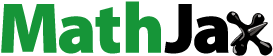
Abstract
Baby carrots were pasteurized under different time-temperature intervals and analyzed for physical and sensory characteristics. Treatments were conducted at 65, 70, 75, 80, 85 and 99°C and analyzed for kinetics of color and texture. Cell damage and shrinkage were analyzed by Field Emission Scanning Electron Microscopy (FESEM) at 70, 80 and 90°C. When temperature and time rise, L*, a*, b*, firmness and energy values decreased. The activation energy (Ea) for color and texture varied between 82.02–106.43 and 73.54–110.08 kJmol−1, respectively. Furthermore, carrots were pasteurized under different time-temperature combinations and showed a difference in cell density in comparison to fresh. Therefore, the pasteurization process consisting of high-temperature technology for a short time of carrots was considered best in terms of quality characteristics in comparison to low-temperature long time.
1. Introduction
Carrot (Daucus carota L.) is a rich source of βeta carotene (vitamin A) along with good amounts of dietary fiber, vitamin (K, C), minerals (iron and potassium; Gonçalves et al., Citation2010; Nagraj et al., Citation2020; Singh et al., Citation2021). Carrot is commonly consumed raw but frozen, dehydrated and with slight thermal processing to achieve consumers’ acceptability, especially in juices or purees products (López-Gámez et al., Citation2021; USDA, United State Department of Agriculture, Citation2012). It is a versatile ingredient in culinary uses and contains many healthy components including phytonutrients, dietary fibers and minerals. Furthermore, quality losses reduce its demand as fresh vegetables and carrots are highly disposed to moisture loss during refrigerated storage and changes in color, texture, flavor and aroma from heating or refrigeration (Zavadlav et al., Citation2020). Regardless of changes in quality from fresh, having a longer shelf life, high nutritional value, fresh like physical proprieties remain important, and consumers are willing to pay more for convenience but require that these items have high nutritional stability and sensory quality (Gonçalves et al., Citation2010; Liu et al., Citation2022).
Food pasteurization and thermal pre-treatment such as blanching are conducted before freezing to inactivate bacteria and enzymes removed to entrap air from vegetable foods (Liu et al., Citation2021; Verghese et al., Citation2021). The significance of thermal processing is to target harmful microbes that are responsible for various diseases in humans, but by applying thermal processing quality of fresh vegetables including carrots is compromised resulting in loss of color, flavor and crunchy texture and most important nutrient loss, not only because of heat but also due to nutrient loss by leaching (Song et al., Citation2003). In addition, the major concern for consumers has processed food with all its nutritional benefits and quality retained (Kong et al., Citation2007; Połata et al., Citation2009; Saeed et al., Citation2021).
The color of vegetables is the first visual representation of the quality of that vegetable as it is representing surface and subsurface freshness. As the quality of vegetables is primarily assessed by the freshness in color; thus, it plays an essential role in concerning the overall acceptance of vegetables (Demırhan & Özbek, Citation2011; Nisha et al., Citation2011). To predict the quality of a vegetable based on its color, instrumental color measurement is used as an indication of quality. Tristimulus colorimetry is an instrumental method that provides rapid and easy access to the visual quality of vegetables (Ahmed et al., Citation2002). Specific terms are commonly used to represent color; these terms include L for “brightness” a for “green-red” and b for “blue-yellow”. For some time, considering the nature of food’s pigment, the combination of these parameters/terms is used (Garza et al., Citation1999; Gunawan & Barringer, Citation2000; Nisha et al., Citation2004). Parameters that are taken from Hunter L, a, and b values define the total color change (ΔE). Chroma represents color concentration, and it is correlated with the intensity of color. For the specification of color in food products, a Hue angle is usually used, an angle of 0 or 360° is to indicate a red hue, an angle of 90 represents yellow hues, an angle of 180 for green hues and 270° indicates blue hues. Browning index (BI) is linked to thermal processes that involve enzymes or oxidative browning (Alipoorfard et al., Citation2020). BI is a factor that changes with pasteurization temperature, being low at lower pasteurization temperature and higher as pasteurization temperature increases. Color parameters such as greenness are also affected by pasteurization temperature and appear to be tied to mechanical damage and cell membrane leakage.
Besides color, textural properties change with heating with alteration occurring in the physicochemical properties of the cell wall research have been conducted to understand the response of blanching to texture deterioration of carrots (Lo et al., Citation2002). Thermal softening in carrots is because of the pectin solubility that occurs due to depolymerization (Wang et al., Citation2021). Tissue firmness is deprived quickly within a few minutes, when carrots are subjected to thermal processing (90°C), followed by slow degradation during the remaining processing time. This cyclic pattern of texture loss is because of two different processes leading to loss of cellular stiffness and cell wall strength (Greve et al., Citation1994).
The objective of this study was to evaluate the kinetics of quality changes in baby carrot (Daucus carota L.) specifically color and texture during pasteurization over a range of temperatures (65–90 °C) for the same total lethality to define an optimal pasteurization process that would result in the greatest quality retention in baby carrots for further product development.
2. Material and methods
2.1. Materials
Baby carrots (packaged fresh) were purchased from a local market. These plastic packages were provided to the National Institute of Food Science & Technology (NIFSAT), where it was used for 1 day with a storage temperature of 4°C. Carrots of the same size, shape and color were selected.
2.2. Sample preparation and thermal treatment
Carrots were washed and cut into slab shape slices (22 mm length, 14 mm width), using a hand slicer. Carrots were subjected to 6 different temperatures (taken within the range of 65–90°C) with varied time lengths for thermal processing (). Taking 3 replicates, each experiment was performed 3 times (N = 3). Treatments were designed in a way to make norovirus and vegetative bacteria such as Listeria monocytogenes inactive by providing an estimated 5–6 log inactivation (Buckow et al., Citation2008; Gaze et al., Citation2006; Gibson & Schwab, Citation2011).
Table 1. Experimental conditions
The method used by Kong et al. (Citation2007), for heating baby carrots. Summarily, a single sample (22 ± 1 length, 14 ± 1 width) was hermetically packed into a custom-built cylindrical aluminum test cell having a 35-millimeter inner dia, 66-millimeter inner height and 2-millimeter wall thickness. The time required by carrots’ centered temperature to reach within 1°C of the total temperature rise, called come up time, was determined using a 0.1 mm thick dia copper-constant thermocouple (Type T) added through the rubber gland into the lid of the container. The immersion length of the probe was 3 mm; thus, the influence of heat conduction along the thin wire probe to the sample temperature measurement was considered to be minimal. Distilled water was added to the test cells to cover the sample, after that cells were heated in a water bath at particular temperatures followed by sealing (Model HAAKE W13, Thermo Electron Corp., Karlsruhe, Germany), by taking ethylene glycol as a medium for thermal processing. After heating, carrots were placed into ice water to cool instantly. After that, samples were pressed softly between filter paper to dry them and then readings were taken.
2.3. Color evaluation
The color of carrot samples was measured with the use of Hunter colorimeter (CM-2002, MINOLTA, Osaka, Japan). This system includes descriptive values (L*, a*, b*) that represent the location where the color falls within three-dimensional visible color space. The colorimeter was made against standardized white reference tile. Value for the intensity of light, L*, indicates the intensity of darkness in the sample (value varying from 0–100, minimum value for white color and maximum for black color), a* is the indicator of the intensity of green and red colors (a value ranging—green to +red), and b* indicate intensity of blue and yellow color [value ranging from—(blue) to + (yellow)]. Samples were taken in a Petri dish (9 n), and three readings of color for each sample were taken.
2.4. Texture evaluation
For textural analysis, only one puncture measurement was taken of every sample. A single puncture probe (5 mm diameter) was used for force-distance curves, while the texture was analyzed by firmness and energy. The probe was fitted in a Texture Analyzer TA-XT2 (Stable Micro Systems Ltd., Surrey, U.K.) linked to a load cell (5 kg). Before taking readings, the raw and thermally processed carrots were placed to stabilize at room temperature (approximately 20 °C), for this 30 min were taken. The carrots were then kept on the support base to make the probe perpendicular to the samples. The probe was set at a traveling speed of 1 mm/s. The force-distance graphs were taken by a computer and analyzed with the Texture Expert for Windows (version 1.15, Stable Micro Systems Ltd.). Samples were placed in small plastic plates (9 replicates) and textural measurements were done in triplicate to minimize biological variations.
2.5. Sensory evaluation
Consumer Sensory Analysis
The analysis was approved for a person’s participation by the National Institute of Food Science & Technology (NIFSAT) Institutional Review Board. A consumer evaluation panel consisting of 60 panelists, selected from the National Institute of Food Science & Technology (NIFSAT) and it’s around. The evaluation was performed in the Sensory Evaluation Facility at the National Institute of Food Science & Technology (NIFSAT). Before the test, all participants signed an informed consent form, and they were given a small non-monetary inducement as a reward for their participation. Demographic information was collected with a questionnaire presented in Appendix A. A complete random block design was implemented with samples receiving 3-digit codes and presented monadically . Carrots were cut into a slab shape slices, heat treated at 70°C (43 min), 80°C (18 min), and 90 °C (12 min) and served in covered Dixie cups (2 oz). Product attributes were divided into four categories including color, texture, flavor and overall acceptance. A 9-hedonic scale (1 for “dislike extremely”, 9 for “like extremely”) was used to analyze these attributes. Water and unsalted crackers were used to clean the palate between samples to avoid carry-over and fatigue as much as possible. The sensory session was taken in separate booths beneath natural, white light equipped with a computerized system and sensory software (Compusense® 5 software, release 5.2; Guelph, ON, Canada).
2.6. Field emission scanning electron microscopy (FESEM)
Cellular destruction caused by thermal processing was studied by the use of FESEM at different temperatures of 70, 80, and 90°C (N = 3). The visible distinction in cell dimension of micrographs for fresh carrots compared to thermally processed carrots was examined. Carrot samples after being thermally processed were stored for 2 h at a low-temperature 4°C. The samples were cut, fixed on tabs and examined by FESEM (Quanta 200 F, FEI Company, Hillsboro, Oregon) with a high voltage of 20KV. Micrographs having transverse section’s magnification 500X were taken.
2.7. Data analysis
Usually, the rate of reaction for quality loss can be presented as follows if the temperature provided is uniform (constant) by following Saxena et al. (Citation2012) method.
Where “k” is the rate constant, “C” is the quantitative indicator of a quality attribute at time t and n is the order of a reaction. Quality data were assessed to find the best empirical relationship, using zero-, first- or second order kinetic models as EquationEq. (2)(2)
(2) —(Equation4
(4)
(4) ):
Where C0 is the initial value (T = 0), Ct is value of color (T = t) and k is the constant here referred to as the rate constant. K (Degradation rate constant) for a specified temperature was calculated by using Arrhenius equation:
where “Ea” is the energy of activation for the reaction taken in (kJ mol−1), “R” is universal gas constant having a value of 8.315 J/mol.K, “T” is absolute temperature measured in kelvin, while k0 is frequency factor measured in per minute.
2.8. Statistical analysis
The quality attribute figures were analyzed by using ANOVA (analysis of variance) through SAS software. Least-significant difference (LSD) procedures were used to compare means. Means difference among treatments was calculated with Fisher’s least-significant difference method, with significance at the 5% level (p greater than 0.05). All the experimentation was carried out in triplicate.
3. Results and discussion
3.1. Color changes during pasteurization of carrot
The color changes in baby carrots during pasteurization at different temperatures over time are presented in Figure . CIE color values of raw carrots before processing were L* (56.08), a* (29.29) and b* (43.21) (N = 3). After processing as the temperature and time increased, the color of carrots got darker results with values being low for the L* -value and as its redness fades its a*-value gets low and due to decrease in yellowness b*-value also decreases. Heat can cause structural modification of carotenoid pigments, resulting in significant color changes in vegetables (Ahmed et al., Citation2002). As observed by Gonçalves et al. (Citation2010), there is a significant decrease in L*, a* and b* values as processing time and temperature increased in the blanching of carrots. Blanching decrease the cell’s opacity by changing its optical properties by providing blanching water in place of intercellular air after the release of cellular liquids as cell membranes got damaged (Su et al., Citation2020; Patras et al., Citation2011; Ahmed et al., Citation2002).
Figure 1. Carrot color degradation (L*, a* and b* parameters) during pasteurization process at different temperatures (65°C (○), 70°C (*), 75°C (◊), 80°C (∆), 85°C (□), 90°C (×)) (N = 3) .
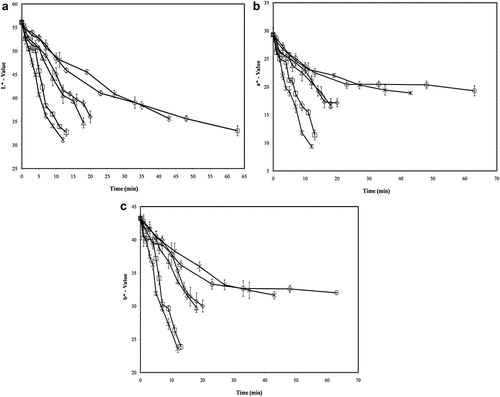
To explain the total color changes of carrot samples, the method by Feng and Tang (Citation1998) was used under mentioned equation to find the color difference (ΔE), ().
Where L0*, a0* and b0* were representing initial color values. The kinetic changes of L*, a*, b* and ΔE were determined. Mathematical models for color change of carrot show that a* and ΔE were best to be fitted in zero-order reaction, while L* and b* are of second-order reaction (Table ). Arrhenius equation was used to show the dependency of rate constants and to calculate the energy of activation values for L*, a*, b* and ΔE on temperature. The value of energy of activation for color deterioration differs between 82.02 and 106.43 kJ mol−1. These intermediate Ea values represent medium thermal sensitivity. Gonçalves et al. (Citation2010) observed high activation energy values (186 to 231 kJ mol−1), which showed larger heat sensitivity for color changes in their studies of carrots. However, some carotenoids containing food materials, such as onion paste (Ahmed & Shivhare Citation2001), mango puree (Ahmed, Shivhare, Kaur et al., Citation2002), red chili puree and paste (Ahmed, Shivhare, Kaur et al., Citation2002) and green chili puree (Ahmed et al., Citation2000), were observed to have lower activation energy values (11.4 to 36.8 kJ mol−1), which means these vegetables are less sensitive to heat than carrot.
Table 2. Kinetic parameters at different temperatures as zero-order and second-order models for L*, a*, b*, ∆E, firmness and energy for carrot treated under pasteurization conditions
The color degradation of carrot under the more extreme conditions of time and temperature (65°C-63 min, and 90°C-12 min) tested here shows the following loss, relative to raw carrot: L* 31% and 45%, a* 34% and 68%, and b* 31% and 45%, respectively. These percentage parameters confirmed the results obtained above and showed that the higher temperature and shorter time pasteurization (HTST) has more effects on the color degradation of carrots.
3.2. Texture degradation during pasteurization of carrot
The initial texture measurement values for firmness and energy (±SD) of non-pasteurized samples (control group) were 51.02 and 131.03, respectively (N = 3), which rapidly decreased as processing temperature and time increased. The curve representing texture deterioration of carrot, stiffness and energy, at various temperatures, 65–90°C, are presented in Figure , as observed by (Gonçalves et al., Citation2010). As the temperature and time of processing increase, the firmness of carrots decreases in the blanching of carrots. Our results for texture behavior are also consistent with the results found in carrots (BOURNE, Citation1987).
Figure 2. Pasteurization temperature (65°C (○), 70°C (*), 75°C (◊), 80°C (∆), 85°C (□), 90°C (×)) and time effects on carrot texture (firmness and energy) parameters (N = 3) .
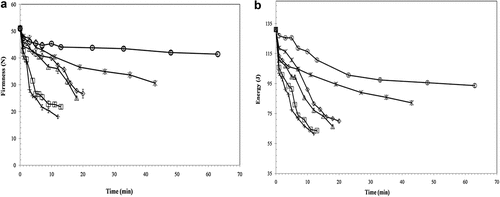
The kinetics of the firmness and energy parameters were evaluated. A second-order kinetic model was applied to fit the firmness energy curves. The results for the rate constants (k), R2, and activation energies (Ea) are presented in Table . The resulted values of R2 were 0.91 and 0.95 for firmness and energy, respectively. The Ea value for texture degradation as firmness is 110.08 kJ mol−1, and it is in the range of values mentioned in the literature. Vu et al. (Citation2004) observed the activation energies in the same range (92–118 kJ mol−1) but at higher temperatures (90–120°C and 80–110°C, respectively), and fitted first order and fractional conversion models. These intermediate Ea values indicated medium heat sensitivity. However, Gonçalves et al. (Citation2010) showed higher Ea value (152 kJ mol−1) at the same temperature (70–90°C) but different time combinations which showed larger heat sensitivity for textural degradation of carrot.
The texture degradation of carrot at the higher time and temperature (65°C-63 min) and 90°C-12 min) pasteurization treatments shows a loss in firmness and energy compared to raw carrot: firmness 19% and 65% and energy 29% and 53% and suggests that higher temperature shorter time pasteurization (HTST) have a greater effect on texture during pasteurization than a lower temperature treatment of similar lethality.
3.3. Effect of heat treatments on carrot cell morphology
The compact structure of tissues in fresh carrots results because of swollen cells packed together forming cell-to-cell strong and close contact throughout (Figure ). This solute aggregation phenomenon is due to the gathering of soluble solutes during sample preparation and processing. This kind of phenomenon is seen as a network of cells of varying sizes that depend on the amount of solutes: the higher the quantity of solutes in the sample, the smaller the cells will be (Llorca et al., Citation2005; Sanjuán et al., Citation2005).
Figure 3. FESEM graphs of non-heated and heated (1, 2, 3, 4) carrot samples, (a) Control, (b) 70°C for 43 min, (c) 80°C for 18 min and (d) 90°C for 12 min.
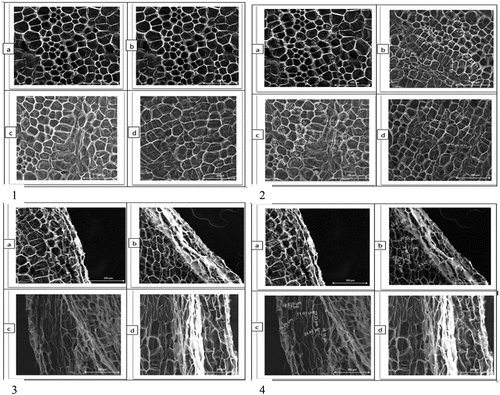
The micrographs of the samples pasteurized in water under various time-temperature graphs are shown in Figure . The solute aggregation phenomenon of these samples indicates the density of different sizes of the cells as compared to that of fresh one, showing the change in solid and water content as induced by heat treatment. Particularly for samples treated at high temperature (90°C) for 12 min, the heat treatment move plasmalemma and the tonoplasts to cell center. Twisting of the corner of cell walls was also noticed in samples. This could be because of solubilizations of solid from the cell wall (FUCHIGAMI et al., Citation1995).
3.4. Sensory analysis of pasteurized carrots
The sensory characteristics (Color, Appearance, Texture, Flavor and Overall acceptability) of the pasteurized carrots at various time-temperature combinations are described in Table . The results show that high-temperature for short time pasteurization processes was found better by the panelists for all sensory attributes. A significant difference was observed between 85°C, 90°C with 65°C, 70°C and 75°C for the color and appearance, while 65°C, 70°C and 75°C were non-significantly different. Similarly, in terms of overall acceptability the pasteurization of carrots at 80° C, 85°C and 90°C got 2.80, 2.80 and 3.80 score from the panelists, respectively.
Table 3. Effect of pasteurization temperature and time effects on carrot sensory attributes (N = 3)
High temperature for short time pasteurization process of carrots was considered best in terms of quality characteristics in comparison to low-temperature long time. The color, texture and sensory results showed a significant reduction by increasing time at low temperature. Similarly, the carrot pasteurized in water under various time-temperature indicates the density of cells depending on their size bigger as compared to the density of fresh, showing a change in their solid and water content as induced by heat treatment. While considering the sensory analysis the panelists also chose the HTST pasteurization process as more reliable for color, appearance, texture, and flavor. The mathematical models and/or equations allow food engineers to predict the thermal properties and save time, material and cost.
4. Conclusion
High-temperature for short time pasteurization process of carrots was considered best in terms of quality characteristics in comparison to low-temperature long time. The color, texture and sensory results showed a significant reduction by increasing time at low temperature. Conclusively, the pasteurization process consisting of high-temperature technology for a short time of carrots was considered best in terms of quality characteristics in comparison to low-temperature for long time.
Declaration
The work described has not been published before (except in the form of an abstract, a published lecture or academic thesis). It is not under consideration for publication elsewhere.
Disclosure statement
No potential conflict of interest was reported by the author(s).
Additional information
Funding
References
- Ahmed, J., & Shivhare, U. S. (2001). Thermal kinetics of colour degradation and storage characteristics of onion paste. LWT-Food Science and Technology, 34(6), 380–12. https://doi.org/10.1006/fstl.2001.0771
- Ahmed, J., Shivhare, U. S., & Kaur, M. (2002). Thermal colour degradation kinetics of Mango puree. International Journal of Food Properties, 5(2), 359–366(a). https://doi.org/10.1081/JFP-120005791
- Ahmed, J., Shivhare, U. S., & Raghavan, G. S. V. (2000). Rheological characteristics and kinetics of colour degradation of green chilli puree. Journal of Food Engineering, 44(4), 239–244. https://doi.org/10.1016/S0260-8774(00)00034-0
- Ahmed, J., Shivhare, U. S., & Ramaswamy, H. S. (2002). A fraction conversion kinetic model for thermal degradation of color in red chilli puree and paste. LWT-Food Science and Technology, 35(6), 497–503. https://doi.org/10.1006/fstl.2002.0897
- Alipoorfard, F., Jouki, M., & Tavakolipour, H. (2020). Application of sodium chloride and quince seed gum pretreatments to prevent enzymatic browning, loss of texture and antioxidant activity of freeze dried pear slices. Journal of Food Science and Technology, 57(9), 3165–3175. https://doi.org/10.1007/s13197-020-04265-0
- BOURNE, M. C. (1987). Effect of blanch temperature on kinetics of thermal softening of carrots and green beans. Journal of Food Science, 52(3), 667–668. https://doi.org/10.1111/j.1365-2621.1987.tb06699.x
- Buckow, R., Isbarn, S., Knorr, D., Heinz, V., & Lehmacher, A. (2008). Predictive model for inactivation of feline calicivirus, a norovirus surrogate, by heat and high hydrostatic pressure. Applied and Environmental Microbiology, 74(4), 1030–1038. https://doi.org/10.1128/AEM.01784-07
- Demırhan, E., & Özbek, B. (2011). Color change kinetics of celery leaves undergoing microwave heating. Chemical Engineering Communications, 198(10), 1189–1205. https://doi.org/10.1080/00986445.2010.525106
- Feng, H., & Tang, J. (1998). Microwave finish drying of diced apples in a spouted bed. Journal of Food Science, 63(4), 679–683. https://doi.org/10.1111/j.1365-2621.1998.tb15811.x
- FUCHIGAMI, M., Hyakumoto, N., & Miyazaki, K. (1995). Programmed freezing affects texture, pectic composition and electron microscopic structures of carrots. Journal of Food Science, 60(1), 137–141. https://doi.org/10.1111/j.1365-2621.1995.tb05623.x
- Garza, S., Ibarz, A., Pagan, J., & Giner, J. (1999). Non-enzymatic browning in peach puree during heating. Food Research International, 32(5), 335–343. https://doi.org/10.1016/S0963-9969(99)00094-0
- Gaze, J. E., Boyd, A. R., & Shaw, H. L. (2006). Heat inactivation of Listeria monocytogenes Scott A on potato surfaces. Journal of Food Engineering, 76(1), 27–31. https://doi.org/10.1016/j.jfoodeng.2005.05.035
- Gibson, K. E., & Schwab, K. J. (2011). Thermal inactivation of human norovirus surrogates. Food and Environmental Virology, 3(2), 74–77. https://doi.org/10.1007/s12560-011-9059-4
- Gonçalves, E. M., Pinheiro, J., Abreu, M., Brandão, T. R., & Silva, C. L. (2010). Carrot (Daucus carota L.) peroxidase inactivation, phenolic content and physical changes kinetics due to blanching. Journal of Food Engineering, 97(4), 574–581. https://doi.org/10.1016/j.jfoodeng.2009.12.005
- Greve, L. C., Shackel, K. A., Ahmadi, H., McArdle, R. N., Gohlke, J. R., & Labavitch, J. M. (1994). Impact of heating on carrot firmness: Contribution of cellular turgor. Journal of Agricultural and Food Chemistry, 42(12), 2896–2899. https://doi.org/10.1021/jf00048a047
- Gunawan, M. I., & Barringer, S. A. (2000). Green color degradation of blanched broccoli (Brassica oleracea) due to acid and microbial growth. Journal of Food Processing and Preservation, 24(3), 253–263. https://doi.org/10.1111/j.1745-4549.2000.tb00417.x
- Kong, F., Tang, J., Rasco, B., & Crapo, C. (2007). Kinetics of salmon quality changes during thermal processing. Journal of Food Engineering, 83(4), 510–520. https://doi.org/10.1016/j.jfoodeng.2007.04.002
- Liu, Y. Y., Sun, W. H., Li, B. Z., Wang, Y., Lv, W. Q., Shang, N., Wang, Y., Li, D., & Wang, L. J. (2022). Dehydration characteristics and evolution of physicochemical properties of Platycodon grandiflorum (Jacq. A. DC.) roots (PGR) during pulse-spouted microwave vacuum drying (PSMVD). Industrial Crops and Products, 177, 114449. https://doi.org/10.1016/j.indcrop.2021.114449
- Liu, Y. Y., Wang, Y., Lv, W. Q., Li, D., & Wang, L. J. (2021). Freeze-thaw and ultrasound pretreatment before microwave combined drying affects drying kinetics, cell structure and quality parameters of Platycodon grandiflorum. Industrial Crops and Products, 164, 113391. https://doi.org/10.1016/j.indcrop.2021.113391
- Llorca, E., Hernando, I., Pérez-Munuera, I., Quiles, A., Larrea, V., Fiszman, S. M., & Lluch, M. Á. (2005). Microstructural study of frozen batter-coated squid rings prepared by an innovative process without a pre-frying step. Food Hydrocolloids, 19(2), 297–302. https://doi.org/10.1016/j.foodhyd.2004.07.002
- Lo, C. M., Grün, I. U., Taylor, T. A., Kramer, H., & Fernando, L. N. (2002). Blanching effects on the chemical composition and the cellular distribution of pectins in carrots. Journal of Food Science, 67(9), 3321–3328. https://doi.org/10.1111/j.1365-2621.2002.tb09586.x
- López-Gámez, G., Elez-Martínez, P., Martín-Belloso, O., & Soliva-Fortuny, R. (2021). Pulsed electric field treatment strategies to increase bioaccessibility of phenolic and carotenoid compounds in oil-added carrot purees. Food Chemistry, 364, 130377. https://doi.org/10.1016/j.foodchem.2021.130377
- Nagraj, G. S., Jaiswal, S., Harper, N., & Jaiswal, A. K. (2020). Carrot. Nutritional Composition and Antioxidant Properties of Fruits and Vegetables, 323–337. https://doi.org/10.1016/B978-0-12-812780-3.00020-9
- Nisha, P., Singhal, R. S., & Pandit, A. B. (2004). A study on the degradation kinetics of visual green colour in spinach (Spinacea oleracea L.) and the effect of salt therein. Journal of Food Engineering, 64(1), 135–142. https://doi.org/10.1016/j.jfoodeng.2003.09.021
- Nisha, P., Singhal, R. S., & Pandit, A. B. (2011). Kinetic modelling of colour degradation in tomato puree (Lycopersicon esculentum L.). Food and Bioprocess Technology, 4(5), 781–787. https://doi.org/10.1007/s11947-009-0300-1
- Patras, A., Brunton, N. P., Tiwari, B. K., & Butler, F. (2011). Stability and degradation kinetics of bioactive compounds and colour in strawberry jam during storage. Food and Bioprocess Technology, 4(7), 1245–1252. https://doi.org/10.1007/s11947-009-0226-7
- Połata, H., Wilińska, A., Bryjak, J., & Polakovič, M. (2009). Thermal inactivation kinetics of vegetable peroxidases. Journal of Food Engineering, 91(3), 387–391. https://doi.org/10.1016/j.jfoodeng.2008.09.017
- Saeed, F., Afzaal, M., Hussain, M., & Tufail, T. (2021). Advances in assessing product quality. In Eds Charis M. Galanakis. Food losses, sustainable postharvest and food technologies (pp. 191–218). Academic Press.
- Sanjuán, N., Hernando, I., Lluch, M. A., & Mulet, A. (2005). Effects of low temperature blanching on texture, microstructure and rehydration capacity of carrots. Journal of the Science of Food and Agriculture, 85(12), 2071–2076. https://doi.org/10.1002/jsfa.2224
- Saxena, A., Maity, T., Raju, P. S., & Bawa, A. S. (2012). Degradation kinetics of colour and total carotenoids in jackfruit (Artocarpus heterophyllus) bulb slices during hot air drying. Food and Bioprocess Technology, 5(2), 672–679. https://doi.org/10.1007/s11947-010-0409-2
- Singh, M. N., Srivastava, R., & Yadav, I. (2021). Study of different varieties of carrot and its benefits for human health: A review. J Pharmacogn Phytochem, 10(1), 1293–1299. https://doi.org/10.22271/phyto.2021.v10.i1r.13529
- song, J. Y., An, G. H., & Kim, C. J. (2003). Color, texture, nutrient contents, and sensory values of vegetable soybeans [Glycine max (L.) Merrill] as affected by blanching. Food Chemistry, 83(1), 69–74. https://doi.org/10.1016/S0308-8146(03)00049-9
- Su, D., Lv, W., Wang, Y., Wang, L., & Li, D. (2020). Influence of microwave hot-air flow rolling dry-blanching on microstructure, water migration and quality of pleurotus eryngii during hot-air drying. Food Control, 114, 107228. https://doi.org/10.1016/j.foodcont.2020.107228
- USDA, United State Department of Agriculture. (2012). Carrot (Daucus carota L.). 114. Vegetable Crops Research: Madison.
- Verghese, M., Willis, S., Boateng, J., Gomaa, A., & Kaur, R. (2021). Effect of food processing on antioxidant potential, availability, and bioavailability. Annual Review of Food Science and Technology, 12(1), 307–329. https://doi.org/10.1146/annurev-food-062420-105140
- Vu, T. S., Smout, C., Sila, D. N., LyNguyen, B., Van Loey, A. M. L., & Hendrickx, M. E. G. (2004). Effect of preheating on thermal degradation kinetics of carrot texture. Innovative Food Science & Emerging Technologies, 5(1), 37–44. https://doi.org/10.1016/j.ifset.2003.08.005
- Wang, H., Karim, M. A., Vidyarthi, S. K., Xie, L., Liu, Z. L., Gao, L., Zhang, J.-S., & Xiao, H. W. (2021). Vacuum-steam pulsed blanching (VSPB) softens texture and enhances drying rate of carrot by altering cellular structure, pectin polysaccharides and water state. Innovative Food Science & Emerging Technologies, 74, 102801. https://doi.org/10.1016/j.ifset.2021.102801
- Zavadlav, S., Blažić, M., Van de Velde, F., Vignatti, C., Fenoglio, C., Piagentini, A. M., Pirovani, M. E., Perotti, C. M., Bursać Kovačević, D., & Putnik, P. (2020). Sous-vide as a technique for preparing healthy and high-quality vegetable and seafood products. Foods, 9(11), 1537. https://doi.org/10.3390/foods9111537