Abstract
Mushrooms have a long history of cultivation, dating back to 600 AD. However, most edible mushroom species worldwide are still gathered from the wild, a practice which is unsustainable in contributing to global food security. Cultivation of saprotrophic mushroom species has successfully used agricultural waste as growing substrate. Their mycorrhizal counterparts, however, remain dependent on their woody hosts and seasonality thereby being less accessible to urban communities. The main reason has been the lack of methods which effectively simulate mycorrhizal symbiotic environments. Apart from requiring particular species of bacteria for mycorrhization with their woody host species, mycorrhizal mushrooms also strictly require glucose or fructose as their only source of carbon. Hence, sustainable off-host cultivation of mycorrhizal mushrooms will require better understanding of exact conditions of mycorrhization and fructification. In this review, a brief history of use and general biology of mushrooms, and the cultivation methods employed for non-mycorrhizal and mycorrhizal mushrooms are discussed. Finally, a discussion is provided on the prospects for Sub-Saharan Africa in developing modern sustainable cultivation methods for wild mushrooms towards meeting the key UN Sustainable Development Goals.
Public interest statement
This review discusses the processes and methods used in cultivating mushrooms without going into the intricate scientific or technical details. It starts with the biology of mushrooms in brief and briefly highlights the technologies of spawn production, use of substrate materials, substrate sterilization methods and an exploration into more difficult-to-cultivate groups of mushrooms. A bias is emphasized on the characteristics of mutualistic mushroom cultivation in view of their strict requirement for associating with woodland trees. This bias is because most edible mushroom species of African woodlands are mycorrhizal. The review further discusses some scientific improvements pertaining to modern mushroom cultivation and some outstanding challenges hindering progress in developing robust cultivation methods for such mycorrhizal mushrooms. It ends by urging concerted research efforts on improvements necessary for successful cultivation of mutualistic mushrooms in the absence of their tree partners, with focus on Sub-Saharan African conditions.
1. Introduction
All over the world mushrooms play significant dietary, medicinal, recreational and socio-economic roles. To date most mushroom species are still harvested from the wild during seasons of their availability. Mushrooms also play an ecologically important nutrient cycling function, making them a major decomposer of organic matter. Mushrooms belong to a macroscopic group of organisms belonging to the kingdom Fungi (=Mycota). Of the estimated 2.2 to 3.8 million fungal species with diverse life forms within this kingdom (compared to 220,000–420,000 plant species), only 3 to 8 percent have been described. Hence, fungi have remained one of the least understood kingdom of life (Hawksworth & Lücking, Citation2017; Tedersoo et al Citation2010). Unlike bacteria, protists and animals, fungi have evolved both microscopic and macroscopic forms without losing their heterotrophic lifestyle (Smith et al., Citation2017). A majority of the microscopic fungal clades are saprotrophic, with quite a few being mutualistic and others parasitic (Pérez-Moreno et al., Citation2021). A variety of plant and animal parasitic fungi often cause diseases while a few mycoparasites cause diseases in mushrooms (Fernandez-Conrad et al., Citation2018; Grossart et al., Citation2019), thereby drawing research interest from farmers and agricultural scientists. Latest phylogenetic classification of fungi, notably one by Naranjo-Ortiz and Gabaldón (Citation2019), have recognised nine phyla of which Basidiomycota (containing true mushrooms) and Ascomycota (containing truffles and morels) are collectively grouped under sub-kingdom Dikarya (de-Miguel et al., Citation2014). Related phylogenetic studies have instead viewed Dikarya as a super-phylum with diverse trophic lifestyles. While saprotrophic Dikarya play a major decomposer role in terrestrial ecosystems (Fernandez-Conrad et al., Citation2018; Grossart et al., Citation2019), mycorrhizal Dikarya form mutualistic associations with plants (Van der Heijden et al., Citation2014). In between saprotrophic and mycorrhizal forms are a few such as Armillaria species known to start as mutualistic, becoming parasitic at later stages of their life cycle as observed in orchids where the association is described as myco-heterotrophic (Baumgartner et al., Citation2011). Hence fungi which employ the different trophic mechanisms make it difficult to cultivate them using one generalised method.
Within the kingdom Mycota cellular organisation varies in complexity. The earliest evolutionary lineages of fungi are unicellular, as with yeasts with the majority being multi-cellular and filamentous (Abebe, Citation2021). Later lineages are also filamentous but more complex and capable of forming specialized organs adapted for feeding, growth, reproduction, or exploration of new habitats (Abebe, Citation2021). The more complex groups invariably possess hyphal strands as functional units which grow into more complex vegetative mycelia. These mycelia may bear special fruiting bodies for sexual or asexual reproduction, and are found growing away from the substratum for efficient spore dispersal (Naranjo-Ortiz & Gabaldón, Citation2019). Fruiting bodies of genetically different fungi have characteristic form, size, colour and texture which also vary with environmental conditions (Riquelme et al., Citation2018). It is under this latter more complex group of fungi that mushrooms (macrofungi) fall and for which the fruiting bodies are treasured as food. The structural differences in the various fungal groups have strong on implications to their trophic lifestyles which become more advanced in some mycorrhizal types.
In the past three centuries mycological studies have focused on microfungi dating back before Linnaeus (Naranjo-Ortiz & Gabaldón, Citation2019). In the first half of the 20th century macrofungi have attracted increasing research interest owing to their emergent commercial value as food, medicine and use in biotechnology which more recently aims to help meet some of the United Nations Sustainable Development Goals 1, 2, 3, 9 and 12 (Díaz-Godínez & Téllez-Téllez, Citation2021; Sharma & Sharma, Citation2016). Mushrooms’ underlying ecological roles as nutrient recyclers and as food for a variety of fauna including insects, reptiles and some mammals have also helped in consolidating recent prioritisation of their research (Pérez-Moreno et al., Citation2021; Wallis et al., Citation2012; X. Zhang et al., Citation2021). Furthermore, the vast subterranean networks of macrofungal mycelia make them a major functional determinant in subterranean ecosystems and food webs (Crotty & Adl, Citation2019) thereby posing a puzzle for scientists to solve. The large number of macrofungi which have also evolved to colonize above-ground habitats on dead wood and grass straw as found with Auricularia (Wood ear), Pleurotus (Oyster) and Ganoderma (Reishi) species (Edman et al., Citation2021), make them a major potential organic matter decomposer in the face of rising challenges in industrial and agricultural waste disposal (D. Grimm et al., Citation2021a; Rashad et al., Citation2019). This decomposer role of macrofungi has greatly assisted forest and environmental managers in formulating suitable policies to maintain and promote global balance of nature and healthy forests.
A mushroom is an enlarged complex epigeous fleshy fruiting body of fungi (such as a basidiomycete) consisting typically of a vegetative stem (stipe) bearing a macroscopic fruiting body (cap = pileus; Pilz & Molina, Citation2001), although several species may have no conspicuous stipe. A truffle is “the dark or light edible hypogeous (subterranean) fruiting body of several ascomycetous fungi (especially genus Tuber)” and a morel is “any of several edible fungi (genus Morchella) having a conical sporocarp with a highly pitted surface” also belonging to Ascomycetes. In this review the term “mushroom” will be applied to any of the three categories; true mushrooms, truffles and morels. However, the lifecycles of truffles and morels are understood to be heterothallic and more complex than for most mushrooms, for example, in Tuber melanosporum the haploid spore germinates into a hyphae which mycorrhizes with the host root before plasmogamy is attained (Payen et al., Citation2014; Qin & Feng, Citation2022). Even the season length for sporocarp development versus the host tree phenology is understood to differ from that of mycorrhizal basidiomycetes. All these mushroom groups have been harvested for several millennia as food, medicine, recreation, tinder, hallucinogens, dyes and for religious purposes among different human cultures. Hence scientists are now seeking deeper and wider understanding of their biology (Ferraro et al., Citation2020; Rizzo et al., Citation2021). Here a focus is presented on understanding of mycorrhizal mushroom biology to promote their cultivation and help meet the rising global demand in an attempt towards complementing existing agricultural crops.
In general, mushrooms vary in their trophic lifestyles, from being saprotrophic, parasitic to mycorrhizal (Salo et al., Citation2019). Most mushrooms currently cultivated ex-situ are saprotrophic or have partially retained that lifestyle during the course of their evolution. To date more than 15 saprotrophic genera are now cultivated all over the world with just six species accounting for 90 per cent by volume of production (Royse et al., Citation2017). For the little understood mycorrhizal species, in-situ production has been used, with ex-situ production having been achieved in nurseries of their natural host tree seedlings or their artificial forests (Díaz et al., Citation2009). Off-host commercial production of mycorrhizal species has remained unattainable so far due to little understanding of conditions required for sporocarp formation and development. The objective of this paper is to give an overview of developments achieved so far in cultivation of mycorrhizal mushrooms with a Sub-Saharan Africa focus (Figure ). For perspective a discussion is given on cultivation methods used for saprotrophic and mycorrhizal mushrooms to date, and highlight opportunities and constraints of these methods in commercial scale production. The approach adopted in discussing the two trophic groups of mushrooms will serve to give insights adapting current cultivation methods to cultivating mycorrhizal species.
Figure 1. A map of Africa showing the relative expanse of the Sub-Saharan region shown in red. Source: https://www.researchgate.net/figure/A-physical-geography-map-of-Africa-from-the-Perry-Castaneda-map-collection_Figure2_32898263 (Accessed 11 October 2022).
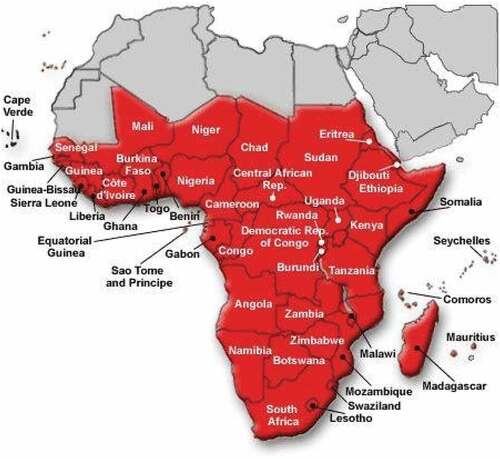
2. Cultivation of saprotrophic mushrooms
Mushroom cultivation dates back to early 600 AD for Wood ear mushroom (Auricularia auricula-judae) in China (Chang, Citation1977), followed by White button mushroom (Agaricus bisporus) a millennium later in France in the 1600s (Gayley, Citation1938). Cultivation methods started from minimal environmental modification in caves, quarries and tunnels with little control over produce yield and quality (Biswas & Biswas, Citation2017). Subsequently scientific methods of cultivation were developed leading to domestication of Paddy straw mushroom (Volvariella volvacea) in China in 1822 (Chang, Citation1972; Gruen, Citation1964). The number of cultivated species rapidly expanded in the late 1900s to include species such as Shiitake (Lentinula edodes), Oyster mushroom (Pleurotus sp.), Reishi (Ganoderma lucidum) and many other saprotrophs (Fernandes et al., Citation2021). These early domestication efforts assured consistent supply of these food commodities in many places in the Far East, Europe and the Americas (Rathod et al., Citation2021). Quite recently other more rare parasitic species such as Lion’s mane (Hericium erinaceus), Chaga (Inonotus obliquus), Turkey tail (Trametes versicolor) and others have attracted attention as cultivable medicinal species (Rathod et al., Citation2021). The attention placed on these latter species as next generation sources of pharmaceutical drugs is likely to accelerate development of more efficient cultivation methods. In the saprotrophic category though, Termitomyces species have evaded cultivation due to their little understood intricate subterranean association with termites.
Prior to development of aseptic techniques rampant contamination of mushroom substrates by competitor fungi and bacteria tended to constrain yield and quality (Gea et al., Citation2021; Ghimire et al., Citation2021). In starting a new mushroom crop the inoculum used was a mixture of crushed sporocarps, or soil harboring mushroom spores dug out from the shroomery (mushroom garden)- the so-called “dirty technology” (Yun & Hall, Citation2004). Back then mushrooms were grown on bat guano in caves and mine quarries where atmospheric humidity and dark conditions were naturally self-modulating to suit fast mycelium growth and subsequent fructification but with many technical limitations such as poor humidity, light and contamination control giving sub-optimal yields (Dulal, Citation2019; Xie et al., Citation2020). The development of aseptic techniques by Joseph Lister (1827–1912) in mushroom production made it possible to cultivate mushrooms using more hygienic methods. Development of mushroom grain spawn by James W. Sinden in 1932 made higher yields and quality possible (Shungchang et al., Citation2019).With worldwide adoption of mushroom commercial cultivation using the foregoing innovations as basic requirements, more saprotrophic species are now being cultivated. Hence most of the current research efforts focus on fine-tuning current methods to suit growth conditions required by specific mushroom types and specific environments.
2.1. Mushroom spawn production
Currently, successful mushroom production relies on use of reliable robust spawning material. Much of the spawn developed is produced by aseptically culturing mushroom context tissue in carbon-rich media such as potato dextrose agar (PDA) or malt extract agar (MEA) media (Harith et al., Citation2014; Hsu et al., Citation2018; Matjuðkova et al., Citation2018). For the commonly grown mushroom species such as Pleurotus, Agaricus and Volvariella, 6 to 12 days of incubation is normally sufficient to obtain their pure primary cultures under their optimum temperature and pH (Abon et al., Citation2020; Mahadevan & Shanmugasundaram, Citation2018). These pure cultures are then inoculated onto easier-to-handle and easier-to-store basal substrates such as grain cereals of wheat, rice, rye, sorghum or millet (Hsu et al., Citation2018; Zied et al., Citation2021). These cereal substrates have the benefits of low nitrogen content, slow release carbon thereby inhibiting most competitor organisms; as well as ease of availability, ease of drilling and uniformity of spawn spread onto the final growing substrates (Afzal et al., Citation2019). Depending on the mushroom species, complete colonisation of the basal substrate may take several days to a few weeks with most species, for example, 15 to 18 days at 25°C for Pleurotus sp. (Figure ). A more detailed discussion of mushroom spawn production can be found in Borah et al. (Borah et al., Citation2019).
As an alternative for cereal grain, wood dowel spawn and liquid spawn have been developed for specific production situations. These media have mostly been peripherally used in research, with the liquid spawn being more adaptable for industrial manufacture of medical drugs under strictly controlled submerged culture conditions (Bedade et al., Citation2016; Liu et al., Citation2018). However, despite proven viability of all these aforementioned modern technologies in developed countries, the mushroom spawn making business in developing countries remains constrained by shortage of skills and appropriate equipment (Higgins et al., Citation2017). Consequently mushroom spawn unavailability and inhibitive cost of importing spawn have become the two most critical mushroom production challenges (Ayanfunke, Citation2019). To date, several studies have been conducted to maximize spawn quality for different mushroom species grown, and for a wider range of farm available agricultural waste-derived growing substrates (Koutrotsios et al., Citation2020).
2.2. Substrates for saprotrophic mushrooms
Substrates used in spawn-running (mycelium colonizing leading to sporocarp production) for saprotrophic mushrooms are relatively easy to procure as agricultural waste is abundant (Besufekad et al., Citation2020; Fufa et al., Citation2021; Piazza et al., Citation2021). Any stover is suitable for mushrooms such as Pleurotus species, which are grown in pasteurised substrates (El-Nour & Ibraheim, Citation2021) and Agaricus species, on composted substrates (Ayanfunke, Citation2019). For the latter species, nitrogen content of substrates in some cases may be improved by adding such materials as urea or nitrate of calcium or ammonium nitrate while pH can be adjusted by incorporating lime into the spawn-running substrate (El-Nour & Ibraheim, Citation2021). Straw of rice, wheat, maize, or banana leaf, soybean, groundnut hulls, cotton waste, wild grass and any other material of high cellulose content, have proved suitable growing substrate materials for most non-wood decaying mushroom species (Ashraf et al., Citation2013). Hence mushroom production can be viewed as a sustainable value addition process for such stover materials which are traditionally ploughed under or fed to livestock as silage (Chen et al., Citation2021). The spent mushroom residue can easily be disposed of as a more nutritious and more palatable stock feed, or as nursery potting compost (D. Grimm et al., Citation2021a; Muchena et al., Citation2021). Where crop stover is stored for prolonged periods it tends to become heavily contaminated and gets depleted in cellulose content, making it less biologically viable in mushroom production (Foley & Yakushenko, Citationundated). However, fresh stover stocks are readily available following harvests of winter and summer cereal and leguminous crops. In terms of the types of lignocellulosic substrates, saprotrophic mushrooms can be conveniently grouped into soil organic matter decomposers, wood decomposers and grass decomposers. Several such alternative substrates which can be prepared using appropriate methods for different mushroom categories are available to mushroom farmers (Table ).
Table 1. List of abbreviations used
2.3. Substrate disinfection methods
At all stages of mushroom production competitor microorganisms pose a great threat to successful establishment. Although standard aseptic techniques are well understood and implemented at spawn production, challenges of modern appropriate equipment still face spawn production in SSA. Across the sub-region, however, well equipped tertiary educational institutions and entrepreneurs exist to adequately address this challenge. The bigger challenge still remains in the lack of technical equipment for substrate disinfection. Competitor fungi such as Trichoderma spp., Penicillium spp., Aspergillus spp. and Coprinus spp., all of which tend to have ubiquitous and copious amounts of spores, can colonise the substrate at any stage of substrate preparation (Gowda & Pandey, Citation2014). In their innovation (Gowda & Kumaran, Citation2014)showed that pasteurization at temperatures of 60°C to 80°C for holding times of 30 minutes to 4 hours has varying effects against the competitor fungi, with least effectiveness at the lower end of the temperature-holding time range. Both their low capacity and bulk pasteurizers versions using hot water or steam for all types of substrates are now in practical use. Alternatively, “cold” sterilisation techniques utilising a variety of chemical treatments such as 12 hour soaking in 500 ppm formalin, 1,5 to 8 % hydrogen peroxide or 0.66 to 3.3 % chlorine solutions have been found to be effective against competitor fungi with insignificant effect of mushroom mycelium growth (Gowda & Pandey, Citation2014). Alkaline immersion of the substrate in 2 % hydrated lime or quicklime for 12 hours and spawning after 24 hours of withdrawal of that treatment has also been reported to be effective particularly in production of Pleurotus species (Gowda & Pandey, Citation2014). Ultimately, the method of choice for substrate disinfection depends on its accessibility, compatibility with substrate and cost reduction ability among other things.
2.4. Mushroom spawn running conditions
Saprotrophic mushroom cultivation is mostly done under enclosed environments (Ferdousi et al., Citation2019). In China and other eastern countries, however, some of the mushrooms, including Volvariella volvacea, are cultivated in open field (Thiribhuvanamala et al., Citation2021; Thuc et al., Citation2020). Enclosed environments with well-programmed activities make it easy to control key growth factors such as relative humidity, lighting, and carbon-dioxide content. Such enclosed environments also bar entry of contaminant spores of competitor fungi (Dey et al., Citation2008; Gea et al., Citation2021). Controlled conditions also make it possible to sustain high sporocarp yields and quality all year round (A. Grimm et al., Citation2021b). Consequently, early methods for growing saprotrophic mushrooms utilised open fields called shroomeries where the natural conditions were only suitable for seasonal mushroom emergence. Modern methods utilise mushroom houses which make higher yields and higher quality possible all year round. Hence the general trend has been to modernize production methods even though it entails additional production costs.
Mushroom cropping cycles are usually complete in two months for species grown on straw and compost. Within this cycle, two to four flushes may be picked before starting the next cropping cycle. Whereas wood and grass rotting mushrooms such as Pleurotus, require pasteurisation of substrates before spawn running, organic matter decomposers like Agaricus, require that fresh straw be fermented first through the ammonia-releasing thermophilic phase first, followed by cellulose-hemicellulose digestion phase, before spawn-running is applied (Kertesz & Thai, Citation2018). Whereas Pleurotus requires lighting to induce pinning (sporocarp formation), Agaricus does not. Species grown using these methods may take 20 to 35 days with two or three productive flushes up to 10 days apart. More details on saprotrophic mushroom production processes can be found in (D. Grimm et al., Citation2021a; Jurak et al., Citation2015; Kabel et al., Citation2017; Noble & Gaze, Citation1996; Noble et al., Citation2002; Royse & Beelman, Citation2016; Straatsma et al., Citation2000; Weil et al., Citation2013). Such production methods have, to date, demonstrated to vary little considering the large volume of research conducted so far. However, due to their vastly different trophic lifestyle, mycorrhizal mushroom production has followed different methods ().
Table 2. Substrates in use and substrate preparation methods for different saprotrophic mushroom species categories
Table 3. Comparison of cultivation methods for saprotrophic versus mycorrhizal mushrooms
Cultivation methods for saprotrophic mushrooms vary among the groups viz. wood-rotting, grass litter and compost decayers but all of these are invariably grown in mushroom houses in SSA with some enhancement including some form of N-enrichment and sterilization/pasteurization. On the other hand, mycorrhizal mushrooms are grown in tree plantations or seedling nurseries with no environmental modifications (Figure ). This figure also gives an overview of the major subterranean entities and processes.
Figure 3. Comparison of subterranean and aerial environments between saprotrophic and mycorrhizal mushroom cultivation.
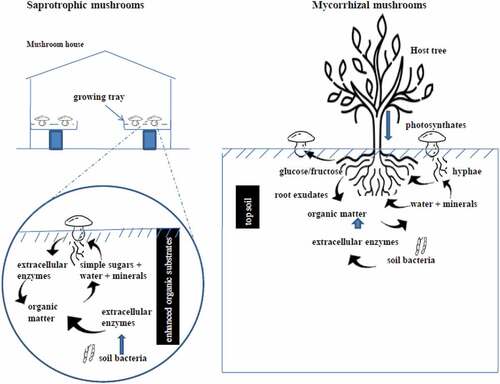
Despite innovation of several technologies, the adoption of mushroom cultivation in SSA has not been impressive so far. To date it seems only South Africa has gone some way in cultivation of mycorrhizal mushrooms, notably the black truffles and (Lyophyllum) shimeji (Table ). This is attributable mostly to existence of geographical regions that sustain woody host species of truffles similar to temperate regions where these truffles are already under cultivation.
Table 4. Cultivated mushrooms in SSA by country, genus of mushroom produced, number of mushroom species cultivated, purpose for production and references
3. Cultivation of mycorrhizal mushrooms
In their provenances, mycorrhizal mushrooms grow in association with woody species, making them obligate symbionts (Pérez-Moreno et al., Citation2021). Several edible mycorrhizal species are found worldwide, including Tuber melanosporum, T. aestivum, T. magnatum, T. gibbosum, Leucangium carthusianum, Boletus edulis, Cantharellus cibarius, C. subalbidus, Hydnum repandum, Lyophyllum shimeji, Lactarius deliciosus, Tricholoma matsutake and Tr. magnivelare, which are all from temperate and boreal forests (García-Montero et al., Citation2007; Pérez-Moreno et al., Citation2021; Stobbe et al., Citation2013). Miombo woodlands of Southern Africa also harbor a large number of edible mycorrhizal mushrooms which include Russula oleifera, Amanita loosii, Cantharellus miomboensis, C. platyphyllus, C. heinemannianus and Lactarius kabansus (Bloesch & Mbago, Citation2008; Mlambo & Maphosa, Citation2017). The mycorrhizal lifestyle of mushrooms is understood to have evolved from saprotrophic lifestyles where the former acquire carbohydrates from woody host species. The mycorrhizal lifestyle is understood to have evolved separately in different terrestrial biomes (Looney et al., Citation2021), making it a physiological consequence of sharing habitats with their host plants. For efficient physiological functioning such mycorrhizal species have co-opted specific bacterial clades that play a crucial role of promoting mycorrhization processes and/or sporocarp formation and development (I. Hall et al., Citation2017a; Yamanaka et al., Citation2020). Yet other mycorrhizal mushrooms have been found to fructify only in the presence of another specific mycorrhizal fungus, further demonstrating a more complex mycorrhizal lifestyle. An example of this complexity is where an association was observed between Suillus mushroom and Chroogomphus, and also between Rhizopogon mushroom and Gomphidius (Miller, Citation2003). It is the lack of understanding of this mycorrhizal mushroom complexity of lifestyle and mode of its functioning which has slowed down efforts to develop sustainable methods needed in off-host cultivation (Sambyal & Singh, Citation2021).To confound these challenges, the mechanisms by which these mycorrhizal partners promote sporocarp formation are not well understood. Several ex-situ successes for mycorrhizal mushroom have been recorded mainly for three clades viz. truffles, boletes (Porcini) and Milkcap (Morte et al., Citation2021; Wang et al., Citation2021). Such methods have, at best, used host tree seedlings to enable sporocarp formation and maturation. Like saprotrophic mushrooms, the methods for successful cultivation of mycorrhizal mushrooms need to progress towards total environmental control (Figure ) in order to play an important part in meeting the rising world demand for food and achieve global food security through their cultivation outside their provenances.
Figure 4. Stepwise process of mycorrhizal (excluding truffles) mushroom commercial cultivation over the years.
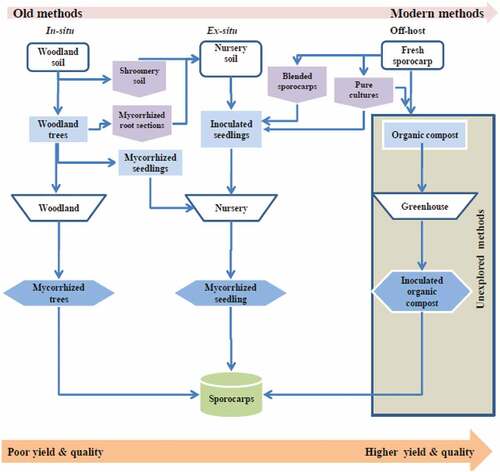
To understand the intricacy in developing cultivation methods for mycorrhizal mushrooms, it is necessary to understand the complex processes around formation of a functional mycorrhiza, sporocarp initiation and development, and the involvement of their bacterial co-symbionts in these processes.
3.1. Ectomycorrhiza formation and establishment
The growth cycle of basidiomycetous mycorrhizal mushrooms starts with spore germination in the vicinity of short roots of suitable host woody species, forming a primary hypha (Xiang et al., Citation2021). Pairs of primary hyphae of opposite polarity then fuse to give dikaryotic secondary hypha (Riquelme et al., Citation2018; Yamanaka et al., Citation2020). It is the secondary hypha which, in the presence of mycorrhiza helper bacteria (MHB), infects the short roots of the woody host. From this infection a meshwork of mycelium forms on the surface of the short roots, establishing close contact with epidermal tissue of the host, culminating in a mycorrhiza. At the same time the extramatrical mycelium forms, with the function of absorbing water and minerals from the surrounding soil. The current understanding of the role of MHB, such as some Microbacterium, Bacillus and Rhizobium species, is to enhance growth rate of the woody species partner short roots first. This enhanced root growth is achieved through MHB mineralizing leaf litter and channeling of several minerals including P, Ca, N and Fe to host tree partner (Gorka et al., Citation2017). The MHB have also been shown to promote germination of the mycobiont through solubilizing phosphate, and production of siderophores and indole acetic acid which, in turn, induce the two biotic partners to find each other (Agnolucci et al., Citation2019; Bruzos, Citation2019). In effect, capacity of the root to mycorrhize with the specific mycobiont is then established (Rigamonte et al., Citation2019). Subsequently, the fungal symbiont takes over the role of mineral supply to host plant. Several studies have traced the MHB presence from the rhizosphere to the sporocarp, with insignificant residual bacterial cells remaining in the soil, implying a progressive functional requirement of MHBs from mycorrhization to sporocarp development (Bonfante & Anca, Citation2009).
The role of MHBs in mycorrhizal mushroom physiology has been analysed through biochemical signatures found to be unique to these fungi. Evidence of chemo-response crosstalk between bacterial and fungal symbionts was found, pointing to the necessity of particular bacterial taxa as mycorrhiza facilitators in the mycorrhizosphere (Obase, Citation2020). Active bacterial exudates such as ostreolysin, veratryl alcohol, β-adenosine, phenol and saponin were found to be used in the crosstalk leading to mycorrhization (Domondon et al., Citation2004; Magae, Citation1999; Sugimoto et al., Citation2001; Upadhyay & Hofrichter, Citation1993; Vidic et al., Citation2005). Terpenes produced by the mycobiont have also been reported to play the tree-fungus-bacteria tripartite communication role (L.A. Lofgren et al., Citation2021). However, several other factors are also thought to elicit similar responses in different macrofungi. Hence the MHB appear to act as the “go-between” facilitators for successful mycorrhizal formation, where they play their role from spore germination, mycelium growth, mycorrhization and mycelium protection against harmful microbes (Veronina & Sidorova, Citation2017).
A fully functional ectomycorrhiza facilitates movement of exchange substances between the partners. Such exchange is done through the Hartig net, which is the ramification of fungal intercellular hyphae with cortical cells of roots (Kuga-Uetake et al., Citation2004; Vaario & Matsushita, Citation2021). While the host root supplies its photoassimilates to the fungus, the fungus passes absorbs minerals and water from the soil to the host root (Nannipieri et al., Citation2011; L. Zhang et al., Citation2014a). It is now well understood that upon the Hartig net establishment carbon flows from the host to the fungus largely as glucose, and less as fructose (Courty et al., Citation2010; Peksen et al., Citation2013). The exact conditions which trigger and sustain such carbon transport are not well understood, although phenological developments of the host have been hypothesized to give successful mycorrhization. A full understanding of the foregoing nutrient exchange mechanism is thus necessary in developing successful cultivation methods for mycorrhizal mushrooms.
3.2. Sporocarp initiation and development
The sporocarp is the fungal reproductive structure of a fungus, which is the organ of most value in all edible macrofungi. Although several greenhouse experiments have succeeded in stimulating mycorrhization, limited sporocarp formation with seedlings of appropriate natural host plants has been realized. Studies suggest that this failure could be due to, among other things, the non-incorporation of MHBs. In Hypsizygus ulmarius, Poonga and Kaviyarasan (Citation2015) found Azotobacter to play an essential role in sporocarp initiation. Earlier, Rainey et al. (Citation1990) had found bacteria to induce sporocarp initiation in Agaricus bisporus, a saprotroph. Cho et al. (Citation2003) found Pseudomonas putida to induce sporocarp primordia in another saprotroph, Pleurotus ostreatus. Frey-Klett et al. (Citation2011) found an association between mycorrhizal Amanita muscaria and Streptomyces bacteria in sporocarp initiation. These foregoing findings suggest the key role bacteria may have in initiating sporocarps for mushroom in general. In addition, other external environmental conditions play a key role in sporocarp initiation as suggested by positive correlations found, for example, between mycorrhizal mushroom emergence and rainfall amount (Martínez-Peña et al., Citation2012). Furthermore, the role of temperature has also been extrapolated using empirical methods (Martínez-Peña et al., Citation2012). Specifically, Butler and Wood (Citation1998), and Godbout and Fortin (Citation1990) found photoperiod to be a major determinant of sporocarp initiation in Laccaria bicolor, a mycorrhizal fungus. Formation of the sporocarp is preceded by hyphal aggregation, which requires communication factors from MHB, followed by fructification of the mushroom (Poonga & Kaviyarasan, Citation2015). During this development, the mycorrhizal hyphae acquire their carbon through the mycorrhizal interface and transport it to the growing sporocarp. It is now understood that all carbon for sporocarp formation and development in mycorrhizal mushrooms is derived from host current photosynthesis (Fortin & Lamhamedi, Citation2009). Hence in off-the-host studies a suitable surrogate directly releasing glucose or fructose in a controlled fashion could be found to simulate the natural C source. Although the requirement of MHB and host C source is likely to be universal for mycorrhizal mushroom sporocarp initiation and development, the specific conditions involved are likely to differ for mycorrhizal mushroom species. To date, it is not understood whether the MHB play any further role during the sporocarp development stage although it is probable that their presence in the sporocarp aids their own dispersal as the mushroom spores eventually disperse. No reports have been found in which these physiological processes, particularly the use of MHB associating with tropical mycorrhizal mushroom species, have been actively manipulated to improve in-situ sporocarp productivity.
3.3. In-situ cultivation of mycorrhizal mushrooms
Continued dependence on hunting for mycorrhizal mushrooms is unsustainable in the long-term. Hence, simple in-situ cultivation methods have evolved. By studying the subtle lifestyles of mycorrhizal mushrooms, modest sporocarp yields have been attained through managing forest health (de-Miguel et al., Citation2014). However, yields have been constrained by anthropogenic factors such as over-harvesting, deforestation, loss of host trees to pests and diseases, and adverse forest management practices such as high tree density (J.S. Hall et al., Citation2003). Replacement of natural with exotic plantations, soil compaction by timber harvesters and mushroom pickers, acid rain and global warming effects have exacerbated the diminishing mushroom productivity of woodlands (Cherfas, Citation1991). The reader is cautioned though, that although mushroom harvesting per se was long found not to result in subsequent sporocarp yield reduction over time, the same may not necessarily be true for the subtropics where there is minimal or no deliberate forest management (Norvell, Citation1995; Pilz & Molina, Citation2001). Effects of trampling, dragging sledges or timber on the ground, and annual veldt fires, however, are likely to further reduce mushroom productivity in the long run, particularly when sporocarp primordia are damaged (Norvell, Citation1995; Pilz & Molina, Citation2001). Other less obvious negative factors such as resettlements, road-building, agriculture, mining, use of fertilizers in areas upland to mushroom habitats, wood harvesting, hunting and selective pest management likely have long-term negative impact on mushroom productivity. With all these challenges and the seasonality pattern of their availability, therefore, reliance on in-situ management for mycorrhizal mushroom supply may not be sustainable in the long run. However, active reversal of these detrimental practices has proved beneficial in improving in-situ mycorrhizal mushroom yields (de-Miguel et al., Citation2014).
3.4. Ex-situ cultivation of mycorrhizal mushrooms
In experiments to grow mycorrhizal mushroom ex-situ, use of tree seedlings on novel compost-based media has had varying success (Yamada et al., Citation2001). First reported by Danell and Camacho (Citation1997) for successful induction of Golden chanterelle (Cantharellus cibarius) sporocarps on pot Pinus seedlings 13 months after inoculation, this method looks quite promising with the potential of all year round sporocarp production. However sporocarp development in pot experiments has been quite unpredictable owing to poor understanding of the exact sporocarp stimulating agents (Endo et al., Citation2013). Most of the recorded successes were achieved after many months of inoculation, casting doubts on effectiveness of the treatments applied versus results obtained (I.R. Hall et al., ; Mediavilla et al., Citation2015; Yagame & Maekawa, Citation2019; Yamanaka et al., Citation2020). This is unlike in their provenances where mycorrhizal mushrooms predictably fructify within five to seven days after adequate rains (Capinha, Citation2018; Mlambo & Maphosa, Citation2021). The low success rates in ex-situ stimulation of sporocarp development for mycorrhizal mushrooms, however, seems to require a shift of focus from in-situ conditional requirements to other less explored approaches based on simulated carbon and nitrogen source.
In their natural habitats, all inorganic mineral requirements of mycorrhizal mushrooms are directly obtained from the soil (Marupakula et al., Citation2017), whereas organic carbon requirements are met initially by leaf litter, root exudates and rotting floral and faunal matter (Doornbos et al., Citation2012). Hence, any substrate materials used in pot culture experiments were essentially mixed with organic compost material deemed to contain mineral reserves to supply both the fungus and the woody host seedlings (Wang et al., Citation2021). However, 98 percent of carbon requirements for mycorrhizal species sporocarp development are woody-host supported (I. Hall et al., Citation2017a; Hibbett et al., Citation2000; Yamanaka et al., Citation2020), which partly explains why pot experiments have had limited success in sporocarp yields owing to the small photosynthetic capacity of seedlings. Application of N in mycorrhizal mushroom-endemic forests has, however, reduced sporocarp yields (Marupakula et al., Citation2017), suggesting inhibitory effects of N fertilizers on sporocarp yields. With their strong ability to mineralize organic P, mycorrhizal mushrooms grown in organic matter-rich substrates are unlikely to experience P shortages. Ultimately, all successes of sporocarp formation ex-situ must depend on provision of a suitable C and N source together with promoter bacteria in the substratum. With all the questions on what conditions are necessary for artificially induced sporocarp production in mycorrhizal mushroom still unanswered, new approaches in research need to be taken to improve world production of these prized products.
4. Future perspectives for mushroom cultivation
Mycologists have realised the close physiological similarities between fungi and humans and hence the unavoidable dependence of man on fungi as food and medicine (Gründemann et al., Citation2019; Joseph et al., Citation2018). The new interest in fungal products as new generation pharmacologicals and bioactives has intensified research in mushroom production (Gründemann et al., Citation2019). The near future is therefore well set to witness systematic cultivation of several more species of mushrooms, which will assist towards reducing food insecurity in SSA and other developing regions. Developments in microbiological techniques such as submerged methods, in-culture based techniques, biotechnology, and growth media technology will likely spur discovery of new practical uses and hence the need for new and effective production methods for mushrooms. It is therefore not far-fetched to expect that more saprotrophic mushroom species that have routinely been gathered from the wild will soon find their way into formal agriculture. This is because the free-living lifestyle of saprotrophic mushrooms is now well understood (Fufa et al., Citation2021; Harith et al., Citation2014; Koutrotsios et al., Citation2020). Such genera as Calocybe, Psilocybe, Agrocybe, Phellinus and others have all been proved to contain bioactive compounds and will need to be cultivated. Cultivation methods for most of these species will likely follow simple variations of those in use for commercially cultivated species saprotrophs. More focus also needs to be cast on termite mushrooms, some of which have shown appreciable content of bioactive compounds (Rouland-Lefèvre & Bignell, undated). Communities in Uganda are reported to already have used elementary methods to domesticate saprotrophic mushrooms, namely, Volvariella speciosa, Agaricus sp., Pleurotus sp., Agrocybe sp. and Termitomyces sp. (Wendiro et al., Citation2019). More scientific efforts have also been successfully pioneered in Burundi by Nteziryayo et al. (Citation2019) for Pleurotus citrinopileatus, Lentinus squarrosulus, Hypholoma fasciculare and Trametes polyzona using the general methods for commercially grown saprotrophic species. In similar experiments, however, Macrolepiota dolichaula and Laetiporus sulfurous failed to form sporocarps while three mycorrhizal species tested failed to even produce mother spawn, suggesting that different approaches have to be tried. Cultivation of those saprotrophic genera which have been domesticated elsewhere outside Africa having African cousins, such as Schizophyllum (Herawati et al., Citation2016), Ganoderma and others simply need to be commercially cultivated in Africa by adapting the proven methods (Thawthong et al., Citation2014).
While the available in-situ and ex-situ cultivation methods for mycorrhizal mushrooms need to be improved, yields and quality are still unpredictable. Furthermore, mycorrhizal mushroom production is still restricted to their climatic origins where availability is still subject to seasonality constraints (Peksen et al., Citation2013; Y. Zhang et al., Citation2014b). An alternative approach is finding new woody hosts for new mushroom production climatic zones. This approach would utilise the enzyme controlled host-switching mechanisms which were found by L.A. Lofgren et al. (Citation2021) for Larix species, the ancestral host of Suillus species. Through careful screening of suitable variants of current commercially and pharmacologically important species among genera Amanita, Russula, Cantharellus, Lactarius, Afroboletus, Boletus and other African mycorrhizal species, new hosts may be found outside provenances of these genera. Use of the seedling nursery cultivation method, which has not been widely used in Africa is another potentially good alternative.
A less explored approach for cultivating mycorrhizal mushrooms is “weaning” them off from their woody hosts with a view to growing them in lands formally designated for agriculture. Positive results of such an approach guarantees spread of geographical and temporal production conditions in fulfilling global food security goals. Successful off-host sporocarp production for Lyophyllum shimeji (Ohta, Citation1994), Laccaria bicolor (Kropp & Fortin, Citation1988) and Hebeloma cylindrosporum (Debaud & Gay, Citation1987) have provided strong prospects for possible domestication of mycorrhizal mushrooms. Furthermore, similar reports were made for Phlebopus portentotus (Kumla et al., Citation2012) although studies have shown this genus to have a possible facultative or non-mycorrhizal lifestyle (Nouhra et al., Citation2008). The “weaning-off” approach has to tackle the physiology of mycorrhizal mushroom cultivation by understanding the mushroom association complex levels, namely; the functional role played by MHB; the mycorrhizal species deficiency in crucial extracellular digestive enzymes, their preferred nitrogen sources and their dependence on glucose or fructose as the only carbon sources they can metabolize (Courty et al., Citation2010; Peksen et al., Citation2013). More research in the role played by MHB in sporocarp formation would give insights into how the MHB could be selected and screened for use in maximizing off-host sporocarp initiation. In Tricholoma matsutake, for example, Oh and Lim (Citation2018) found bacteria that were strongly associated with growth promotion of its mycelium from bacterial clades Dietzia, Ewingella, Pseudomonas, Paenibacillus, and Rodococcus. Furthermore, the finding by Bonfante and Anca (Citation2009) that MHB are also found in the sporocarp suggests that apart from their possible role in sporocarp initiation, MHB may continue to play a vital role in the physiology of sporocarp growth and development in an endosymbiotic association through small signaling molecules (Hazarika et al., Citation2020). If this is confirmed through systematic biochemical process studies, future work can determine suitable MHB species which may be co-opted into artificial growing substrates in off-host cultivation for mycorrhizal mushrooms.
The deficiency in enzymes such as endoglucanase, cellobiohydrolase, invertase and others involved in metabolism of complex carbohydrates in mycorrhizal species is one of the reasons for limited success in their cultivation on organic wastes (Mei et al., Citation2021). An in-depth molecular study of the order Boletales, which contains both saprotrophic and mycorrhizal species, could be used to resolve the critical differences between their mycorrhizal and non-mycorrhizal genets as a way of bridging the functional biochemical differences. By analyzing multi-host boletes (L. Lofgren et al., Citation2018) an even higher resolution could be obtained, giving more insights into which enzymes are redundant in sporocarp formation and development physiology for off-host mycorrhizal species cultivation approach. Alternatively, closer examination of mycorrhizal mushroom species host lifestyles may prove reversible as observed by L.A. Lofgren et al. (Citation2021), making it necessary to explore the possibility of environmental manipulation to switch on the genes involved in sporocarp development in the absence of the host species.
Reliance of mycorrhizal fungi on glucose or fructose as the carbon source necessary for sporocarp formation and development was intensively studied by Courty et al. (Citation2010). Such a specialist lifestyle necessitated mutualistic associations that evolved between the fungi and their woody hosts. In such associations, the woody host supplies the monosaccharide across the Hartig net interface prior to and during sporocarp development (Treseder et al., Citation2006). In this regard, one of the biggest barriers in attempting to cultivate mycorrhizal mushrooms in organic substrates is the inability of the mycorrhizal mycelium to hydrolyze lignocellulosic matter (Wolfe et al., Citation2012). This necessitates inclusion of either glucose or fructose in the basal substrate as the usable sources of carbon (Courty et al., Citation2010) and the appropriate metabolic nitrogen. In practice such nutritional conditions, however, also introduce the challenge of contamination. To eliminate possible contamination of basal growing material, it will be necessary to incorporate natural selective growth promoting substances, such as those normally produced by MHB like Streptomyces (auxofuran and antibiotics) under natural symbiotic conditions (Khalid & Keller, Citation2021) Alternatively or additionally, the incorporation of volatile organic compounds such as terpenes in growing substrates, which are known to be selective functional antimicrobials in natural mycorrhizospheres needs to be explored in the off-host approach (L.A. Lofgren et al., Citation2021). Through metagenomic studies of soil biota, quorum sensing molecules which might influence endorrhizospheric interactions were found (Chetia et al., Citation2019; Sessitsch et al., Citation2012). Such substances may also prove to be useful in sporocarp inducement if included in growing substrates for mycorrhizal mushrooms. Success of this approach would therefore require very strictly selective methods against some microbes while promoting others, a measure likely to be difficult to implement under greenhouse conditions (Khalid & Keller, Citation2021). In future there is great demand for stringent and systematic aseptic, and hence selective methods which need to be developed if the suggested approaches are to work.
In addition to all the foregoing constraints mainly of technological challenges in cultivating mycorrhizal and non-mycorrhizal mushrooms, quite a few challenges apply particularly in SSA countries. Marshall and Nair (Citation2009) specifically cite the lack of technical supportive policies, undeveloped technology, lack of suitable mushroom spawn, lack of locally adapted species and varieties, and low prices offered on the market. Higgins et al. (Citation2017) also observed the challenges of spawn availability, and post-harvest handling and transportation to the markets, also being serious constraints for the SSA mushroom industry. Hence, integrated efforts by researchers, commerce and government support will need to be applied to lessen these constraints in the future. The prospects of reigning in the vices of rampant unemployment and the rapidly increasing available labour and slow-growing industrial base in the region will thus provide the impetus for adoption and expansion of mushroom production sector and development of downstream industry. Although wide gaps still exist in the science of mushroom biology, quite a lot of knowledge has been accumulated over the years, as the literature shows. Hence mushroom cultivation has become more scientific since the last century. Additional literature sources have been recommended for more detailed reference where further details fell outside the scope of this review.
5. Conclusion
There is a wide disparity between the number of saprotrophic and mutualistic mushroom under cultivation with the latter lagging far behind the former. This is because the development of cultivation methods for mutualistic mushrooms (mycorrhizal and termitophilic species) has been very elusive owing to inadequate understanding of the intricate associations. While it has been easy to replicate the natural environmental growth requirements in mushroom houses for saprotrophic species, the same has only achieved adjusting suitable requirements for the plant hosts in the case of mycorrhizal mushrooms. It is also observed that within the successfully cultivated saprotrophic mushroom group, only a few species, notably in the Pleurotus and Agaricus genera, are cultivated in Africa unlike the vast array cultivated in Europe, Eurasia and the Americas. Such a huge gap may imply a slow adoption of technologies in Africa in this regard, lack of infrastructure or even some degree of mycophobia in the African region. With the rising need to increase food production and the potential income earnings from mushroom trade, Africa is poised to make its contribution in mushroom cultivation to fill the gap in world mushroom trade.
Disclosure statement
No potential conflict of interest was reported by the author(s).
Additional information
Funding
Notes on contributors
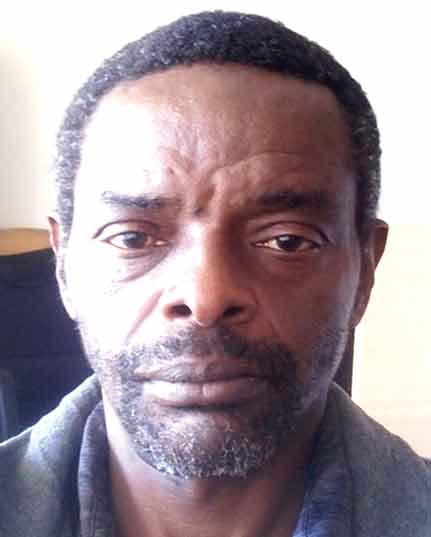
Alec Mlambo
The author is a researcher with interests in mushroom science, technologies and social aspects of mushroom gathering and consumption. Both MPhil and DPhil research work have focused largely on aspects of mycorrhizal mushrooms of the miombo woodlands of Southern Africa. This research is yet another contribution towards development of cultivation methods for African mushrooms. Interests also spread to modern mushroom cultivation status, medicinal mushroom exploitation and development of methods for their production using established and new low cost appropriate technologies. Peripheral interests cover research on African indigenous fruit and vegetable biodiversity for inclusion in national food policy. One of the researcher’s ultimate goals is to initiate and enhance cultivation of Southern African indigenous crop resources and expand the dietary resources in the region through collaborative efforts with regional agricultural research institutional and botanical databanks throughout the region.
References
- Abebe, W. (2021). Review on physiology of fungi. International Journal of Novel Research in Interdisciplinary Studies, 8, 40–24. https://www.noveltyjournals.com/upload/paper/REVIEW%20ON%20PHYSIOLOGY%20OF%20FUNGI.pdf
- Abon, M. D., Dulay, R. M. R., Kalaw, S. P., Romero-Roman, M. E., Arana-Vera, L. P., Reyes-Borja, W. O., & Reyes, R. G. (2020). Effects of culture media and physical factors on the mycelial growth of the three wild strains of Volvariella volvacea from Ecuador. Journal of Applied Biology & Biotechnology, 8, 60–63. https://doi.org/10.7324/JABB.2020.80610
- Afzal, R., Akram, A., Qureshi, R., Akram, Z., & Sultana, K. N. (2019). Efficacy of grain spawn and lime/gypsum ratio on mycelial growth of Oyster mushroom. Advances in Biotechnology & Microbiology, 13, 1–5. https://doi.org/10.19080/AIBM.2019.13.555871
- Agnolucci, M., Avio, L., Pepe, A., Turrini, A., Cristani, C., Bonini, P., Cirino, V., Colosimo, F., Ruzzi, M., & Giovannetti, M. (2019). Bacteria associated with a commercial mycorrhizal inoculum: Community composition and multifunctional activity as assessed by Illumina sequencing and culture-dependent tools. Frontiers in Plant Science, 9, 1–13. https://doi.org/10.3389/fpls.2018.01956
- Ashraf, J., Ali, M. A., Ahmad, W., Ayyub, C. M., & Shafi, J. (2013). Effect of different substrate supplements on Oyster mushroom (Pleurotus spp.) production. Food Science and Technology, 1(3), 44–51. https://doi.org/10.13189/fst.2013.010302
- Atikpo, M., Onokpise, O., Abazinge, M., Louime, C., Dzomeku, M., Boateng, L., & Awumbilla, B. (2008). Sustainable mushroom production in Africa: A case study in Ghana. African Journal of Biotechnology, 7(3), 249–253. http://www.academicjournals.org/AJB
- Ayanfunke, T. S. (2019). Training needs of mushroom (Agaricus biosporus) farmers in Oyo state, Nigeria. Journal of Agricultural Extension, 23. https://dx.doi.org/10.4314/jae.v23i3.8
- Baumgartner, K., Coetzee, M. P. A., & Hoffmeister, D. (2011). Secrets of the subterranean pathosystem of Armillaria. Molecular Plant Pathology, 12(6), 515–534. https://doi.org/10.1111/j.1364-3703.2010.00693.x
- Bedade, D. K., Singhal, R. S., Turunen, O., Deska, J., & Shamekh, S. (2016). Biochemical characterization of extracellular cellulase from Tuber maculatum mycelium produced under submerged fermentation. Applied Biochemistry and Biotechnology, 1–12. https://doi.org/10.1007/s12010-016-2248-8
- Beejan, P. H. F., & Nowbuth, R. D. (2010). Shiitake, a new mushroom variety proposed in Mauritius. University of Mauritius Research Journal, 16(1), 100–113
- Besufekad, Y., Mekonnen, A., Girma, B., Daniel, R., Tassema, G., Melkamu, J., Asefa, M., Fikiru, T., & Denboba, L. (2020). Selection of appropriate substrate for production of Oyster mushroom (Pleurotus ostreatus). Journal of Yeast and Fungal Research, 11(1), 15–25. https://doi.org/10.5897/JYFR2019.0187
- Biswas, D., & Biswas, J. (2017). Major deteriorative, pathogenic and beneficial fungi reported from various subterranean caves of the world: A mini review. International Journal of Ecosystem, 7, 11–16. https://doi.org/10.5923/j.ije.20170701.02
- Bloesch, U., & Mbago, F. (2008). The potential of wild edible mushrooms in the Miombo woodlands of the Selous-Niassa Wildlife Corridor for the livelihood improvement of the local population. Dar es Salaam: Ministry of Natural Resources and Tourism-Wildlife Division. Mushroom Study Selous Niassa Wildlife Corridor. Yumpu Publishing.
- Bonfante, P., & Anca, I. A. (2009). Plants, mycorrhizal fungi, and bacteria: A network of interactions. Annual Review of Microbiology, 63(1), 363–383. https://doi.org/10.1146/annurev.micro.091208.073504
- Borah, T. R., Singh, A. R., Paul, P., Talang, H., Kuamr, B., & Hazarika, S. (2019). Mushroom production for livelihood improvement Spawn production and mushroom cutlivation technology (pp. 46). ICAR Research Complex for NEH Region.
- Bruzos, M. M. (2019). The potential of mycorrhizal helper bacteria as PGPR. In H. B. Singh, B. K. Sarma, & C. Keswani (Eds.), Advances in PGPR Research. CAB International; (pp. 246–254). CABI.
- Büntgen, U., Čejka, T., Trnka, M., & Thomas, P. W. (2021). Rethinking a sustainable truffle sector under global crises. ERDKUNDE, 75(4), 311–314. https://doi.org/10.3112/erdkunde.2021.04.06
- Butler, G. M., & Wood, A. E. (1998). Effects of environmental factors on basidiome development in the resupinate polypore Phellinuscontiguous. Transaction of the British Mycological Society, 90(1), 75–83. https://doi.org/10.1016/S0007-1536(88)80182-7
- Capinha, C. (2018). Predicting the timing of ecological phenomena using dates of species occurrence records: A methodological approach and test case with mushrooms. International Journal Biometeorology, 63(8), 1–10. https://doi.org/10.1007/s00484-019-01714-0
- Chakwiya, A., Van der Linde, E. J., Chidamba, L., & Korsten, L. (2019). Diversity of cladobotryum mycophilum isolates associated with cobweb disease of agaricus bisporus in the South African mushroom industry. European Journal of Plant Pathology, 154(3), 767–776. https://doi.org/10.1007/s10658-019-01700-7
- Chang, S. T. (1972). The chinese mushroom (Volvariella volvacea): morphology, cytology, genetics, nutrition and cultivation. Chinese University Press;.
- Chang, S. T. (1977). The origin and early development of straw mushroom cultivation. Economic Botany, 31(3), 374–376. https://doi.org/10.1007/BF02866890
- Chang, S.-T., & Miles, P. G. (2004). Mushrooms: Cultivation, nutritional value, medicinal effect and environmental impact (2nd ed.). CRC Press;.
- Chen, Y., Sossah, F. L., Zhiwen, L., Yancong, L., Tian, L., Sun, X., Li, C., Song, B., & Li, Y. (2021). Effect of wheat bran and maize straw substrates on the agronomic traits and nutritional content of Auricularia cornea cv Yu Muer. Scientia Horticulturae, 286(2021), 1–7. 110200 0304-4238. https://doi.org/10.1016/j.scienta.2021.110200
- Cherfas, J. (1991). Disappearing mushrooms: Another mass extinction? Science, 254(5037), https://doi.org/10.1126/science.254.5037.1458
- Chetia, H., Kabiraj, D., Bharali, B., Ojha, S., Barkataki, M., Saikia, D., Singh, T., Mosahari, P. V., Sharma, P., & Bora, U. (2019). Exploring the benefits of endophytic fungi via omics. In B. P. Singh (Ed.), Advances in endophytic fungal research (pp. 51–81). Springer;.
- Chioza, A., & Ohga, S. (2014). Cultivated mushrooms in Malawi: A look at the present situation advances in microbiology, 4, 6–11. http://dx.doi.org/10.4236/aim.2014.41002
- Cho, Y. S., Kim, J. S., Crowley, D., & Cho, B. G. (2003). Growth promotion of the edible fungus Pleurotus ostreatus by fluorescent pseudomonads. FEMS Microbiology Letters, 218(2), 271–276. https://doi.org/10.1016/S0378-1097(02)01144-8
- Courty, P., Buée, M., Diedhiou, A. G., Frey-Klett, P., Tacon, F. L., Rineau, F., Turpault, M., Uroz, S., & Garbaye, J. (2010). The role of ectomycorrhizal communities in forest ecosystem processes: New perspectives and emerging concepts. Soil Biololgy and Biochemistry, 42, 679–698. https://doi.org/10.1016/j.soilbio.2009.12.006
- Crotty, F. V., & Adl, S. M. (2019). Competition and predation in soil fungivorous microarthropods using stable isotope ratio mass spectrometry. Frontiers in Microbiology, 10. https://doi.org/10.3389/fmicb.2019.01274
- Danell, E., & Camacho, F. J. (1997). Successful cultivation of the golden chanterelle-. Nature, 385(6614), 303. https://doi.org/10.1038/385303b0
- Debaud, J. C., & Gay, G. (1987). IN VITRO FRUITING UNDER CONTROLLED CONDITIONS OF THE ECTOMYCORRHIZAL FUNGUS HEBELOMA CYLINDROSPORUM ASSOCIATED WITH PINUS PINASTER. New Phytology, 105(3), 429–435. https://doi.org/10.1111/j.1469-8137.1987.tb00880.x
- De-miguel, S., Bonet, J. A., Pukkala, T., & de Aragón, J. M. (2014). Impact of forest management intensity on landscape-level mushroom productivity: A regional model-based scenario analysis. Forest Ecology and Management, 330, 218–227. https://doi.org/10.1016/j.foreco.2014.07.014
- Dey, R. C., Nasiruddin, K. M., Haque, M. S., & Al Munsur, M. A. Z. (2008). Production of oyster mushroom on different substrates using cylindrical block system. Progressive Agriculture, 19(1), 7–12. https://doi.org/10.3329/pa.v19i1.16983
- Díaz, G., Carrillo, C., & Honrubia, M. (2009). Production of Pinus halepensis seedlings inoculated with the edible fungus Lactarius deliciosus under nursery conditions. New Forests, 38(2), 215–227. https://doi.org/10.1007/s11056-009-9142-y
- Díaz-Godínez, G., & Téllez-Téllez, M. (2021). Mushrooms as edible foods. In X. Dai, M. Sharma, & J. Chen (Eds.), Fungi in sustainable food production (pp. 143–164). Springer.
- Dissasa, G. (2022). Cultivation of different oyster mushroom (Pleurotus species) on coffee waste and determination of their relative biological efficiency and pectinase enzyme production, Ethiopia. International Journal of Microbiology, 2022, 1–10. https://doi.org/10.1155/2022/5219939
- Domondon, D. L., He, W., De Kimpe, N., Höfte, M., & Poppe, J. (2004). b-Adenosine, a bioactive in grass chaff stimulating mushroom production. Phytochemistry, 65(2), 181–187. https://doi.org/10.1016/j.phytochem.2003.11.004
- Doornbos, R. F., van Loon, L. C., & Bakker, P. A. H. M. (2012). Impact of root exudates and plant defensesignaling on bacterial communities in the rhizosphere- A review. Agronomy for Sustainable Development, 32, 227–243. https://doi.org/10.1007/s13593-011-0028-y
- Dulal, S. (2019). An overview of mushroom farming. Conference Paper. January 2019. https://doi.org/10.13140/RG.2.2.28189.87526
- Edman, M., Hagos, S., & Carlsson, F. (2021). Warming effects on wood decomposition depend on fungal assembly history. Journal of Ecology, 109(4), 1919–1930. https://doi.org/10.1111/1365-2745.13617
- El-Nour, H. H. A., & Ibraheim, A. M. (2021). Effect of some organic applications on biological efficiency and productivity of mushroom (Pleurotus columbines) grown under uncontrolled conditions. Journal of Plant Production, 12(5), 495–503. https://doi.org/10.21608/jpp.2021.178923
- Endo, N., Gisusi, S., Fukuda, M., & Yamada, A. (2013). In-vitromycorrhization and acclimatization of Amanita caesareoides and its relatives on Pinusdensiflora. Mycorrhiza, 23(4), 303–315. https://doi.org/10.1007/s00572-012-0471-x
- Ferdousi, J., Al Riyadh, Z., Hossain, M. I., Saha, S. R., & Zakaria, M. (2019). Mushroom production benefits, status, challenges and opportunities in Bangladesh: A review. Ann. Res. & Rev. Biol, 34, 1–13. https://doi.org/10.9734/ARRB/2019/v34i630169
- Fernandes, T., Garrine, C., Ferrão, J., Bell, V., & Varzakas, T. (2021). Mushroom nutrition as preventative healthcare in Sub-Saharan Africa. Annual Research & Review in Biology, 34(6), 1–13. https://doi.org/10.3390/app11094221
- Fernandez-Conrad, P., Jactel, H., Robin, C., Tack, A. J. M., & Castagneyrol, B. (2018). Fungi reduce preference and performance of insect herbivores on challenged plants. Ecology, 99(2), 300–311. https://doi.org/10.1002/ecy.2044
- Ferraro, V., Venturella, G., Pecoraro, L., Gao, W., & Gargano, M. R. (2020). Cultivated mushrooms: Importance of a multipurpose crop, with special focus on Italian fungiculture. Plant Biosystems, 156(1), 130–142. https://doi.org/10.1080/11263504.2020.1837283
- Foley, M., & Yakushenko, V. (undated). Oyster mushroom cultivation: Substrate preparation and growing in pictures. Diperolehdaripada. https://www.zanaravo.comFocusEditorSpecialIssue-DrLamKuokChoy]
- Fortin, J. A., & Lamhamedi, M. S. (2009). Ecophysiology of sporocarp development of ectomycorrhizal basidiomycetes associated with boreal forest gymnosperms. In D. Khasa, Y. Piché, & A. P. Coughlan (Eds.), Advances in Mycorrhizal Science and Technology (pp. 161–172). NRC Research Press.
- Frey-Klett, P., Burlinson, P., Deveau, A., Barret, M., Tarkka, M., & Sarniguet, A. (2011). Bacterial-fungal interactions, hyphens between agricultural, clinical, environmental, and food microbiologists. Microbiology and Molecular Biology Reviews, 75(4), 583–609. https://doi.org/10.1128/MMBR.00020-11
- Fufa, B. K., Tadesse, B. A., & Tulu, M. M. (2021). Cultivation of Pleurotus ostreatus on agricultural waste and their combination. Research Square Preprints. https://doi.org/10.21203/rs.3.rs-418176/v1
- Gao, S., Huang, Z., Feng, X., Bian, Y., Huang, W., & Liu, Y. (2020). Bioconversion of rice straw agro-residues by Lentinula edodes and evaluation of non-volatile taste compounds in mushrooms. Scientific Reports, 10(1), 1814. https://doi.org/10.1038/s41598-020-58778-x
- García-Montero, L. G., Pascual, C., García-Abril, A., & García-Cañete, J. (2007). Problems of using rockroses in Tuber melanosporum culture: Soil and truffle harvest associated with Cistus laurifolius. Agroforestry Systems, 70(3), 251–258. https://doi.org/10.1007/s10457-007-9053-x
- Gateri, M. W., Muriuki, A. W., Waiganjo, M. W., & Ngeli, P. 2009. Cultivation and commercialization of edible mushrooms in Kenya: A review of prospects and challenges for smallholder production. Acta Hort 806. https://doi.org/10.17660/ActaHortic.2009.806.59
- Gayley, D. (1938). Experimental spawn and mushroom culture II artificial composts. Annals of Applied Biology, 25(2), 322–340. https://doi.org/10.1111/j.1744-7348.1938.tb02337.x
- Gea, F. J., Navarro, M. J., Santos, M., Diánez, F., & Carrasco, J. (2021). Control of fungal diseases in mushroom crops while dealing with fungicide resistance: A Review. Microorganisms, 9(3), 585. https://doi.org/10.3390/microorganisms9030585
- Ghimire, A., Pandey, K. R., Joshi, Y. R., & Subedi, S. (2021). Major fungal contaminants of mushrooms and their management. International Journal of Applied Sciences and Biotechnology, 9(2), 80–93. https://doi.org/10.3126/ijasbt.v9i2.37513
- Godbout, C., & Fortin, J. A. (1990). Cultural control of basidiome formation in Laccariabicolor in container-grown white pine seedlings. Mycological Research, 94(8), 1051–1058. https://doi.org/10.1016/S0953-7562(09)81332-4
- Gorka, S., Mayerhofer, W., Dietrich, M., Gabriel, R., Wiesenbauer, J., Martin, V., Schreiber, P., Woebken, D., Richter, A., & Kaiser, C. (2017). The effect of local ectomycorrhizal nitrogen supply on allocation of recent photosynthates within the mycorrhizosphere. ui.adsabs.harvard.edu. Accessed 23 January 2022. https://meetingorganizer.copernicus.org/EGU2017/EGU2017-15917-1.pdf
- Gowda, N., & Kumaran, G. S. (2014). Design and development of a hot water paddy straw pasteurizer for mushroom cultivation. Agricultural Mechanization in Asia, Africa & Latin America (AMA), 45(2), 11–18. https://www.researchgate.net/publication/285514646
- Gowda, N., & Pandey, M. (2014). Performance evaluation of developed paddy straw pasteurizer for mushroom cultivation. Agricultural Mechanization in Asia, Africa & Latin America (AMA), 45(3), 28–36. https://www.researchgate.net/publication/293173966
- Grimm, A., Eilertsen, L., Chen, F., Huang, R., Atterhem, L., & Xiong, S. (2021b). Cultivation of Pleurotus ostreatus mushroom on substrates made of cellulose Fibre rejects: product quality and spent substrate fuel properties. Waste and Biomass Valorization, 12(8), 4331–4340. https://doi.org/10.1007/s12649-020-01311-y
- Grimm, D., Kuenz, A., & Rahmann, G. (2021a). Integration of mushroom production into circular food chains. Organic Agriculture, 11(4), 309–317. https://doi.org/10.1007/s13165-020-00318-y
- Grossart, H.-P., Van den Wyngaert, S., Kagami, M., Wurzbacher, C., Cunliffe, M., & Rojas-Jimenez, K. (2019). Fungi in aquatic ecosystems. Nature Reviews. Microbiology, 17(6), 339–354. accessed 090821. https://doi.org/10.1038/s41579-019-0175-8
- Gruen, H. E. (1964). Mushroom growing in Japan. Boston Mycological Club Bull, 1, 1–5.
- Gründemann, C., Reinhardt, J. K., & Lindequist, U. (2019). European medicinal mushrooms: Do they have potential for medicine-An update. Phytomedicine, 66. https://doi.org/10.1016/j.phymed.2019.153131
- Hall, I., Fitzpatrick, N., Miros, P., & Zambonelli, A. (2017a). Counter-season cultivation of truffles in the Southern Hemisphere: An update. Italian Journalof Mycology, 46, 21–36. https://doi.org/10.6092/issn.2531-7342/6794
- Hall, J. S., Harris, D. J., Medjib, V., & Ashtona, P. M. S. (2003). The effects of selective logging on forest structure and tree species composition in a Central African forest: Implications for management of conservation areas. Forest Ecology and Management, 183(1–3), 249–264. https://doi.org/10.1016/S0378-1127(03)00107-5
- Harith, N., Abdullah, N., & Sabaratnam, V. (2014). Cultivation of Flammulina velutipes mushroom using various agro residues as a fruiting substrate. Pesq. Agropec. Bras., Brasília, 49(3), 181–188. https://doi.org/10.1590/S0100-204X2014000300004
- Hawksworth, D. L., & Lücking, R. (2017). Fungal diversity revisited: 2.2 to 3.8 million species. Microbiology Spectrum, 5(4), 1–17. https://doi.org/10.1128/microbiolspec.FUNK-0052-2016
- Hazarika, D. J., Gautom, T., Parveen, A., Goswami, G., Barooah, M., Modi, M. K., & Boro, R. C. (2020). Mechanism of interaction of an endofungal bacterium Serratia marcescens D1 with its host and non-host fungi. PLoS ONE, 15(4), 1–19. https://doi.org/10.1371/journal.pone.0224051
- Herawati, E., Arung, E. T., & Amirta, R. (2016). Domestication and nutrient analysis of schizopyllumcommune,alternative natural food sources in East Kalimantan agriculture and agricultural science procedia 9: 291–296 International Conference on Food, Agriculture and Natural Resources. Elsevier B.V. publishers.
- Hibbett, D. S., Gilbert, L., & Donoghue, M. J. (2000). Evolutionary instability of ectomycorrhizal symbioses in basidiomycetes. Nature, 407(6803), 506–508. https://doi.org/10.1038/35035065
- Higgins, C., Margot, H., Warnquist, S., Obeysekare, E., & Mehta, K. (2017). Mushroom cultivation in the developing world: A comparison of cultivation technologies. IEE Global Humanitarian Technology Conference (GHTC): 1–7. San Jose, CA, USA: IEEE
- Hsu, C. M., Hameed, K., Cotter, V. T., & Liao, H. L. (2018). Isolation of mother cultures and preparation of spawn for oyster mushroom cultivation. http://edis.ifas.ufl.edu. Accessed 11 November 2021
- Joseph, T. P., Chanda, W., Padhiar, A. A., Batool, S., LiQun, S., Zhong, M., & Huang, M. (2018). A preclinical evaluation of the antitumor activities of edible and medicinal mushrooms: A mol. insight. Integrative Cancer Therapies, 17(2), 200–209. https://doi.org/10.1177/1534735417736861
- Jurak, E., Punt, A. M., Arts, W., Kabel, M. A., & Gruppen, H. (2015). Fate of carbohydrates and lignin during composting and mycelium growth of Agaricus bisporus on wheat straw based compost. PLoS One, 10(10), e0138909. https://doi.org/10.1371/journal.pone.0138909
- Kabel, M. A., Jurak, E., Mäkelä, M. R., & de Vries, R. P. (2017). Occurrence and function of enzymes for lignocellulose degradation in commercial Agaricus bisporus cultivation. Applied Microbiology and Biotechnology, 101(11), 4363–4369. https://doi.org/10.1007/s00253-017-8294-5
- Kavi, R. K., Bugyei, K. A., Obeng-Koranteng, G., & Folitse, B. Y. (2018). Assessing sources of information for urban mushroom growers in Accra, Ghana. Journal of Agriculture and Food Information, 19(2), 176–191. https://doi.org/10.1080/10496505.2017.1361328
- Kazige, O. K., Chuma, G. B., Lusambya, A. S., Mondo, J. M., Balezi, A. Z., Mapatano, S., & Mushagalusa, G. N. (2022). Valorizing staple crop residues through mushroom production to improve food security in eastern Democratic Republic Of Congo. Journal of Agricultural Food Research, 8(2022), 100285. https://doi.org/10.1016/j.jafr.2022.100285
- Kertesz, M. A., & Thai, M. (2018). Compost bacteria and fungi that influence growth and development of Agaricus bisporus and other commercial mushrooms. Applied Microbiology and Biotechnology, 102(4), 1639–1650. https://doi.org/10.1007/s00253-018-8777-z
- Khalid, S., & Keller, N. P. (2021). Chemical signals driving bacterial–fungal interactions. Environmental Microbiology, 23(3), 1334–1347. https://doi.org/10.1111/1462-2920.15410
- Khare, K. B., Khonga, E. B., Jongman, M., & Leteane, M. (2015). Economic profitability of oyster mushroom production in Botswana. Mushroom Research, 24(1), 49–55.
- Kinge, T. R., Nji, T. M., Ndam, L. M., & Mih, A. M. (2014). Mushroom research, production and marketing in Cameroon: A review. Issues in Biol. Sc. Pharm. Res, 2(7), 069–074. http://www.journalissues.org/IBSPR/
- Kortei, N. K., Odamtten, G. T., Obodai, M., Wiafe-Kwagyan, M., & Mensah, D. L. N. (2018). Correlations of cap diameter (pileus width), stipe length and biological efficiency of Pleurotus ostreatus(Ex.Fr.) Kummer cultivated on gamma-irradiated and steam-sterilized composted sawdust as an index of quality for pricing. Journal of Agriculture & Food Security, 7(35), 1–8. https://doi.org/10.1186/s40066-018-0185-1
- Koutrotsios, G., Danezis, G., Georgiou, C., & Zervakis, G. I. (2020). Elemental content in Pleurotus ostreatus and Cyclocybe cylindracea mushrooms: Correlations with concentrations in cultivation substrates and effects on the production process. Molecules, 25(9), 2179. https://doi.org/10.3390/molecules25092179
- Kropp, B. R., & Fortin, J. A. (1988). The incompatibility system and relative ectomycorrhizal performance of monokaryons and reconstituted dikaryons of Laccaria bicolor. Canadian Journal of Botany, 66(2), 289–394. https://doi.org/10.1139/b88-047
- Kuga-Uetake, Y., Purich, M., Massicotte, H. B., & Peterson, R. L. (2004). Host microtubules in the Hartig net region of ectomycorrhizas, ectendomycorrhizas, and monotropoid mycorrhizas. Canadian Journal of Botany, 82(7), 938–946. https://doi.org/10.1139/b04-085
- Kumla, J., Bussaban, B., Suwannarach, N., Lumyong, S., & Danell, E. (2012). Basidiome formation of an edible wild, putatively ectomycorrhizal fungus, Phlebopus portentosus without host plant. Mycologia, 104(3), 597–603. https://doi.org/10.3852/11-074
- Liu, S., Zhang, W., & Kuang, Y. (2018). Production of stalk spawn of an edible mushroom (Pleurotus ostreatus) in liquid culture as a suitable substitute for stick spawn in mushroom cultivation. Scientia Horticulturae, 240(2018), 572–577. https://doi.org/10.1016/j.scienta.2018.06.068
- Lofgren, L., Nguyen, N. H., & Kennedy, P. G. (2018). Ectomycorrhizal host specificity in a changing world: Can legacy effects explain anomalous current associations? New Phytologist, 220(4), 1273–1284. https://doi.org/10.1111/nph.15008
- Lofgren, L. A., Nguyen, N. H., Vilgalys, R., Ruytinx, J., Liao, H.-L., Branco, S., Kuo, A., La Butti, K., Lipzen, A., Andreopoulos, W., Pangilinan, J., Riley, R., Hundley, H., Na, H., Barry, K., Grigoriev, I. V., Stajich, J. E., & Kennedy, P. G. (2021). Comparative genomics reveals dynamic genome evolution in host specialist ectomycorrhizal fungi. New Phytologist, 230(2), 774–792. https://doi.org/10.1111/nph.17160
- Looney, B., Miyauchi, S., Morin, E., Drula, E., Courty, P. E., Kohler, A., Lindquist, E., Kuo, A., LaButti, K., Pangilinan, J., Lipzen, A., Riley, R., Andreopoulos, W., He, G., Johnson, J., Barry, K. W., Grigoriev, I. V., Nagy, L. G., Hibbett, D., … Martin, F. M. (2021). Evolutionary priming and transition to the ectomycorrhizal habit in an iconic lineage of mushroom-forming fungi: Is preadaptation a requirement? bioRxiv Preprint. version posted February 24, 2021 https://doi.org/10.1101/2021.02.23.432530
- Mabuza, M. L., Ortmann, G. F., & Wale, E. Z. (2012). Collective action in commercial mushroom production: The role of social capital in the management of informal farmer groups in Swaziland. Selected paper for presentation at the International Association of Agricultural Economists (IAAE) Triennial Conference, Foz do Iquaçu, Brazil, 18-24 August, 2012. IAAE.
- Magae, Y. (1999). Saponin stimulates fruiting of the edible basidiomycete Pleurotus ostreatus. Bioscience, Biotechnology, and Biochemistry, 63(10), 1840–1842. https://doi.org/10.1271/bbb.63.1840
- Magingo, F. S., Oriyo, N. M., Kivaisi, A. K., & Danell, E. (2004). Cultivation of Oudemansiella tanzanica nom. prov. on agricultural solid wastes in Tanzania. Mycologia, 96(2), 197–204. https://doi.org/10.1080/15572536.2005.11832967
- Mahadevan, K., & Shanmugasundaram, K. (2018). Comparative effect of different culture media on mycelial growth performance of Pleurotussapidus. Journal of Pharmacognosy and Phytochemistry, 7(4), 874–878. https://www.phytojournal.com/archives/2018/vol7issue4/PartO/7-3-733-395.pdf
- Marshall, E., & Nair, N. G. (Eds.) (2009). (Tan) Make money by growing mushrooms: FAO Diversification booklet number 7. Rural Infrastructure and Agro-Industries Division Food and Agriculture Organization of the United Nations Rome 2009. Rome, Italy: FAO.
- Martínez-Peña, F., De-miguel, S., Pukkala, T., Bonet, J. A., Ortega-Martínez, P., Aldea, J., & de Aragón, J. M. (2012). Yield models for ectomycorrhizal mushrooms in Pinus sylvestris forests with special focus on Boletus edulis and Lactarius group deliciosus. Forest Ecology and Management, 282, 63–69. https://doi.org/10.1016/j.foreco.2012.06.034
- Marupakula, S., Mahmood, S., Jernberg, J., Nallanchakravarthula, S., Fahad, Z. A., & Finlay, R. D. (2017). Bacterial microbiomes of individual ectomycorrhizal Pinus sylvestris roots are shaped by soil horizon and differentially sensitive to nitrogen addition. Environmental Microbiology, 19(11), 4736–4753. https://doi.org/10.1111/1462-2920.13939
- Masevhe, M. R., Soundy, P., & Taylor, N. J. (2016). Alternative substrates for cultivating oyster mushrooms (Pleurotus ostreatus). South African Journal of Plant and Soil, 33(2), 97–103. https://doi.org/10.1080/02571862.2015.1079932
- Matjuðkova, N., Okmane, L., Zaïâ, D., Rozenfelde, L., Puíe, M., Krûma, I., Vederòikovs, N., & Rapoport, A. (2018). Effect of lignin-containing media on growth of medicinal mushroom Lentinulaedodes. Proceedings of the Latvian Academy of Sciences, Section B, 71(1/2 (706/707)), 38–42. https://doi.org/10.1515/prolas-2017-0007
- Mediavilla, O., Olaizola, J., Santos-del-Blanco, L., Oria-de-rueda, J. A., & Martín-Pinto, P. (2015). Mycorrhization between Cistus ladanifer L. and Boletus edulis Bull is enhanced by the mycorrhiza helper bacteria Pseudomonas fluorescensMigula. Mycorrhiza, 26(2), 1–8. https://doi.org/10.1007/s00572-015-0657-0
- Mei, Y., Liu, C.-Y., Li, S.-H., Guerin-Laguette, A., Xiao, Y.-J., Tang, P., Wan, S.-P., Bonito, G., & Wang, Y. (2021). Phlebopus roseus, a new edible bolete from China, is associated with insects and plants. Mycologia, 113(1), 33–42. https://doi.org/10.1080/00275514.2020.1816781
- Miller, O. K. (2003). The Gomphidiaceae revisited: A worldwide perspective. Mycologia, 95(1), 176–183. https://doi.org/10.1080/15572536.2004.11833147
- Mlambo, A., & Maphosa, M. (2017). Miombo woodland mushrooms of commercial food value: A survey of central districts of Zimbabwe. Journal of Food Security, 5(2), 51–57. https://doi.org/10.12691/jfs-5-2-5
- Mlambo, A., & Maphosa, M. (2021). Ectomycorrhizal mushroom yield association with woody species and leaf litter in a miombo woodland, central Zimbabwe. African Journal of Ecology and Ecosystems, 8(8), 1–10.
- Morte, A., Kagan-Zur, V., Navarro-Ródenas, A., & Sitrit, Y. (2021). Cultivation of desert truffles—A crop suitable for arid and semi-arid zones. Agronomy, 11(8), 1462. https://doi.org/10.3390/agronomy11081462
- Mshandete, A. M., & Cuff, J. (2008). Cultivation of three types of indigenous wild edible mushrooms: Coprinus cinereus, Pleurotus flabellatus and Volvariella volvocea on composted sisal decortications residue in Tanzania. African Journal of Biotechnology, 7(24), 4551–4562. http://www.academicjournals.org/AJB
- Muchena, F. B., Pisa, C., Mutetwa, M., Govera, C., & Ngezimana, W. (2021). Effect of spent button mushroom substrate on yield and quality of Baby Spinach (Spinacia oleracea). International Journal of Agronomy, 2021, 1–9. https://doi.org/10.1155/2021/6671647
- Muswati, C., Simango, K., Tapfumaneyi, L., Mutetwa, M., & Ngezimana, W. (2021). The effects of different substrate combinations on growth and yield of oyster mushroom (Pleurotus ostreatus).International Journal of Agronomy, 2021, 1–10. Article ID 9962285. https://doi.org/10.1155/2021/9962285
- Mwinyi, W. Y., Héritier, M. K., & Hippolyte, N. S. W. M. (2022). Spawn production and cultivation of two local edible fungal species in Kisangani (DRC) (case of Pleurotus tuber-regium and Lentinus squarrosulus). Journal of Microbiology Experiments, 10(1), 45‒48. https://doi.org/10.15406/jmen.2022.10.00351
- Nannipieri, P., Giagnoni, L., Landi, L., & Renella, G. (2011). Role of phosphatase enzymes in soil. In E. Bunemann, A. Oberson, & E. Frossard (Eds.), Phosphorus in Action. Soil Biology 26 (pp. 215–243). Springer.
- Naranjo-Ortiz, M. A., & Gabaldón, T. (2019). Fungal evolution: Diversity, taxonomy and phylogeny of the Fungi. Biology Reviews, 94(6), 2101–2137. https://doi.org/10.1111/brv.12550
- Ndem, J. U., & Oku, M. O. 2016. Mushroom production for food security in Nigeria. Food Science Quality Management, 48 2225-0557, 44–50. www.iiste.org 2224-6088 (Paper)
- Ngétich, O. K., Nyamangyoku, O. I., Rono, J. J., Niyokuri, A. N., & Izamuhaye, J. C. (2013). Relative performance of oyster mushroom (Pleurotus Florida) on agro-industrial and agricultural substrate. International Journal of Agronomy and Plant Production, 4(1), 109–116. https://www.cabdirect.org/cabdirect/abstract/20133059495
- Noble, R., & Gaze, R. H. (1996). Preparation of mushroom (Agaricus bisporus) composts in controlled environments: Factors influencing compost bulk density and productivity. International-Biodeterioration-and-Biodegradation, 37(1–2), 93–100. https://doi.org/10.1016/0964-8305(95)00072-0
- Noble, R., Hobbs, P. J., Mead, A., & Dobrovin-Pennington, A. (2002). Influence of straw types and nitrogen sources on mushroom composting emissions and compost productivity. Journal of Industrial Microbiology & Biotechnology, 29(3), 99–110. https://doi.org/10.1038/sj.jim.7000292
- Norvell, L. (1995). Loving the chanterelle to death? The 10-year Oregon chanterelle project. McIIvanea, 12(1), 5–25. https://www.researchgate.net/publication/255719162_Loving_the_chanterelle_to_death_The_ten-year_Oregon_Chanterelle_Project
- Nouhra, E., Urcelay, C., Becerra, A., & Dominguez, L. (2008). Mycorrhizal status of Phlebopus bruchii (Boletaceae): Does it form ectomycorrhizas with Fagara coco (Rutaceae)? Symbiosis, 46, 113–120. https://www.researchgate.net/publication/260258789_Mycorrhizal_status_of_Phlebopus_bruchii_Boletaceae_Does_it_form_ectomycorrhizas_with_Fagara_coco_Rutaceae
- Nteziryayo, V., Tibuhwa, D. D., Kiyuku, P., Muvunyi, R., & Masharabu, T. (2019). Characterization and domestication of wild edible mushrooms from selected indigenous forests in Burundi. Tanzania Journal of Science, 45(3), 417–430. https://www.ajol.info/index.php/tjs/article/view/191719/180876
- Obase, K. (2020). Effects of bacterial strains isolated from the ectomycorrhizal roots of Laccaria parva on sporocarp production by the fungus in vitro. Mycoscience, 61(1), 9–15. https://doi.org/10.1016/j.myc.2019.10.002
- Oh, S.-Y., & Lim, Y. W. (2018). Effect of fairy ring bacteria on the growth of Tricholoma matsutake in vitro culture. Mycorrhiza, 28(5–6), 411–419. https://doi.org/10.1007/s00572-018-0828-x
- Ohta, A. (1994). Production of fruit bodies of a mycorrhizal fungus, Lyophyllum shimeji in pure culture. Mycoscience, 35(2), 147–151. https://doi.org/10.1007/BF02318492
- Onwubuya, E. A., Ajani, E. N., Dike, C., & Uzokwe, U. N. (2015). Popularization of mushroom production technologies among small-scale farmers in Abia State, Nigeria. International Journal of Research in Agriculture and Forestry, 2(1), 1–7. https://www.ijraf.org/pdf/v2-i1/1.pdf
- Osarenkhoe, O. O., John, O. A., & Theophilus, D. A. (2014). Ethnomycological Conspectus of West African Mushrooms: An Awareness Document. Advances in Microbiology, 4(1), 39–54. https://doi.org/10.4236/aim.2014.41008
- Pavlík, M., & Byandusya, P. (2016). The effectiveness of the oyster mushroom growing on the locally available substrates in rural regions of Africa and Europe. Science and Cultivation of Edible Fungi, Baars & Sonnenberg (pp.1-6). International Society for Mushroom Science. https://www.researchgate.net/publication/305639301
- Payen, T., Murat, C., & Bonito, G. (2014). Truffle phylogenomics: New insights into truffle evolution and truffle life cycle. In F. Martin (Ed.), Genomics of plant-related fungi. Advances in Botanical Research (pp. 211–234). Elsevier Academic Press.
- Peksen, A., Kibar, B., & Yakupoglu, G. (2013). Favourable culture conditions for mycelial growth of Hydnumrepandum, a medicinal mushroom. African Journal of Traditional & Complimentary Altitude Medicine, 10(6), 431–434. https://doi.org/10.4314/ajtcam.v10i6.4
- Pérez-Moreno, J., Guerin-Laguette, A., Rinaldi, A. C., Yu, F., Verbeken, A., Hernández-Santiago, F., & Martínez-Reyes, M. (2021). Edible mycorrhizal fungi of the world: What is their role in forest sustainability, food security, biocultural conservation and climate change? Plants People Planet, 00, 1–20. https://doi.org/10.1002/ppp3.10199
- Piazza, S. D., Benvenuti, M., Damonte, G., Cecchi, G., Mariotti, M. G., & Zotti, M. (2021). Fungi and circular economy: Pleurotus ostreatus grown on a substrate with agricultural waste of Lavender, and its promising biochemical profile. Recycling, 6(2), 1–12. https://doi.org/10.3390/recycling6020040
- Pilz, D., & Molina, R. (2001). Commercial harvests of edible mushrooms from the forests of the Pacific Northwest United States: Issues, management, and monitoring for sustainability. Forest Ecology & Management, 5593, 1–14. https://doi.org/10.1016/S0378-1127(01)00543-6
- Poonga, P. R. J., & Kaviyarasan, V. (2015). Basidiome Initiation in medicinal mushroom Hypsizygus ulmarius by free living nitrogen fixing Azotobacter sp. International Journal of Pharmaceutical Sciences Review and Research, 31(15), 85–88. https://www.researchgate.net/publication/275230236
- Production of fruit bodies of a mycorrhizal fungus, Lyophyllum shimeji in pure culture. 4, 39–54. http://dx.doi.org/10.4236/aim.2014.41008
- Qin, J., & Feng, B. (2022). Life cycle and phylogeography of true truffles. Genes, 13(145), 1–10. https://doi.org/10.3390/genes13010145
- Rainey, P. B., Cole, A. L. J., Fermor, T. R., & Wood, D. A. (1990). A model system for examining involvement of bacteria in basidiome initiation of Agaricus bisporus. Mycological Research, 94(2), 191–195. https://doi.org/10.1016/S0953-7562(09)80612-6
- Rashad, F. M., El Kattan, M. H., Fathy, H. M., Abd El-Fattah, D. A., El Tohamy, M., & Farahat, A. A. (2019). Recycling of agro-wastes for Ganoderma lucidum mushroom production and Ganoderma post mushroom substrate as soil amendment. Waste Management, 88, 147–159. https://doi.org/10.1016/j.wasman.2019.03.040
- Rathod, M. G., Gadade, R. B., Thakur, G. M., & Pathak, A. P. 2021. Oyster mushroom: Cultivation, bioactive significance and commercial status. Frontiers in Life Sciences 2. https://www.bhumipublishing.com/book/
- Rigamonte, T. A., Pylro, V. S., & Duarte, G. F. (2019). The role of mycorrhization helper bacteria in the establishment and action of ectomycorrhizae associations. Brazilian Journal of Microbiology, 41(4), 832–840. https://doi.org/10.1590/S1517-83822010000400002
- Riquelme, M., Aguirre, J., Bartnicki-García, S., Braus, G. H., Feldbrügge, M., Fleig, U., Hansberg, W., Herrera-Estrella, A., Kämper, J., Kück, U., Mouriño-Pérez, R. R., Takeshita, N., & Fischer, R. (2018). Fungal morphogenesis, from the polarized growth of hyphae to complex reproduction and infection structures. Microbiology & Molecular Biology Reviews, 82(2), 1–47. https://doi.org/10.1128/MMBR.00068-17
- Rizzo, G., Goggi, S., Giampieri, F., & Baroni, L. (2021). A review of mushrooms in human nutrition and health. Trends in Food Science &technology???, 117, 60–73. https://doi.org/10.1016/j.tifs.2020.12.025
- Royse, D. J., Baars, J., & Tan, Q. (2017). Current overview of mushroom production in the world. In C. Z. Diego & A. Pardo-Giménez (Eds.), Edible and Medicinal Mushrooms: Technology and Applications (pp. 5–13). John Wiley & Sons Ltd.
- Royse, D. J., & Beelman, R. B. (2016). Six steps to mushroom farming. https://extension.psu.edu/six-steps-to-mushroom-farming Accessed 13 November 2021
- Salo, K., Domisch, T., & Kouki, J. (2019). Forest wildfire and 12 years of post-disturbance succession of saprotrophic macrofungi (Basidiomycota, Ascomycota). Forest Ecology and Management, 451(2019), 1–29. https://doi.org/10.1016/j.foreco.2019.117454
- Sambyal, K., & Singh, R. V. (2021). A comprehensive review on Morchella importuna: Cultivation aspects, phytochemistry, and other significant applications. Folia Microbiology, 66(2), 147–157. https://doi.org/10.1007/s12223-020-00849-7
- Sessitsch, A., Hardoim, P., Döring, J., Weilharter, A., Krause, A., Woyke, T., & Reinhold-Hurek, B. (2012). Functional Characteristics of an Endophyte Community Colonizing Rice Roots as Revealed by Metagenomic Analysis. Molecular & Plant-Microbe Interactions, 25(1), 28–36. https://doi.org/10.1094/MPMI-08-11-0204
- Sharma, M., & Sharma, R. (2016). Drugs and drug intermediates from fungi: Striving for greener processes. Critical Reviews in Microbiology, 42(2), 322–338. https://doi.org/10.3109/1040841X.2014.947240
- Shungchang, J., Mark, M., & Qi, T. (2019). The 86th Anniversary of the Invention of Grain Spawn:Witnessing the modernization of commercial cultivation of the mushroom Agaricus bisporus. Acta Edulis Fungi, 26(1), 77–98. https://www.cabdirect.org/cabdirect/abstract/20193278412
- Smith, G. R., Finlay, R. D., Stenlid, J., Vasaitis, R., & Menkis, A. (2017). Growing evidence for facultative biotrophy in saprotrophic fungi: Data from microcosm tests with 201 species of wood-decay basidiomycetes. New Phytologist, 215(2), 747–755. https://doi.org/10.1111/nph.14551
- Stobbe, U., Egli, S., Tegel, W., Peter, M., Sproll, L., & Büntgen, U. (2013). Potential and limitations of Burgundy truffle cultivation. Applied Microbiology and Biotechnology, 97(12), 5215–5224. https://doi.org/10.1007/s00253-013-4956-0
- Straatsma, G., Gerrits, J. P. G., Thissen, J. T. N. M., Amsing, J. G. M., Loeffen, H., & van Griensven, L. J. L. D. (2000). Adjustment of the composting process for mushroom cultivation based on initial substrate composition. Bioresearch Technology, 72(1), 67–74. https://doi.org/10.1016/S0960-8524(99)00088-7
- Sugimoto, H. H., Barbosa, A. M., Dekker, R. F. K., & Castro-Gomez, R. J. (2001). Veratryl alcohol stimulates fruiting body formation in the oyster mushroom, Pleurotus ostreatus. FEMS Microbiology Letters, 194(2), 235–238. https://doi.org/10.1111/j.1574-6968.2001.tb09475.x
- Tedersoo, L., May, T. W., & Smith, M. E. (2010). Ectomycorrhizal lifestyle in fungi: Global diversity, distribution, and evolution of phylogenetic lineages. Mycorrhiza, 20(20104), 217–263. https://doi.org/10.1007/s00572-009-0274-x
- Thawthong, A., Karunarathna, S. C., Thongklang, N., Chukeatirote, E., Kakumyan, P., Chamyuang, S., Rizal, L. M., Mortimer, P. E., Xu, J., Callac, P., & Hyde, K. D. (2014). Discovering and domesticating wild tropical cultivatable mushrooms. Chiang Mai Journal of Science, 41(4), 731–764. https://www.researchgate.net/publication/266022771_Discovering_and_Domesticating_Wild_Tropical_Cultivatable_Mushrooms
- Thiribhuvanamala, G., Krishnamoorthy, A. S., Kavitha, C., Shwet, K., Anil, K., & Sharma, V. P. (2021). Strategic approaches for outdoor cultivation of paddy straw mushroom (Volvariella volvacea) as intercrop under different cropping systems. Madras Agricultural Journal, 108, 71–78. https://doi.org/10.29321/MAJ.10.000486
- Thongklang, N., & Luangharn, T. (2016). Testing agricultural wastes for the production of Pleurotus ostreatus. Mycosphere, 7(6), 766–772. https://doi.org/10.5943/mycosphere/7/6/6
- Thuc, L. V., Corales, R. G., Sajor, J. T., Truc, N. T. T., Hien, P. H., Ramos, R. E., Bautista, E., Tado, C. J. M., Ompad, V., Son, D. T., & Hung, N. V. (2020). Rice-straw mushroom production. M. Gummert, N. Van Hung, P. Chivenge, & B. Douthwaite Eds., Sustainable rice straw management, Springer Open (pp 93-109). https://doi.org/10.1007/978-3-030-32373-8
- Treseder, K., Torn, M., & Masiello, C. (2006). An ecosystem-scale radiocarbon tracer to test use of litter carbon by ectomycorrhizal fungi. Soil Biology & Biochemistry, 38(5), 1077–1082. https://doi.org/10.1016/j.soilbio.2005.09.006
- Upadhyay, R. C., & Hofrichter, M. (1993). Effect of phenol on the mycelia growth and fructification in some of basidiomycetous fungi. Journal of Basic Microbiology, 33(5), 343–347. https://doi.org/10.1002/jobm.3620330512
- Vaario, L.-M., & Matsushita, N. (2021). Conservation of edible ectomycorrhizal mushrooms: Understanding of the ECM fungi mediated carbon and nitrogen movement within forest ecosystems. book. T. Ohyama & K. Inubushi Eds., Nitrogen in Agriculture - Physiological, Agricultural and Ecological Aspects (pp. 95399). Intechopen. https://doi.org/10.5772/intechopen.95399
- van der Heijden, M. G. A., Martin, F. M., Selosse, M.-A., & Sanders, I. R. (2014). Mycorrhizal ecology and evolution: The past, the present and the future Tansley review. New Phytologist, 205(4), 1406–1423. https://doi.org/10.1111/nph.13288
- Veronina, E., & Sidorova, I. (2017). Rhizosphere, mycorrhizosphere and hyphosphere as unique niches of soil – Inhabiting bacteria and micromycetes. In H. B. Singh, B. K. Sarma, & C. Keswani (Eds.), Advances in PGPR Research (pp. 165–186). CABI.
- Vidic, I., Berne, S., Drobne, D., Macӗk, P., Frane, Z. R., Turk, T., Strus, J., & Sepcic, K. (2005). Temporal and spatial expression of ostreolysin during development of the oyster mushroom (Pleurotus ostreatus). Mycological Research, 109(3), 377–382. https://doi.org/10.1017/S0953756204002187
- Wallis, I. R., Claridge, A. W., & Trappe, J. M. (2012). Nitrogen content, amino acid composition and digestibility of fungi from a nutritional perspective in animal mycophagy. Fungal Biology, 116(5), 590–602. https://doi.org/10.1016/j.funbio.2012.02.007
- Wang, D., Zhang, J. L., Wang, Y., Zambonelli, A., Hall, I. R., & Xiong, W.-P. (2021). The cultivation of Lactarius with edible mushrooms. Italian Journal of Mycology, 50(2021), 63–77. https://doi.org/10.6092/issn.2531-7342/12908
- Weil, J. D., Cutter, C. N., Beelman, R. B., & LaBorde, L. F. (2013). Inactivation of human pathogens during phase II composting of manure-based mushroom growth substrate. Journal of Food Protection, 76(8), 1393–1400. https://doi.org/10.4315/0362-028X.JFP-12-508
- Wendiro, D., Wacoo, A. P., & Wise, G. (2019). Identifying indigenous practices for cultivation of wild saprophytic mushrooms: Responding to the need for sustainable utilization of natural resources. Journal of Ethnobiology&Ethnomedicine, 15(64), 1–15. https://doi.org/10.1186/s13002-019-0342-z
- Wolfe, B. E., Tulloss, R. E., & Pringle, A. (2012). The irreversible loss of a decomposition pathway marks the single origin of an ectomycorrhizal symbiosis. PLoSONE, 7(7), 1–9. https://doi.org/10.1371/journal.pone.0039597
- Xiang, Q., Adil, B., Chen, Q., Gu, Y., Zeng, X., & Li, X. (2021). Shiitake mushroom (Lentinula edodes (Berk.) Sing. breeding in China. In J. M. Al-Khayri, S. M. Jain, & D. V. Johnson (Eds.), Advances in Plant Breeding Strategies: Vegetable Crops (pp. 443–476). Springer.
- Xie, H., Zhao, J. W., Zhou, H. W., Ren, S. H., & Zhang, R. X. (2020). Secondary utilizations and perspectives of mined underground space. Tunnel and Underground Space Technology, 96(8), 1–14. https://doi.org/10.1016/j.tust.2019.103129
- Yaeger, W., Jawed, M., Tauman, D., Ho, P., Ali, A., Gomanie, N. N., & Mehta, K. (2022). Modular methods for oyster mushroom cultivation in low-resource settings. 2022 IEEE Global Humanitarian Technology Conference (GHTC). Santa Clara, CA, USA: IEEE.
- Yagame, T., & Maekawa, N. (2019). Ectomycorrhiza synthesis and basidiome formation of an orchid symbiont of the genus Thelephora on Quercus serrata. Mycoscience, 60(6), 343–350. https://doi.org/10.1016/j.myc.2019.08.001
- Yamada, A., Ogura, T., & Ohmasa, M. (2001). Cultivation of mushrooms of edible ectomycorrhizal fungi associated with Pinus densiflora by in vitro mycorrhizal synthesis I. Primordium and basidiocarp formation in open-pot culture. Mycorrhiza, 11(2), 59–66. https://doi.org/10.1007/s005720000092
- Yamanaka, T., Yamada, A., & Furukawa, H. (2020). Advances in the cultivation of the highly-prized ectomycorrhizal mushroom Tricholoma matsutake. Mycoscience, access–090821. https://doi.org/10.1016/j.myc.2020.01.001
- Yassine, M. W., Héritier, M. K., & Hippolyte, N. S. W. M. (2022). Spawn production and cultivation of two local edible fungal species in Kisangani (DRC) (Case of Pleurotus tuber-regium and Lentinus squarrosulus). Journal of Microbiology Experimentation, 10(1), 45–48. https://doi.org/10.15406/jmen.2022.10.00351
- Yongabi, K. (2014). Current developments in mushroom biotechnology in Sub-Saharan Africa. WSMBMP Bulletin 11. July 31, 2014. https://www.researchgate.net/publication/296651945
- Yun, W., & Hall, I. R. (2004). Edible ectomycorrhizal mushrooms: Challenges and achievements. Canadian Journal of Botany, 82(8), 1063–1073. https://doi.org/10.1139/b04-051
- Zhang, L., Fan, J., Ding, X., He, X., Zhang, F., & Feng, G. (2014a). Hyphosphere interactions between an arbuscularmycorrhizal fungus and a phosphate solubilizing bacterium promote phytate mineralization in soil. Soil Biology & Biochemistry, 1–7. https://doi.org/10.1016/j.soilbio.2014.03.004
- Zhang, Y., Geng, W., Shen, Y., Wang, Y., & Dai, Y.-C. (2014b). Edible mushroom cultivation for food security and rural development in China: Bio-innovation, technological dissemination and marketing. Sustainability, 6(5), 2961–2973. https://doi.org/10.3390/su6052961
- Zhang, X., Li, L., Kesner, L., Aurélie, C., & Robert, M. (2021). Chemical host-seeking cues of entomopathogenic nematodes. Current Opinion in Insect Science, 44, 72–81. https://doi.org/10.1016/j.cois.2021.03.011
- Zhou, G., & Parawira, W. (2021). The effect of different substrates found in Zimbabwe on the growth and yield of oyster mushroom. Pleurotus ostreatus.SAJEST, 5(2), 73–86. https://doi.org/10.4314/sajest.v5i2.39831
- Zied, D. C., Vieira Junior, W. G., Soares, D. M. M., Stevani, C. V., Dias, E. S., Iossi, M. R., & Pardo-Giménez, A. (2021). Overview of four Agaricussubrufescens strains used in the last 15 years in Brazil and other countries and current potential materials for the future. Mycological Progress, 20(8), 953–966. https://doi.org/10.1007/s11557-021-01711-x