Abstract
Cassia tora Linn. is a medicinal herb that has traditionally been used to prevent diseases. The study investigated the changes in bioactive compounds of Cassia tora seeds during 3-days of germination. The seeds were thoroughly cleaned, soaked in deionized water at 20 °C for 2 h, and germinated at 25 °C and 90% relative humidity in a seed germinator. Germinating C. tora seeds were picked up at 24 h intermissions over 3-day germination duration. The germinating C. tora was freeze-dried and pulverized through a 1 mm mesh screen. During the germination process, the crude ash, crude fat, and crude protein content of C. tora seeds increased slightly. The main carotenoid compounds identified in this study were lutein and β-carotenes, and the total carotenoid content increased up to 4.35 µg/g after 72-hours of germination, which was 1.73-times higher compared to that in raw C. tora seeds. The anthocyanin and vitamin C content also increased during the germination period, being 1.44-times and 1.72-times, respectively, higher after 72-hours of germination than that in the raw C. tora seeds. In addition, phenolics and flavonoids in C. tora seeds had a tendency to increase after germination, subsequently increasing in vitro antioxidant activities (ABTS and DPPH-radical scavenging activities). Proper germination of C. tora might be a convenient and efficient method to increase antioxidant bioactive compounds in our diet to exploit the related health benefits.
1. Introduction
Cassia tora Linn. is a wild plant species in the family Leguminosae, native to Central America; its common English name is sickle senna. Whole plants, seeds, leaves, and roots have been widely used in folk medicine in East Asia. The seeds are edible, and roasted seeds can be used as a substitute for coffee (Mostafa et al., Citation2021). C. tora tea is used in Korea to rejuvenate human vision (Jain & Patil, Citation2010); furthermore, it is used to treat arthritis, snakebites, swelling, and skin diseases (leprosy, ringworm, itching, and psoriasis). In addition, C. tora seeds have antidiabetic (Ko et al., Citation2020), antifungal (Kim et al., Citation2004), antihepatotoxic (Wong et al., Citation1989), antimutagenic (Choi et al., Citation1997), antigenotoxic (Wu & Yen, Citation2004), and larvicidal effects (Vats, Citation2018). Anthraquinone found in C. tora is also used in Ayurvedic medicine (Jain & Patil, Citation2010). Moreover, C. tora seeds contain chrysophanic acid used to treat wounds (Joshi, Citation2000), skin diseases (eczema, ringworms, and scabies), gastrointestinal disorders (uterus disorders, and ulcers) (Elujoba et al., Citation1999), jaundice, rheumatism, and anorexia (Pieme et al., Citation2006).
C. tora seeds contain bioactive compounds, particularly phenolics (Hatano et al., Citation1999), flavonoids, anthocyanins, and antioxidants (Vats & Kamal, Citation2014). The main constituents of C. tora seeds are anthraquinone and naphthalene (Choi et al., Citation1997). C. tora contains 12.6% moisture, 13.4% crude protein, 7.2% crude fat, 5.2% ash, 48.3% soluble fiber, and 8.8% insoluble fiber (Joshi, Citation2000).
Germination of grains and legumes increases phytochemicals and antioxidant activity, extending the health benefits (Islam et al., Citation2022; Nkhata et al., Citation2018). Seed priming with water can increase enzymatic synthesis and metabolic activity in seeds during germination (Zhou et al., Citation2021). Furthermore, metabolic nutrients and their functions, bioactive phytochemicals, and antioxidants increase (Islam et al., Citation2022). Germination is a safe, inexpensive, environmentally friendly, and effective way to add useful components to plant-based diets.
Optimum germination conditions for grains and legumes are important for improving their phytochemicals and functional activities. For example, the phytochemicals of C. tora have antibacterial and radical scavenging effects (Hatano et al., Citation1999), and prevent eye diseases and intestinal disorders. However, there are very few studies on the influence of germination on the bioactive phytochemicals of C. tora seeds. Therefore, this study conducted the changes in the antioxidative bioactive compounds of C. tora seeds during germination.
2. Materials and methods
2.1. Cassia tora seeds and germination process
The C. tora Linn. seeds (harvested in 2019) used in this experiment were purchased from Sohwa Farm (Yecheon, Korea). The seeds were thoroughly cleaned, soaked in deionized water at 20 °C for 2 h, and germinated at 25 °C and 90% relative humidity in a seed germinator (Dasol Scientific Co., Ltd. Hwaseong, Korea). Germinating C. tora seeds were picked up at 24 h intermissions over a 3-day germination duration. The germinating C. tora was freeze-dried (Ilshin Lab, Dongducheon, Korea) and pulverized through a 1 mm mesh screen (MF 10, IKA-WERKE, Germany).
2.2. Proximate analysis
Moisture, crude ash, crude fat, and crude protein contents were determined using the AACC approved methods 44–15A, 08–01, 30–10, and 46–13, respectively (AACC, Citation2000).
2.3. Analysis of carotenoid content
Carotenoids were extracted and analyzed based on the Kim et al. (Citation2020) method. Carotenoids were released from the germinated C. tora seed powder (50 mg) by adding ethanol (3 mL) containing ascorbic acid (0.1%, w/v), and vortexing for 20 s. The carotenoids (µg/g) were separated on a C30 YMC column (250 × 4.6 mm, 3 µm; YMC Co., Kyoto, Japan) by High Performance Liquid Chromatography (Agilent 1100 HPLC instrument, Santa Clara, CA, USA) equipped with a diode-array detector at 450 nm wavelength with 40 °C column temperature.
2.4. Total anthocyanin content
Two grams of C. tora samples were extracted for one day (24 h) at low temperature (4 °C) in the dark with 10 mL of 1.5 N HCl-95% ethanol (15:85, v/v). The total anthocyanin content was determined using a spectrophotometer (Beijing Karaltay Scientific Instruments Co., Ltd, Beijing, China) at 530 nm. Anthocyanin content was computed using the following formula: Anthocyanin (µg/mL) = (absorbance at 530 nm × volume of extraction solution in mL × 100)/(volume of sample in mL × 98.2), where 98.2 is the molar absorption value of the HCl-ethanol solvent.
2.5. Total vitamin C content
Two grams of C. tora samples were extracted with 20 mL distilled water at 4 °C for 2 h. C. tora seed extract (2 mL) was mixed with metaphosphoric acid (5 mL of 5%; w/v) for 30 min and centrifuged. The absorbance of the supernatant was measured at 525 nm using a spectrophotometer to calculate the total vitamin C (μg/g).
2.6. Total phenolic content
The total phenolic content was measured according to the technique described by Islam et al. (Citation2022) with slight modifications. Two grams of C. tora samples were extracted for 2 h at 20 °C in 20 mL distilled water or in 20 mL 80% ethanol. The extracts were centrifuged for 30 min at 3,500 × g, and the supernatants were analyzed. Supernatant (0.2 mL) and deionized water (0.8 mL) were mixed with 2 N Folin and Ciocalteu reagent (0.2 mL) at 20 °C for 5 min. This mixture was incubated for 1 h by adding sodium carbonate (7%, 2 mL) to measure the absorbance at 734 nm using a spectrophotometer. A gallic acid was used to prepare the standard curve to calculate the total phenolic content (μg/g).
2.7. Total flavonoid content
The total flavonoid content was measured according to the technique described by Pękal and Pyrzynska (Citation2014), with a slight modification. Two grams of C. tora samples were extracted at 20 °C for 2 h in 20 mL distilled water or in 20 mL 80% ethanol. Extract (0.5 mL), ethanol (1.5 mL), aluminum nitrate (0.1 mL of 10%), potassium acetate (0.1 mL of 1 M), and 2.8 mL of deionized water were mixed in a test tube. The mixture was reacted for 40 min at 20 °C. The absorbance was measured at 415 nm using a UV-spectrophotometer. A quercetin was used to prepare the standard curve to calculate the total flavonoid content (μg/g).
2.8. In vitro antioxidant activity
2.8.1 DPPH radical scavenging activity
DPPH radical scavenging activity was determined according to the technique described by Islam et al. (Citation2022) with slight modifications. Two grams of C. tora samples were extracted at 20 °C for 2 h in 20 mL distilled water or in 20 mL 80% ethanol. The extracts were centrifuged for 30 min at 3,500 × g, and the supernatants were analyzed. Supernatant (0.2 mL) and methanol containing a DPPH (0.4 mM) solution (0.8 mL) were mixed for 10 s and left to stand for 10 min in the dark. The absorbance was measured at 525 nm using a spectrophotometer. The scavenging activity was calculated using the following formula:
DPPH radical scavenging activity (%) = (A − B)/A × 100
where A is the absorbance of DPPH radical + methanol and B is the absorbance of DPPH radical + sample extract.
2.8.2. ABTS radical scavenging activity
ABTS radical scavenging activity was determined according to the technique described by Re et al. (Citation1999) with slight modifications. Two grams of C. tora samples were extracted at 20 °C for 2 h in 20 mL distilled water or 80% ethanol. ABTS solution (1.9 mL) was mixed with 50 µL of standard or test extract. The samples were stored for 10 min in the dark at 20 °C before the absorbance was measured at 734 nm using a spectrophotometer. The percentage inhibition of ABTS was computed according to the following formula:
Inhibition of A734 (%) = (1 - AS/Ab) × 100, where AS is the absorbance of the sample extract and Ab is the absorbance of the blank.
2.9. Statistical analysis
Duncan’s multiple range test (SPSS V.25, SPSS Inc., Chicago, Illinois, USA) was used to test for statistical analysis at 5% (p ≤ 0.05) significance level.
3. Results and discussion
3.1. Proximate composition of Cassia tora seed during germination
The proximate composition of C. tora Linn. seed during 3-day germination is presented in Table . Before germination, the raw C. tora seeds contained 12.57% crude protein, 4.56% crude fat, and 4.48% crude ash. As per previous reports, C. tora Linn. seed had 13.1–13.4% protein, 7.2–9.4% fat, and 4.9–5.2% ash content (Hong et al., Citation2012; Kim et al., Citation1995). The crude protein content decreased after 24-h but increased to 14.00% after 72-h germination. In this experiment, the crude protein content increased. Crude protein content may increase owing to the synthesis of enzymatic proteins (Bau et al., Citation1997) and loss of dry weight (Uppal & Bains, Citation2012) after 72-h germination. The crude fat content was lower after 24-h germination than raw C. tora, but increased slightly between 48 to 72-h germination. Fat is a primary carbon source for seed growth (Bau et al., Citation1997). However, the increase in crude protein and fat contents after 48-h germination may result from the relatively high loss in total carbohydrates consumed during seed germination (Hung et al., Citation2020). The ash content gradually increased during the germination process, being 1.10-times higher after 72-h germination than the raw sample.
Table 1. Proximate composition (%) of Cassia tora seed during 3-day germination
3.2. Carotenoid contents of Cassia tora seed during germination
The carotenoid contents of C. tora seeds during germination are shown in Table . Three individual carotenoid compounds were identified in the raw samples, with the highest lutein content, followed by E-β-carotene and 9Z-β-carotene. The total carotenoid content in raw C. tora seed was 2.51 µg/g, close to 0.12 mg/g oil fraction reported by Górnaś et al. (Citation2018). The lutein content gradually increased during germination, being 1.51-times higher after 72-h compared to raw C. tora. Lutein is localized in the chloroplast or chromoplast (Calvo, Citation2005) and is released by the catalytic activity of hydroxylase from zeinoxanthin (Cazzonelli, Citation2011). An increase in lutein and its isomers content is important. The compounds play important roles in vision performance in young and later adulthood, in lowering the risk of the development of common age-related eye diseases in older age, and ocular development in utero and throughout the life span (Mares, Citation2016). Zeaxanthin and 13Z-β-carotene were not found until 48-h germination, but zeaxanthin (1.14 µg/g) and 13Z-β-carotene (0.18 µg/g) were detected after 72-h germination (Figure ). E-β-carotene and 9Z-β-carotene contents decreased slightly after 24–48-h germination but tended to increase again after 72-h germination. Before germination, the soaking process might cause a possible loss of carotenoids. Still, the content slowly increased during germination, reaching 4.35 µg/g at 72-h germination, 1.73-times higher compared to raw C. tora.
Figure 1. HPLC carotenoids chromatogram of Cassia tora seed at 0 h (A), 24 h (B), 48 h (C), and 72 h (D) after germination. Peak: 1. Lutein; 2. Zeaxanthin; 3. Trans-β-apo-8’-carotenal (internal standard); 4. 13Z-β-Carotene; 5. β-Carotene; 6. 9Z-β-Carotene.
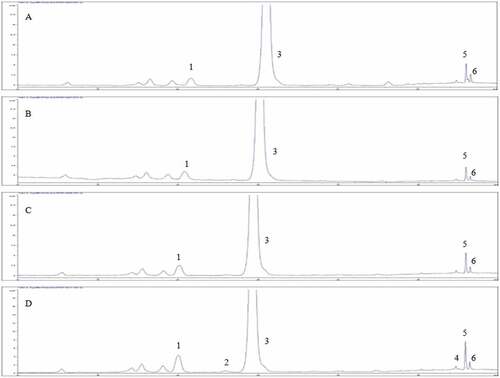
Table 2. Carotenoid content (µg/g) of Cassia tora seed during 3-day germination
3.3. Total anthocyanin and vitamin C contents of Cassia tora seed during germination
The total anthocyanin and vitamin C contents of C. tora seeds during germination are given in Table . Anthocyanin is a plant pigment produced in plant parts, especially in dark flowers, buds, roots, and seeds. Anthocyanin has anti-cancer, anti-inflammatory, and antiallergic effects owing to its excellent antioxidant value (Chen et al., Citation2016). The anthocyanin content gradually increased during germination, becoming 1.44-times higher at 72-h than in the raw C. tora seeds. During germination, soaking in water may influence the optimum conditions for enhancing the anthocyanin content through enzymatic reactions. Protocatechuic acids and vanillic acid are major accelerants or precursors of the anthocyanin biosynthesis pathway (Shao et al., Citation2018).
Table 3. Total anthocyanin and vitamin C content of Cassia tora seed during 3-day germination
The total vitamin C content gradually increased during germination and reached its highest after 72 hours, 1.72-times higher than that of raw seeds. Soaking may enable an increase in vitamin C content (Table ) due to de novo enzyme synthesis activated in the presence of water (Benincasa et al., Citation2019), thus contributing to ROS scavenging (Ramel et al., Citation2012).
3.4. Total phenolic and flavonoid content of Cassia tora seed during germination
Phenolic and flavonoid compounds are generally extracted using water, ethanol, methanol, or acetone. Distilled water and 80% ethanol were used as extraction solvents. The total phenolic content (Table ) extracted from raw C. tora using distilled water and ethanol were 2,122.80 and 2,865.30 µg/g, respectively. The total phenolic content of C. tora seed was 180.64 mg/g in the water extract (Yen & Chuang, Citation2000). The phenolic content in germinated C. tora seeds was 2,127.60–2,237.20 µg/g in water and 2,776.40–3,224.70 µg/g in ethanol extraction, similar to or higher than those of raw C. tora seeds. The ethanol extraction showed a higher yield than the water extraction, similar to the data result reported by Mehta et al. (Citation2017).
Table 4. Total phenolic (μg/g) and flavonoid (μg/g) contents of Cassia tora seed during 3-day germination
The total flavonoid contents of raw C. tora seed extracted using water and ethanol were 131.1 and 70.1 µg/g, respectively, and increased over 3-day germination. In both solvents, the total flavonoid content was the highest after 72-h germination. The result is similar to that of Fouad and Rehab (Citation2015), who found that germination process increased the total flavonoid content. Flavonoid levels increase because of the expression of flavone synthesis genes during the germination process (Galland et al., Citation2014). Ethanol extracts fewer flavonoids than water.
3.5. In vitro antioxidant activity of Cassia tora seed during germination
The antioxidant activities of C. tora during germination are shown in Table . DPPH scavenging activity was the lowest in raw C. tora seeds and gradually increased as the germination progressed. It was increased 1.10-time in water extract and 1.13-times in ethanol extract at 72 h for germinated seeds compared to raw C. tora seeds. The DPPH scavenging activity ranged from 71.48% to 79.13% for water extraction and 80.90% to 91.55% for ethanol extraction. Ethanol extraction showed a higher DPPH radical scavenging activity than water extraction, possibly due to ethanol’s increased mass transfer rate. ABTS radical scavenging activity did not show any significant differences in the water-extracted samples, but it slightly increased during germination in the ethanol-extracted samples. The increased value of ABTS was 1.04-time higher in an ethanol extract at 72 h for germinated seeds compared to raw C. tora seeds. ABTS radical scavenging activity was higher in both water and ethanol extracts than DPPH radical scavenging activity.
Table 5. Antioxidant activity (%) of Cassia tora seed during 3-day germination
ABTS and DPPH are very important assays applied to antioxidant measurement (Giuffrè et al., Citation2017a; Giuffrè et al., Citation2017b; Islam et al., Citation2022). During germination, seeds are modified by endogenous hydrolytic enzymes and they liberate bound compounds that may act as antioxidants (Alvarez-Jubete et al., Citation2010). The increase in ABTS and DPPH radical scavenging activities is closely related to the rise in phenolic and flavonoid compounds during germination. The growth in radical scavenging activity may also be partly due to the synthesis of vitamin C, and possessing antioxidant activity (Sharma & Gujral, Citation2010).
4. Conclusions
This study examined the effect of germination on the content of bioactive and antioxidative compounds in C. tora seeds. Bioactive compounds, including carotenoids, anthocyanins, vitamin C, phenolics, and flavonoids, increased as C. tora seeds germinated. This research contributes to developing new food products by enhancing antioxidative functional components in C. tora seeds by adopting an appropriate germination process. Also, further studies are needed to investigate more functional properties of bioactive phytochemicals from germinating C. tora seeds.
Disclosure statement
No potential conflict of interest was reported by the authors.
References
- Alvarez-Jubete, L., Wijngaard, H., Arendt, E. K., & Gallagher, E. (2010). Polyphenol composition and in vitro antioxidant activity of amaranth, quinoa buckwheat and wheat as affected by sprouting and baking. Food Chemistry, 1191, 770–9.
- American Association of Cereal Chemists. (2000). Approved Methods of AACC (10th ed.). AACC International, St Paul, MN.
- Bau, H., Villaume, C., Nicolas, J., & Mejean, L. (1997). Effect of germination on chemical composition, biochemical constituents and antinutritional factors of soya bean (Glycine max) seeds. Journal of the Science of Food and Agriculture, 73(1), 1–9.
- Benincasa, P., Falcinelli, B., Lutts, S., Stagnari, F., & Galieni, A. (2019). Sprouted Grains: A comprehensive review. Nutrients, 11(421), 1–29.
- Calvo, M. M. (2005). Lutein: A valuable ingredient of fruit and vegetables. Critical Reviews in Food Science and Nutrition, 45(7–8), 671–696.
- Cazzonelli, C. I. (2011). Carotenoids in nature: Insights from plants and beyond. Functional Plant Biology: FPB, 38(11), 833–847.
- Chen, H., Pu, J., Liu, D., Yu, W., Shao, Y., Yang, G., Xiang, Z., & He, N. (2016). Anti-inflammatory and antinociceptive properties of flavonoids from the fruits of black mulberry (Morus nigra L.). Plos One, 11(4), e0153080.
- Choi, J. S., Lee, H. J., Park, K. Y., Ha, O. J., & Kang, S. S. (1997). In vitro antimutagenic effects of anthraquinone aglycones and naphthopyrone glycosides from Cassia tora. Planta medica, 63(01), 11–14.
- Elujoba, A. A., Abere, A. T., & Adelusi, S. A. (1999). Laxative activities of Cassia pods sourced from Nigeria. Nigerian Journal of Natural Products and Medicine, 3(1), 51–53.
- Fouad, A. A., & Rehab, F. M. (2015). Effect of germination time on proximate analysis, bioactive compounds and antioxidant activity of lentil (Lens culinaris Medik.) sprouts. Acta Scientiarum Polonorum Technologia Alimentaria, 14(3), 233–246.
- Galland, M., Boutet-Mercey, S., Lounifi, I., Godin, B., Balzergue, S., Grandjean, O., Morin, H., Perreau, F., Debeaujon, I., & Rajjou, L. (2014). Compartmentation and dynamics of flavone metabolism in dry and germinated rice seeds. Plant & Cell Physiology, 55(9), 1646–1659.
- Giuffrè, A. M., Capocasale, M., & Zappia, C. (2017a). Tomato seed oil for edible use: Cold break, hot break, and harvest year effects. Journal of Food Processing and Preservation, 41(6), e13309.
- Giuffrè, A. M., Zappia, C., & Capocasale, M. (2017b). Physicochemical stability of blood orange juice during frozen storage. International Journal of Food Properties, 20(S2), S1930–1943.
- Górnaś, P., Czubinski, J., Rudzińska, M., Grygier, A., Ying, Q., Chakradhari, S., Sahu, P. K., Mišina, I., Urvaka, E., & Patel, K. S. (2018). Selected uncommon legumes as a source of essential fatty acids, tocopherols, tocotrienols, sterols, carotenoids, and squalene. Plant Foods for Human Nutrition, 74(1), 91–98.
- Hatano, T., Uebayashi, H., Ito, H., Shiota, S., Tsuchiya, T., & Yoshida, T. (1999). Phenolic constituents of Cassia seeds and antibacterial effect of some naphthalenes and anthraquinones on methicillin-resistant Staphylococcus aureus. Chemical & Pharmaceutical Bulletin, 47(8), 1121–1127.
- Hong, K. H., Choi, W. H., Ahn, J., Jung, C. H., & Ha, T. Y. (2012). Physicochemical properties of ethanol extracts and dietary fiber from Cassia tora L. seed. The Korean Journal of Food and Nutrition, 25(3), 612–619.
- Hung, P. V., Trinh, L. N. D., Thuy, N. T. X., & Morita, N. (2020). Changes in nutritional composition, enzyme activities and bioactive compounds of germinated buckwheat (Fagopyrum esculantum M.) under unchanged air and humidity conditions. International Journal of Food Science and Technology, 56(7), 3209–3217.
- Islam, M. Z., Shim, M. J., Jeong, S. Y., & Lee, Y.T. (2022). Effects of soaking and sprouting on bioactive compounds of black and red pigmented rice cultivars. International Journal of Food Science and Technology, 57(1), 201–209.
- Jain, S., & Patil, U. K. (2010). Phytochemical and pharmacological profile of Cassia tora Linn.– an overview. Indian Journal of Natural Products and Resources, 1(4), 430–437.
- Joshi, S. G. (2000). Caesalpiniaceae-Cassia auriculata. Textbook of medicinal plants, 3rd Edn. India Book House.
- Kim, T. J., Hyeon, H., Park, N. I., Yi, T. G., Lim, S. -H., Park, S. -Y., Ha, S. -H., & Kim, J. K. (2020). A high-throughput platform for interpretation of metabolite profile data from pepper (Capsicum) fruits of 13 phenotypes associated with different fruit maturity states. Food Chemistry, 331, 127286.
- Kim, Y. M., Lee, C. H., Kim, H. G., & Lee, H. S. (2004). Anthraquinones isolated from Cassia tora (Leguminosae) seed show an antifungal property against phytopathogenic fungi. Journal of Agricultural and Food Chemistry, 52(20), 6096–6100.
- Kim, J. K., Moon, K. D., Kang, W. W., & Kim, G. Y. (1995). Study on the organoleptic quality characteristics of Cassia tora teas by roasting conditions. Journal of the Korean Society of Food Culture, 10(4), 241–245.
- Ko, E., Um, M. Y., Choi, M., Han, T., Kim, I. H., & Shin, S. (2020). Cassia tora seed improves pancreatic mitochondrial function leading to recovery of glucose metabolism. The American Journal of Chinese Medicine, 48(03), 615–629.
- Mares, J. (2016). Lutein and zeaxanthin isomers in eye health and disease. Annual Review of Nutrition, 36, 571–602.
- Mehta, J. P., Parmar, P. H., Vadia, S. H., Patel, M. K., & Tripathi, C. B. (2017). In-vitro antioxidant and in-vivo anti-inflammatory activities of aerial parts of Cassia species. Arabian Journal of Chemistry, 10(S2), S1654–1662.
- Mostafa, M. M., Ali, E., Gamal, M., & Farag, M. A. (2021). How do coffee substitutes compare to coffee? A comprehensive review of its quality characteristics, sensory characters, phytochemicals, health benefits and safety. Food Bioscience, 43, 101290.
- Nkhata, S. G., Ayua, E., Kamau, E. H., & Shingiro, J. B. (2018). Fermentation and germination improve nutritional value of cereals and legumes through activation of endogenous enzymes. Food Science & Nutrition, 6(8), 2446–2458.
- Pękal, A., & Pyrzynska, K. (2014). Evaluation of aluminium complexation reaction for flavonoid content assay. Food Anal Methods, 7(9), 1776–1782.
- Pieme, C. A., Penlap, V. N., Nkegoum, B., Taziebou, P. C. L., Tekwu, E. M., Etoa, F. X., & Ngongang, J. (2006). Evaluation of acute and subacute toxicities of aqueous ethanolic extract of leaves of Senna alata (L.) Roxb (Ceasalpiniaceae). African Journal of Biotechnology, 5(3), 283–289.
- Ramel, F., Birtic, S., Cuiné, S., Triantaphylidès, C., Ravanat, J. L., & Havaux, M. (2012). Chemical quenching of singlet oxygen by carotenoids in plants. Plant Physiology, 158(3), 1267–1278.
- Re, R., Pellegrini, N., Proteggente, A., Pannala, A., Yang, M., & Rice-Evans, C. (1999). Antioxidant activity applying an improved ABTS radical cation decolorization assay. Free Radical Biology and Medicine, 26(9–10), 1231–1237.
- Shao, Y., Hu, Z., Yu, Y., Mou, R., Zhu, Z., & Beta, T. (2018). Phenolic acids, anthocyanins, proanthocyanidins, antioxidant activity, minerals and their correlations in non-pigmented, red, and black rice. Food Chemistry, 239, 733–741.
- Sharma, P., & Gujral, H. S. (2010). Antioxidant and polyphenols oxidase activity of germinated barley and its milling fractions. Food Chemistry, 120(3), 673–678.
- Uppal, V., & Bains, K. (2012). Effect of germination periods and hydrothermal treatments on in vitro protein and starch digestibility of germinated legumes. Journal of Food Science and Technology, 49(2), 184–191.
- Vats, S. (2018). Larvicidal activity and in vitro regulation of rotenoids from Cassia tora L. 3biotech, 8(1), 13.
- Vats, S., & Kamal, R. (2014). Identification of flavonoids and antioxidant potential of Cassia tora L. American Journal of Drug Discovery and Development, 4(1), 50–57.
- Wong, S. M., Wong, M. M., Seligmann, O., & Wagner, H. (1989). New antihepatotoxic naphtho-pyrone glycosides from the seeds of Cassia tora 1. Planta medica, 55(03), 276–280.
- Wu, C. H., & Yen, G. C. (2004). Antigenotoxic properties of Cassia tea (Cassia tora L.): Mechanism of action and the influence of roasting process. Life Sciences, 76(1), 85–101.
- Yen, G. C., & Chuang, D. Y. (2000). Antioxidant properties of water extracts from Cassia tora L. in relation to the degree of roasting. Journal of Agricultural and Food Chemistry, 48(7), 2760–2765.
- Zhou, M., Hassan, M. J., Peng, Y., Liu, L., Liu, W., Zhang, Y., & Li, Z. (2021). γ-Aminobutyric acid (GABA) priming improves seed germination and seedling stress tolerance associated with enhanced antioxidant metabolism, DREB expression, and dehydrin accumulation in white clover under water stress. Frontiers in Plant Science, 12, 2675.