Abstract
Management of disease by using agrochemicals has possessed serious issues and threats to food safety and environment due to excess use. The management of diseases by enhancing resistance or tolerance through plant nutrition contributes to food safety as well as sustainable and integrated disease management. Further, these nutrients optimize yield. Utilization of nutrients in disease management requires the knowledge of their association with plant defence mechanisms. This review describes the association of Zinc (Zn) in developing a plant’s defence mechanism and reducing the severity of diseases. It was found that Zn plays an important role in the functioning of enzymes/proteins like alcohol dehydrogenase, superoxide dismutases, metallothionein and zinc-finger proteins involved in plants’ response to pathogens. Zn supply increases the expression of various antioxidant systems detoxifying the harmful level of free radicals upon infection. Zn enhances the signalling of various defence pathways like salicylic acid-dependent pathways and jasmonic acid/ethylene-dependent pathways as well as improves membrane integrity assisting in defence against pathogens’ attack. A deficiency of Zn makes a plant susceptible to infection due to a deprived condition while an excess level negatively impacts growth and defence due to toxicity. Thus, high and non-toxic levels of Zn supply can be beneficial in enhancing a plant’s defence responses which depend on the nature of the plant and its interaction with pathogens. This study provides a basis for the exploration of the negative relationship between nutrient management and plant diseases.
1. Introduction
Phyto-pathogens are responsible for the occurrence of infectious diseases in a plant (Abdulkhair & Alghuthaymi, Citation2016). These include fungi, bacteria, viruses, nematodes, viroids, mollicutes, protozoa and parasitic higher plants. Among these, the former four pathogens are commonly found to cause the majority of diseases which damage crops and reduce yield. Crop damage by pathogens depends on the severity of the disease. The disease severity is determined by the degree of infection, colonization and damage to host tissues (Gaunt, Citation1995). Disease severity determines the host growth and development on which yield depends. Plant diseases are responsible for yield loss however, loss of yield not only depends on the disease but also on weather parameters, the virulence of a pathogen and the host’s defensive nature. Globally, the potential yield loss by pathogens is estimated to be 16% (Ficke et al., Citation2018). Crop production needs to be increased by 60–110% to meet the demands of food by 2050 (Segal & Wilson, Citation2018). Likewise, a disease reduces the quality and shelf-life of produce. So, management of the disease is necessary to establish a healthy plant population and obtain quality products with improved yield. A conventional management practice includes the use of chemicals like pesticides, herbicides, fungicides and so on (Gupta et al., Citation2017). Excessive use of these chemicals has raised serious concerns about food safety, soil, environmental pollution and induced resistance of pathogens towards these chemicals as a result of prolonged exposure (Dordas, Citation2008). This demands the requirement of eco-friendly, economical and sustainable alternative disease management practices. The concept of sustainable agriculture has evolved and its importance has increased in recent years (Dordas, Citation2008). The global population increase, reduction in agricultural lands and outbreaks of pathogens due to climate change have been challenging factors in agricultural production. This necessitates the requirement of sustainable practices and management of problems before economic damage. In this context, Integrated disease management (IDM) can be a contributing factor to sustainability. One of the important aspects of IDM is an assurance of plant health and immunity in which plant nutrition plays an important role.
Proper nutrition of crops enhances the health and yield of a plant (Gupta et al. Citation2017). Nutrients influence the interaction between plants and pathogens which affects disease development (Gaunt, Citation1995). The nutritional status of a crop determines its susceptibility to pathogens. Enhanced nutrition benefits plants against diseases, however, it may not be the case for all diseases. A particular nutrient may decrease the disease severity of some diseases, while it may increase in others (Huber & Haneklaus, Citation2007). Mineral nutrients include macronutrients like nitrogen (N), phosphorus (P), potassium (K), calcium (Ca), magnesium (Mg) and Sulphur (S), and micronutrients like boron (B), zinc (Zn), copper (Cu), molybdenum (Mo), manganese (Mn), chlorine(Cl) and nickel (Ni) (Gupta et al., ,Citation2017). Macronutrients are required in high amounts while micronutrients are low. Though the requirement for micronutrients is less, it is equally essential for metabolism, growth and development (Bhanukar et al., Citation2018). Apart from various quality and yield parameters of a crop, micronutrients also affect disease development. The incidence or severity of some diseases is found to be influenced by micronutrients (Huber & Haneklaus, Citation2007). Various studies suggest the role of several macronutrients and micronutrients in reducing the severity of plant diseases, however, this review focuses on the role of Zn against plant diseases.
Zinc (Zn), found as a divalent cation (Zn2+) in plants, is an essential micronutrient for proper growth and development (Bouain et al., Citation2017), and the functioning of proteins (Andreini et al., Citation2006). Zn is an imperative element in the triangular association of plant, pathogen and nutrient, and the hypothesis of metal defence propounds that myriad amassing of Zn and other metals serves as a defensive process against pathogens (Helfenstein et al., Citation2015). Zn is an important element in plants’ response to diseases. However, it may vary depending upon how effective will Zn-related responses be able to limit the pathogens’ attack and the pathogenicity of invaders by averting plant defences with a consideration of environmental conditions too. Zn proteins either aid plants or avoid invaders to defend against the pathogen attack, but the certainty of its beneficial effect doesn’t hold for all plants since it may create susceptibility in some plants (Cabot et al., Citation2019). Various studies suggest the role of Zn nutrition in enhancing plant response against diseases. Several Zn-based compounds and Nano-particles (NPs) are found to be used in the controlling of diseases or pathogen growth. NPs are eco-friendly alternatives to synthetic chemical fungicides because of their high effectiveness at low doses (Malandrakis et al., Citation2019). Several studies suggest the role of Zn against plant pathogens which are discussed below in the study.
Enhancing resistance or tolerance to diseases by plant nutrition is one of the emerging disease management concepts in recent agriculture as a part of IDM which contributes to sustainability. But, the utilization of nutrients in disease management requires knowledge of their association with the development of mechanisms against infection/diseases. So, this review was done with the purpose to understand the involvement of Zn in the mechanisms of plant defence. In this review, the association of Zn with different enzymes and proteins involved in mechanisms against plant pathogens has been discussed. Several studies were reviewed that suggest the role of Zn against pathogens focusing especially on fungus, bacteria, viruses and nematodes. This review summarizes the findings of these studies and helps readers to see the possibilities for future research. The severity of plant diseases and the defensive mechanisms in response to Zn supply are also discussed.
2. Functions of Zn in plants concerning plant defence response
Zn is involved in the activation of the various enzyme system that regulates different physiological processes along with defence mechanism in plant-pathogen interaction. Most of the enzymes that need Zn for activation and those containing Zn bind Zn through imidazole and cysteine (Brown et al., Citation1993). Removal of the bound Zn can cut off the enzymatic activity (Mccall et al., Citation2000). Zn serves as a cofactor of catalytic and structural proteins and has key functions in protein domains that interact with other molecules (Cabot et al., Citation2019). It is pivotal for the constitution of metalloenzymes which participates in different processes, such as metabolism, nucleic acid and protein synthesis, and breakdown of protein (Bouain et al., Citation2017). The chief role of Zn ions in Zn-proteins/enzymes can be catalytic, co-catalytic or structural. In a catalytic role, the Zn ions explicitly show presence in making or breaking the bond, whereas in co-catalytic, various metal ions are attached near to one another, where one shows a catalytic role and the other metal ions enhance the catalytic activity of the site. But, in a structural role, the Zn ion participates to stabilize the tertiary structure of enzymes in a way analogous to disulfide bonds. The most commonly assigned functions of Zn-enzyme/proteins (Cabot et al., Citation2019) are discussed below:
2.1. Alcohol dehydrogenase (AD)
Alcohol dehydrogenase (AD) of medium-chain dehydrogenase/reductase (MDR) is Zn-metalloenzymes with frequently two tetrahedral coordination of Zn2+ per subunit: one as catalytic at the active site and the next as structural at a site thought to be affecting subunit interplay (Auld & Bergman, Citation2008). These are NADP-dependent oxidoreductases that participate in the transfer of hydride ions from alcohols to NAD+, thus catalyzing reversible oxidation of alcohols to aldehydes or ketones (Kumar et al., Citation2016). Anaerobic condition alters chemical reaction due to lack of oxygen and leads to the production of toxic substances as a result of microbial activity. AD metabolizes alcohol produced in such conditions (Castillo-Gonzalez et al., Citation2018). Zn binding AD (AdZADH2) was upregulated in Arachis diogoi (wild peanut) against inoculated leaf spot pathogen (Phaeoisariopsis personata). AdZADH2 from Peanut resulted in hypersensitive response (HR) like cell death and enhanced expression of defence-related genes, marker genes and antioxidant enzymes in Tobacco leaf under estradiol-inducible promoter (Kumar et al., Citation2016). Likewise, Zn binding Cinnamyl alcohol dehydrogenase (CAD) plays a role in lignin biosynthesis presenting defensive activity by strengthening the cell wall (Kumar et al., Citation2016). Wheat CAD (TaCAD12) showed an important role in the resistance against sharp eyespot, caused by Rhizoctonia cerealis, through positive regulation of expression of certain defence genes and Monolignol-biosynthesis-related (MBR) genes in Wheat. MBR genes (CAD, CCR and COMT) may also have a role in resistance since they help in the formation of lignin through monolignols and the deposition of lignin is thought to strengthen cell wall due to its extreme resistance to microbial degradation (Tronchet et al., Citation2010). This serves as a medium of defence against infection.
2.2. Metallothionein (MT)
MTs are cysteine-rich proteins involved in metal tolerance or homeostasis, as they attach to metal ions via thiol groups of their cysteine residues (Hassinen et al., Citation2011). MT has a great role against the toxicity of heavy metals and osmotic stresses (Yang et al., Citation2015). MT acts as an antioxidant against harmful reactive oxygen and nitrogen species produced due to pathogens’ infection. MT can scavenge free radicals. Zn as well as Cd and Cu bind with MTs (Hassinen et al., Citation2011). Zn has an important role in the function of MT due to its close interaction. Zn induces Zn-binding MT increment and its expression through metal regulatory transcription factor 1 (MTF-1) which explicitly respond with an increment of free Zn (Dumanović et al., Citation2021; Ruttkay-Nedecky et al., Citation2013)). MT-like proteins are also bounded with Zn (Rema & Philip, Citation1996). During Magnoporthe grisea infection, four pathogenesis-related genes and MTs-1 were highly expressed in Rice to defend the pathogen. Though the expression of MTs-1 was seen in healthy plants also, the expression was high in case of infection to show defensive action (Kim et al., Citation2001). Mt gene in Beech tree (Fagus grandifolia) codes mRNA for MT-like proteins whose high expression is associated with resistance to beech bark disease (a scale-fungal complex) and MT proteins may have an important role against beech-scale insect (vector of the disease) (Chen et al., Citation2021).
2.3. Super-oxide dismutase (SOD)
SOD is an enzyme found in all aerobic cells and subcellular compartments sensitive to oxidative stress and takes part in the dismutation of superoxide anion to hydrogen peroxide which is detoxified by Ascorbate peroxidase (APX), Glutathione peroxidase (GPX) and Catalase (CAT) (Dumanović et al., Citation2021). SOD is an integral anti-oxidant defensive enzyme as it works against superoxide anion, also known as reactive oxygen species (ROS), in oxygen-exposed plant cells. There are several other enzymatic antioxidants like CAT, GPX, APX, Monodehydroascorbate reductase (MDHAR), Dehydroascorbate reductase (DHAR) and Glutathione reductase (GR) as well as non-enzymatic antioxidants like Ascorbate (AsA), Glutathione (GSH), carotenoids, tocopherols, proline and phenolics that also play role in scavenging or detoxification of ROS (Sharma et al., Citation2012). There is an indirect requirement of Zn for the activity of CAT, APX and GPX (Cakmak, Citation2000). Sharma et al. (Citation2012) mentioned that ROS acts as both signalling () and damaging () molecule that depends upon the balance between the production and scavenging of ROS. So, a cell needs to control the levels of ROS. The levels of ROS increase after a pathogen’s attack (Wongpia & Lomthaisong, Citation2010) and its amassing is harmful to cells as it induces oxidative stress (Subramanian et al., Citation2005).
Figure 1. Role of low concentration ROS in signalling different plant responses (Sharma et al., Citation2012).
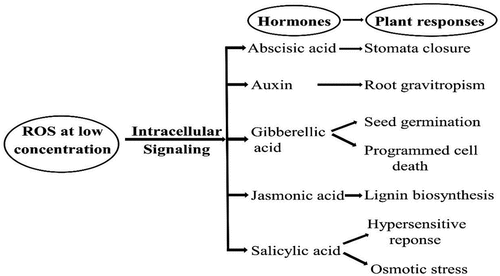
Figure 2. Harmful effects of high concentration ROS within a plant (Sharma et al., Citation2012).
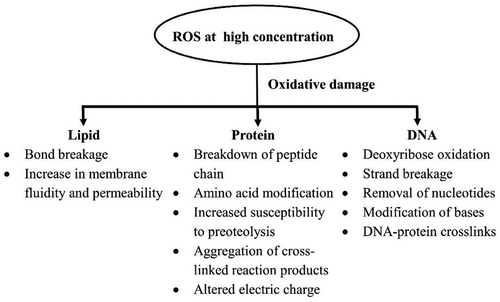
In enzyme-mediated reactions, the removal of ROS produced during infection is highly efficient when catalyzed by SOD (Dumanović et al., Citation2021). The proteome analysis of crops from Brassicaceae suggested the detoxification of superoxide radicals produced during fungal infection may be due to the involvement of SOD (Wongpia & Lomthaisong, Citation2010). Three types of SOD are classified according to their sensitivity to cyanide and H2O2. Activity of CuZn-SOD is sensitive to both H2O2 and cyanide, Fe-SOD is sensitive to H2O2 only and Mn-SOD is not affected by both cyanide and H2O2 (Cakmak, Citation2000). Among these, CuZn-SOD is the most abundant in higher plants while Mn-SOD and Fe-SOD form a smaller proportion of total SOD activity (Jackson et al., Citation1978). Zn is associated with copper at the active site of CuZn-SOD in a plant. Zn is associated with the expression of SOD activity, mainly CuZn-SOD which contributes to a greater extent of SOD activity in plants. The activity of SOD is reduced under Zn deficiency (Castillo-Gonzalez et al., Citation2018). Zn deficiency reduced SOD activity along with the parallel increment of superoxide anion radicals in the roots of cotton plants (Cakmak & Marschner, Citation1988). Bharti et al. (Citation2014) reported increased SOD activity with increased Zn level and a drop in activity was seen under deficit conditions. Apart from Zn nutritional status, the activity of CuZn-SOD is reduced under the exposure of plants to photo-oxidative conditions resulting in the production of a high amount of H2O2 (Cakmak, Citation2000). Cakmak (Citation2000) also mentioned that H2O2 is found to inhibit the activity of CuZn-SOD since Cu2+ is reduced to Cu+ by H2O2 resulting in the production of OH−. Bharti et al. (Citation2014) also reported a drop in SOD activity under deficit conditions while the activity was increased with increased Zn level. The increment in SOD expression might help a plant during defence and resistance mechanisms. The activity of SOD was overexpressed in transgenic Potato with conferred Cu/Zn-SOD gene that enhanced resistance against Erwinia carotovora (Wongpia & Lomthaisong, Citation2010). Zn plays a crucial role in controlling the production of toxic oxygen through interference in NADPH oxidation as well as the removal of O2 radicals. In the case of Zn deficiency, there exists an increment in O2 radicals and the mounting of toxic O2 free radicals breaks bonds present in poly-unsaturated fatty acids and phospholipids of membranes, ultimately leading to increased permeability of plasma membrane. The increased permeability will ascend the leakage of carbohydrates and amino acids from the root as well as aerial parts predisposing the plant towards pathogens’ attack (Castillo-Gonzalez et al., Citation2018). Secretion of root exudates was more under Zn deficiency while lowered secretion was seen in the case of zinc sufficiency (Bharti et al., Citation2014). ROS production is also raised by increased concentration of iron (Fe) due to Zn deficiency (Cakmak, Citation2000). In tobacco leaf, expression of SOD enzyme was also seen during HR-like cell death to show resistance towards leaf spot pathogen (Kumar et al., Citation2016). High SOD activity was seen in cultivars resistant to stem canker or black leg caused by Leptospaeria maculans. This implies that SOD is associated with plant defence mechanism and Zn play important role in its expression.
2.4. Zinc finger (Znf)
Zinc finger proteins, the superfamily of proteins, is any functional freely folded domain that uses finger-like structures containing one or more Zn ions to stabilize its structure (Chen et al., Citation2021; Gupta et al., Citation2012) and are active in regulating various metabolic processes as well as stress conditions in a plant (Gupta et al., Citation2012). Zn2+ present in the network binds with nucleotides and recognizes DNA-RNA complexes or other proteins (Chen et al., Citation2021). This helps in the proper functioning of important processes of plants like growth, apoptosis, cell proliferation, and differentiation along with plant response to biotic stress through transcription and translation of genes (Cabot et al., Citation2019; Chen et al., Citation2021). Cabot et al. (Citation2019) studied 70 plant proteins resistant to disease from various crops and among the proteins, 37% contain Znf domains suggesting the pivotal role of these proteins against pathogens. Znf proteins are categorized into various types like C2H2, C2C2, C2HC and C2HCC2C2 based on cysteine and histidine residues bound to Zn ion in secondary structure. Among them, C2H2 is most abundant in eukaryotes (Noman et al., Citation2019). A genomic study of C2H2 type Znf transcription factors in Tomato reported the expression of 32 C2H2 genes in response to multiple stresses and among these, 8 genes were specially expressed under pathogenic stress (Zhao et al., Citation2020). Meta-analysis was done to analyze the protein sequences of 70 resistance (R) genes from different crops in which 34 Znf domains were found in 26 R-proteins from 9 crops (Gupta et al., Citation2012). Pi54 gene involved in enduring resistance against blast in Rice has NFX type Znf domain. Multiple Znf domains are present in many disease resistance genes like pI8 (Sunflower), Pib (Rice), Lr10 (Wheat), Gro1–4 (Potato), RCY1 (Arabidopsis) and Rpg1 (Barley) (Gupta et al., Citation2012). According to Oh et al. (Citation2005), EPF-type Znf protein (CaPIF1) of hot pepper (Capsicum annuum) has a role in disease resistance since CaPIF1 gene was expressed rapidly and specifically under bacterial infection in resistant variety while expression was weak in case of susceptible one. In addition to this, over-expression of CaPIF1 in transgenic tobacco increased resistance against bacterial pathogens (Oh et al., Citation2005).
The expression of all the above enzymes/proteins is regulated by sufficient availability of Zn. The deficiency of Zn results in different physiological irregularities as well as makes the plant susceptible to infection. For most of the crops, critical deficiency concentrations of Zn are below 15–20 mg kg−1 dry weight (Marschner, Citation2012). However, sensitivity to deficiency of Zn varies with crops, like Rice, Maize and Apple are more sensitive than Rye, Oats or Pea. Zn deficiency is prevalent in a widespread cropping system. Graham (Citation2008) mentioned that 49% of global agricultural soils are deficient in Zn. Zn deficiency has been reported in a wide range of cropping systems. Zn deficiency is also widespread in flooded rice with high pH and organic matter content (Marschner, Citation2012). The most common deficiency in Citrus is also Zn deficiency (Boaretto et al., Citation2001). The supply and availability of Zn are beneficial, however, an excess of Zn may create toxicity unless the plant is hyperaccumulating species. Hyperaccumulators are those plants that can accumulate abnormally high concentrations of heavy metals like Zn and continue their growth, where sensitive crops cannot grow (Balafrej et al., Citation2020). Such species tend to amass Zn at high concentrations within a plant which doesn’t harm the plant but rather can cause toxicity to pathogens in case of infection and serves as a defence factor (Helfenstein et al., Citation2015). This may not be the situation in all plants since Zn hyper level may be toxic to certain plants. The toxicity of Zn occurs when soil is contaminated by mining, treated with sewage sludge or in case of excessive supply. Critical toxicity concentrations of Zn range from 100 to more than 300 mg kg−1 dry weight (later being more typical) (Marschner, Citation2012). So, based on the plant’s nature and soil condition, Zn can be supplied to increase the production of defensive factors.
3. Role of Zn supply against plant pathogens/diseases
Phyto-pathogens include fungi, bacteria, viruses, nematodes, viroids, mollicutes, protozoa and parasitic higher plants (Abdulkhair & Alghuthaymi, Citation2016). Three responses are found to be involved in plant defence against pathogens named as gene-for-gene resistance responses, salicylic acid (SA)-dependent responses and jasmonic acid (JA)/ethylene (ET) dependent responses. The first two are thought to be involved in defence against pathogens with a biotrophic lifestyle while JA/ET mediated responses are responsible for defence against the necrotrophic lifestyle. Biotrophs are those pathogens that obtain nutrients from the living tissues of a host, while Necrotrophs derive nutrients from dead and dying cells. Hemi-biotrophs show both biotrophic and necrotrophic behaviour depending upon life stages. Hemi-biotrophs first grow in living host tissues and later kills the host (Segal & Wilson, Citation2018). Zn is associated with these defence signalling as shown in Figure (above) which is discussed below wherever relevant.
The roles of Zn supply against major plant pathogens/diseases are discussed below. Though the roles of Zn in the pathogenesis of pathogens are also found, it is not discussed here.
3.1. Zinc against viral diseases
Plant nutrition helps to defend against viral damage as nutrition helps to improve plant growth and development, vigour and robustness. Islam et al. (Citation2002) reported a significant reduction in the incidence and severity of yellow mosaic disease of Mungbean under soil application of ZnSO4 in combination with or without weeding, however, best results were seen with Zn+B (Boron)+S (Sulphur) when weeding was done. According to Kalsoom et al. (Citation2019), ZnSO4 significantly reduced the severity of the Cotton leaf curl virus (CLCuV) and also created a harsh condition for whitefly (vector of CLCuV). Foliar application of ZnSO4 was able to abate the symptoms and concentration of potato viruses (PVY and PLRV) by increasing resistance to viral diseases as a result of enhanced level of phenolic compounds which plays a key role in the development of defence mechanism against viruses (Ibrahim et al., Citation2016). However, the findings of Deepika et al. (Citation2021) revealed no significant effect of ZnSO4 (0.5%) alone on reducing the severity of papaya ringspot disease while spraying of zinc sulphate (0.5%) + boric acid (0.1%) + urea (1%) + soil drenching of humic acid (0.2%) showed significant reduction of the disease severity. Zn is involved in protein and starch synthesis. Plants low in Zn tend to accumulate amino acids and reducing sugars in their tissues (Dutta et al., Citation2017). This amassing of amino acids in a plant makes it favourable for feeding and reproduction by sucking insects. Dutta et al. (Citation2017) also mentioned that, from a vector point of view, increased feeding intensity and reproduction may increase viral transmission and disease development.
In recent advancements, several zinc-based nanoparticles (NPs) are used for nutrition and also have low ecological toxicity. Sofy et al. (Citation2021) found ZnO-NPs as an eco-friendly and economic antiviral agent against TMV that reduces oxidative damage of the virus by lowering the levels of MDA (Malondialdehydye; an indicator of oxidative stress), O2 and H2O2. The antiviral properties of ZnO-NPs were reported by Cai et al. (Citation2019) where the foliar spray of ZnO-NPs directly affected the TMV as well as enhanced the plant’s defensive system. In the study, they found that the application of ZnO-NPs led to the production of ROS which further resulted in the activation of the antioxidant system and upregulation of resistance-related genes PR1 and PR2 mediated by SA signalling. In addition to this, the abscisic acid level was also increased which might have induced stomata closure and prevented the entry of the pathogen. This reduces the infection of viruses.
3.2. Zn against bacteria
Some studies suggest the role of Zn nutrition against bacterial pathogens. Zn nutrition is useful in controlling bacterial diseases like Ginseng bacterial leaf spot caused by Pseudomonas cichorii (Dutta et al., Citation2017). There are also reports of inhibition of growth and infectivity of Xanthomonas campestris pv. vesicatoria on Pepper (Duffy, Citation2007). Similarly, Quaglia et al. (Citation2021) found the protective effect of zinc phosphate against Pseudomonas syringae pv. tomato that causes bacterial speck disease in Tomato. In the experiment by Quaglia et al., in vitro assays confirmed the direct antimicrobial toxicity of higher Zn levels. They also reported the induction of defence responses like callose deposition and expression of the SA-dependent pathogenesis-related protein PR1b1 gene by the zinc phosphate. Callose is a β-(1,3)-D-glucan polysaccharide deposited between the plasma membrane and the cell wall at the site of infection that slows down the pathogen invasion and spread (Wang et al., Citation2021). The optimal Zn level increased the resistance of Soybean to bacterial pustule (Xanthomonas axonopodis pv. glycines), however, a very high Zn level didn’t increase resistance relative to the optimal level. The high concentration resulted in a very high level of Zn in the leaf (1063 mg kg−1) which is above the accepted toxic level (Marschner, Citation2012). This causes toxicity. Zn deficiency increased the severity of pathogen in Soybean (Helfenstein et al., Citation2015).
The antibacterial property of ZnO NPs was studied by Siddiquiet al. (Citation2018) in lentils inoculated with P. syringae pv. syringae and X. axonopodis pv. phaseoli that cause bacterial blight and bacterial leaf spots and found ZnO-Nps are beneficial in reducing the severity of bacterial diseases. In a study by Siddiqui et al. (Citation2018), increased ZnO-NPs concentration showed greater inhibitory action against X. campestris pv. beticola, P. syringae pv. aptata and Pectobacterium betavasculorum in nutrient agar medium. They also reported that ZnO-NPs in inoculated plants enhanced plant growth and pigment synthesis along with the increased activity of enzymatic antioxidants (SOD, CAT and APX) and non-enzymatic antioxidants (PAL, GSH and proline). MDA was increased in the plants without treatment due to the pathogen but reduced in the case of treatment indicating the role of ZnO-NPs in reducing lipid peroxidation (Siddiquiet al., Citation2018). This contributes to membrane integrity.
3.3. Zn against fungus
Zn has an important role in reducing the severity of plant pathogenic fungal infection. A sufficient supply of Zn may enhance the cellular integrity and improve the permeability of cell membrane which acts as a defence factor against fungal infection (Machado et al., Citation2018). Simoglou & Dordas, (Citation2006)) reported that foliar application of Zn was able to significantly reduce the severity of tan spot disease of Winter durum wheat caused by Pyrenophora tritici-repentis. Similarly, Khoshgoftarmanesh et al. (Citation2010) found the beneficial role of Zn nutrition in reducing the severity of Fusarium root-rot infection in Wheat. According to them, Zn nutrition enhanced the resistance of a plant to disease by stabilizing root cell membranes as root membrane permeability was reduced under Zn treatment and also through increased plant vigour and root growth. In the previous study as cited in Khoshgoftarmanesh et al. (Citation2010), the severity of crown rot disease in wheat caused by Fusarium graminearum was enhanced due to Zn deficiency, whereas infection was suppressed in the case of Zn sufficiency. Zn sufficient plants are more tolerant to the fungal infection (Rhizoctonia solani) in Medicago truncatula (Streeter et al., Citation2001). Soil application of Zn in interaction with NPK managed early blight disease in Tomato, caused by Alternaria solani, in a pot experiment (Awan et al., Citation2019). The management of early blight is attributed to the increased activity of antioxidant enzymes like SOD and defence-related proteins. Application of Zn-compounds was able to reduce the mycelial growth and severity of Helminthosporium leaf spot of Sesame in both in-vitro and field conditions (El-Fawy & El-Said, Citation2018). Savi et al. (Citation2013) studied antifungal and antimycotoxin properties of Zn compounds against virulent strains of Fusarium graminearum, Penicillium citrinum and Aspergillus flavus, and found that ZnSO4 and Zn(ClO4)2 completely inhibited the fungal growth and ability to produce mycotoxins. The Zn compounds challenged fungi cellular metabolism which interfered with conidia production and altered hyphae morphology. A study on the effect of Zn on Arabidopsis thaliana against a fungal pathogen (Alternaria brassicicola) revealed that high but non-phytotoxic concentration of Zn improved plants defence response against the pathogen through enhanced JA/ET dependent defence signalling pathway (Martos et al., Citation2016). Though the Zn concentrations couldn’t substitute the role of Camalexin, a phytoalexin involved in defence against Alternaria, it enhanced the expression of PAD3 which catalyzes the final step in camalexin synthesis. Soil application of Zn reduced the incidence and severity of potato early blight disease (Machado et al., Citation2018). Zinc phosphite (10 ppm) showed complete inhibition of mycelial growth of Phytophthora nicotianae in-vitro (Lucero et al., Citation2014). According to Shoaib et al. (Citation2021), in in-vitro, ZnSO4 reduced the growth of Alternaria alternata in a dose-dependent manner. Also, in-vivo foliar spray of ZnSO4 reduced Alternaria leaf spot disease in Chili pepper plant through induction of resistance by improving the activities of antioxidants (CAT and POX) and defence compounds (PPO and PAL). Soil application of Zn was effective in enhancing the resistance of Clusterbean seedlings in pot culture against Rhizoctonia species after carbendazim (Wadhwa et al., Citation2014). The activities of enzymes Peroxidase (POX), Polyphenol oxidase (PPO), Phenylalanine ammonia lyase (PAL) and Tyrosine ammonia lyase (TAL) increased due to infection as a part of defence, which was further enhanced by Zn nutrition (Wadhwa et al., Citation2014). They also stated that POX forms a barrier at the site of pathogen infection through lignification while PAL and TAL play a pivotal role in phenylpropanoid metabolism as well as perform defensive functions. The severity of coffee rust caused by Hemileia vastatrix was reduced accordingly with an increase in Zn concentration from 0.25 to 2 mg L−1 while the reduction was low at concentrations out of this range. Li et al. (Citation2016) reported the beneficial effect of ZnSO4 in Peach shoots that reduced the severity of gummosis caused by Lasiodiplodia theobromae as well as decreased the expression of genes (endo-polygalacturonase and pectin methylesterase) which produces cell wall degrading enzymes in Peach shoots during infection. Li et al. (Citation2016) also reported that in-vitro mycelial growth of the fungus L. theobromae was also inhibited thereby showing the dual function of the compound as direct inhibition to the fungus and reduction in susceptibility of Peach. Quaglia et al. (Citation2022) reported increased susceptibility of Arabidopsis thaliana Col-0 (wild type) to Botrytis cinerea (necrotrophic pathogen) at null and excess Zn concentrations, and this could be associated with the lack of induction of PDF 1.2 (gene related to the pathogen resistance) transcripts. Also, susceptibility to Golovinomyces cichoracearum, a biotrophic fungus, increased with the increased dose of Zn because of the inability to reduce the development of the pathogen as well as the lack of oxidative burst due to activation of antioxidant systems at that Zn level (higher than physiological condition) that led to ROS scavenging. Oxidative burst, defined as the rapid amassing of ROS around the site of infection, is a primary disease resistance response by plant (Zhang et al., Citation2020). R gene-mediated resistance goes along with an oxidative burst and ROS production is also required for HR cell death (Glazebrook, Citation2005). R gene-mediated resistance and HR response are thought to be involved in resistance against biotrophs which deprives these pathogens from food source. But, in case of necrotrophs, programmed cell death favours pathogen. According to Zhang et al. (Citation2020), the burst inhibits the growth and development of biotrophic fungus while the host cell death due to ROS accumulation benefits necrotrophic pathogens like B. cinerea and Leptosphaeria maculans since they survive in dead cells. Quaglia et al. (Citation2022) mentioned that the lower susceptibility to G. cichoracearum under Zn deficit can be ascribed to higher oxidative stress that makes cellular environment unfavourable for biotrophic fungus. Qualiga et al., also mentioned that in Zn deficient plants, oxidative stress could explain lower susceptibility to biotrophs and higher susceptibility to necrotrophs. This is because oxidative stress results in cellular death which favours necrotrophic fungus. Jain et al. (Citation2010) reported increased oxidative stress at toxic Zn concentrations as well as the activity of antioxidant enzymes also increased in response to oxidative stress with an increase in Zn level.
Some Zn-based nanoparticles are found to be used as antifungal agents. Siddiqui et al. (Citation2018) studied the effect of spraying zinc oxide nanoparticles (ZnO-NPs) against blight and wilt of lentil caused by Alternaria alternata and Fusarium oxysporum f. sp. lentis and found that the disease severity was reduced by the treatment in inoculated lentil. In-vitro assay performed by Pan et al. (Citation2022) revealed the antifungal activity of ZnO-NPs against Fusarium proliferatum. They also found a reduced abundance of Neocosmospora, Gibberella and Fusarium after an application of the ZnO-NPs in soil, and the treatment formed a new microbial community in the soil to overcome Apple replant disease (a complex disease caused by various biotic and abiotic factors whose main factor is soil-borne pathogens). Malandrakis et al. (Citation2019) also reported an antifungal activity of ZnO-NPs than ZnSO4 against B. cinerea, A. alternata, Monilia fructicola, Verticillium dahliae, Colletotrichum gloeosporioides, F. oxysporum f. sp. radicis lycopersici and Fusarium solani.
3.4. Zn against nematode
Management of nematode under Zn nutrition might be associated with enhancement of cellular integrity since Zn nutrition enhances the activity of SOD to detoxify the effect of high levels of ROS which otherwise would have stimulated lipid peroxidation and reduced membrane integrity and thereby have increased the permeability of roots making prone to nematode infection (Cakmak & Marschner, Citation1988; Couto et al., Citation2016). There may be a high number of free amino acids due to inhibition of protein synthesis under Zn deficit condition and these amino acids are leaked in root exudates towards which nematodes are attracted and thus nematode attack is ascended (Couto et al., Citation2016; Streeter et al., Citation2001). Shaukat and Siddiqui (Citation2003) studied the effect of Zn in association with different strains of Rhizobium (produces potential nematicidal compound) culture against root-knot nematode (Meloidogyne icognita) of Tomato and found that mortality of nematode in-vitro increased with increase in Zn concentration. The mortality further increased in combination with Rhizobial strains as Zn enhanced the nematicidal activity of various strains. Similarly, in a greenhouse culture of Tomato, soil application of Zn alone was also able to reduce root-knot development suggesting the role of Zn nutrition in the management of nematode damage in Tomato, however, the knot development was further lowered to a minimum when combined with selected Rhizobial strains (PSG1 and MIG1). This suggests that Zn nutrition improves the efficacy of biocontrol agents like Rhizobia to manage root-knot nematode. PSG1 and MIG1 are the strains of Rhizobium leguminosarum and Sinorhizobium meliloti (Shaukat & Siddiqui, Citation2003). But the findings of Couto et al. (Citation2016) revealed that there is no direct effect of Zn on embryonic development or juvenile hatching of M. icognita, however, the number of galls and number of eggs was reduced. Zn significantly reduced the number of nematodes per gram and somehow the total nematode population per root system, however, the reduction was significantly better in case of Zn+MOS (Mannan-oligosaccharide derived from the cell walls of Saccharomyces cerevisiae). Soil application of Zn alone or in combination with Pseudomonas aeruginosa IE-65+ reduced the penetration of nematode Meloidogyne javanica significantly. Spraying of ZnO NPs reduced the galling and nematode population of M. icognita in inoculated lentil plants (Siddiqui et al., Citation2018).
4. Conclusion
Zinc is an important nutrient element to plants as it regulates various physiological processes resulting in the development of responses against diseases. Zn is responsible for the activation of various defence related enzymes like alcohol dehydrogenase and superoxide dismutase as well as helps in the synthesis of defence-related proteins like metallothionein and zinc-finger proteins. Upon infection, there is rapid production of reactive oxygen species (ROS) which accumulates at the site of infection. This initiates the defence response in the plant. However, levels of ROS need to be controlled by anti-oxidant systems. In Zn deficit plants, lack of activation of various anti-oxidant enzymes results in hyperaccumulation of ROS which is toxic to cells. The harmful level of ROS oxidizes cellular components thereby leading to bond breakage and reduction in membrane integrity. This results in enhanced permeability and leakiness of roots and shoots favouring disease development. Zn deficiency increases the amount of amino acids in root and shoot exudates which further potentiates the pathogen infection. Enhanced Zn nutrition activates anti-oxidant systems detoxifying or scavenging excess ROS. Further, it plays an important role in the expression of R-gene mediated response, salicylic acid (SA) dependent pathways and jasmonic acid (JA)/ethylene (ET) dependent pathways. The first two are found to be involved in defence response against biotrophic pathogens while JA/ET plays a role against a necrotrophic pathogen. R gene-mediate response, SA signalling and anti-oxidant enzymes are found to be responsible for defence against viruses. Zn also enhances various defence-related mechanisms in a plant like enhancing membrane integrity and callose deposition that directly or indirectly create a barrier to infection. Enhanced membrane integrity and mortality by the use of Zn-compounds are responsible for defence against nematodes. A very high concentration of Zn results in toxicity and susceptibility due to hyper-production of ROS. The toxicity depends on the nature of plants and their tolerance to Zn. Hyperaccumulating species tend to accumulate a hyper level of Zn without showing detrimental effects, which becomes toxic to pathogens. So, maintaining the level of Zn is necessary ,depending on plants and their sensitivity, to enhance defence mechanisms. This study helps in utilizing the nutrients in the management of diseases by enhancing defence responses and further opens area for more anti-pathogenic studies.
Abbreviations
AD | = | Alcohol dehydrogenase |
APX | = | Ascorbate peroxidase |
CAD | = | Cinnamyl alcohol dehydrogenase |
CAT | = | Catalase |
CCR | = | Cinnamoyl-CoA reductase |
CLCuV | = | Cotton leaf curl virus |
COMT | = | Caffeic acid O-methyltransferase |
DNA | = | Deoxyribonucleic acid |
GPx | = | Glutathione peroxidase |
GSH | = | Glutathione |
JA/ET | = | Jasmonate/Ethylene |
MBR | = | Monolignol-biosynthesis-related |
MDA | = | Malondialdehyde |
MT | = | Metallothionein |
NAD+ | = | Nicotinamide adenine dinucleotide |
NADP | = | Nicotinamide adenine dinucleotide phosphate |
NADPH | = | Nicotinamide adenine dinucleotide phosphate hydrogen |
NPs | = | Nanoparticles |
PAL | = | Phenylalanine ammonia-lyase |
PLRV | = | Potato leaf roll virus |
POX | = | Peroxidase |
PPO | = | Polyphenol oxidase |
PVY | = | Potato Virus-Y |
RNA | = | Ribonucleic acid |
ROS | = | Reactive oxygen species |
R-protein | = | Resistance protein |
SOD | = | Superoxide dismutase |
TAL | = | Tyrosine ammonia lyase |
TMV | = | Tobacco mosaic virus |
Znf | = | Zinc finger |
Disclosure statement
The author declares no conflict of interest.
Additional information
Funding
References
- Abdulkhair, W. M., & Alghuthaymi, M. A. (2016). Plant pathogens. Plant Growth, 49. https://doi.org/10.5772/65325
- Andreini, C., Banci, L., Bertini, I., & Rosato, A. (2006). Zinc through the three domains of life. Journal of Proteome Research, 5(11), 3173–13. https://doi.org/10.1021/pr0603699
- Auld, D. S., & Bergman, T. (2008). Medium- and short-chain dehydrogenase/reductase gene and protein families. Cellular and Molecular Life Sciences, 65(24), 3961. https://doi.org/10.1007/s00018-008-8593-1
- Awan, Z. A., Shoaib, A., & Khan, K. A. (2019). Crosstalk of Zn in combination with other fertilizers underpins interactive effects and induces resistance in tomato plant against early blight disease. Plant Pathology Journal, 35(4), 330–340. https://doi.org/10.5423/PPJ.OA.01.2019.0002
- Balafrej, H., Bogusz, D., Triqui, Z. E. A., Guedira, A., Bendaou, N., Smouni, A., & Fahr, M. (2020). Zinc hyperaccumulation in plants: A review. Plants, 9(5), 562. https://doi.org/10.3390/plants9050562
- Bhanukar, M., Rana, G. S., Sehrawat, S. K., & Preeti. (2018). Effect of exogenous application of micronutrients on growth and yield of sweet orange cv. Blood Red. Journal of Pharmacognosy and Phytochemistry, 7(2), 610–612.
- Bharti, K., Pandey, N., Shankhdhar, D., Srivastava, P. C., & Shankhdhar, S. C. (2014). Effect of different zinc levels on activity of superoxide dismutases & acid phosphatases and organic acid exudation on wheat genotypes. Physiology and Molecular Biology of Plants, 20(1), 41–48. https://doi.org/10.1007/s12298-013-0201-7
- Boaretto, A. E., Boaretto, R. M., Muraoka, T., NascimentoFilho, V. F., Tiritan, C. S., & Mourãofilho, F. A. A. (2001). Foliar micronutrient application effects on citrus fruit yield, soil and leaf Zn concentrations and 65zn mobilization within the plant. Acta Horticulturae, (594), 203–209. https://doi.org/10.17660/ActaHortic.2002.594.22
- Bouain, N., Satbhai, S., Saenchai, C., Desbrosses, G., Berthomieu, P., Busch, W., & Rouached, H. (2017). Zinc availability modulates plant growth and immune responses via AZI1. BioRxiv, 1(858), 166645.
- Brown, P.H., Cakmak, I., Zhang, Q. (1993). Form and Function of Zinc Plants. In: Robson, A.D. (eds) Zinc in Soils and Plants. Developments in Plant and Soil Sciences, vol 55. Springer, Dordrecht. https://doi.org/10.1007/978-94-011-0878-2_7
- Cabot, C., Martos, S., Llugany, M., Gallego, B., Tolrà, R., & Poschenrieder, C. (2019). A Role of zinc in plant defence against pathogens and herbivores. Frontiers in Plant Science, 10, 1–15. https://doi.org/10.3389/fpls.2019.01171
- Cai, L., Liu, C., Fan, G., Liu, C., & Sun, X. (2019). Preventing viral disease by ZnONPs through directly deactivating TMV and activating plant immunity in Nicotiana benthamiana. Environmental Science: Nano, 6(12), 3653–3669. https://doi.org/10.1039/C9EN00850K
- Cakmak, I. (2000). Tansley review No. 111 possible roles of zinc in protecting plant cells from damage by reactive oxygen species. The New Phytologist, 146(2), 185–205. https://doi.org/10.1046/j.1469-8137.2000.00630.x
- Cakmak, I., & Marschner, H. (1988). Enhanced superoxide radical production in roots of zinc-deficient plants. Journal of Experimental Botany, 39(10), 1449–1460. https://doi.org/10.1093/jxb/39.10.1449
- Castillo-Gonzalez, J., Ojeda-Barrios, D., Hernandez-Rodriguez, A., González-Franco, A. C., Robles-Herández, L., & López-Ochoa, G. R. (2018). Zinc Metalloenzymes in plants. Interciencia, 43(4), 242–248.
- Chen, X., Pei, Z., Peng, L., Qin, Q., Duan, Y., Liu, H., Chen, X., Zheng, L., Luo, C., & Huang, J. (2021). Genome-wide identification and functional characterization of CCHC-type zinc finger genes in Ustilaginoidea virens. Journal of Fungi, 7(11), 947. https://doi.org/10.3390/jof7110947
- Couto, E. A. A., Dias-Arieira, C. R., Kath, J., Homiak, J. A., & Puerari, H. H. (2016). Boron and zinc inhibit embryonic development, hatching and reproduction of Meloidogyne incognita. Acta Agriculturae Scandinavica Section B: Soil and Plant Science, 66(4), 346–352. https://doi.org/10.1080/09064710.2015.1118154
- Deepika, S., Manoranjitham, S. K., Sendhilvel, V., & Karthikeyan, G. (2021). Foliar nutrition enhances the host immunity against papaya ringspot virus. The Pharma Innovation, 10(11), 165–169.
- Dordas, C. (2008). Role of nutrients in controlling plant diseases in sustainable agriculture. A review. Agronomy for Sustainable Development, 28(1), 33–46. https://doi.org/10.1051/agro:2007051
- Duffy, B. (2007). Zinc and plant disease. In L. E. Datnoff, W. H. Elmer, & D. M. Huber (Eds.), Mineral Nutrition and Plant Disease (pp. 155–178). St. Paul, MN: APS Press.
- Dumanović, J., Nepovimova, E., Natić, M., Kuča, K., & Jaćević, V. (2021). The significance of reactive oxygen species and antioxidant defence system in plants: A concise overview. Frontiers in Plant Science, 11, 552969. https://doi.org/10.3389/fpls.2020.552969
- Dutta, S., Ghosh, P. P., Ghorai, A. K., Roy, M. D., & Das, S. (2017). Micronutrients and plant disease suppression. Fertilizers and Environment News, 3(2), 5–9.
- El-Fawy, M., & El-Said, M. (2018). Effect of foliar application of some zinc and phosphorus sources on controlling Helminthosporium leaf spot disease and production of Sesame. Journal of Plant Protection and Pathology, 9(3), 201–207. https://doi.org/10.21608/jppp.2018.41386
- Ficke, A., Cowger, C., Bergstrom, G., & Brodal, G. (2018). Understanding yield loss and pathogen biology to improve disease management: Septoria nodorum blotch-a case study in wheat. Plant Disease, 102(4), 696–707. https://doi.org/10.1094/PDIS-09-17-1375-FE
- Gaunt, R. E. (1995). The relationship between plant disease severity and yield. Annual Review of Phytopathology, 33(1), 119–144. https://doi.org/10.1146/annurev.py.33.090195.001003
- Glazebrook, J. (2005). Contrasting mechanisms of defence against biotrophic and necrotrophic pathogens. Annual Review of Phytopathology, 43(1), 205. https://doi.org/10.1146/annurev.phyto.43.040204.135923
- Graham, R. D. (2008). Micronutrient deficiencies in crops and their global significance. In B. J. Alloway (Ed.), Micronutrient deficiencies in global crop production (pp. 221–276). Springer. https://doi.org/10.1016/S0065-2296(08)60261-X
- Gupta, N., Debnath, S., Sharma, S., Sharma, P., & Purohit, J. (2017). Role of nutrients in controlling the plant diseases in sustainable agriculture. In V. Singh Meena, P. Kumar Mishra, J. Kumar Bisht, & A. Pattanayak (Eds.), Agriculturally important microbes for sustainable agriculture (pp. 217–262). Springer. https://doi.org/10.1007/978-981-10-5343-6_8
- Gupta, S. K., Rai, A. K., Kanwar, S. S., Sharma, T. R., & Zhang, T. (2012). Comparative analysis of zinc finger proteins involved in plant disease resistance. PLoS One, 7(8), e42578. https://doi.org/10.1371/journal.pone.0042578
- Hassinen, V. H., Tervahauta, A. I., Schat, H., & Kärenlampi, S. O. (2011). Plant metallothioneins - metal chelators with ROS scavenging activity? Plant Biology, 13(2), 225–232. https://doi.org/10.1111/j.1438-8677.2010.00398.x
- Helfenstein, J., Pawlowski, M. L., Hill, C. B., Stewart, J., Lagos-Kutz, D., Bowen, C. R., Frossard, E., & Hartman, G. L. (2015). Zinc deficiency alters soybean susceptibility to pathogens and pests. Journal of Plant Nutrition and Soil Science, 178(6), 896–903. https://doi.org/10.1002/jpln.201500146
- Huber, D. M., & Haneklaus, S. (2007). Managing nutrition to control plant disease. Landbauforschung Volkenrode, 57(4), 313.
- Ibrahim, H. A., Ibrahim, M. F. M., & Bondok, A. M. (2016). Improving growth, yield and resistance to viral diseases of potato plants through modifying some metabolites using Zinc Sulphate and Jasmonic Acid. Journal of Horticultural Science & Ornamental Plants, 8(3), 161–172.
- Islam, M. R., Ali, M. A., Islam, M. S., Golam, A. F. M., & Hossain, G. F. (2002). Effect of nutrients and weeding on the incidence of mung bean mosaic. Pakistan Journal of Plant Pathology, 1(2), 48–50. https://doi.org/10.3923/ppj.2002.48.50
- Jackson, C., Dench, J., Moore, A. L., Halliwell, B., Foyer, C. H., & Hall, D. O. (1978). Subcellular localisation and identification of superoxide dismutase in the leaves of higher plants. European Journal of Biochemistry, 91(2), 339–344. https://doi.org/10.1111/j.1432-1033.1978.tb12685.x
- Jain, R., Srivastava, S., Solomon, S., Shrivastava, A. K., & Chandra, A. (2010). Impact of excess zinc on growth parameters, cell division, nutrient accumulation, photosynthetic pigments and oxidative stress of sugarcane (Saccharum spp.). Acta Physiologiae Plantarum, 32(5), 979–986. https://doi.org/10.1007/s11738-010-0487-9
- Kalsoom, H., Ali, S., Sahi, G. M., Habib, A., Zeshan, M. A., Anjum, R., Yousaf, M., & Abdullah, A. (2019). Differential response of micronutrients and novel insecticides to reduce cotton leaf curl virus disease and its vector in Gossypium hirsutum varieties. International Journal of Agriculture and Biology, 22(6), 1507–1512.
- Khoshgoftarmanesh, A. H., Kabiri, S., Shariatmadari, H., Sharifnabi, B., & Schulin, R. (2010). Zinc nutrition effect on the tolerance of wheat genotypes to Fusarium root-rot disease in a solution culture experiment. Soil Science & Plant Nutrition, 56(2), 234–243. https://doi.org/10.1111/j.1747-0765.2009.00441.x
- Kim, S., Ahn, I. -P., & Lee, Y. -H. (2001). Analysis of genes expressed during rice - Magnaporthe grisea interactions. Molecular Plant-Microbe Interactions, 14(11), 1340–1346. https://doi.org/10.1094/MPMI.2001.14.11.1340
- Kumar, D., Rampuria, S., Singh, N. K., & Kirti, P. B. (2016). A novel zinc-binding alcohol dehydrogenase 2 from Arachis diogoi, expressed in resistance responses against late leaf spot pathogen, induces cell death when transexpressed in tobacco. FEBS Open Bio, 6(3), 200–210. https://doi.org/10.1002/2211-5463.12040
- Li, Z., Fan, Y., Gao, L., Cao, X., Ye, J., & Li, G. (2016). The dual roles of zinc sulfate in mitigating Peach gummosis. Plant Disease, 100(2), 345–351. https://doi.org/10.1094/PDIS-01-15-0131-RE
- Lucero, G., Boiteux, J., Pizzuolo, P., & Hapon, M. V. (2014). Effect of copper, zinc and potassium phosphates on the mycelium growth of Phytophthora nicotianae in olive tree dry branch disease. Acta horticulturae, 1057(1057), 437–442. https://doi.org/10.17660/ActaHortic.2014.1057.55
- Machado, P. P., Steiner, F., Zuffo, A. M., & Machado, R. A. (2018). Could the supply of boron and zinc improve resistance of potato to early blight? Potato Research, 61(2), 169–182. https://doi.org/10.1007/s11540-018-9365-4
- Malandrakis, A. A., Kavroulakis, N., & Chrysikopoulos, C. V. (2019). Use of copper, silver and zinc nanoparticles against foliar and soil-borne plant pathogens. The Science of the Total Environment, 670, 292–299. https://doi.org/10.1016/j.scitotenv.2019.03.210
- Marschner, P. (2012). Marschner's mineral nutrition of higher plants (pp. 684). Amsterdam, Netherlands: Elsevier/Academic Press. -0-63043-9
- Martos, S., Gallego, B., Cabot, C., Llugany, M., Barceló, J., & Poschenrieder, C. (2016). Zinc triggers signalling mechanisms and defence responses promoting resistance to Alternaria brassicicola in Arabidopsis thaliana. Plant Science, 249, 13–24. https://doi.org/10.1016/j.plantsci.2016.05.001
- Mccall, K. A., Huang, C. -C., & Fierke, C. A. (2000). Function and mechanism of zinc metalloenzymes. In Zinc and Health: Current status and future directions. The Journal of Nutrition, 130(5), 1437–1446. https://doi.org/10.1093/jn/130.5.1437S
- Noman, A., Aqeel, M., Khalid, N., Islam, W., Sanaullah, T., Anwar, M., Khan, S., Ye, W., & Lou, Y. (2019). Zinc finger protein transcription factors: Integrated line of action for plant antimicrobial activity. Microbial Pathogenesis, 132, 141–149. https://doi.org/10.1016/j.micpath.2019.04.042
- Oh, S. K., Jeong, M. P., Young, H. J., Lee, S., Chung, E., Kim, S. Y., Choi, D., & Choi, D. (2005). A plant EPF-type zinc-finger protein, CaPIF1, involved in defence against pathogens. Molecular Plant Pathology, 6(3), 269–285. https://doi.org/10.1111/j.1364-3703.2005.00284.x
- Pan, L., Zhao, L., Jiang, W., Wang, M., Chen, X., Shen, X., Yin C, & Mao, Z. (2022). Effect of zinc oxide nanoparticles on the growth of Malus hupehensis Rehd. seedlings. Frontiers in Environmental Science, 10, 1–14. https://doi.org/10.3389/fenvs.2022.835194
- Quaglia, M., Bocchini, M., Orfei, B., D’amato, R., Famiani, F., Moretti, C., & Buonaurio, R. (2021). Zinc phosphate protects tomato plants against Pseudomonas syringae pv. Tomato Journal of Plant Diseases and Protection, 128(4), 989–998. https://doi.org/10.1007/s41348-021-00444-z
- Quaglia, M., Troni, E., D’amato, R., Ederli, L., & Pastor, V. (2022). Effect of zinc imbalance and salicylic acid co‐supply on Arabidopsis response to fungal pathogens with different lifestyles. Plant Biology, 24(1), 30–40. https://doi.org/10.1111/plb.13344
- Rema, L. P., & Philip, B. (1996). Metallothionein or metallothionein like proteins and heavy metal toxicity in Oreochromis mossambicus (Peters). Indian Journal of Experimental Biology, 34(6), 527–530.
- Ruttkay-Nedecky, B., Nejdl, L., Gumulec, J., Zitka, O., Masarik, M., Eckschlager, T., Stiborova, M., Adam, V., & Kizek, R. (2013). The role of metallothionein in oxidative stress. International Journal of Molecular Sciences, 14(3), 6044–6066. https://doi.org/10.3390/ijms14036044
- Savi, G. D., Bortoluzzi, A. J., & Scussel, V. M. (2013). Antifungal properties of Zinc-compounds against toxigenic fungi and mycotoxin. International Journal of Food Science and Technology, 48(9), 1834–1840. https://doi.org/10.1111/ijfs.12158
- Segal, L. M., & Wilson, R. A. (2018). Reactive oxygen species metabolism and plant-fungal interactions. Fungal Genetics and Biology, 110, 1–9. https://doi.org/10.1016/j.fgb.2017.12.003
- Sharma, P., Jha, A. B., Dubey, R. S., & Pessarakli, M. (2012). Reactive oxygen species, oxidative damage and antioxidative defence mechanism in plants under stressful conditions. Journal of Botany, 2012, 1–26. https://doi.org/10.1155/2012/217037
- Shaukat, S. S., & Siddiqui, I. A. (2003). Zinc improves biocontrol of meloidogyne javanica by the antagonistic Rhizobia. Pakistan Journal of Biological Sciences, 6(6), 575–579. https://doi.org/10.3923/pjbs.2003.575.579
- Shoaib, A., Akhtar, M., Javaid, A., Ali, H., Nisar, Z., & Javed, S. (2021). Antifungal potential of zinc against leaf spot disease in chili pepper caused by Alternaria alternata. Physiology and Molecular Biology of Plants, 27(6), 1361–1376. https://doi.org/10.1007/s12298-021-01004-3
- Siddiqui, Z. A., Khan, M. R., AbdAllah, E. F., & Parveen, A. (2018). Titanium dioxide and zinc oxide nanoparticles affect some bacterial diseases and growth and physiological changes of beetroot. International Journal of Vegetable Science, 25(5), 409–430. https://doi.org/10.1080/19315260.2018.1523267
- Siddiqui, Z. A., Khan, A., Khan, M. R., & Abd-Allah, E. F. (2018). Effects of zinc oxide nanoparticles (ZnO NPs) and some plant pathogens on the growth and nodulation of lentil (lens culinaris Medik.). Acta Phytopathologica Et Entomologica Hungarica, 53(2), 195–212. https://doi.org/10.1556/038.53.2018.012
- Simoglou, K. B., & Dordas, C. (2006). Effect of foliar applied boron, manganese and zinc on tan spot in winter durum wheat. Crop Protection, 25(7), 657–663.
- Sofy, A. R., Sofy, M. R., Hmed, A. A., Dawoud, R. A., Alnaggar, A. E. A. M., Soliman, A. M., & El-Dougdoug, N. K. (2021). Ameliorating the adverse effects of tomato mosaic tobamovirus infecting tomato plants in Egypt by boosting immunity in tomato plants using zinc oxide nanoparticles. Molecules, 26(5), 1–18. https://doi.org/10.3390/molecules26051337
- Streeter, T. C., Rengel, Z., Neate, S. M., & Graham, R. D. (2001). Zinc fertilisation increases tolerance to Rhizoctonia solani (AG 8) in Medicago truncatula. Plant and Soil, 228(2), 233–242. https://doi.org/10.1023/A:1004874027331
- Subramanian, B., Bansal, V. K., & Kav, N. N. V. (2005). Proteome-level investigation of Brassica carinata-derived resistance to Leptosphaeria maculans. Journal of Agricultural and Food Chemistry, 53(2), 313–324. https://doi.org/10.1021/jf048922z
- Tronchet, M., BalaguÉ, C., Kroj, T., Jouanin, L., & Roby, D. (2010). Cinnamyl alcohol Dehydrogenases-C and D, key enzymes in lignin biosynthesis, play an essential role in disease resistance in Arabidopsis. Molecular Plant Pathology, 11(1), 83–92. https://doi.org/10.1111/j.1364-3703.2009.00578.x
- Wadhwa, N., Joshi, U. N., & Mehta, N. (2014). Zinc induced enzymatic defense mechanisms in rhizoctonia root rot infected cluster bean seedlings. Journal of Botany, 2014, 1–7. https://doi.org/10.1155/2014/735760
- Wang, Y., Li, X., Fan, B., Zhu, C., & Chen, Z. (2021). Regulation and function of defence-related callose deposition in plants. International Journal of Molecular Sciences, 22(5), 2393. https://doi.org/10.3390/ijms22052393
- Wongpia, A., & Lomthaisong, K. (2010). Changes in the 2DE protein profiles of chilli pepper (Capsicum annuum) leaves in response to Fusarium oxysporum infection. ScienceAsia, 36(4), 259–270. https://doi.org/10.2306/scienceasia1513-1874.2010.36.259
- Yang, M., Zhang, F., Wang, F., Dong, Z., Cao, Q., & Chen, M. (2015). Characterization of a type 1 metallothionein gene from the stresses-tolerant plant Ziziphus jujuba. International Journal of Molecular Sciences, 16(8), 16750–16762. https://doi.org/10.3390/ijms160816750
- Zhang, Z., Chen, Y., Li, B., Chen, T., & Tian, S. (2020). Reactive oxygen species: A generalist in regulating development and pathogenicity of phytopathogenic fungi. Computational and Structural Biotechnology Journal, 18, 3344–3349. https://doi.org/10.1016/j.csbj.2020.10.024
- Zhao, T., Wu, T., Zhang, J., Wang, Z., Pei, T., Yang, H., Li J, & Xu, X. (2020). Genome-wide analyses of the genetic screening of C2H2-Type zinc finger transcription factors and abiotic and biotic stress responses in Tomato (Solanum lycopersicum) based on RNA-Seq data. Frontiers in Genetics, 11, 1–17. https://doi.org/10.3389/fgene.2020.00540