and SSPMRP-emulsion released only 50% and 30% of citral, respectively. Complete release of citral from SPI- and SPP-stabilized emulsions after incubation in SIF took around 3 h. On the other hand, complete release of citral was found in the SSPMRP-stabilized emulsion after 5 h of incubation. The results suggest that SSPMRP-stabilized emulsions might help to increase the citral amount in the intestine with its release in a controlled manner. These results are supported by Yang et al. (Citation2015) and Fafaungwithayakul et al. (Citation2011). Negatively charged SSP might attach to the positively charged SPI molecules via electrostatic attraction, thus providing the extra stabilizing effect. On the other hand, SSP might not efficiently bond to SPI as both SSP and SPI are negatively charged and exhibit electrostatic repulsion under SIF conditions. In the SIF, due to a lack of protection from SSP, SPI might easily undergo digestion by pancreatin, leading to the destabilization of the droplet, hence, release of citral (Yang et al., Citation2015). SSPMRP improved the physical stability of citral-loaded emulsions and reduced the release of citral. Furthermore, chemical stability of citral was boosted in SSPMRP-stabilized emulsion under-simulated gastrointestinal conditions, compared to SSP- and SPI-stabilized emulsions. SSPMRP shows better capability to control the citral release under digestive conditions via two mechanisms, namely i) covalent linkage between SPI and SSP-which successfully inhibits digestion of SPI by pancreatin, and ii) SSP-SPI bonding—which provides steric effect to prevent destabilization of emulsion (Yang et al., Citation2015).
Our research found that in the SSPMRP-stabilized emulsion, the retention rate of citral decreased with decreasing pH but at a limited level. After SGF incubation at pH between 2.0 and 2.5, SSPMRP-stabilized emulsion showed 15% higher retention rate than SSP-stabilized emulsion. However, only 8% higher retention rate was observed for SSPMRP-stabilized emulsion at the same pH than SSP-stabilized emulsion. Yang et al. (Citation2015a) also found the same trend. They found that the retention rate of citral in SPPMP-stabilized emulsions showed 10% higher retention rate after SGF incubation at pH 1.5 than SSP-stabilized emulsion at the same pH. However, only 4% higher retention rate of citral was observed in SPP-stabilized emulsion at pH 2.0. We also found that the pepsin digestibility of SSPMRP–stabilized emulsion was reduced during heat treatment. This might be due to enhanced stability of the emulsion in the gastric conditions which exhibited better resistance to heat treatment (Yang et al., Citation2015a). On the contrary, prior to heat treatment the pepsin digestibility of SPI-stabilized emulsion was increased, resulting in the disintegration of emulsion droplets and release of citral during SGF incubation. The pepsin digestibility of SPI was reported to increase after heat treatment (Tsumura et al., Citation2004). Therefore, SSPMRP-stabilized emulsion improves the chemical stability of citral and enhances the emulsion stability. Our research showed that after SGF incubation, total retention rates of citral in the SSPMRP and SSP-stabilized emulsions were about 2.0 times and 1.5 times, respectively, higher than SPI-stabilized emulsions. Emulsion incubated only in SGF showed low concentrations of citral transformation than SGF-SIF incubation. Subsequently, citral concentration was the highest in SPI-stabilized and lowest in SSPMRP-stabilized emulsions (Figure ) was found by Yang et al. (Citation2015a). However, concentration of citral transformation was increased after SGF-SIF incubation rather than SGF only.
Figure 1. Gas chromatograms of citral and its transformation products in emulsion stabilized by soy protein isolate (SPI), soy protein-polysaccharide (SSP) and soy protien-polysaccharide Maillard reaction product (SPPMP) after simulated gastric fluids (SGF) incubation at pH 2.0 for 2 h followed by intestinal fluids (SIF) incubation at pH 7.5 for 4 h (Yang et al., Citation2015a).
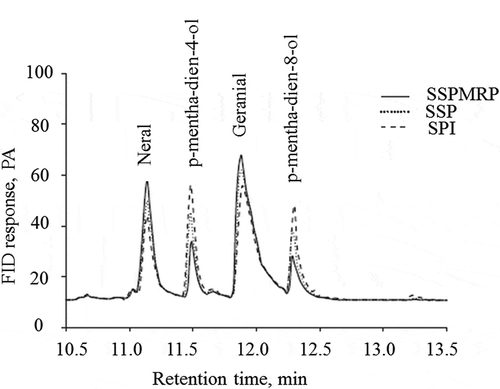
The influence of pH of SGF and pre-heating of emulsions was studied by Yang et al. (Citation2015a) and they found that the stability of the citral in unheated SPPMP-stabilized emulsions was responsive to the pH of SGF. In SGF, decreasing the pH effectively decreased the retention rate for geranial, but in case of neral, there was no change in retention rate even when the pH decreased to 2.0 from 2.5. When the pH value decreased to 1.5, the retention rate of both geranial and neral were different. High concentrations of H+ were observed in the emulsion at low pH, which might be the main reasons for the transformation of citral to monoterphene alcohols. Research revealed that H+ join in the acid-induced transformation of citral to form monoterphene alcohols (Kimura et al., Citation1982). The results were supported by Yang et al. (Citation2011a) who found the quick transformation of citral in the emulsion during decreasing of pH from 7.0 to 3.0.
The differences between heated and unheated emulsions on the retention rate of citral are due to the pH of the SGF. The effect of heat treatment on the stability of citral stabilized by SSPMRP prior to SGF incubation is shown in Figure . The difference in the retention rate of citral between heated and unheated emulsions prior to SGF incubation is pH-dependent (pH of the SGF). Research suggested that there was a significant difference in the retention rate of both geranial and neral and citral between heated and unheated SPPMP-stabilized emulsions only at pH 1.5. In contrast, prior to SGF incubation at pH 1.5, 2.0 and 2.5, the retention rate of citral in the heated emulsions decreased significantly in the case of SPI and SSP-stabilized emulsion than unheated ones. During SGF incubation the stability of citral is related to the pepsin digestibility of the emulsifier. An increase in the pepsin digestibility of SPI prior to SGF incubation resulted in the disintegration of emulsion droplets and the release of the citral in heated emulsions (Tsumura et al., Citation2004). Soy protein hydrolyzed by protease enzyme in the SIF and the digesta lead to the disintegration of emulsion droplets. Addition or presence of SSP to SPI by physical interaction and/or covalent linkage protects peptide bonds against proteolysis, thus delaying citral release from droplets (Yang et al., Citation2015). It is interesting that the retention rate of citral was lower in chicken digesta than in SIF. It might be due to the presence of some chemical compounds such as metal cations, amino acids, and other anti-oxidant. These chemical compounds might interact with the released citral, including its transformation. The concentration and locations of citral transformation might also influence the rate and extent of transformation of citral in emulsion systems (Choi et al., Citation2010a).
Figure 2. Retention rate of neral and geranial heated (TH) and unheated soy soluble polysaccharide Maillard reaction product (SSPMRP)–stabilized emulsions after incubation in simulated gastric fluids (SGF) at around stomach pH (Yang et al., Citation2015a).
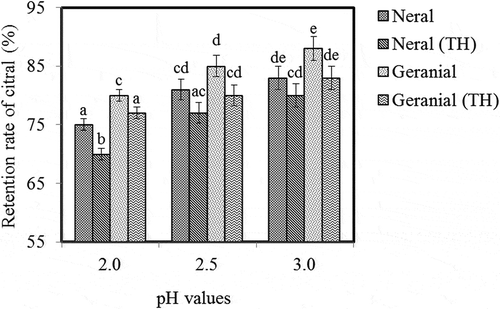
2.3. Emulsifying capacity of SSP in the peptic and tryptic digestions
The addition of pectinase-hydrolysed soy polysaccharide (PHSSP) (2% concentration) in o/w emulsion stabilized by unheated (U) SPI and heated ((H), 80°C, 30 min) SPI, did not show any significant differences in the height of oil phase or emulsion phase. The sediment phase height of PHSSP stabilized emulsion was higher at pH 2.0 (near about stomach pH) than emulsions having pH 4.5 and 7.0. This might be due to result of associating flocculation via linkage of negatively charged PHSSP and positively charged SPI (Fafaungwithayakul et al., Citation2011). When the H-SPI was used in the emulsion, the sedimentation was low at pH 7.0. It was likely due to the electrostatic repulsion between negatively charged SPI and PHSSP. This repulsion was responsible for the low degree of insoluble conservation in the sediment. At pH 7.0, heat treatment (80°C, 30 min) of SPI during emulsification might reduce the insoluble conservation formation between protein and PHSSP. This might be due to the unfolding of proteins and the exposure of hydrophobic and charged reactive groups of proteins for inhibition of aggregation (Fafaungwithayakul et al., Citation2011). At pH 7.0, emulsion stabilized by H-SPI with 2.0% PHSSP exhibited a slight increase of protein (0.57 to 0.13%) in the sediment phase, although most of the proteins were in the emulsion phase. However, emulsion stabilized by U-SPI and containing 2.0% PHSSP showed around 75% of the total protein in the sediment phase. This might be due to the aggregation of unheated soy proteins in the aqueous phase (bulk) (Kuipers et al., Citation2006). In the presence of PHSSP at pH 7.0, heated soy proteins were better absorbed in the o/w interface rather than dispersed in the serum phase or in the sediment phase. Nevertheless, around 75% of proteins were in the sediment phase due to the conservation of opposite charge of proteins and PHSSP at pH 2.0 only (Fafaungwithayakul et al., Citation2011). During peptic digestion, increasing the PHSSP content (6% of PHSSP) notably increased the oil separation in the undigested emulsion at pH 2.0. Emulsions with above 2% PHSSP was prone to sediment (extreme increase of sediment phase height), when compared to the emulsions without PHSSP. This might be due to insoluble conservation formation between two opposite molecules at pH 2.0 (Fafaungwithayakul et al., Citation2011). On the other hand, prior to peptic digestion at pH 2.0 the emulsion phase was not affected by PHSSP addition even at a concentration of 6% PHSSP. In the absence of PHSSP, oil phase height and sediment phase height were increased by peptic digestion whereas; emulsion phase height was unaffected (Fafaungwithayakul et al., Citation2011). The result suggested that an increase in the sedimentation phase of the emulsion was due to aggregation of the SPI hydrolysate (Kuipers et al., Citation2006).
The presence of bile salt during trypsin digestion considerably decreased the sediment phase height without altering the oil phase and emulsion phase height. The result indicated that tryptic digestion readily digests the proteins in sediment phase. In contrast, peptic digestion was not able to release oil from the emulsions with PPSSP above 4%. It could be concluded that the oil phase only released if the high fiber emulsion were digested by trypsin. At neutral pH, the insoluble coacervate in the emulsion might be lowered using H-SPI. However, the addition of PHSSP up to 2.0% in o/w could be achievable (Fafaungwithayakul et al., Citation2011). Addition of PHSSP as a source of fibre in soy stabilized emulsion affects the emulsion stability before and after digestion by both pepsin and trypsin. In the concentration ranges of PHSSP between 0% and 2.0% w/v, the oil phase of the soy stabilized emulsion might be released under the stomach condition. Oil release in stomach or during the entrance in duodenum might favour lipase digestion in the small intestine. The presence of high fibre in the diet, offers advantages including delaying and/or modifying protein digestion. This is due to the formation of coacervates between the fibres with proteins at acidic pH (Fafaungwithayakul et al., Citation2011).
3. Effect of SSP in the encapsulated products to improve the emulsion stability and bio-accessibility against low pH and thermal treatment
3.1. SSP-polysaccharides complex
Studies on linoleic acid emulsification with SSP (unmixed), gum Arabic and maltodextrin revealed that emulsions stabilized by SSP had the smallest diameter of oil droplets in the emulsion followed by gum Arabic and maltodextrin (Minemoto et al., Citation2002). Maltodextrin produced a coarse emulsion which was prone to phase-separation due to lack of emulsion stability. The median diameter of the SSP-maltodextrin emulsion droplets decreased and encapsulation efficiency increased as the weight fraction of SSP was increased. The weight fraction of gum Arabic barely affected the median diameter and the encapsulation efficiency. SSP and gum Arabic contain protein residue and possesses emulsifying stability (Maeda, Citation2000; Randall et al., Citation1988) which resulted in the small median diameter and produced high encapsulation efficiency. This might be due to the adsorption of proteins on the surface of oil droplets, thus acting as a stabilizer (Matsumura, Citation2001). Proteinous residues of gum Arabic could be adsorbed and produce a viscoelastic film at the oil–water interface of the emulsion. SSP contained much more proteinaceous residues than gum Arabic; as a result SSP could strongly adsorb and form a film in the interface (Matsumura et al., Citation2000). Emulsion prepared with only maltodextrin was unstable. However, SSP mixed with maltodextrin showed the stability of the emulsion at any weight fraction (weight fraction 0.25, 0.5, 0.75 and 1.0). The mixture of gum Arabic and maltodextrin at the weight fraction of 0.25 did not show any stabilizing ability of the emulsion although the median diameter was small; however, the mixtures at the weight fractions ≤0.5 had stabilizing effect (Minemoto et al., Citation2002).
3.2. SSP-β carotene-chitosan complex
Research on SSP concentrations required to fully cover the oil-water interface and stabilize the droplets of emulsion showed that low SSP concentrations did not stabilize emulsions. However, if the SSP concentration is too high in the emulsions, there would be a lot of non-adsorbed SSP in the aqueous phase, which would interact with the cationic chitosan molecules leading to the creation of multiple-layered interfacial membranes. The average droplet size distribution of the β- carotene emulsion was independent of SSP concentration even at SSP concentrations above 4 wt%. The concentration of non-adsorbed SSP should be minimized to avoid the interaction of non-adsorbed SSP with chitosan. Emulsions with low concentration (0.5–3 wt%) of SSP were very unstable, as the emulsions exhibited a remarkable increase in the transmission due to creaming and floating during the centrifugation at 4000 rpm for 40 min. The emulsion was stable at SSP concentration above 4%, as there was a minimum change in the integral transmission. It might be concluded that the oil droplets could not be fully covered at <4 wt% SSP concentration, thus, the emulsions exposed large particle size and unstable to creaming and coalescence. Only SSP-coated droplets showed ∼ −34 mV net charge, whereas, the net charge of the droplets shifted from negative to positive prior to increasing the chitosan concentration. At 2 wt% chitosan concentrations, the net charge of oil droplet concentration showed a positive value of ∼ +58 mV). The change in ζ- potential suggested that positively charged chitosan might adsorb onto the negatively charged SSP-β-carotene emulsions (Hou et al., Citation2010). Research reveals that charged polyelectrolytes have the ability to absorb onto the surface of oppositely charged colloidal particles (Chodanowski & Stoll, Citation2001; Dobrynin, Citation2001; Jonsson & Linse, Citation2001).
Low concentration of chitosan (0.167 wt%) did not show any significant increase of the mean diameter. Results indicate that a minimum droplet aggregation and a negative charge on the emulsion droplets are sufficient to prevent droplet flocculation via electrostatic repulsion (Ogawa et al., Citation2003). However, increasing the chitosan concentration showed an increase in the mean diameter, which is related to the extensive droplet aggregation. The maximum level of droplet aggregation occurred at a chitosan concentration of 0.333 wt% with net charge of the droplets being −6.76 mV. Chitosan adsorption on to the SSP-stabilized oil droplets promoted the formation of an elastic and stronger structure. This structure might be attributed to the development of highly flocculated droplet network structure by SSP-chitosan. Electrostatic deposition of cationic chitosan on to anionic SSP-coated droplets generated multilayer interfacial complexes. SSP-stabilized emulsions with adsorbed chitosan exhibited viscoelastic properties that can be characterized as weak gel (Mandala et al., Citation2004).
In another study, it is reported that a strong droplet network structure is formed due to the adsorption of chitosan onto the SSP-coated droplet interface. This increases the viscosity of SSP-stabilized β-carotene emulsion. Consequently, the chitosan concentration above 0.5 wt% showed a higher amount of β-carotene retention in the SSP-stabilized emulsion due to the formation of the SSP-chitosan interface complexes. This SSP-chitosan complex offered an inhibitory effect on lipid oxidation. Nonetheless, a chitosan concentration of 1 wt% in the SSP-stabilized emulsions exposed a stronger droplet network, but lower chemical stability than 0.5% chitosan concentration. Results indicated that flocculation might be promoted as a result of free polyelectrolytes in the solution (Cho & McClements, Citation2009).
3.3. Iron–lactoferrin (FeLf) complex
Iron-lactoferrin complex containing 2% (w/w) SSP is reported to suppress heat (100°C for 20 min) induced aggregation and FeLf precipitation. However, gum Arabic, gellan gum and micro-crystalline cellulose did not have any effect on this phenomenon (Ueno et al., Citation2012). The stability of lactoferrin-polysaccharide mixtures is affected by the type of polysaccharides used. For eg., lactoferrin-pectin complex was reported to yield the most stable complex (Peinado et al., Citation2010). SSP and pectin have similar structural characteristics, and these structural characteristics might be responsible for the stabilization of lactoferrin. At pH 6.5, SSP-FeLf suspensions remain clear after heating at 120°C for 4 min while Fe-Lf without SSP shows cloudy solutions. Addition of SSP was observed to increase the thermal stability of Fe-Lf complex, regardless of the level of addition up to a level of 0.001 to 0.004% (w/w) of SSP. Moreover, turbidity of the heat-induced Fe-Lf was completely inhibited by adding ≥0.01% (w/w) SSP.
Addition of SSP was also found to decrease the heat-induced degradation of iron in Fe-Lf complex. Heated Fe-Lf reduced in its iron content to 18.9% of the unheated Fe-Lf supernatant. On the other hand, SSP-FeLf retained 90% of the soluble lectoferrin and more than 78% soluble iron after heated at 120°C for 4 min. SSP at 0.001% (w/w) concentration retained 82% of the soluble iron and 16% soluble lectoferrin with very low viscosity. The result indicated that SSP at 0.001% (w/w) was not enough to improve heat stability of Fe-Lf. By contrast, heat stability of Fe-Lf was improved by addition of ≥0.01% (w/w) SSP at pH 6.5 (Ueno et al., Citation2012). Thus, it is observed that when present alone, SSP at 0.01% (w/w) might not solubilize inorganic iron, but on addition to Fe-Lf complex, solubilize Fe-Lf via interaction between SSP and Lf. At ≥0.01% (w/w) SSP concentration, SSP-FeLf showed stable suspensions with ζ-potential—22 mV. The negative ζ-potential associated with improved stability of Lf-pectin mixture at neutral pH (Ueno et al., Citation2012). The addition of pectin also decreased ζ-potential to improve the stability of an Lf-pectin mixture against heating (Bengoechea et al., Citation2011). The stability of Fe-Lf resembles those of Lf-pectin suggesting that electrostatic interactions were responsible for the improvement of thermal stability of SSP-FeLf. Overall, the results show important implications for the development of SSP-FeLf complex food products with pasteurization (Ueno et al., Citation2012).
3.4. In vitro bio-accessibility of SSP-curcumin complex
Curcumin, obtained from the rhizomes of Curcuma longa, is known to be a bioactive with properties including antioxidant, anti-inflammatory and anti-cancer activity (Tang, Citation2004). However, the limitations in its solubility under aqueous conditions, poses a challenge in effectively using this bioactive. Methods to improve the bioavailability of curcumin have been an area of interest in the recent years. This is done by processes including encapsulation, micro/nano emulsions and fabrication of nano particles. Addition of SSP as an encapsulant for curcumin revealed the reduction of curcumin degradation tested at 80°C for 2.5 h at pH 7.0 and 4.0. Curcumin degradation was about 98% at pH 7.0 after heating at 80°C for 2.5 h, whereas the value was only 36% at pH 4.0 without the addition of SSP. Curcumin degradation was observed remarkably higher prior to initial heating for 30 min, after that the rate of degradation gradually declined at pH 7.0. Result suggested that curcumin without addition of SSP was susceptible to degradation in aqueous suspension and the degradation is faster at neutral pH than acidic pH (Chen et al., Citation2017). In contrast, addition of to the SSP-curcumin complex significantly (p < 0.05) improved the heat stability of curcumin both at neutral and acidic pH. In SSP-curcumin complex, curcumin degradation after heating at 80°C for 2.5 h was reduced to 90 from 98% at pH 7.0, whereas, at pH 4.0 curcumin degradation was reduced to 20 from 36%. However, the increase in heat stability of curcumin by the SSP-curcumin complex at pH 7.0 was poorer than that of SPI-curcumin complex (Chen et al., Citation2015a). The degradation of curcumin was 55–65% at pH 7.0 after heating at 85°C for 3 h for SPI-curcumin complex. The difference in heat stability between SSP-curcumin and SPI-curcumin complex might be due to the difference in polarity of the curcumin-binding sites between SPI and SSP (Chen et al., Citation2017).
For curcumin without SSP, bio-accessibility was 25 and 35% at pH 7.0 and pH 4.0, respectively. The bioavailability of SSP-curcumin was 77–83% was similar to SPI-curcumin complex which was about 85% (Chen et al., Citation2015b). At pH 7.0 and 4.0, SSP-curcumin complex improved the curcumin stability against digestion. Without SSP, 25–30% curcumin degraded during whole digestion (whole-simulated gastric and intestinal digestion (SGF + SIF)), whereas only 4–15% degraded for SSP-curcumin complexes (Chen et al., Citation2017). The bioavailability of curcumin without SSP can reach about 35 and 46%; however, SSP-curcumin complex reached 90 and 87%, respectively. The results indicated that SSP-curcumin would outstandingly improve the bio-accessibility of curcumin (Chen et al., Citation2017). The probable mechanism of SSP-curcumin complex is illustrated in Figure .
Figure 3. The possible mechanism of soy soluble polysaccharide (SSP)-curcumin complexes, adapted with permission (Chen et al., Citation2017) Copyright © 2017, American Chemical Society.
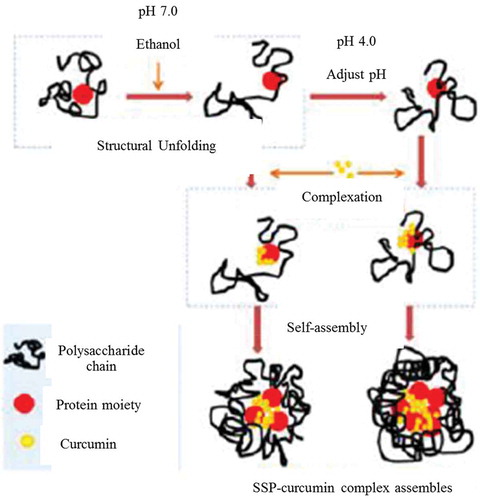
3.5. SSP-denatured soy whey proteins (dSWP) complex
Low concentrations dSWP or SSP prepared emulsions demonstrated limited stability. SSP-dSWP mixture stabilized o/w emulsions (5 wt%) against coalescence and phase separation. At an optimal SSP: dSWP (1.5:1.0) ratio, these emulsions showed a pH-dependent stability against phase separation, with the emulsion more stable at pH 3.0 than at pH 8.0. At pH 3.0–4.0, emulsions exhibited long-term stability than pH 5.0–8.0, which was regardless of the protein–polysaccharide ratio. A low surface charge (−2 mV) at lower pHs and the resultant stabilization through steric hindrance, is suggested to be the reason for emulsion stability. This is attributed to the due to the dense packing of SSP-dSWP complex around the oil droplets facilitated by pH 3.0 and 4.0. As pH of the complex was increased, a higher negative charge (−20 mV) at pH 7.0 to 8.0 leads to electrostatic repulsion amongst the SSP-dSWP complexes preventing layer formation around the dispersed oil droplets. This results in limited emulsion stability (Ray & Rousseau, Citation2013).
Low concentration of adsorbed SSP-dSWP complexes was thinner; there might be a greater possibility of direct droplet-droplet contact, flocculation and coalescence of emulsion. Higher SSP loads increased the emulsion stability, depending on the pH (Ray & Rousseau, Citation2013). Stabilization of the emulsions by electrolytes like NaCl depended on the sequence of addition of the electrolyte. Addition of NaCl, prior to the emulsification process, resulted in hindering the electrostatic interaction between SSP and dSWP, thus destabilizing the emulsion. Addition of NaCl after the emulsification process-induced surface charge screening of the oil droplets, thus improving emulsion stability. Thus it is observed that, stability of SSP–dSWP emulsions is affected by the concentration of the individual polymer, pH and ionic strength (Bengoechea et al., Citation2011).
4. Conclusion
SSP could stabilize acid milk dispersions, emulsions via steric stabilizing mechanism due to the presence of a dense neutral sugar side chain of SSP on the surface of protein particles. Stabilization of dispersions/emulsions through SSP against creaming, coalescence and sedimentation depends on SSP concentration, pH, and ionic strength of the emulsions and dispersions. Addition of SSP to the linoleic acid, iron-lactoferrin and β carotene-chitosan enhanced the thermal stability of emulsion by shifting the environment of the liquid phase. Soy soluble polysaccharide-Maillard reaction products (SSPMRP) considerably enhanced the emulsification capacity for maintaining the physical stability of citral emulsion for a long period of storage upon thermal treatment and under-simulated gastric conditions compared with soy soluble polysaccharide and soy protein isolate only. Research on in vitro analysis in our lab revealed that SSPMRP performed better stability against simulated gastric fluids and might be used for encapsulation of citral in the emulsions. It can be concluded that SSPMRP might be used in the food and pharmaceutical industries to improve their potential applications in the food and drug industry. The article helps to develop an interest for the development of SSP enrich hydrophobic bioactives to hold the potential formulas in the functional foods. However, it is very much necessary to investigate the SSP roles in the stability of emulsion prior to in vivo peptic and tryptic digestion and also the release of SSP during in vivo digestions.
Acknowledgements
The authors acknowledge Ministry of Education (2018/497/MoE), Bangladesh for research grants.
Disclosure statement
The authors declare that they have no conflict of interest.
Additional information
Funding
Notes on contributors
Md. Anisur Rahman Mazumder
Md. Anisur Rahman Mazumder, working as an Associate Professor at the Department of Food Technology and Rural Industries, Bangladesh Agricultural University (BAU) for more than 12 years. He completed his first degree in B. Sc. Food Engineering and Master of Science in Food Engineering from Bangladesh Agricultural University, Bangladesh. Thereafter, he has been awarded Doctor of Philosophy (PhD) major in Food Science from Kasetsart University, Bangkok, Thailand and post-doctoral research in Micro-encapsulation from Karunya Institute of Technology and Sciences, Tamil Nadu, India. He has published more than 25 national and international papers in the reputed journals. Moreover, he attended in different International conferences across the world. His area of speciality is in functional foods, phytochemical analysis, micro-encapsulations and Maillard reaction. This research is based on his earned knowledge on polysaccharide and emulsion stability against Stimulated Gastric Conditions and food complexes.
References
- Akhtar, M., & Dickinson, E. (2003). Emulsifying properties of whey protein-dextran conjugates at low pH and different salt concentration. Colloids and Surfaces. B, Biointerfaces, 31(1–4), 125–14. https://doi.org/10.1016/S0927-7765(03)00049-3
- Bengoechea, C., Jones, O. G., Guerrero, A., & McClements, D. J. (2011). Formation and characterization of lactoferrin/pectin electrostatic complexes: Impact of composition, pH and thermal treatment. Food Hydrocolloids, 25(5), 1227–1232. https://doi.org/10.1016/j.foodhyd.2010.11.010
- Chen, F. P., Ou, S. Y., Chen, Z., & Tang, C. H. (2017). Soy soluble polysaccharide as a nano carrier for curcumin. Journal of Agricultural and Food Chemistry, 65(8), 1707–1714. https://doi.org/10.1021/acs.jafc.6b05087
- Chen, F-P., Li, B-S., & Tang, C-H. (2015a). Nanocomplexation between Curcumin and Soy Protein Isolate: Influence on Curcumin Stability/Bioaccessibility and in Vitro Protein Digestibility. Journal of Agricultural and Food Chemistry, 63(13), 3559−3569. doi:10.1021/acs.jafc.5b00448
- Chen, F-P.., Li, B-S.., & Tang, C-H.. (2015b). Nanocomplexation of soy protein isolate with curcumin: Influence of ultrasonic treatment.Food Research International.2015 2015 75.06.009
- Cho, Y. H., & McClements, D. J. (2009). Theoretical stability maps for guiding preparation of emulsions stabilized by protein-polysaccharide interfacial complexes. Langmuir, 25(12), 6649–6665. https://doi.org/10.1021/la8006684
- Chodanowski, P., & Stoll, S. (2001). Polyelectrolyte adsorption on charged particles: Ionic concentration and particle size effects - A Monte Carlo approach. The Journal of Chemical Physics, 115(10), 4951–4960. https://doi.org/10.1063/1.1392357
- Choi, S. J., Decker, E. A., Henson, L., Popplewell, L. M., Henson, L., Popplewell, L. M., & McClements, D. J. (2010a). Influence of droplet charge on the chemical stability of citral in oil-in-water emulsions. Journal of Food Science, 75(6), C536–C540. https://doi.org/10.1111/j.1750-3841.2010.01693.x
- Choi, S. J., Decker, E. A., Henson, L., Popplewell, L. M., Henson, L., Popplewell, L. M., & McClements, D. J. (2010b). Inhibition of citral degradation in model beverage emulsions using micelles and reverse micelles. Food Chemistry, 122(1), 111–116. https://doi.org/10.1016/j.foodchem.2010.02.025
- Dickinson, E., & Semenova, M. G. (1992). Emulsifying properties of covalent protein-dextran hybrids. Colloids and Surfaces, 64(3), 299–310. https://doi.org/10.1016/0166-6622(92)80109-F
- Dobrynin, A. V. (2001). Effect of solvent quality on polyelectrolyte adsorption at an oppositely charged surface. The Journal of Chemical Physics, 114(18), 8145–8153. https://doi.org/10.1063/1.1350818
- Doublier, J. L., Garnier, C., Renard, D., & Sanchez, C. (2000). Protein-polysaccharide interactions. Current Opinion in Colloid & Interface Science, 5(3–4), 202–214. https://doi.org/10.1016/S1359-0294(00)00054-6
- Drzikova, B., Dongowski, G., Gebhardt, E., & Habel, A. (2005). The composition of dietary fiber-rich extrudates from oat affects bile acid binding and fermentation in vitro. Food Chemistry, 90(1–2), 181–192. https://doi.org/10.1016/j.foodchem.2004.03.041
- Fafaungwithayakul, N., Hongsprabhas, P., & Hongsprabhas, P. (2011). Effect of soy soluble polysaccharide on the stability of soy-stabilised emulsions during in vitro protein digestion. Food Biophysics, 6(3), 407–415. https://doi.org/10.1007/s11483-011-9216-1
- Gancz, K., Alexander, M., & Corredig, M. (2005). Interactions of high methoxyl pectin with whey proteins at oil/water interfaces. Journal of Agricultural and Food Chemistry, 53(6), 2236–2241. https://doi.org/10.1021/jf048683f
- Gu, Y. S., Decker, E. A., & McClements, D. J. (2004). Influence of pH and α-carrageenan concentration on physicochemical properties and stability of α-lactoglobulin-stabilized oil-in-water emulsions. Journal of Agricultural and Food Chemistry, 52(11), 3626–3632. https://doi.org/10.1021/jf049728f
- Hou, Z., Gao, Y., Yuan, F., Liu, Y., Li, C., & Xu, D. (2010). Investigation into the physicochemical stability and rheological properties of β-carotene emulsion stabilized by soybean soluble polysaccharides and chitosan. Journal of Agricultural and Food Chemistry, 58(15), 8604–8611. https://doi.org/10.1021/jf1015686
- Jonsson, M., & Linse, P. (2001). Polyelectrolyte-macro ion complexation. I. Effect of linear charge density, chain length, and macro-ion charge. The Journal of Chemical Physics, 115(7), 3406–3418. https://doi.org/10.1063/1.1385792
- Kasapis, S. (2008). Phase separation in biopolymer gels: A low- to high-solid exploration of structural morphology and functionality. Critical Reviews in Food Science and Nutrition, 48(4), 341–359. https://doi.org/10.1080/10408390701347769
- Kasran, M., Cui, S. W., & Goff, H. D. (2013). Covalent attachment of fenugreek gum to soy whey protein isolate through natural Maillard reaction for improved emulsion stability. Food Hydrocolloids, 30(2), 552–558. https://doi.org/10.1016/j.foodhyd.2012.08.004
- Kato, A., Sasaki, Y., Furuta, R., & Kobayashi, K. (1990). Functional protein/polysaccharide conjugate prepared by controlled dry heating of ovalbumin/dextran mixtures. Agricultural and Biological Chemistry, 54(1), 107–112. https://doi.org/10.1080/00021369.1990.10869907
- Kimura, K., Iwata, I., & Nishimura, H. (1981). Degradation of products of citral by acid: Minor components responsible for off odor of deteriorated lemon. Journal of the Agricultural Chemical Society of Japan, 55(11), 1073–1079. https://doi.org/10.1271/nogeikagaku1924.55.1073
- Kimura, K., Iwata, I., & Nishimura, H. (1982). Relationship between acid-catalyzed cyclization of citral and deterioration of lemon flavor. Agricultural and Biological Chemistry, 46(5), 1387–1389. https://doi.org/10.1080/00021369.1982.10865253
- Kuipers, B. J. H., van Koningsveld, G. A., Alting, A. C., Driehuis, F., Vorgen, A. G. J., & Gruppen, H. (2006). Opposite contribution of glycinin and β-conglycinin-derived peptides to the aggregation behavior of soy protein isolate hydrolysates. Food Biophysics, 1(4), 178–188. https://doi.org/10.1007/s11483-006-9019-y
- Lesmes, U., & McClements, D. J. (2012). Controlling lipid digestibility: Response of lipid droplets coated by β-lactoglobulin-dextran Maillard conjugates to simulated gastrointestinal conditions. Food Hydrocolloids, 26(1), 221–230. https://doi.org/10.1016/j.foodhyd.2011.05.011
- Liu, S. K. (1997). Soybeans: Chemistry, technology, and utilization. Chapman & Hall.
- Maeda, H. (2000). Soluble soybean polysaccharide. In G. O. Phillips & P. A. Williams (Eds.), Handbook of hydrocolloids (pp.693-709). CRC Press, Woodhead Publishing.
- MaMandala, I. G., Savvas, T. P., & Kostaropoulos, A. E. (2004). Xanthan and locust bean gum influence on the rheology and structure of a white model-sauce. Journal of Food Engineering, 64(3),335–342.
- Matsumura, Y. (2001). Interaction of protein at oil droplet surfaces in food emulsions. Japan Journal of Food Engineering (In Japanese), 2(3), 87–95. https://doi.org/10.11301/jsfe2000.2.87
- Matsumura, Y., Satake, C., Egami, M., & Mori, T. (2000). Interaction of gum arabic, maltodextrin and pullulan with lipids in emulsions. Bioscience, Biotechnology and Biochemistry, 64(9),1827–1835.
- McClements, D. J. (2006). Non-covalent interactions between proteins and polysaccharides. Biotechnology Advances, 24(6), 621–625. https://doi.org/10.1016/j.biotechadv.2006.07.003
- Minemoto, Y., Fang, X., Hakamata, K., Watanabe, Y., Adachi, S., Kometani, T., & Matsuno, R. (2002). Oxidation of linoleic acid encapsulated with soluble soybean polysaccharide by spray-drying. Bioscience, Biotechnology, and Biochemistry, 66(9), 1829–1834. https://doi.org/10.1271/bbb.66.1829
- Na Nakornpanom, N., Hongsprabhas, P., & Hongsprabhas, P. (2010). Effect of soy residue (okara) on in vitro protein digestibility and oil release in high-calorie emulsion stabilized by heated mixed proteins. Food Research International, 43(1), 26–32. https://doi.org/10.1016/j.foodres.2009.08.002
- Nakamura, A., Furuta, H., Maeda, H., Nagamatsu, Y., & Yoshimoto, A. (2001). Analysis of structural components and molecular construction of soybean soluble polysaccharides by stepwise enzymatic degradation. Bioscience, Biotechnology, and Biochemistry, 65(10), 2249–2258. https://doi.org/10.1271/bbb.65.2249
- Nakamura, A., Yoshida, R., Maeda, H., & Corredig, M. (2006a). Soy soluble polysaccharide stabilization at oil-water interfaces. Food Hydrocolloid, 20(2–3), 277–283. https://doi.org/10.1016/j.foodhyd.2005.02.018
- Nakamura, A., Yoshida, R., Maeda, H., & Corredig, M. (2006b). The stabilizing behavior of soybean soluble polysaccharide and pectin in acidified milk beverages. International Dairy Journal, 16(4), 361–369. https://doi.org/10.1016/j.idairyj.2005.01.014
- Nakamura, A., Yoshida, R., Maeda, H., Furuta, H., & Corredic, M. (2004a). Study of the role of the carbohydrate and protein moieties of soy soluble polysaccharides in their emulsifying properties. Journal of Agricultural and Food Chemistry, 52(17), 5506–5512. https://doi.org/10.1021/jf049728f
- Nakamura, A., Yoshida, R., Maeda, H., Furuta, H., & Corredig, M. (2004b). Study of the role of the carbohydrate and protein moieties of soy soluble polysaccharides in their emulsifying properties. Journal of Agricultural and Food Chemistry, 52(17), 5506–5512. https://doi.org/10.1021/jf049728f
- Nakao, M., Ogura, Y., Satake, S., Ito, I., Iquchi, A., Takagi, K., & Nabeshima, T. (2002). Usefulness of soluble dietary fiber for the treatment of diarrhea during enteral nutrition in elderly patients. Nutrition, 18(1), 35–39. https://doi.org/10.1016/S0899-9007(01)00715-8
- Ogawa, S., Decker, E. A., & McClements, D. J. (2003). Production and characterization of O/W emulsions containing cationic droplets stabilized by lecithin-chitosan membranes. Journal of Agricultural and Food Chemistry, 51(9), 2806–2812. https://doi.org/10.1021/jf020590f
- Oliver, C. M., Augustin, M. A., & Sanguansri, L. (2009). Maillard-based casein carbohydrate microcapsules for the delivery of fish oil: Emulsion stability during in vitro digestion. Australian Journal of Dairy Technology, 64(1), 80–83. http://hdl.handle.net/102.100.100/112979?index=1
- Pasquier, B., Armand, M., Guillon, F., Castelain, C., Borel, P., Barry, J. L., Pleroni, G., & Lairon, D. (1996). Viscous soluble dietary fibers alter emulsification and lipolysis of triacylglycerols in duodenal medium in vitro. The Journal of Nutritional Biochemistry, 7(5), 293–302. https://doi.org/10.1016/0955-2863(96)00030-7
- Peinado, I., Lesmes, U., Andrés, A., & McClements, J D.. (2010). Fabrication and morphological characterization of biopolymer particles formed by electrostatic complexation of heat treated lactoferrin and anionic polysaccharides. Langmuir, 26(12), 9827–9834. https://doi.org/10.1021/la1001013 12 doi:10.1021/la1001013
- Randall, R. C., Phillips, G. O., & Williams, P. A. (1988). The role of the proteineous component on the emulsifying properties of gum Arabic. Food Hydrocolloids, 2(2), 131–140. https://doi.org/10.1016/S0268-005X(88)80011-0
- Ray, M., & Rousseau, D. (2013). Stabilization of oil-in-water emulsions using mixtures of denatured soy whey proteins and soluble soybean polysaccharides. Food Research International, 52(1), 298–307. https://doi.org/10.1016/j.foodres.2013.03.008
- Samant, S. K., Singhal, R. S., Kulkarni, P. R., & Rege, D. V. (1993). Protein–polysaccharide interactions: A new approach in food formulations. International Journal of Food Science, 28(6), 547–562. https://doi.org/10.1111/j.1365-2621.1993.tb01306.x
- Sharma, R. (2005). Market trends and opportunities for functional dairy beverages. Australian Journal of Dairy Technology, 60(2), 195–198.
- Tang, C. H. (2004). Functional foods from spice turmeric. In C. H. Tang (Ed.), Plant functional foods (pp. 379-402). Chemical Industry Press.
- Thongngam, M., & McClements, D. (2005). Isothermal titration calorimetry study of the interactions between chitosan and a bile salt (sodium taurocholate). Food Hydrocolloids, 19(5), 813–819. https://doi.org/10.1016/j.foodhyd.2004.11.001
- Tolstoguzov, V. B. (1991). Functional properties of food proteins and role of protein-polysaccharide interaction. Food Hydrocolloids, 4(6), 429–468. https://doi.org/10.1016/S0268-005X(09)80196-3
- Tolstoguzov, V. B. (1997). Protein-polysaccharide interactions. In S. Damodaran & A. Paraf (Eds.), Food proteins and their applications in foods (pp. 25-55). Marcel Dekker Inc.
- Tolstoguzov, V. B. (2003). Some thermodynamic considerations in food formulation. Food Hydrocolloids, 17(1), 1–23. https://doi.org/10.1016/S0268-005X(01)00111–4
- Tolstoguzov, V. B. (2007). Ingredient interactions in complex foods: Aggregation and phase separation. In D. J. McClements (Ed.), Understanding and controlling the microstructure of complex foods (pp. 186-194). Wood head publishing.
- Tsumura, K., Saito, T., Kugimiya, W., & Inouye, K. (2004). Selective proteolysis of the glycinin and β‐conglycinin fractions in a soy protein isolate by pepsin and papain with controlled pH and temperature. Journal of Food Science, 69(5), C363–C367. https://doi.org/10.1111/j.1365-2621.2004.tb10698.x
- Ueno, H. M., Ueda, N., Morita, M., Kakehi, Y., & Kobayashi, T. (2012). Thermal stability of the iron–lactoferrin complex in aqueous solution is improved by soluble soybean polysaccharide. Food Biophysics, 7(3), 183–189. https://doi.org/10.1007/s11483-012-9256-1
- Yang, X., Tian, H., Ho, C. -T., & Huang, Q. (2011a). Inhibition of citral degradation by oil-in water nano emulsions combined with antioxidants. Journal of Agricultural and Food Chemistry, 59(11),6113–6119. doi:https://doi.org/10.1021/jf2012375
- Yang, X., Tian, H., Ho, C.-T., & Huang, Q. (2011b). Stability of citral in emulsions coated with cationic biopolymer layers. Stability of citral in emulsions coated with cationic biopolymer layers. Journal of Agricultural and Food Chemistry, 60(1), 402–409. https://doi.org/10.1021/jf203847b
- Yang, Y., Cui, S., Gong, J., Guo, Q., Wang, Q., & Hua, Y. (2014). Enhanced stability of citral loaded emulsions prepared with a soy protein–polysaccharides Maillard reaction product. Food Hydrocolloids. Unpublished results.
- Yang, Y., Cui, S., Gong, J., Miller, S. S., Wang, Q., & Hua, Y. (2015a). Stability of citral in oil-in-water emulsions protected by a soy protein-polysaccharide Maillard reaction product. Food Research International, 69, 357–363. https://doi.org/10.1016/j.foodres.2015.01.006
- Yang, Y., Cui, S. W., Gong, J., Guo, Q., Wang, Q., & Hua, Y. (2015). A soy protein-polysaccharides Maillard reaction product enhanced the physical stability of oil-in-water emulsions containing citral. Food Hydrocolloids, 48, 155–164. https://doi.org/10.1016/j.foodhyd.2015.02.004
- Zhao, Q., Ho, C. T., & Hung, Q. (2013). Effect of ubiquinol-10 on citral stability and off-flavor formation in O/W nano-emulsions. Journal of Agricultural and Food Chemistry, 61(31), 7462–7469. https://doi.org/10.1021/jf4017527