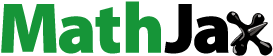
Abstract
Tomato, an important vegetable crop cultivated worldwide is an essential component of the Ghanaian diet. However, production in Ghana is hampered by heat stress (HS) during certain periods of the year. Tomato is sensitive to HS, especially during the reproductive stage, inducing pollen sterility, floral and fruit abortion, and culminating in low yield. This necessitates development of HS-tolerant tomato varieties. The HS-tolerance potential of 13 tomato lines was assessed under both poly-house and open field conditions at day temperatures of ~35°C. Evaluation was based on agro-morphological and physiological traits. Most lines had their highest fruit yield (FY) under poly-house conditions, but declined drastically (up to 92%) in the field when day/night temperatures reached 40/28°C respectively. A second field experiment revealed that the low FY was due to poor fruit set despite the many flowers produced. Correlations between days to 50% fruiting and physiological traits (ΦII, chlorophyll content, and leaf temperature) were high and significant, whereas those between FY and all physiological parameters were negligible. Lines KAC13 and KAU03 consistently had high FY, ΦII, and chlorophyll content. Nevertheless, KAU01 had the highest FY in two out of three experiments in addition to having the highest percentage fruit set. The lines that show high potential tolerance to HS may be incorporated into breeding programmes to further improve the tolerance of tomatoes to HS.
1. Introduction
Tomato is one of the most important vegetable crops in the world. This is due to its increasing commercial and dietary value, widespread production as well as its role as a model plant for research (Kimura & Sinha, Citation2008). The crop is consumed fresh or processed into various forms, such as paste, puree and juices. It is a rich source of vitamins (A and C), minerals (iron and phosphorus), lycopene, beta-carotene and contains high amount of water and low calories (Willcox et al., Citation2003). Shippers (Citation2000) emphasizes that tomato is the most important vegetable in Ghana. This opinion can be right with the continuing increase in the demand for both fresh and processed tomatoes coupled with the daily consumption of tomato in almost every household (Wolff, Citation1999). The high volumes of tomato produced locally (510,000 MT/Yr) as well as imported (570,000 MT/Yr) are indication of the importance of tomato in every Ghanaian meal (Melomey et al., Citation2019).
Current production levels, however, fall short of the country’s demand with average yields under 8 t/ha compared to the world average of 40 t/ha. The steady decline in production levels has been partly attributed to production seasonality and in particular high temperatures. During the dry season, very high day and night temperatures greater than 35°C and 29°C respectively can occur in Ghana (Kugblenu et al., Citation2013). Rick (Citation1978) estimated that temperatures above 32°C for more than 3 hours a day is sufficient to induce abortion of flowers in tomato. Such extreme temperatures cause a low local production of tomato during this period. Thus, tomato production during the dry season is not enough to meet the local demand. The high temperatures disrupt several physiological and biochemical processes leading to excessive flower drop, poor fruit set, and consequently, low fruit yield. The vegetative and reproductive stages of tomato are also adversely affected by high temperatures, resulting in a reduction in fruit quality and yield (Alsadon et al., Citation2006). High temperature increases root heat stress (HS) and affects nutritional quality because of a disturbed root nutrient relationship (Giri et al., Citation2017). High temperature thus limits root growth and reduces the shoot system and subsequent fruit production by limiting water supply, nutrients and the production of hormones that influence sink-source associations between roots and shoots (Wahid et al., Citation2007; Zinn et al., Citation2010). HS is therefore a significant cause of reduced tomato yield in most tropical countries (Mansour et al., Citation2009). High temperatures have been shown to affect not only the flowering and early fruiting stages, but also the later development and maturity of the fruit, resulting in reduced yields (Van Ploeg & Heuvelink, Citation2005). High temperature is the prevalent characteristic of subtropical and tropical regions and it has become an important limiting factor for tomato production and yield.
Although tomato exhibits anatomical, morphological, physiological and molecular responses to cope with HS, their reproductive stage and yields however, are extremely influenced by high temperatures. In Ghana, the bulk of tomatoes are produced in open fields. In recent years, poly-house-based tomato production has been increasingly popular as it is believed to minimize the risk of crop failure caused by biotic and abiotic stresses. However, production in such controlled environments requires resources, which increase production costs, especially when a micro-climate-controlled system is included. Thus, most crop production in polyethylene-roofed greenhouses of small- and medium-holder farmers do not have micro-climate controlling systems, and thus when the interior temperature of the poly-house increases during the day due to solar radiation, it causes HS, thereby decreasing yields.
Sensitivity to high temperature in most tomato cultivars presents a major limitation for growing an economic crop in regions where the temperature during periods of the growing season or even for short durations, reaches 38°C or higher (Stevens & Rudich, Citation1978). Progress in developing heat-tolerant cultivars has been hindered by the complexity of the trait and its low heritability (Villareal & Lai, Citation1979). High temperature has been observed to increase transpiration (Khanal, Citation2012; Li et al., Citation2015; Rivero et al., Citation2001) and reduce photosynthesis (Islam, Citation2011; Salvucci & Crafts-Brandner, Citation2004) by altering membrane fluidity and disrupting the overall balance of metabolic processes. It is argued that the breeding, screening and selection of tomato lines is more effective at the vegetative and fruit bearing stages (S. Z. Berry & Uddin, Citation1988). Pedigree selection and backcross hybridization have been used extensively to improve yield and fruit quality traits under HS conditions (Ibrahim, Citation2016). The unavailability of HS tolerant tomato varieties remains one of the main challenges for off-season commercial tomato cultivation in Ghana. Therefore, the identification of heat tolerant tomato varieties will provide a good starting point for future tomato breeding programs and also serve as a climate change adaptation strategy to combat increasing global temperature. Improving tolerance of tomato to HS can help to increase local production. Zhou et al. (Citation2015) reported that sensitivity to high temperature varies with genotypes. Hence, the objective of this study was to evaluate key physiological traits and fruit yield of different tomato lines grown under open field and poly-house conditions to determine their HS tolerance potential. We hypothesized that phenotypic variation among tomato lines in HS conditions can reveal the HS tolerance potential of the different lines.
2. Materials and methods
2.1. Poly-house experiment
This was conducted in the Poly-house facility of Horticulture Division at the Kwadaso station of CSIR-Crops Research Institute. Seeds of 12 tomato lines (Table ) were nursed in seed trays and transplanted on the ground in the poly-house in July, 2020. Lines were arranged in randomized complete block design with two replications. Although the poly-house was not climate-controlled automatically, the temperature in the poly-house could be modulated within a certain range by natural ventilation which involved rolling up or down plastic sheets on its sides. Therefore, the tomato lines were subjected to high temperatures (above 35°C) at both vegetative and reproductive stages to determine their heat tolerance at both the physiological and whole plant levels.
Table 1. List of tomato lines assembled and their source
2.2. Open field experiment
The field experiment was conducted in two seasons spanning a period of 2 years (2020/21 and 2021/22) at the Vegetable Garden (N 09° 23’ 55.7”, W 000 ° 59’ 40.6”) of CSIR-Savannah Agricultural Research Institute, Nyankpala, Tamale in the Guinea Savannah agro-ecological zone of Ghana. The weather conditions for these periods were as shown in Table . The first field experiment was carried out under drip irrigation between December 2020 and April 2021. Seedlings of the same 12 tomato lines were raised on heat sterilized beds and transplanted four weeks after nursing. The seedlings were transplanted at a spacing of 0.5 m x 0.5 m onto raised beds with plot dimensions of 6 m x 1 m giving a total of 24 seedlings per plot. Inorganic fertilizer was applied at the rate of 60–40-40 kg/ha N, P2O5 and K2O respectively. Agronomic practices such as weed control, mulching, irrigation, staking, diseases and insect pest control were carried out as and when necessary to ensure successful crop establishment. Tomato fruits were harvested when they were mature and red ripe.
Table 2. Weather conditions during the period of the experiment
The second field experiment was conducted between October 2021 and February 2022. Ten tomato lines (nine from the previous experiment and one new line) viz; KAU01, KAU03, KAI06, KAL10, KAC12, KAC13, WV009, WV017, WV020 and WV021 were nursed in October, 2021 on heat-sterilized raised beds. Seedlings were transplanted on 6 × 1 m raised bed plots at a spacing of 0.5 m x 0.5 m. The same agronomic practices from the 2020/21 season were followed.
Both field experiments were laid out in randomized complete block design with three (3) replications.
2.3. Data collection and analysis
In the poly-house, data collected included number of days to flowering, number of aborted flowers, number of locules per fruit, total soluble solids (TSS)/brix, fruit yield in addition to physiological attributes such as the operating and maximum efficiency of PSII in light adapted leaves, non-photochemical quenching (NPQ), relative chlorophyll content and leaf temperature. These physiological parameters were measured on the middle leaflet of the 4th to 5th node from the top of the canopy using the MultipseQ V1 linked to the PhotosynQ platform (www.photosynq.org; Kuhlgert et al., Citation2016). The MultispeQ protocol selected was the standard “Leaf photosynthesis V1” which simultaneously measured all the data (ΦII, NPQ(T), Fv’/Fm’, chlorophyll content and leaf temperature) within 20 s. Measurements were made on three tomato plants per line in each replicate between 10 AM and 3 PM during the fruiting stage. The brix was measured with a digital refractometer (MA871 Refractometer, Milwaukee Instruments Inc, USA).
In both field experiments, data were collected on plant height (cm) from the base of the plant to the highest growing tip, stem diameter (mm) using a vernier caliper, SPAD chlorophyll meter reading (SCMR) using the SPAD 502 plus chlorophyll meter (Spectrum Technologies, Inc., USA), days to 50% flowering and fruiting, days to maturity, fruit length (mm), fruit width (mm), fruit weight (g) and brix using a digital refractometer (MA871 Refractometer, Milwaukee Instruments Inc, USA). Physiological traits indicated above were also measured in the 2020/21 field experiment but not in the 2021/22 season. On the other hand, additional data such as number of flowers/plant, number of fruits/plant and percent fruit set (%FS) were assessed only in the 2021/2022 season.
Percent fruit set was calculated as, %FS =
Analysis of variance (ANOVA) was carried out in GenStat statistical software 19th edition (Payne, Citation2009) with mean separation done using the Fisher’s protected least significant difference (LSD) at 5% level of probability.
3. Results
3.1. Agronomic and fruit quality traits of tomato lines exposed to HS in a poly-house
In general, significant (p < 0.001) differences were observed among the lines for all the traits except brix (Table ). KAU01 produced the highest number of flowers/plant (~61) but it was not significantly different from KAC13 (51). The lowest number of flowers/plant was also produced by WV015 but this was not significantly different from KAI-06, KAL10, and WV020. KAC13 (10.5) had the highest number of aborted flowers but this was not significantly different from KAC12 (7.8). WV009 (1.7) had the least number of aborted flowers but it was not significantly different from all others except KAC12 and KAC13. Tomato line KAU01 (37.5 t/ha) was the highest yielding line which was significantly different from KAC13 (32.7 t/ha) and WV006 (33.2 t/ha). KAC12 (6.8 ton/ha) was the line with significantly lowest yield (Table ). Brix ranged from 5.2 to 6.0, although differences between the lines with the highest brix (KAC13, WV009, and WV015) and all other lines were not significant. WV009 had a significantly greater number of locules per fruit (6) than all the lines except WV015 and WV020 (Table ).
Table 3. Mean agronomic and fruit quality traits of tomato lines under HS in the poly-house
3.2. Physiological parameters of tomato lines subjected to HS in the poly-house
The tomato lines exhibited variation in all the physiological parameters measured (Table ). Nevertheless, only differences between the lines for relative chlorophyll content were significant (p < 0.001). Tomato line WV020, had the highest photosynthetic efficiency (ΦII, 0.64), but it was not significantly different from the others (Table ). Fv’/Fm’, the maximum efficiency of PSII in light adapted leaves ranged from 0.77 to 0.78. Minor variations could also be seen in the leaf temperature of the tomato lines, with KAC12 (33.2°C) and KAU03 (31.5°C) having the highest and lowest leaf temperature respectively, although, the differences were not significant.
Table 4. Mean physiological parameters of tomato lines under HS in the poly-house
Non-photochemical quenching (NPQ) was assessed by the NPQ(T) parameter as described by Tietz and co-authors (Tietz et al., Citation2017). The tomato lines generally had low NPQ(T), ranging from 0.35 to 0.48. Tomato line WV020 had the lowest NPQ (0.43), although it was not significantly lower than the rest.
Relative chlorophyll content (RCC) is a measure of leaf greenness similar to the SPAD chlorophyll meter readings (SCMR; Kuhlgert et al., Citation2016). Under HS conditions in the poly-house, RCC ranged from 44.8 to 59.5. Tomato line WV015 had the highest RCC (59.5), but this was not significantly different from KAI-06, KAL10, WV006, and WV009. KAU03 had the least RCC (44.8) but it was not significantly different from WV020 (48.1).
3.3. Agro-morphological traits and yield of tomato lines exposed to HS in the field (2020/2021 season)
The agro-morphological characters and yield of the tomato lines under HS in the open field in Nyankpala during the 2020/21 season are presented in Table . The tomato lines varied significantly (p < 0.001 and p < 0.01) for all traits measured except fruit width and brix. The line with the highest value for plant height (PH) was KAC13 (49.3 cm) with WV021 (31.7 cm) being the shortest. Tomato line WV021 had the smallest stem diameter (5.9 mm), whereas the widest stem diameter was found in KAI-06 (9.2 mm), closely followed by WV015 (9.0 mm). The longest fruits were observed in KAI-06 and KAL10, whereas KAU01 had the shortest fruits. Line KAU02 (50.7 days) took fewer number of days to 50% flowering (D50F), whereas lines KAC12, KAC13, WV009, WV015 and WV020 exhibited longer D50F. Line KAU03 (62.3 days) took fewer days to 50% fruiting (D50Fr) whereas WV020 had the highest value for D50Fr (84.0 days), both of which were significantly different from the rest. Line KAC13 (23.2 t/ha) had the highest fruit yield (FY), however, it was not significantly different from KAC12, KAU03, KAI-06 and KAL10 with yields of 18.8, 16.3, 15.8, and 15.5 t/ha respectively. On the other hand, WV006 (4.1 t/ha) and WV021 (3.9 t/ha) displayed the lowest FY. KAU01 had the highest brix (5.9), though this was not significantly different from all the others (Table ).
Table 5. Mean performance and fruit quality of tomato lines under HS field conditions (2020/21 season)
3.4. Physiological parameters of tomato lines exposed to heat stress under field conditions (2020/2021 season)
Unlike the poly-house experiment, significant differences (p < 0.001 and p < 0.05) could be observed among the tomato lines for all the physiological parameters assessed in the open field (Table ). Tomato line WV021 (0.45) had the highest photosynthetic efficiency (ΦII), but this was not significantly different from KAL10 (0.37), WV006 (0.39), and WV015 (0.42). The lowest ΦII was however, observed in KAU01. The tomato lines exhibited similar patterns for Fv’/Fm’ as seen for ΦII. WV015, KAL10, WV021, and WV006 in descending order had high Fv’/Fm’ values (0.68, 0.64, 0.63 and 0.62 respectively), whereas KAU01 had the least value of 0.47.
Table 6. Chlorophyll fluorescence-based photosynthetic parameters and leaf temperature of tomato lines exposed to HS in the open field
Although WV015 had the lowest NPQ(T) (1.34), it was not significantly different (p > 0.05) from all other tomato lines except KAU01, KAC13, WV009 and WV020 which in descending order had high NPQ(T) (Table ). On the other hand, WV006 and WV021 had significantly higher RCC (61.4) compared to KAU01 and KAU03 but were not significantly different from the rest. KAL10 had the lowest LT (33.4 °C) but this was only significantly different from KAC13, KAI-06, KAU01 and KAU03.
Instantaneous values of photosynthetically active radiation (PAR), ambient temperature and relative humidity measured in the poly-house and the field during measurement of physiological parameters are shown in Table . Temperatures and PAR in the field were mostly higher than that of the poly-house. On the other hand, relative humidity was higher in the poly-house than on the field.
Table 7. Environmental parameters in the field and poly-house during photosynthesis data collection
Results of correlation analyses among both physiological and agro-morphological traits are shown in Figure . In general, there were significant correlations between all the physiological parameters. The strongest negative correlation was between NPQ(T) and Fv’/Fm’ with an r2 value of (-)0.99. LT had the next strongest negative correlation with RCC. Correlation among agro-morphological traits and between agro-morphological and physiological traits varied widely in terms of strength and significance. Interestingly, SD was the only morphological trait that had a significant and strong correlation with FY. Phi2 (ΦII), RCC and LT had significant correlation with D50Fr, although leaf temperature was negatively correlated with D50Fr. None of the parameters had any significant or strong correlation with Brix and D50F.
Figure 1. Correlation and distribution plots of physiological and agro-morphological traits assessed during HS in the open field for the 2020/21 season. Phi2: ΦII; NPQ: non-photochemical quenching; RCC: relative chlorophyll content; LT: leaf temperature; PH: plant height; SD: stem diameter; D50F: days to 50% flowering; D50Fr: days to 50% fruiting; FL: fruit length; FW: fruit width; FY: fruit yield.
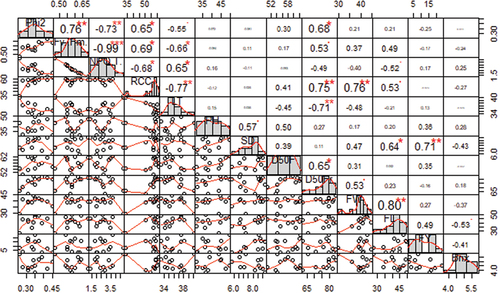
3.5. Agro-morphological traits and yield of tomato lines exposed to heat stress in the field (2021/22 season)
The results of analysis of variance (ANOVA) revealed significant (p < 0.05) differences among the tomato lines for SCMR, D50Fr, number of fruits/plant, FL, FW, mean fruit weight (MFW), and FY. However, there were no significant (p > 0.05) differences among the tomato lines for PH, SD, D50F, number of flowers/plant, and brix.
SCMR, a measure of leaf greenness, ranged from 49.3 to 63.0 with a mean of 55.1 (Table ). Line WV009 had plants with the highest SCMR value which was significantly greener than all the other lines except WV017 and WV020. D50Fr ranged from 68 to 82 days with a mean of 74 days after sowing. Line WV020 took 82 days to reach 50% fruiting and this was significantly longer than the D50Fr observed in lines KAU03 (68 days), KAI-06 (68 days), KAC13 (69 days), WV017 (71 days) and KAC12 (72 days). Line KAU03 produced the longest tomato fruits (34.71 mm) while line WV020 produced the shortest fruits (16.64 mm). FL recorded from line WV020 was significantly lower than the other lines except WV021 (17.74 mm), KAU01 (22.99 mm) and WV009 (22.01 mm). FW among the tomato lines ranged from 20.89 mm to 43.76 mm with a mean of 32.10 mm. Line WV017 produced the widest fruits (43.76 mm) which significantly differed from lines WV021 (20.89 mm), WV020 (24.22 mm), KAU01 (28.49 mm), WV009 (30.22 mm) and KAL10 (30.41 mm). MFW significantly varied among the tomato lines. It ranged from 10.71 g to 36.73 g with a mean of 27.90 g. Line WV009 produced plants with the highest MFW (36.73 g) which was significantly heavier than lines KAU01 and KAL10 with MFW of 15.32 g and 10.71 g respectively. Tomato FY ranged from 12.76 t/ha to 29.59 t/ha with a mean of 18.81 t/ha. Line KAU01 produced the highest FY which was significantly different from all the other lines except KAU03 and KAI06 with FY of 22.28 t/ha and 22.48 t/ha respectively (Table ).
Table 8. Growth and yield components of tomato lines evaluated under HS in the open field (2021/22 season)
Even though the number of flowers/plant among the lines was not significant, the number of fruits/plant was highly significant (p < 0.001). The number of fruits/plant ranged from 12 (WV009 and WV021) to 82 (KAU01) with a mean of 24 fruits/plant (Figure ). The number of fruits/plant produced by line KAU01 was significantly higher than all the other lines. Line KAU03 had the second highest number of fruits/plant and this was significantly higher than the rest of the lines. A similar trend was observed among the tomato lines for percent fruit set which is an important parameter for screening for HS tolerance in tomato (Figure ). Line KAU01 had the highest percentage fruit set (35%) followed by line KAU03 (25%) while WV009 had the lowest percentage fruit set (5.7%).
4. Discussion
In this study, 13 tomato lines were evaluated for their tolerance to HS in the poly-house and/or open field conditions using agro-morphological as well as physiological traits. The data revealed variation within the lines for all the traits assessed, although, the statistical significance of differences between the lines was trait- and environment-dependent. The temperatures experienced during the experiments were high enough to create a HS environment to assess the lines for their tolerance to HS. Rivero et al. (Citation2001) reported that heat stress in tomato occurs around 35 °C. There have been a number of studies assessing heat tolerance of different tomato lines using various morphological and physiological parameters (Abdul-Baki, Citation1991; Alsamir et al., Citation2017; Hameed et al., Citation2015; Zhou et al., Citation2015; Zhang et al., Citation2016). Our research expands on previous work by evaluating the tomato lines under poly-house and actual open field conditions in two seasons and including additional physiological parameters such as leaf temperature as a proxy for transpiration-induced cooling to aid in identification of HS tolerant lines.
4.1. Agro-morphological traits of tomato lines exposed to HS
Fruit yield has been a key trait of interest for many researchers seeking to evaluate different crops under HS conditions since it is the most desirable output for any farmer. There appeared to be an influence of the number of flowers per plant on FY in the poly-house since two out of the three top performing lines with respect to yield (KAU01 and KAC13) had the highest number of flowers per plant. In a study by Kugblenu et al. (Citation2013), lines that appeared to be tolerant to HS also produced abundant flowers per plant. However, high production of flowers alone is not a sufficient measure of HS tolerance since the flowers could be aborted before they form fruits. Loss of pollen viability induced by exposure of crops to HS prevents successful pollination and often leads to floral and fruit abortion (Bokszczanin, Citation2013; Prasad et al., Citation2017; Sita et al., Citation2017). Interestingly, one of the highest yielding tomato lines in this study, KAC13, had the highest number of aborted flowers. However, converting to percentage flower abortion revealed that its 20% aborted flowers was not the worst compared to some lines such as KAI-06 and WV015 which lost 47% and 39% of their flowers respectively. KAU01 had the least percentage of flowers lost (4.6%). Losing a smaller fraction of flowers under HS is indicative of tolerance to HS (Kugblenu et al., Citation2013; Saeed et al., Citation2007).
The variation in fruit quality traits such as brix was not significant, suggesting that, HS did not strongly influence the tomato lines in terms of this trait. Nevertheless, comparisons need to be made under non-HS conditions to be able to fully rule out any influence of HS on brix in these tomato lines.
In the open field experiment during the 2020/21 season, additional data such as PH, SD, D50F and D50Fr were recorded to better understand the performance of the tomato lines under HS. Fruit attributes were also assessed. There was significant variation (p ≤ 0.05) among the tomato lines for PH and SD with KAC13 being the tallest whereas KAI-06 had the widest stem. This is contrary to Zhou et al. (Citation2017) who found no significant difference between HS sensitive and tolerant lines under HS conditions. However, it should be noted that, the absolute value of PH and SD may not necessarily reveal the HS tolerance of a line unless it is being compared to values under non-HS conditions since the lines may have their inherent differences in these traits irrespective of HS. For example, Rajametov et al. (Citation2021) found that HS-sensitive tomato lines recorded declines in PH whereas tolerant lines recorded no change. Vegetative traits such as PH and SD have been considered not ideal for indirect selection due to many contrasting results reported in the literature (Lee et al., Citation2022). Furthermore, the correlation analyses revealed that there was no strong and significant relationship between PH and FY. Interestingly, there was a moderately strong positive correlation between SD and FY, suggesting some influence of stem diameter on HS tolerance based on FY.
The traits D50F and D50Fr are important traits that can reveal certain aspects of the mechanism of HS tolerance adapted by crops. Lines that have at least 50% of their plants flowering and fruiting early, show early maturation and exhibit the escape or avoidance mechanism such that they can produce fruits quickly before the onset of HS or before the stress becomes severe. KAU02 was the earliest to reach 50% flowering, however, it was KAU03 which first had 50% of its plants fruiting. Lines that had 50% of their plants flowering early did not necessarily have 50% of their plants fruiting early possibly due to differences in degree and/ or rate of floral and fruit abortion, although, these traits were not assessed during this season. Moreover, contrary to observations made by Kugblenu et al. (Citation2013), correlations between either D50F or D50Fr and FY were low and not significant. Based on the results, KAU01 and KAU03 can be considered as having potential HS escape traits. However, it is likely the HS escape trait may have a yield penalty if sowing is not timed right to enable the plant to fully avoid the HS. This could be the reason for the low FY produced by KAU01 during the 2020/21 season.
Our results support the widely accepted notion that fruit yield is strongly influenced by the environment. The tomato lines generally had higher yield under poly-house conditions but there was drastic decline in yield under the more stressful environmental conditions that existed in the open field. In the 2020/21 season where the temperatures were the highest, line WV021, KAU01, and WV006 had the highest declines of 79%, 79%, 92% in yield. Abdul-Baki (Citation1991) reported that tomato lines that were sensitive to HS had the greatest reduction in yield under higher temperature conditions in the greenhouse. On the other hand, KAC13 had the least decline in yield (29%), although KAC12, KAI-06 actually increased in yield and KAL10 recorded negligible change. These suggest that KAC12, KAI-06, KAL10 and KAC13 have potential tolerance to HS. However, care should be taken when comparing yields from protected culture and the open field since some tomato lines are well suited to either of the two environments than others independent of the temperature within the two environments. Therefore, a second open field experiment was necessary to resolve the HS tolerance of the tomato lines.
Although variation in FW was not significant, that of FL was significant and these two traits provide insights into the differences in yield observed. In general, KAU01 had the smallest FW and FL, and thus consequently the smallest fruit. If fruit size was not compensated for by number of fruits, this could have contributed to the low yield observed for this line. Interestingly, correlation analysis revealed that there was no strong and/ or significant relationship between either FW or FL and FY. This observation is similar to a report by Kugblenu et al. (Citation2013). It is possible that the number of fruits/plant and/ or total number of fruits for each line, although not recorded during this season, compensated for variation in FW and FL, at least for some lines, thereby strongly influencing yield as has been observed by other authors (Kugblenu et al., Citation2013; Lin et al., Citation2006).
In contrast to observations in the 2020/21 season, variations in PH, SD, and D50F during the 2021/22 season in the open field were not significant. This suggests that these three traits could not have influenced the yield and consequently the HS tolerance of the tomato lines to any appreciable degree.
The ability of plants to maintain green leaves in the face of environmental stress, often referred to as the stay green trait has been associated with tolerance to HS. Leaf greenness was assessed by the SCMR parameter during this season. There was significant variation in SCMR with WV009, having the highest value. Interestingly, tomato lines that were in the top rank for SCMR also had some of the lowest FY and vice versa. This suggests a negative correlation between SCMR and FY, although such an association was not observed in the previous season.
Significant variation was observed for FL, FW, MFW and FY. Interestingly, lines that had the smallest size by virtue of their low FL and FW (WV021 and WV020) were among the lines with the highest MFW. However, such high MFW did not culminate into higher yield since they were the lines with the lowest FY. This was likely due to the fact that they had the lowest fruit number as a consequence of having the lowest percent fruit set. Heat sensitive lines generally have lower percentage fruit set under high temperature conditions (Abdul-Baki, Citation1991; Alsamir et al., Citation2017). Therefore, lines that have low yield, especially resulting from low percentage fruit set were considered as heat sensitive. Another surprising observation was that, KAU01 which was one of two lines with the lowest MFW, had the highest FY. This could be attributed to the fact that it had the highest number of fruits/plant due to its ability to set more fruits per flowers produced. Such an observation has been made by other authors (Kugblenu et al., Citation2013). KAU03 and KAI-06 had the next highest FY, also resulting from a high percentage fruit set and number of fruits per plant.
4.2. Physiological responses of tomato lines exposed to HS
The photosynthetic parameter ΦII (F’q/F’m) measures the efficiency of photosystem II (PSII) in the light for photochemistry. In order words, it is an indication of the fraction of captured light energy used in the photochemical reactions of photosynthesis. Generally, lines that have high ΦII imply that they are efficiently utilizing light energy even under HS. The two parameters, ΦII and Fv’/Fm’, were among others described by Ayenan et al. (Citation2019) as important traits for breeding heat tolerant tomato varieties. It should be noted however, that in the poly-house, the differences between the highest and lowest performing lines for both traits were low (about 0.03) possibly due to the low light intensity (<500 µmol photons m−2s−1) within the poly-house, attributable to the shading net hung over the facility. These differences were not significant and it is possible that under field HS conditions, where the light intensity is high, variations will be significant.
Under HS in the open field all the physiological parameters measured varied widely with highly significant differences between the tomato lines. For ΦII and Fv’/Fm’, lines WV021 and WV015 had the highest values for these two parameters, suggesting that they were using the light energy efficiently despite the high temperature. On the other hand, KAU01, KAU02 and KAU03 had the lowest values for these two parameters. Having high Fv’/Fm’ also implies that these lines had mechanisms in place to protect against photodamage to the photosynthetic machinery, particularly the components involved in the light reaction. The Fv’/Fm’ parameter is closely related to Fv/Fm, a measure of the maximum efficiency of PSII in dark adapted plants, and have both been used to screen for tolerance to HS in tomato plants (Hameed et al., Citation2015), with the authors acknowledging that Fv’/Fm’ was better at foretelling sensitivity heat-shock. Further, lines that had the highest HS tolerance potential were found to have high ΦII and Fv/Fm (Arena et al., Citation2020). However, it should be noted that high ΦII and Fv’/Fm’ under HS may not always result in higher yield. This is because, as shown in Osei‐Bonsu et al. (Citation2021), electrons extracted in the light reactions could be diverted to photorespiration and other alternative electron acceptors and thus may not end up being used in the carbon fixation reactions. Another reason accounting for low and insignificant correlations between photosynthetic parameters and FY has to do with pollen viability. HS influences viability of pollen and this in turn affects successful pollination and fruit set, consequently affecting fruit yield (Alsamir et al., Citation2021; Bhandari et al., Citation2016; Sato et al., Citation2000). Thus, despite a high photosynthetic rate, most fruits formed will be aborted if the pollen is not viable.
Non-photochemical quenching (NPQ) generally represents a number of processes such as energy dependent quenching (qE) and photo-inhibitory quenching (qI) which quench excitation energy (Muller et al., Citation2001). NPQ reduces efficiency of photosynthesis but this loss could be photoprotective in nature if it is mostly due to qE, preventing permanent damage to PSII. However, excessive quenching (NPQ), especially if it is predominated by the qI component representing photoinhibition, as is mostly the case under environmental stresses (Murata et al., Citation2007; Takahashi & Murata, Citation2008), can lead to overall loss of productivity. In the poly-house experiment, NPQ values were generally low, implying minimal quenching which was likely due to the relatively low light during the period of measurement. This is consistent with the high maximal PSII efficiency in the light (Fv’/Fm’) observed for these lines. The tomato lines which showed the lowest NPQ also had the highest Fv’/Fm’.
The NPQ data from the field was in direct contrast to the values for Fv’/Fm’ with lines that had the highest Fv’/Fm’ in most cases having the lowest NPQ(T). Line KAU01 which had the lowest Fv’/Fm’ and ΦII had the highest NPQ(T) values, suggesting possible involvement of photo-inhibitory quenching, although this was not directly measured in this study. In Arena et al. (Citation2020), heat-sensitive lines were found to have high NPQ whereas those that were heat-tolerant in terms of their fruit yield also had low NPQ. Short-lived reductions in CO2 fixation results from specific changes in PSII conformation which are linked with high NPQ (Kromdijk et al., Citation2016).
Chlorophylls are pigments essential for photosynthesis. In general, the higher the chlorophyll content, the higher the rate of photosynthesis, although there may be exceptions to this rule where reduced chlorophyll content has little impact on photosynthesis (Walker et al., Citation2018). Several authors have found significantly high correlation between SPAD chlorophyll meter readings also referred as relative chlorophyll content and the total chlorophyll content (Kapotis et al., Citation2003; Kumagai et al., Citation2009). Indeed, Kumagai et al. (Citation2009) found a relatively high correlation between chlorophyll meter readings and Fv/Fm in rice. Interestingly, under poly-house conditions, the tomato line WV015 which had one of the lowest photosynthetic performance (measured by ΦII and Fv’/Fm’) had the highest relative chlorophyll content, although it was not significantly different from KAL10 which also had high ΦII. Nevertheless, care should be taken in interpreting the results of relative/ SPAD chlorophyll content under environmental stresses since some lines may naturally have higher chlorophyll content which may not be strongly influenced by HS. Thus, it is best to compare chlorophyll content under stress and non-stress conditions in order to eliminate inherent differences between the lines.
Under open field conditions clear and significant differences were observed between the tomato lines in terms of RCC. This suggests that unlike the poly-house experiment, conditions in the field were more stressful for the tomato lines thereby revealing their genetic potential. Decreased chlorophyll content was found to be associated with sensitivity to HS whereas HS tolerant tomato plants were found to maintain high chlorophyll content (R Zhou et al., Citation2017). Although KAU01 which had the lowest RCC also had the lowest yield, no strong or significant correlation could be observed between RCC and FY, suggesting that under these conditions, RCC was not a main driver of FY.
When plants are exposed to HS, they cool down their leaves through transpiration. Thus varieties with enhanced capacity for cooling will effectively maintain a lower leaf temperature even under hot conditions, thereby escaping the heat and protecting against any damage to photosynthetic machinery (Deva et al., Citation2020). This transpiration-induced cooling can be assessed indirectly by measuring the leaf temperature under HS, finding the difference between the leaf and air temperature or difference between canopy and air temperature, referred to as canopy temperature depression (Reynolds et al., Citation2001; Chaudhary et al., Citation2020). In the poly-house experiment, differences between the tomato lines for LT were not found to be significant. Leaf temperature influences the rate of many ecophyiological traits (Blonder & Michaletz, Citation2018). It is therefore not surprising that differences between photosynthetic parameters were also not significant.
In open field conditions, LT data showed significant variation between the tomato lines. This suggests that these lines had different capacity for transpiration-induced cooling, which is an essential trait for HS tolerance (Wahid et al., Citation2007) and key priority for development of HS tolerant crops (Prasad et al., Citation2017). Significant correlations were found between LT and other photosynthetic traits, suggesting that these traits were strongly influenced by the LT as generally known (J. A. Blonder & Michaletz, Citation2018; Joseph A Berry & Bjorkman, Citation1980). The warmest leaves were found in KAU01, corroborating results from the other traits and indicating that KAU01 could be sensitive to HS under more stressful conditions.
5. Conclusion
Significant genotypic variations were observed in physiological parameters such as ΦII, NPQ(T), chlorophyll content, and leaf temperature which could be attributed to differential responses of the tomato lines to HS during the reproductive stage of the crop. These traits can potentially be used to screen for HS tolerant tomato lines.
Lines KAC13 and KAU03 were consistently among lines with the highest fruit yield, photosynthetic efficiency, chlorophyll content, and moderate to high percent fruit set and can be considered as having high HS tolerance potential under most conditions. On the other hand, KAU01 and KAI-06 may be considered as having potential tolerance to HS under moderately high temperature conditions. Furthermore, KAL10 and KAC12 can be considered as potential moderately HS tolerant lines and the low performance of KAC12 and KAI-06 under poly-house conditions suggest they may not be suitable for poly-house cultivation.
Lines WV021 and WV015 had good physiological attributes such as high ΦII, Fv’/Fm’, chlorophyll content as well as low LT and NPQ(T), in addition to high fruit weight but were classified as sensitive to HS due to low FY and percent fruit set under HS.
Disclosure statement
No potential conflict of interest was reported by the authors.
Data availability statement
The data analyzed during the current study are available from the corresponding author on rational request.
Additional information
Funding
References
- Abdul-Baki, A. A. (1991). Tolerance of tomato cultivars and selected germplasm to heat stress. Journal of American Society for Horticultural Science, 116(6), 1113–17. https://doi.org/10.21273/JASHS.116.6.1113
- Alsadon, A. A., Wahb-Allah, M. A., & Khalil, S. O. (2006). In vitro evaluation of heat stress tolerance in some tomato cultivars. Agricultural Science, 19(1), 13–24 http://www.biocas-fao.com/wp-content/uploads/2016/10/Heat-Stress-Evaluation.pdf.
- Alsamir, M., Ahmad, N. M., Mahmood, T., & Trethowan, R. (2017). Morpho-physiological traits linked to high temperature stress tolerance in tomato (S. Lycopersicum L.). American Journal of Plant Sciences, 08(11), 2681–2694. https://doi.org/10.4236/ajps.2017.811180
- Alsamir, M., Mahmood, T., Trethowan, R., & Ahmad, N. (2021). An overview of heat stress in tomato (Solanum Lycopersicum L.). Saudi Journal of Biological Sciences, 28(3), 1654–1663. https://doi.org/10.1016/j.sjbs.2020.11.088
- Arena, C., Conti, S., Francesca, S., Melchionna, G., Hájek, J., Barták, M., Barone, A., & Manuela Rigano, M. (2020). Eco-physiological screening of different tomato genotypes in response to high temperatures: A combined field-to-laboratory approach. Plants, 9(4), 1–16 https://doi.org/10.3390/plants9040508.
- Ayenan, M. A. T., Danquah, A., Hanson, P., Ampomah-Dwamena, C., Ariel Kpedetin Sodedji, F., Asante, I. K., & Yirenkyi Danquah, E. (2019). Accelerating breeding for heat tolerance in tomato (Solanum Lycopersicum L.): An integrated approach. Agronomy, 9(11), 720. https://doi.org/10.3390/agronomy9110720
- Berry, J. A., & Bjorkman, O. (1980). Photosynthetic response and adaptation to temperature in higher plants In Higher Plants. Annual Review of Plant Physiology, 31(31), 491–543. https://doi.org/10.1146/annurev.pp.31.060180.002423
- Berry, S. Z., & Uddin, M. R. (1988). Effect of high temperature on fruit-set in tomato cultivars and selected germplasm. HortScience, 23 3 , 606–608 .
- Bhandari, K., Siddique, K. H. M., Turner, N. C., Kaur, J., Singh, S., Kumar Agrawal, S., & Nayyar, H. (2016). Heat stress at reproductive stage disrupts leaf carbohydrate metabolism, impairs reproductive function, and severely reduces seed yield in lentil. Journal of Crop Improvement, 30(2), 118–151 https://doi.org/10.1080/15427528.2015.1134744.
- Blonder, B., & Michaletz, S. T. (2018). A model for leaf temperature decoupling from air temperature. Agricultural and Forest Meteorology, 262(November), 354–360. https://doi.org/10.1016/j.agrformet.2018.07.012
- Bokszczanin, K. (2013). Perspectives on deciphering mechanisms underlying plant heat stress response and thermotolerance. Frontiers in Plant Science, 4(August), 1–20. https://doi.org/10.3389/fpls.2013.00315
- Chaudhary, S, Devi, P., Bhardwaj, A., Chand Jha, U., Sharma, K. D., Prasad, P. V. V., Siddique, K. H. M., Bindumadhava, H., Kumar, S., & Nayyar, H. (2020, October). Identification and characterization of contrasting genotypes/cultivars for developing heat tolerance in agricultural crops: Current status and prospects. Frontiers in Plant Science, 11 587264 https://doi.org/10.3389/fpls.2020.587264 .
- Deva, C. R., Urban, M. O., Challinor, A. J., Falloon, P., & Svitákova, L. (2020). Enhanced leaf cooling is a pathway to heat tolerance in common bean. Frontiers in Plant Science, 11(March), 19. https://doi.org/10.3389/fpls.2020.00019
- Giri, A., Heckathorn, S., Mishra, S., & Krause, C. (2017). Heat stress decreases levels of nutrient-uptake and -assimilation proteins in tomato roots. Plants, 6(1), 6. https://doi.org/10.3390/plants6010006
- Hameed, M., Keitel, C., Ahmad, N., Mahmood, T., & Trethowan, R. (2015). Screening of tomatoes germplasm for heat stress tolerance under controlled conditions. Procedia Environmental Sciences, 29(Agri), 173–174. https://doi.org/10.1016/j.proenv.2015.07.245
- Ibrahim, E. A. (2016). Seed priming to alleviate salinity stress in germinating seeds. Journal of Plant Physiology, 192(March), 38–46 doi:10.1016/j.jplph.2015.12.011.
- Islam, M. (2011). Effect of temperature on photosynthesis, yield attributes and yield of tomato genotypes. International Journal of Experimental Agriculture, 2 1 , 8–11 http://ggfjournals.com/assets/uploads/MIN-171_Tomato_(Tariqul)_(8-11).pdf .
- Kapotis, G., Zervoudakis, G., Veltsistas, T., & Salahas, G. (2003). Comparison of chlorophyll meter readings with leaf chlorophyll concentration in Amaranthus Vlitus: Correlation with physiological processes. Russian Journal of Plant Physiology, 50(3), 395–397. https://doi.org/10.1023/A:1023886623645
- Khanal, B. (2012). Effect of day and night temperature on pollen characteristics, fruit quality and storability of tomato. Norwegian University of Life Sciences. https://nmbu.brage.unit.no/nmbu-xmlui/bitstream/handle/11250/189462/Thesis_BikashKhanal.pdf?sequence=1
- Kimura, S., & Sinha, N. (2008). Tomato (Solanum lycopersicum): A model fruit-bearing crop. Cold Spring Harbor Protocols, 11(11), db.emo105. https://doi.org/10.1101/pdb.emo105
- Kromdijk, J., Głowacka, K., Leonelli, L., Gabilly, S. T., Iwai, M., Niyogi, K. K., & Long, S. P. (2016). Improving photosynthesis and crop productivity by accelerating recovery from photoprotection. Science, 354(6314), 857–861 doi:10.1126/science.aai8878.
- Kugblenu, Y. O., Oppong Danso, E., Ofori, K., Andersen, M. N., Abenney-Mickson, S., Sabi, E. B., Plauborg, F., Abekoe, M. K., Ofosu-Anim, J., Ortiz , R., Jorgensen, S.T. et al. (2013). Screening tomato genotypes for adaptation to high temperature in West Africa. Acta Agriculturae Scandinavica Section B: Soil and Plant Science, 63(6), 516–522. https://doi.org/10.1080/09064710.2013.813062
- Kuhlgert, S., Austic, G., Zegarac, R., Osei-Bonsu, I., Hoh, D., Chilvers, M. I., Roth, M. G., Bi, K., TerAvest, D., Weebadde, P., & Kramer, D. M. (2016, 10). MultispeQ beta: A tool for large-scale plant phenotyping connected to the open PhotosynQ network. Royal Society Open Science, 3(10), 160592. https://doi.org/10.1098/rsos.160592
- Kumagai, E., Araki, T., & Kubota, F. (2009). Correlation of chlorophyll meter readings with gas exchange and chlorophyll fluorescence in flag leaves of rice (Oryza Sativa L.) plants. Plant Production Science, 12(1), 50–53 https://doi.org/10.1626/pps.12.50.
- Lee, K., Nigmatullayevich Rajametov, S., Jeong, H.-B., Cho, M.-C., Lee, O.-J., Kim, S.-G., Yang, E.-Y., & Chae, W.-B. (2022). Comprehensive understanding of selecting traits for heat tolerance during vegetative and reproductive growth stages in tomato. Agronomy, 12(4), 834. https://doi.org/10.3390/agronomy12040834
- Li, L., Li, J., Gao, Q., & Chen, J.-X. (2015). Effects of day and night temperature difference on growth, development, yield and fruit quality of tomatoes. Ying Yong Sheng Tai Xue Bao = the Journal of Applied Ecology, 26(9), 2700–2706. http://www.ncbi.nlm.nih.gov/pubmed/26785551
- Lin, K.-H., Lee, S.-P., George Kuo, C., Chen, J.-T., Yeh, W.-L., & Lin, K.-H. (2006). RAPD markers for the identification of yield traits in tomatoes under heat stress via bulked segregant analysis. Hereditas, 143(2006), 142–154. https://doi.org/10.1111/j.2006.0018-0661.01938.x
- Mansour, A., Ismail, H. M., Fawzy Ramadan, M., & Gyulai, G. (2009). Variations in tomato (Lycopersicon Esculentum) cultivars grown under heat stress. Journal Für Verbraucherschutz Und Lebensmittelsicherheit, 4(May), 118–127. https://doi.org/10.1007/s00003-009-0474-5
- Melomey, L., Danquah, A., Offei, S. K., Ofori, K., Danquah, E., & Osei, M. (2019). Review on tomato (Solanum Lycopersicum, L.) Improvement Programmes in Ghana Nyaku, S. T., Danquah, A. eds. . In Recent advances in tomato breeding and production. IntechOpen. https://doi.org/10.5772/intechopen.75843
- Muller, P., Li, X.-P., & Niyogi, K. K. (2001). Non-Photochemical quenching. A response to excess light energy. Plant Physiology, 125(4), 1558–1566. https://doi.org/10.1104/pp.125.4.1558
- Murata, N., Takahashi, S., Nishiyama, Y., & Allakhverdiev, S. I. (2007). Photoinhibition of photosystem II under environmental stress. Biochimica et Biophysica Acta, 1767(6), 414–421 https://doi.org/10.1016/j.bbabio.2006.11.019.
- Osei‐Bonsu, I., McClain, A. M., Walker, B. J., Sharkey, T. D., & Kramer, D. M. (2021). The roles of photorespiration and alternative electron acceptors in the responses of photosynthesis to elevated temperatures in cowpea. Plant, Cell & Environment, 44(7), 2290–2307. https://doi.org/10.1111/pce.14026
- Payne, R. W. (2009). Genstat. WIREs Computational Statistics, 1(2), 255–258. https://doi.org/10.1002/wics.32
- Prasad, P. V. V., Bheemanahalli, R., & Krishna Jagadish, S. V. (2017). Field crops and the fear of heat stress—Opportunities, challenges and future directions. Field Crops Research, 200 2017 , 114–121. https://doi.org/10.1016/j.fcr.2016.09.024
- Rajametov, S. N., Yang, E. Y., Jeong, H. B., Cho, M. C., Chae, S. Y., & Paudel, N. (2021). Heat treatment in two tomato cultivars: A study of the effect on physiological and growth recovery. Horticulturae, 7(5), 119. https://doi.org/10.3390/horticulturae7050119
- Reynolds, M. P., Nagarajan, S., Razzaque, M. A., & Ageeb, O. A. A. (2001). Heat tolerance. In M. P. Reynolds & A. Mcnab (Eds.), Application of physiology in wheat breeding (pp. 124–135). CIMMYT.
- Rick, C. M. (1978). The Tomato. The Scientific American, 239(2), 66–67. https://doi.org/10.1038/scientificamerican0878-76
- Rivero, R. M., Ruiz, J. M., García, P. C., López-Lefebre, L. R., Sánchez, E., & Romero, L. (2001). Resistance to cold and heat stress: Accumulation of phenolic compounds in tomato and watermelon plants. Plant Science : An International Journal of Experimental Plant Biology, 160(2), 315–321 doi:10.1016/s0168-9452(00)00395-2.
- Saeed, A., Hayat, K., Ali Khan, A., & Iqbal, S. (2007). Heat tolerance studies in tomato (Lycopersicon Esculentum Mill.). International Journal of Agriculture & Biology, 9(4), 649–652 1560–8530/2007/09–4–649–652.
- Salvucci, M. E., & Crafts-Brandner, S. J. (2004). Inhibition of photosynthesis by heat stress: The activation state of rubisco as a limiting factor in photosynthesis. Physiologia Plantarum, 120(2), 179–186. https://doi.org/10.1111/j.0031-9317.2004.0173.x
- Sato, S., Peet, M. M., & Thomas, J. F. (2000). Physiological factors limit fruit set of tomato (Lycopersicon Esculentum Mill.) under chronic, mild heat stress. Plant, Cell & Environment, 23(7), 719–726. https://doi.org/10.1046/j.1365-3040.2000.00589.x
- Shippers, R. R. (2000). African indigenous vegetables. An overview of the cultivated species. University of Greenwich, Natural Resources Institute.
- Sita, K., Sehgal, A., Nair, R. M., Nair, R. M., Vara Prasad, P. V., Kumar, S., Gaur, P. M., Singh, P. K., Prasad, S. M., Dubey, N. K., Pandey, A. C., Sahi, S., & Chauhan, D. K. (2017). Food legumes and rising temperatures: Effects, adaptive functional mechanisms specific to reproductive growth stage and strategies to improve heat tolerance. Frontiers in Plant Science, 8(October), 1–30. https://doi.org/10.3389/fpls.2017.01658
- Stevens, M. A., & Rudich, J. (1978). Genetic potential for overcoming physiological limitations on adaptability, yield, and quality of the tomato. HortScience, 13 6 , 673–678 .
- Takahashi, S., & Murata, N. (2008). How do environmental stresses accelerate photoinhibition? Trends in Plant Science, 13(4), 178–182. https://doi.org/10.1016/j.tplants.2008.01.005
- Tietz, S., Hall, C. C., Cruz, J. A., & Kramer, D. M. (2017). NPQ (T): A chlorophyll fluorescence parameter for rapid estimation and imaging of non-photochemical quenching of excitons in photosystem-II-associated antenna complexes. Plant, Cell & Environment, 40(8), 8. https://doi.org/10.1111/pce.12924
- Van Ploeg, D., & Heuvelink, E. (2005). Influence of sub-optimal temperature on tomato growth and yield: A review. The Journal of Horticultural Science and Biotechnology, 80(6), 652–659. https://doi.org/10.1080/14620316.2005.11511994
- Villareal, R. L., & Lai, S. H. (1979 Development of heat-tolerant tomato varieties in the tropics 1st International Symposium on Tropical Tomato October 23-31 Shanhua, Tainan, Taiwan). . In . Asian Vegetable Research and Development Center 188–200 .
- Wahid, A., Gelani, S., Ashraf, M., & Foolad, M. R. (2007). Heat tolerance in plants: An overview. Environmental and Experimental Botany, 61(3), 199–223. https://doi.org/10.1016/j.envexpbot.2007.05.011
- Walker, B. J., Drewry, D. T., Slattery, R. A., VanLoocke, A., Cho, Y. B., & Ort, D. R. (2018). Chlorophyll can be reduced in crop canopies with little penalty to photosynthesis. Plant Physiology, 176(2), 1215–1232 doi:https://doi.org/10.1104/2Fpp.17.01401 .
- Willcox, J. K., Catignani, G. L., & Lazarus, S. (2003). Tomatoes and cardiovascular health. Critical Reviews in Food Science and Nutrition, 43(1), 1–18. https://doi.org/10.1080/10408690390826437
- Wolff, H. (1999). Economics of tomato production with special reference to aspects of plant protection: A case study of two tomato production systems in Brong-Ahafo Region, Ghana. Ghanaian-German project for integrated crop protection (GTZ: Eschborn).
- Zhang, X., Zhou, Q., Wang, X., Cai, J., Dai, T., Cao, W., & Jiang, D. (2016). Physiological and transcriptional analyses of induced post-anthesis thermo-tolerance by heat-shock pretreatment on germinating seeds of winter wheat. Environmental and Experimental Botany, 131, 181–189. https://doi.org/10.1016/j.envexpbot.2016.08.002
- Zhou, R., Kjaer, K. H., Rosenqvist, E., Yu, X., Wu, Z., & Ottosen, C.-O. (2017). Physiological response to heat stress during seedling and anthesis stage in tomato genotypes differing in heat tolerance. Journal of Agronomy and Crop Science, 203(1), 68–80. https://doi.org/10.1111/jac.12166
- Zhou, R, Xiaqing, Y., Kjær, K. H., Rosenqvist, E., Otto Ottosen, C., & Zhen, W. (2015). Screening and validation of tomato genotypes under heat stress using Fv/Fm to reveal the physiological mechanism of heat tolerance. Environmental and Experimental Botany, 118(October), 1–11 https://doi.org/10.1016/j.envexpbot.2015.05.006.
- Zinn, K. E., Tunc-Ozdemir, M., & Harper, J. F. (2010). Temperature stress and plant sexual reproduction: Uncovering the weakest links. Journal of Experimental Botany, 61(7), 1959–1968. https://doi.org/10.1093/jxb/erq053