Abstract
A novel coronavirus strain called SARS-CoV-2 first appeared in China in December 2019. Natural products are significant sources of prospective and new antiviral medications, and new antiviral drug research has advanced significantly in recent years. The current study allows us to select specific components of olive oil that are thought to be anti-SARS-CoV-2 and assess their impact on SARS-CoV-2 in vitro. The 26 compounds of olive oil were obtained from the PubChem database and docked against the RdRP of SARS-CoV-2 (pdb id: 6XQB) by autodock vina 1 1 2 linux x86 software. Cytotoxicity and antiviral activity were measured by the MTT assay protocol (the crystal violet method). The findings revealed that the range of the olive oil compound’s molecular docking binding affinity score against the RdRP SARS-CoV-2 target was 5.9–18.2 kcal/mol. The best compound is apigenin since it has a low energy value of −18.2 kcal/mol, followed by taxifolin, which has an energy value of −14.2 kcal/mol. On the other hand, the molecule with the lowest energy is believed to be the good one. Additionally, Lipinski’s criteria and AD-MET analysis supported the created apigenin and taxifolin’s status as a secure pharmaceutical substance. Also, apigenin and taxifolin showed moderate antiviral effectiveness against SARS-CoV-2 in vitro, with SI values of 9.7 and 8.79, respectively, compared with olive oil’s crude SI value of 9.57. According to our results, we think that olive oil is an essential source of cutting-edge SARS-CoV-2 antiviral drugs, especially apigenin and taxifolin compounds.
1. Introduction
SARS-CoV-2-caused coronavirus disease COVID-19 is fatal and causes large numbers of infections [Hui et al., Citation2019]. Several recognized and very effective COVID-19 vaccines were created in just one year globally. Because these vaccinations are not universally accessible, new SARS-CoV-2 variants have already developed and may impact the future efficacy of the currently available vaccines (Zumla et al., Citation2016). Parallel to this, more work is required to locate and improve alternative treatments for SARS-CoV-2-infected patients who do not respond to or cannot tolerate vaccines (Song et al., Citation2019). The membrane glycoprotein (M), spike protein (S), envelope protein (E), and nucleocapsid protein (N) are the four crucial proteins found in SARS-CoV-2 (Mahmoud et al., Citation2020). The N protein wraps the single stranded positive sense viral RNA, while the first three proteins are encased in a lipid bilayer (Jin et al., Citation2020). Nonstructural proteins (nsps) include nsps such as nsp12 for RNA-dependent RNA polymerase (RdRP), nsp3 for papain-like protease (PLpro), and nsp5 for the significant viral protease (Mpro) (Dai et al. Citation2020). RdRP is one enzyme that is necessary for the growth of RNA viruses. It has been targeted in several viral illnesses, including those brought on by HCoVs and the Zika virus (ZIKV) (A. A. Elfiky, Citation2017, Citation2019; A. Ganesan & Barakat, Citation2017). The RdRP active site is conserved across different species and is surrounded by a beta-turn motif and two consecutive, surface-exposed aspartate residues (A. A. A-H. Elfiky, Citation2020).
It was possible to find new therapeutic candidates to stop the replication of this virus once the molecular structure of RdRP SARS-CoV-2 (PDB ID: 6M71) was discovered in April 2020. Drug interactions are predicted to involve SARS-CoV-2 polymerase residues ARG 553, ARG 555, LYS 545, and ASN 691 (Afonine et al., Citation2018). Additionally, it is essential to discover novel therapeutic medications derived from sustainable natural sources during pandemics (El Gizawy et al., Citation2021; Elebeedy et al., Citation2021; Vicidomini et al., Citation2021). According to some natural bioactive chemicals’ descriptions, they can act as antiviral agents (Donalisio et al., Citation2013). According to (Owen et al., Citation2000), those who eat a diet high in olive oil, such as the Mediterranean diet, have lower odds of developing coronary heart disease and cancer. One of the fundamental elements of a Mediterranean diet with health-promoting properties is olive oil (Tapsell, Citation2014). It has been utilised for millennia because of its medicinal and preventative properties (Caramia et al., Citation2012; Scoditti et al., Citation2012). Atherosclerosis, serum lipoprotein levels, cardiovascular disease, obesity, oxidative stress, inflammation, type 2 diabetes, and cancer can all be prevented or treated using olive oil (Amiot-Carlin, Citation2014; Bulotta et al., Citation2014; Cicerale et al., Citation2010; Gorzynik-Debicka et al., Citation2018; Wani et al., Citation2018).
The chemical makeup of olive oil underlies its ability to safeguard health (Al-Mokadem et al., Citation2022; Bulotta et al., Citation2014; Perez-Martinez et al., Citation2011; Scoditti et al., Citation2012; Wani et al., Citation2018). Olive oil’s chemical composition may be divided into major and minor components. The amount of fatty acids in olive oil is one of the main components. Oleic acid is the main monounsaturated fatty acid (MUFA) in olive oil (major components) (Scoditti et al., Citation2012). More than 230 minor chemical compounds make up the almost 2% of the weight of olive oil that is made up of minor components (Scoditti et al., Citation2012). These chemical compounds include pigments, volatile compounds, aliphatic alcohols, phenolic, sterols, hydrocarbons, and triterpene alcohols compounds (Caramia et al., Citation2012; Scoditti et al., Citation2012). At least 30 phenolic compounds are present in olive oil. These include lignans, phenolic alcohols, phenolic acids, secoiridoids, flavones, and their derivatives (Bendini et al., Citation2007; Cerretani et al., Citation2009; Scoditti et al., Citation2012).
Simple phenols, secoiridoids, oleuropein, the ligstroside aglycone, and their respective decarboxylated dialdehyde derivatives, along with lignans [(1)-1-acetoxypinoresinol and pinoresinol], are the three main types of phenolic compounds that are present in varying levels in olive oil (Bonoli et al., Citation2003; Owen et al., Citation2000). Strong antioxidant properties are present in all three varieties (Owen et al., Citation2000). In experiments against coronaviruses, flavonoids demonstrated antiviral and immunomodulatory effects (Ngwa et al., Citation0000). Therefore, flavonoids’ antiviral effects may help combat the present COVID-19 outbreak. Apigenin and taxifolin are the compounds of olive oil (Bendini et al., Citation2007). Apigenin has antihyperglycemic (Villa-Rodriguez et al., Citation2018), antioxidant (Fidelis et al., Citation2019), anti-apoptotic effects (Wang et al., Citation2018), and anti-inflammatory (Lim et al., Citation2013). Recent papers have also demonstrated antiviral efficacy of apigenin against African swine fever virus (AFSV) (Hakobyan et al., Citation2016), hepatitis C virus (HCV) (Shibata et al., Citation2014), Epstein–Barr virus (EBV) (Wu et al., Citation2017), and picornaviruses (Dai et al., Citation2019; Qian et al., Citation2015; ZeinEldin et al., Citation2023; Zhang et al., Citation2014). A flavonoid called taxifolin has drawn interest due to its potent pharmacological effects (Asmi et al., Citation2017; Sunil & Xu, Citation2019), along with anticancer activities such as the prevention of cytochrome P450 enzymes, angiogenesis, P-glycoprotein, cell cycle regulators, and reactive oxidative species (ROS), as well as apoptosis triggering (Butler, Citation2004). Computational drug design is one of the most effective techniques in drug discovery processes (Soltan et al., Citation2021). The discovery of novel medications is greatly accelerated by molecular docking studies (Al-Karmalawy & Khattab, Citation2020; Khattab & Al‐Karmalawy, Citation2021). The novelty and significance of our research are to investigate fatty acids and phenolic compounds of olive oil as a potential treatment for the SARS-CoV-2 virus by computational methods to lower the cost and in vitro for sure results. In the current study, we have experimented with the antiviral efficacy of olive oil crude, apigenin, and taxifolin against SARS-CoV-2 using in silico (docking, pharmacokinetics, and ADMET) and in-vitro (MTT assay protocol).
2. Results
2.1. Computational screening and molecular docking
The Protein Data Bank (PDB ID: 6XQB) has the RdRP SARS-CoV-2 protein with resolution (3.4) in Figure and Table , while PubChem has the 3D structures of the fatty acids and phenolic compounds in olive oil. Figure and Table showed that data for various ligands and the chemical structure, PubChem id, Smile, and affinity scores of the compounds apigenin and taxifolin were stored in supplemental file S1. The range of the olive oil compound’s molecular docking binding affinity score against the RdRP SARS-CoV-2 target was −5.9 to −18.2 kcal/mol. The best complex, however, is apigenin since it has a low energy value of −18.2 kcal/mol, followed by taxifolin, which has an energy value of −14.2 kcal/mol. On the other hand, the molecule with the lowest energy is believed to be the good one. Software from the drug discovery studio is used to predict and show the interactions between olive oil molecules as ligands and the RdRb protein, which is a target of SARS-CoV-2. In the case of apigenin molecules, Figure demonstrated van der Wall interactions with the residues VAL 128, TYR 129, SER 239, PRO 243, THR 701, ASN 705, LEU 708, and GLN 789, as well as conventional hydrogen bonds with the residues GLN 468 AND TYR 732 and Pi Alkyl type interactions with the residues ARG 132 and LEU 240. Additionally, visualization of docking of taxifolin molecules with the RdRP protein provides binding styles of protein with green color for amino acid residues intricate in van der Waals interaction with residues PHE 45, ARG 132, HIS 133, LEU 240, CYS 464, GLN 468, LEU 469, VAL 472, LEU 630, CYS 697, THR 701, ASN 705, LEU 708, VAL 785, TY.
Figure 1. 3-Dimensional structures of RdRP SARS CoV-2 target proteins. 1- green color: RNA direct RNA polymerase (chain A), 2- brown and violet color non-structure protein 8 (chain B and chain D), 3- pink color: non-structure protein 7 (Chain C).
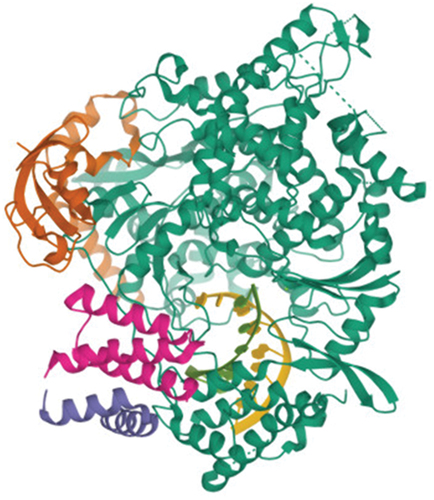
Figure 3. H-bond and interactions between ligands and RdRP SARS2-CoV-2. (a) apigenin interaction, (b) apigenin H-bond, (c) taxifolin interaction and (d) taxifolin H-bond.
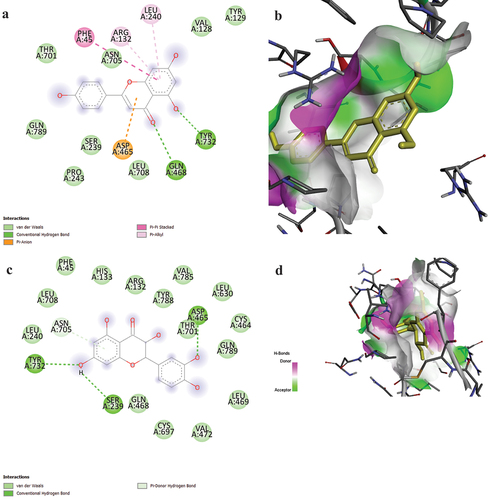
Table 1. Affinity score, PubChem ID, and Smile of olive oil molecules which are top ranked in docking
2.2. ADMET & Lipinski’s rules
It is necessary to look at the pharmacokinetic properties of novel molecules before they may be identified as prospective drugs. Therefore, utilizing in silico methodologies and the online services provided by Pkcsm, the assessment of ADMET attributes is thought to be the best way to check for and select the target molecules.
2.2.1. Absorption
According to a review of the literature, substances that produce outcomes (>30% abs) are more potent at penetrating the intestinal barrier (Norinder et al., 2006). The findings reveal that the highly ranked compounds investigated had high values; apigenin had a human oral absorption percentage of 93.25%, and taxifolin had a value of 64.71%, ranging from 43.73% to 96.42% (table S1 in the results), showing that these substances were well absorbed.
2.2.2. Distribution
Apigenin and taxifolin, two molecules found in olive oil that are of interest, were predicted by distribution values to have low values of −0.73 and −0.72 in Blood-Brain Barrier Permeability, respectively, indicating that they do not penetrate the brain. As a result, adverse effects will be diminished, demonstrating their effectiveness and drug-like behavior (Abdelrheem et al., Citation2021).
2.2.3. Metabolism
Computing metabolic activity can be used to determine how a drug is chemically transformed in the body; CYP plays a vital role in the conversion of pharmacological compounds. Apigenin and taxifolin are substrates and inhibitors of the two main CYP subtypes, 2D6 and 3A4, as seen in Table (Abdelrheem et al., Citation2021; Pauwels et al., Citation1988; Tabti et al., Citation2021). The liver may be able to metabolize them.
Table 2. The ADMET test with pKCSM of apigenin and taxifolin compound
2.2.4. Excretion and toxicity
The excretion result on organic cation transport 2 (OCT2) and the AMES toxicity test for all ligands and apigenin and taxifolin compounds indicate an acceptable negative value. Parallel to this, apigenin and taxifolin compounds are non-toxic regarding hepatotoxicity, which supports their efficacy. Figures and S1.
2.2.5. Lipinski’s rule
All the olive oil molecules used in this study were the safest and least poisonous ones imaginable, according to the results of the ADMET method’s toxicity testing. Furthermore, it is clear from Table , and S1 show that all these compounds satisfy the conditions of Lipinski’s rule: they created 10 H-bond acceptors and 5 H-bond donors, had an MW of 500 Daltons, and had a five octanol/water partition coefficient. The created apigenin and taxifolin were verified in silico as specific pharmaceutical molecules and had the best affinity score according to Lipinski’s guidelines, ADMET analysis, and molecular docking.
According to Lipinski’s guidelines, the compounds displayed drug-like properties (Table ), which indicated a higher likelihood of effective oral absorption. Lipinski’s rule said that these compounds had drug-like characteristics.
Table 3. Lipinski’s rule results for apigenin and taxifolin compounds
2.3. The activity of apigenin and taxifolin compounds as anti-SARS CoV2
The discovery with an IC50 of 1.23 mg/mL less than the CC50 of 11.93 mg/mL and SI of 20.77 (Figure & Table ), taxifolin compound has moderate antiviral activity against SAR-CoV2, whereas apigenin compound has moderate antiviral activity against SAR-CoV2 because SI was 8.79 (Table ), IC50 of 2.18 mg/mL (Figure ) less than CC50 of 19.17 mg/mL, and (Figure ), as well as, olive oil crude has moderate antiviral activity against SAR-CoV2 because SI was 9.57 (Table ), IC50 of 1.78 mg/mL (Figure ) less than CC50 of 17.05 mg/mL (Figure ).
Table 4. CC50, IC50, and SI of apigenin and taxifolin compound against SARS-CoV-2
3. Discussion
One of the most discussed topic research issues now in the globe is the continuing COVID-19 pandemic, which is brought on by the severe acute respiratory syndrome coronavirus 2 (SARS-CoV-2). Olive oil is most frequently used by patients to treat high blood pressure, diabetes, and heart disease. It is also claimed to treat obesity, high cholesterol, cancer, poor memory and cognitive abilities, migraines, and many other illnesses; however, many of these other applications lack strong scientific backing. In this study, an olive oil compound was utilized as a pharmacological model against RdRP SARS-CoV-2 and led to the inhibition of RdRP proteins. This agreement with the traditional use of plants as medicines was based on the presence of phytochemicals (A. A. A-H. Elfiky, Citation2020).
The affinity score of apigenin:RdRP complex is −18.2 kcal/mol and taxifolin:RdRp complex is −14.2 kcal/mol based on interactions formed. Apigenin:RdRP complex demonstrated van der Wall interactions, conventional hydrogen bonds, Pi anion, Pi Pi stacked and Pi Alkyl type interactions, while taxifolin:RdRP complex demonstrated van der Waals interaction, conventional hydrogen bonds and Pi donor hydrogen bond (Almuhayawi et al., Citation2023; Kasende et al., Citation2017; Matondo et al., Citation2022; Ngbolua et al., Citation2022).
Our results in vitro and virtual studies have shown that olive oil’s phytochemicals are potentially active against SARS-CoV-2. This may be because olive oil contains a variety of bioactive compounds, such as oleanolic acid, oleuropein, oleocanthal, and hydroxytyrosol, which has anti-inflammatory and cardioprotective properties (Elshazly et al., Citation2023; Majumder et al., Citation2022). Flavonoids and phenolic compounds were potential candidates for disrupting the coronavirus life cycle in this study, based on complementary approaches using virtual and in-silico screening. This is similar to most antiviral drugs currently available, single targets designed against distinct viral enzymes (Mishra et al., Citation2021). As physiologically active compounds, flavonoids can impact coronaviruses at the stages of viral entry and penetration into cells, virion release from cells and viral nucleic acid replication. They can also alter the host’s cellular targets (Reynolds et al., Citation2021; Russo et al., Citation2020). These organic substances may be an essential tool in the fight against coronaviruses, such as the one that is currently spreading over the globe (Kaul et al., Citation2021). However, due to their bioavailability, apigenin and taxifolin in this investigation demonstrated modest effectiveness against a real pandemic, following (CitationWHO, W.G.R. on T. and C. No Title).
Flavonoids have potent bioactivity and a variety of pharmacological and toxicological applications, making them promising treatments for both ongoing and new public health issues. In fact (Martin & Ernst, Citation2003; Piccolella et al., Citation2019), their structures are frequently used as inspiration for synthetic medicines. The autodocking results in this study revealed that olive oil compounds as flavonoids as medicinal products act and can compete with the RdRP SARS-CoV-2 binding site and thereby induce RdRP SARS-CoV-2 inhibition, which agrees with (Akrayi & Tawfeeq, Citation2012). Secondary plant metabolites known as flavonoids have a polyphenolic structure and a variety of biological properties, such as antiviral, anti-inflammatory, and immunomodulatory actions. On the other hand, apigenin (4,5,7-trihydroxyflavone), on the other hand, celery, parsley celeriac, thyme, grapes, oregano, apples, basil, wine, beer, and chamomile tea are all rich sources of apigenin (4,5,7-trihydroxyflavone), a naturally occurring flavone molecule (M. Elnosary et al., Citation2022).
The apigenin, which is a flavonoid compound, exhibits antibacterial and also anti-inflammatory activity. In fact, it is important for a compound that represents a potential inhibitor of the SARS-CoV-2 main protease to have an anti-inflammatory property, since in the most severe cases of the disease; there is an excessive production of proinflammatory cytokines. This compound was also identified as a potential candidate for inhibiting the viral replication and for controlling inflammation during COVID-19 (Matondo et al., Citation2021).
Additionally, apigenin displayed anti-coronavirus activity (A. A. A-H. Elfiky, Citation2020). Adenovirus (ADV) and African swine fever virus (ASFV), hepatitis B virus in vitro, enterovirus-71 (EV71) viral RNA, and picornaviruses were among the viruses it also displayed a wide range of antiviral activities against another joint viral agent that causes respiratory illnesses is the rhinovirus (RV). Using BEAS-2B cell-based approaches, quercetin reduces several strains of; furthermore, quercetin pretreatment prior to RV infection significantly reduced viral endocytosis; this result is probably explained by quercetins interacting with and controlling the cellular enzyme PI-3-kinase; flavonol also significantly reduces the viral reproduction phase (S. Ganesan et al., Citation2012). The novel flavonoid derivatives 6-chloro-3-methoxy-40-oxazolinyl-flavone, 6-chloro-40-oxazolinyl, and 6-chloro-3-methoxy-40-carboxylic acid reduced the human rhinovirus (HRV-1B) activity without significantly damaging the cells (Desideri et al., Citation1995; M. E. Elnosary et al., Citation2022). These images show potential use for flavonoids in combating the novel coronavirus (n-CoV). It has been demonstrated that apigenin, along with other flavonoids like taxifolin, inhibits the proteolytic activity of SARS-CoV 3CLpro. For chronic disorders like COVID, apigenin intake may be increased by a regular diet or supplements, as antiviral actions are thought to be directly tied to reducing SARS-CoV 3CLpro activity (Elebeedy et al., Citation2021; A. A. Elfiky & Ismail, Citation2018).
In the final, the essential oil molecules were also reported to interact with the viral life cycle, such as the viral entry, replication, assembly, and release, as well as targeting virus—host through specific or noncovalent interactions such as hydrogen bonds, π/πand van der Waals interactions (Tshibangu et al., Citation2020).
4. Materials and methods
4.1. Materials
Olive oil crude collected from Olive Research Center, Jouf University, Sakaka, Saudi Arabia, in purity 100%. The apigenin and the sodium taxifoline came from the American company Sigma Aldrich.
4.2. Methods
4.2.1. Ligands collected, preparation, and optimization
The 26 fatty acids and phenolic ligands of olive oil were obtained for this investigation from the PubChem database (https://pubchem.ncbi.nlm.nih.gov/), saved as SDF format, and then the three-dimensional structures of the ligands were obtained from Open Babel (O’Boyle et al., Citation2011), saved as pdbqt format, for use in subsequent molecular docking studies.
4.2.2. Receptor preparation
RdRP SARS CoV2’s three-dimensional crystal structure (PDB ID: 6XQB) was downloaded from the Protein Data Bank (PDB) database of the Research Collaborative for Structural Bioinformatics (RCSB) (https://www.rcsb.org/structure/6XQB). The MGL-tools program was used to create the receptor protein, which was then saved in the pdbqt format, while water (solvent) molecules were left inside the active site to facilitate the establishment of a hydrogen bond between the ligand and the target.
4.2.3. Molecular docking
Autodock_vina_1_1_2_linux_x86 software was used to perform molecular docking and scoring calculations. The resolution for the crystal structure of the RdRP SARS CoV2 (PDB ID: 6XQB) was 3.4. For the docking simulation, the receptors were chosen to be rigid and the ligands to be flexible. Details of the three-dimensional crystal structure (6XQB) and lattice box of RdRP SARS CoV2 are presented in Table . On the other hand, the visualization was carried out by the BIOVIA Discovery Studio 2021 used (Norinder & Bergström, Citation2006).
Table 5. Details of the three-dimensional crystal structure and grid box of HIV-RT and ZV-RdRP
4.2.4. Predicted pharmacokinetic and toxicity properties
The science of drug kinetics studies how drugs are absorbed, distributed, metabolized, and eliminated. [A] absorption is the process by which a drug leaves the delivery site and enters the bloodstream. The transport of blood into and out of tissues is known as [D]Distribution. Drug metabolism refers to the chemical transformation of a drug to remove it; enzymes are often involved in metabolism. Elimination is the final, permanent expulsion of the original material from the body. [T]Toxicity: This filter, as its name implies, evaluates the value of a molecule and its metabolites (Pratama et al., Citation2019; Rbaa et al., Citation2021; Tong et al., Citation2021). Chris Lipinski of Pfizer created standards to follow while creating readily available compounds. The four recommendations are: (1) The compound’s molecular weight (MW) must be 500 g/mol or less. (2) There should not be more than five hydrogen bond donors HBDs in the molecule. These OH and NH groupings predominate. (3) There should not be more than 10 hydrogen bond acceptors per molecule. Essentially, they are the atoms of oxygen and nitrogen in the molecule (4). The molecule’s logP-measured lipophilicity cannot be higher than 5. These are referred to as “Lipinski’s Rules” or the “Rule of 5” (Chen et al., Citation2020; Protti et al., Citation2021). Lipinski said that the molecules should follow at least three of the four recommendations to have a decent probability of having advantageous oral bioavailability. Therefore, Lipinski’s principles help meet the letter A-absorption, have higher efficacy, or have lesser toxicity when we consider ADME. These recommendations consequently exclusively focus on improving solubility and membrane permeability to maximize oral absorption. Computational techniques can be used to minimize toxicological risks. Where the pKCSM program (https://biosig.lab.uq.edu.au/pkcsm/prediction) was used to create the Simplified Molecular Input Line Entry System (SMILES) (Pires et al., Citation2015).
4.2.5. The activity of apigenin and taxifolin compounds as anti-SARS CoV2
In DMEM media supplemented with 10% fetal bovine serum and 0.1% antibiotic/antifungal solution, SARS-CoV-2 and Vero-E6 cells were cultured. The DMEM medium, trypsin-EDTA, fetal bovine serum, and antibiotic and antifungal solution were all provided by Gibco BRL (Grand Island, NY, USA). The recently discovered cytopathic (CPE) inhibitory effect was employed with antiviral activity and cytotoxicity tests with the crystal violet method (Donalisio et al., Citation2013).
In conclusion, 96-well culture plates containing 2 × 104 Vero E6 cells each were seeded two days before infection. The cells were washed with phosphate-buffered saline after the growth media were taken out the following day. The crystal violet method, which was used to estimate the SARS CoV-2 infection rate, can be utilized to determine the CPE and the percentage of cell viability. Mammalian cells received 0.1 mL of a virus suspension prepared from the SARS-CoV-2 containing CCID50 (1.0 × 104) virus stock. This dose was selected to ensure that the proper CPEs would be produced two days following infection. A 0.01 mL of medium containing the desired chemical concentration was added to administer the drug to the cells. Each test sample’s antiviral activity was assessed against virus controls (virus-infected, untreated cells) and cell controls, starting at a concentration of 1000 mg/ml (non-infected, nondrug-treated cells). Seventy-two hours were spent incubating culture plates at 37°C and 5% CO2. By using light microscopy, the cytopathic impact’s development was tracked. A PBS wash was followed by the fixation and staining of the cell monolayers in a 0.03% crystal violet solution in 2% ethanol and 10% formalin.
Each well’s optical density was measured spectrophotometrically at 570/630 nm after washing and drying. The percentage of antiviral activity of the substances examined was determined by Pauwels et al. (Citation1988) (Pauwels et al., Citation1988) using the formula: Antiviral activity = [(optical density of the test/mean optical density of virus controls)/(optical density of the cell controls/mean optical density of virus controls)] 100%. These results led to the establishment of the 50% CPE inhibitory dose (IC50). According to Vanicha and Kanyawim (2006), we assessed the cytotoxicity before doing this test; cells were plated in 96-well culture plates at a density of 2 × 104 cells per well. The serially diluted samples were added to the culture medium the following day, and the cells were cultured for 72 hours before the culture medium was withdrawn and PBS was applied to them. The steps that were performed after the antiviral activity assay that was previously disclosed were the same. Utilizing the GraphPad PRISM program (Graph-Pad Software, San Diego, USA), the 50% inhibitory concentration (CC50) and 50% cytotoxic concentration (CC50) was calculated (IC50).
5. Conclusions
Numerous research utilizing antiviral medication therapy are being conducted to treat the potentially fatal coronavirus infection. This research will help develop new medications in the near and distant future to treat SARS-CoV-2. Based on the binding energy score, ADMET, and Lipinski’s rule, we propose that these compounds, such as apigenin and taxifolin, can be tested against SARS-CoV-2 and used to develop efficient antiviral drugs. In this study, docking analyses showed that some compounds might inhibit the SARS-CoV-2 from olive oil. Therefore, these compounds have been tested in vitro against SARS-CoV-2, and the results indicate that taxifolin and apigenin have moderate antiviral properties compared to olive oil crude, which also have moderate antiviral properties. In the future perspective, we will study the effect of taxifolin and apigenin on the mode of action and gene expression of SARS-CoV-2.
Supplemental Material
Download MS Excel (15.3 KB)Disclosure statement
No potential conflict of interest was reported by the author(s).
Supplementary material
Supplemental data for this article can be accessed online at https://doi.org/10.1080/23311932.2023.2236828
Additional information
Funding
References
- Abdelrheem, D. A., Rahman, A. A., Elsayed, K. N. M., Abd El-Mageed, H. R., Mohamed, H. S., & Ahmed, S. A. (2021). Isolation, characterization, in vitro anticancer activity, dft calculations, molecular docking, bioactivity score, drug-likeness and admet studies of eight phytoconstituents from brown alga sargassum platycarpum. Journal of Molecular Structure, 1225, 129245. https://doi.org/10.1016/j.molstruc.2020.129245
- Afonine, P. V., Klaholz, B. P., Moriarty, N. W., Poon, B. K., Sobolev, O. V., Terwilliger, T. C., Adams, P. D., & Urzhumtsev, A. (2018). New tools for the analysis and validation of Cryo-EM maps and atomic models. Acta Crystallographica Section D: Structural Biology, 74(9), 814–16. https://doi.org/10.1107/S2059798318009324
- Akrayi, H. F., & Tawfeeq, J. D. (2012). Antibacterial activity of Lepidium sativum and allium porrum extracts and juices against some gram positive and gram negative bacteria. Medical Journal of Islamic World Academy of Sciences, 20(1), 10–16. https://jag.journalagent.com/ias/pdfs/IAS_20_1_10_16.pdf
- Al-Karmalawy, A. A., & Khattab, M. (2020). Molecular modelling of mebendazole polymorphs as a potential colchicine binding site inhibitor. New Journal of Chemistry, 44(33), 13990–13996. https://doi.org/10.1039/D0NJ02844D
- Al-Mokadem, A. Z., Alnaggar, A. E.-A. M., Mancy, A. G., Sofy, A. R., Sofy, M. R., Mohamed, A. K. S. H., Abou Ghazala, M. M. A., El-Zabalawy, K. M., Salem, N. F. G., Elnosary, M. E., & Agha, M. S. (2022). Foliar application of chitosan and phosphorus alleviate the potato virus Y-Induced resistance by modulation of the reactive oxygen species, antioxidant defense system activity and gene expression in potato. Agronomy, 12(12), 3064. https://doi.org/10.3390/agronomy12123064
- Almuhayawi, M. S., Alruhaili, M. H., Gattan, H. S., Alharbi, M. T., Nagshabandi, M. K., Al Jaouni, S. K., Selim, S., & Elnosary, M. E. (2023). In silico molecular modeling of cold pressed garden cress (Lepidium sativum L.) seed oil toward the binding pocket of antimicrobial resistance Staphylococcus aureus DNA-gyrase complexes. European Review for Medical & Pharmacological Sciences, 27(4), 1238–1247. https://doi.org/10.26355/eurrev_202302_31356
- Amiot-Carlin, M. J. (2014). Olive oil and health effects: from epidemiological studies to the molecular mechanisms of phenolic fraction. OCL Oilseeds and Fats Crops and Lipids, 21(5), 1–8. https://doi.org/10.1051/ocl/2014029
- Asmi, K. S., Lakshmi, T., Balusamy, S. R., & Parameswari, R. (2017). Therapeutic aspects of taxifolin–an update. Journal of Advanced Pharmacy Education & Research| Jul-Sep, (3), 7.
- Bendini, A., Cerretani, L., Carrasco-Pancorbo, A., Gómez-Caravaca, A. M., Segura-Carretero, A., Fernández-Gutiérrez, A., & Lercker, G. (2007). Phenolic molecules in virgin olive oils: A survey of their sensory properties, health effects, antioxidant activity and analytical methods. An overview of the last decade alessandra. Molecules, 12(8), 1679–1719. https://doi.org/10.3390/12081679
- Bonoli, M., Montanucci, M., Toschi, T. G., & Lercker, G. (2003). Fast separation and determination of tyrosol, hydroxytyrosol and other phenolic compounds in extra-virgin olive oil by capillary zone electrophoresis with ultraviolet-diode array detection. Journal of Chromatography A, 1011(1–2), 163–172. https://doi.org/10.1016/S0021-9673(03)01100-2
- Bulotta, S., Celano, M., Lepore, S. M., Montalcini, T., Pujia, A., & Russo, D. (2014). Beneficial effects of the olive oil phenolic components oleuropein and hydroxytyrosol: Focus on protection against cardiovascular and metabolic diseases. Journal of Translational Medicine, 12(1), 1–9. https://doi.org/10.1186/s12967-014-0219-9
- Butler, M. S. (2004). The role of natural product chemistry in drug discovery. Journal of Natural Products, 67(12), 2141–2153. https://doi.org/10.1021/np040106y
- Caramia, G., Gori, A., Valli, E., & Cerretani, L. (2012). Virgin olive oil in preventive medicine: From legend to epigenetics. European Journal of Lipid Science and Technology, 114(4), 375–388. https://doi.org/10.1002/ejlt.201100164
- Cerretani, L., Gallina Toschi, T., & Bendini, A. (2009). Phenolic fraction of virgin olive oil: An overview on identified compounds and analytical methods for their determination. Functional Plant Science and Biotechnology, 3(Special issue), 69–80. https://hdl.handle.net/11585/82069
- Chen, X., Li, H., Tian, L., Li, Q., Luo, J., & Zhang, Y. (2020). Analysis of the physicochemical properties of acaricides based on Lipinski’s rule of five. Journal of Computational Biology, 27, 1397–1406. https://doi.org/10.1089/cmb.2019.0323
- Cicerale, S., Lucas, L., & Keast, R. (2010). Biological activities of phenolic compounds present in virgin olive oil. International Journal of Molecular Sciences, 11(2), 458–479. https://doi.org/10.3390/ijms11020458
- Dai, W., Bi, J., Li, F., Wang, S., Huang, X., Meng, X., Sun, B., Wang, D., Kong, W., Jiang, C., & Su, W. (2019). Antiviral efficacy of flavonoids against enterovirus 71 infection in vitro and in newborn mice. Viruses, 11(7), 625. https://doi.org/10.3390/v11070625
- Desideri, N., Conti, C., Sestili, I., Tomao, P., Stein, M. L., & Orsi, N. (1995). In Vitro evaluation of the anti-picornavirus activities of new synthetic flavonoids. Antiviral Chemistry and Chemotherapy, 6(5), 298–306. https://doi.org/10.1177/095632029500600503
- Donalisio, M., Nana, H. M., Ngono Ngane, R. A., Gatsing, D., Tiabou Tchinda, A., Rovito, R., Cagno, V., Cagliero, C., Boyom, F. F., Rubiolo, P., Bicchi, C., & Lembo, D. (2013). In Vitro anti-Herpes simplex virus activity of crude extract of the roots of Nauclea latifolia Smith (Rubiaceae). BMC Complementary and Alternative Medicine, 13(1), 1–8. https://doi.org/10.1186/1472-6882-13-266
- Elebeedy, D., Elkhatib, W. F., Kandeil, A., Ghanem, A., Kutkat, O., Alnajjar, R., Saleh, M. A., Abd El Maksoud, A. I., Badawy, I., & Al-Karmalawy, A. A. (2021). Anti-SARS-CoV-2 activities of tanshinone iia, carnosic acid, rosmarinic acid, salvianolic acid, baicalein, and glycyrrhetinic acid between computational and in vitro insights. RSC Advances 2021, 11(47), 29267–29286. https://doi.org/10.1039/D1RA05268C
- Elfiky, A. A. (2017). Zika virus: Novel guanosine derivatives revealed strong binding and possible inhibition of the polymerase. Future Virology, 12(12), 721–728. https://doi.org/10.2217/fvl-2017-0081
- Elfiky, A. A. (2019). Novel guanosine derivatives as anti-HCV NS5b polymerase: A QSAR and molecular docking study. Medicinal Chemistry, 15(2), 130–137. https://doi.org/10.2174/1573406414666181015152511
- Elfiky, A. A. A.-H. (2020). Anti-HCV, nucleotide inhibitors, repurposing against COVID-19. Life Sciences, 248, 117477. https://doi.org/10.1016/j.lfs.2020.117477
- Elfiky, A. A., & Ismail, A. M. (2018). Molecular docking revealed the binding of nucleotide/side inhibitors to zika viral polymerase solved structures. SAR and QSAR in Environmental Research, 29(5), 409–418. https://doi.org/10.1080/1062936X.2018.1454981
- El Gizawy, H. A., Boshra, S. A., Mostafa, A., Mahmoud, S. H., Ismail, M. I., Alsfouk, A. A., Taher, A. T., Al-Karmalawy, A. A., & Pimenta Dioica, M. (2021). Pimenta dioica (L.) merr bioactive constituents exert anti-SARS-CoV-2 and anti-inflammatory activities: Molecular docking and dynamics, in vitro, and in vivo studies. Molecules, 26(19), 5844. https://doi.org/10.3390/molecules26195844
- Elnosary, M. E., Aboelmagd, H. A., Habaka, M. A., Salem, S. R., & El-Naggar, M. E. (2022). Synthesis of bee venom loaded chitosan nanoparticles for anti-MERS-COV and multi-drug resistance bacteria. International Journal of Biological Macromolecules, 224, 871–880. https://doi.org/10.1016/j.ijbiomac.2022.10.173
- Elnosary, M., Aboelmagd, H., Sofy, M. R., Sofy, A., & Elshazly, E. (2022). Hamdy antiviral and antibacterial properties of synthesis silver nanoparticles with nigella arvensis aqueous extract. Egyptian Journal of Chemistry, 0(0), 0–0. https://doi.org/10.21608/ejchem.2022.159976.6894
- Elshazly, E. H., Nasr, A., Elnosary, M. E., Gouda, G. A., Mohamed, H., & Song, Y. (2023). Identifying the Anti-MERS-CoV and anti-hcoV-229E potential drugs from the ginkgo biloba leaves extract and its eco-friendly synthesis of silver nanoparticles. Molecules, 28(3), 28. https://doi.org/10.3390/molecules28031375
- Fidelis, Q. C., Faraone, I., Russo, D., Aragão Catunda-Jr, F. E., Vignola, L., de Carvalho, M. G., de Tommasi, N., & Milella, L. (2019). Chemical and biological insights of ouratea hexasperma (A. St.-Hil.) Baill.: A source of bioactive compounds with multifunctional properties. Natural Product Research, 33(10), 1500–1503. https://doi.org/10.1080/14786419.2017.1419227
- Ganesan, A., & Barakat, K. (2017). Applications of computer-aided approaches in the development of hepatitis c antiviral agents. Expert Opinion on Drug Discovery, 12(4), 407–425. https://doi.org/10.1080/17460441.2017.1291628
- Ganesan, S., Faris, A. N., Comstock, A. T., Wang, Q., Nanua, S., Hershenson, M. B., & Sajjan, U. S. (2012). Quercetin inhibits rhinovirus replication in vitro and in vivo. Antiviral Research, 94(3), 258–271. https://doi.org/10.1016/j.antiviral.2012.03.005
- Gorzynik-Debicka, M., Przychodzen, P., Cappello, F., Kuban-Jankowska, A., Marino Gammazza, A., Knap, N., Wozniak, M., & Gorska-Ponikowska, M. (2018). Potential health benefits of olive oil and plant polyphenols. International Journal of Molecular Sciences, 19(3), 686. https://doi.org/10.3390/ijms19030686
- Hakobyan, A., Arabyan, E., Avetisyan, A., Abroyan, L., Hakobyan, L., & Zakaryan, H. (2016). Apigenin inhibits African swine fever virus infection in vitro. Archives of Virology, 161(12), 3445–3453. https://doi.org/10.1007/s00705-016-3061-y
- Jin, Y., Yang, H., Ji, W., Wu, W., Chen, S., Zhang, W., & Duan, G. V. (2020). Epidemiology, pathogenesis, and control of COVID-19. Viruses, 12(4), 372. https://doi.org/10.3390/v12040372
- Kasende, O. E., Matondo, A., Muya, J. T., & Scheiner, S. (2017). Interactions between temozolomide and guanine and its S and Se‐substituted analogues. International Journal of Quantum Chemistry, 117(3), 157–169. https://doi.org/10.1002/qua.25294
- Kaul, R., Paul, P., Kumar, S., Büsselberg, D., Dwivedi, V. D., & Chaari, A. (2021). Promising antiviral activities of natural flavonoids against SARS-CoV-2 targets: Systematic review. International Journal of Molecular Sciences 2021, 22(20), 11069. https://doi.org/10.3390/ijms222011069
- Khattab, M., & Al‐Karmalawy, A. A. (2021). Revisiting activity of some nocodazole analogues as a potential anticancer drugs using molecular docking and DFT calculations. Frontiers in Chemistry, 9, 628398. https://doi.org/10.3389/fchem.2021.628398
- Lim, R., Barker, G., Wall, C. A., & Lappas, M. (2013). Dietary phytophenols curcumin, naringenin and apigenin reduce infection-induced inflammatory and contractile pathways in human placenta, foetal membranes and myometrium. Molecular Human Reproduction, 19(7), 451–462. https://doi.org/10.1093/molehr/gat015
- Mahmoud, I. S., Jarrar, Y. B., Alshaer, W., & Ismail, S. (2020). SARS-CoV-2 entry in host cells-multiple targets for treatment and prevention. Biochimie, 175, 93–98. https://doi.org/10.1016/j.biochi.2020.05.012
- Majumder, D., Debnath, M., Sharma, K. N., Shekhawat, S. S., Prasad, G., Maiti, D., & Ramakrishna, S. (2022). Olive oil consumption can prevent non-communicable diseases and COVID-19: A Review. Current Pharmaceutical Biotechnology, 23(2), 261–275. https://doi.org/10.2174/1389201022666210412143553
- Martin, K. W., & Ernst, E. (2003). Antiviral agents from plants and herbs: A systematic review. Antiviral Therapy, 8(2), 77–90. https://doi.org/10.1177/135965350300800201
- Matondo, A., Dendera, W., Isamura, B. K., Ngbolua, K. T. N., Mambo, H. V. S., Muzomwe, M., & Mudogo, V. (2022). In silico drug repurposing of anticancer drug 5-FU and analogues against SARS-CoV-2 main protease: Molecular docking, molecular dynamics simulation, pharmacokinetics and chemical reactivity studies. Advances and Applications in Bioinformatics and Chemistry: AABC, 15, 59–77. https://doi.org/10.2147/AABC.S366111
- Matondo, A., Kilembe, J. T., Ngoyi, E. M., Kabengele, C. N., Kasiama, G. N., Lengbiye, E. M., Mbadiko, C. M., Inkoto, C. L., Bongo, G. N., Gbolo, B. Z., Falanga, C. M., Mwanangombo, D. T., Opota, D. O., Tshibangu, D. S. T., Tshilanda, D. D., Ngbolua, K. T.-N., & Mpiana, P. T. (2021). Oleanolic acid, ursolic acid and apigenin from Ocimum basilicum as potential inhibitors of the SARS-CoV-2 main protease: A molecular docking study. International Journal of Pathogen Research, 6(2), 1–16. https://doi.org/10.9734/ijpr/2021/v6i230156
- Mishra, C. B., Pandey, P., Sharma, R. D., Malik, M. Z., Mongre, R. K., Lynn, A. M., Prasad, R., Jeon, R., & Prakash, A. (2021). Identifying the natural polyphenol catechin as a multi-targeted agent against SARS-CoV-2 for the plausible therapy of COVID-19: An integrated computational approach. Briefings in Bioinformatics, 22(2), 1346–1360. https://doi.org/10.1093/bib/bbaa378
- Ngbolua, J. K., Kilembe, J. T., Matondo, A., Ashande, C. M., Mukiza, J., Nzanzu, C. M., Ruphin, F. P., Baholy, R., Mpiana, P. T., & Mudogo, V. (2022). In silico studies on the interaction of four cytotoxic compounds with angiogenesis target protein HIF-1α and human androgen receptor and their ADMET properties. Bulletin of the National Research Centre, 46, 101. https://doi.org/10.1186/s42269-022-00793-1
- Ngwa, W., Kumar, R., Thompson, D., Lyerly, W., Moore, R., Reid, T.-E., Lowe, H., & Toyang, N. Potential of flavonoid-inspired phytomedicines against COVID-19. Molecules, 2020(11), 25, 2707. https://doi.org/10.3390/molecules25112707
- Norinder, U., & Bergström, C. A. S. (2006). Prediction of ADMET Properties. ChemMedchem: Chemistry Enabling Drug Discovery, 1(9), 920–937. https://doi.org/10.1002/cmdc.200600155
- O’Boyle, N. M., Banck, M., James, C. A., Morley, C., Vandermeersch, T., & Hutchison, G. R. (2011). Open babel: An open chemical toolbox. Journal of Cheminformatics, 3(1), 1–14. https://doi.org/10.1186/1758-2946-3-33
- Owen, R. W., Giacosa, A., Hull, W. E., Haubner, R., Würtele, G., Spiegelhalder, B., & Bartsch, H. (2000). Olive-oil consumption and health: The possible role of antioxidants. The Lancet Oncology, 1(2), 107–112. https://doi.org/10.1016/S1470-2045(00)00015-2
- Pauwels, R., Balzarini, J., Baba, M., Snoeck, R., Schols, D., Herdewijn, P., Desmyter, J., & De Clercq, E. (1988). Rapid and automated tetrazolium-based colorimetric assay for the detection of anti-HIV compounds. Journal of Virological Methods, 20(4), 309–321. https://doi.org/10.1016/0166-0934(88)90134-6
- Perez-Martinez, P., Garcia-Rios, A., Delgado-Lista, J., Perez-Jimenez, F., & Lopez-Miranda, J. (2011). Mediterranean diet rich in olive oil and obesity, metabolic syndrome and diabetes mellitus. Current Pharmaceutical Design, 17(8), 769–777. https://doi.org/10.2174/138161211795428948
- Piccolella, S., Crescente, G., Candela, L., & Pacifico, S. (2019). Nutraceutical polyphenols: New analytical challenges and opportunities. Journal of Pharmaceutical and Biomedical Analysis, 175, 112774. https://doi.org/10.1016/j.jpba.2019.07.022
- Pires, D. E., Blundell, T. L., & Ascher, D. B. (2015). pkCSM: Predicting small-molecule pharmacokinetic and toxicity properties using graph-based signatures. Journal of Medicinal Chemistry, 58(9), 4066–4072. https://doi.org/10.1021/acs.jmedchem.5b00104
- Pratama, M. R. F., Poerwono, H., & Siswodiharjo, S. (2019). ADMET properties of novel 5-O-Benzoylpinostrobin derivatives. Journal of Basic and Clinical Physiology and Pharmacology, 30(6), 30. https://doi.org/10.1515/jbcpp-2019-0251
- Protti, Í. F., Rodrigues, D. R., Fonseca, S. K., Alves, R. J., de Oliveira, R. B., & Maltarollo, V. G. (2021). Do drug‐likeness rules apply to oral prodrugs? ChemMedChem 2021, 16(9), 1446–1456. https://doi.org/10.1002/cmdc.202000805
- Qian, S., Fan, W., Qian, P., Zhang, D., Wei, Y., Chen, H., & Li, X. (2015). Apigenin restricts FMDV infection and inhibits viral IRES driven translational activity. Viruses, 7(4), 1613–1626. https://doi.org/10.3390/v7041613
- Rbaa, M., Oubihi, A., Hajji, H., Tüzün, B., Hichar, A., Ajana, E., Berdimurodov, M. A., Lakhrissi, A., Zarrouk, B. S., & Lakhrissi, B. (2021). Bioinformatics and biological evaluation of novel pyridine based on 8-hydroxyquinoline derivatives as antibacterial agents: DFT, molecular docking and ADME/T studies. Journal of Molecular Structure, 1244, 130934. https://doi.org/10.1016/j.molstruc.2021.130934
- Reynolds, D., Huesemann, M., Edmundson, S., Sims, A., Hurst, B., Cady, S., Beirne, N., Freeman, J., Berger, A., & Gao, S. (2021). Viral inhibitors derived from macroalgae, microalgae, and cyanobacteria: A review of antiviral potential throughout pathogenesis. Algal Research, 57, 102331. https://doi.org/10.1016/j.algal.2021.102331
- Russo, M., Moccia, S., Spagnuolo, C., Tedesco, I., & Russo, G. L. (2020). Roles of flavonoids against coronavirus infection. Chemico-Biological Interactions, 328, 109211. https://doi.org/10.1016/j.cbi.2020.109211
- Scoditti, E., Calabriso, N., Massaro, M., Pellegrino, M., Storelli, C., Martines, G., De Caterina, R., & Carluccio, M. A. (2012). Mediterranean diet polyphenols reduce inflammatory angiogenesis through MMP-9 and COX-2 inhibition in human vascular endothelial cells: A potentially protective mechanism in atherosclerotic vascular disease and cancer. Archives of Biochemistry and Biophysics, 527(2), 81–89. https://doi.org/10.1016/j.abb.2012.05.003
- Shibata, C., Ohno, M., Otsuka, M., Kishikawa, T., Goto, K., Muroyama, R., Kato, N., Yoshikawa, T., Takata, A., & Koike, K. (2014). The flavonoid apigenin inhibits hepatitis C virus replication by decreasing mature MicroRNA122 levels. Virology, 462, 42–48. https://doi.org/10.1016/j.virol.2014.05.024
- Soltan, M. A., Elbassiouny, N., Gamal, H., Elkaeed, E. B., Eid, R. A., Eldeen, M. A., & Al-Karmalawy, A. A. (2021). In Silico prediction of a multitope vaccine against Moraxella catarrhalis: Reverse vaccinology and immunoinformatics. Vaccines, 9(6), 669. https://doi.org/10.3390/vaccines9060669
- Song, Z., Xu, Y., Bao, L., Zhang, L., Yu, P., Qu, Y., Zhu, H., Zhao, W., Han, Y., & Qin, C. (2019). From SARS to MERS, thrusting coronaviruses into the spotlight. Viruses, 11(1), 59. https://doi.org/10.3390/v11010059
- Sunil, C., & Xu, B. (2019). An Insight into the health-promoting effects of taxifolin (dihydroquercetin). Phytochemistry, 166, 112066. https://doi.org/10.1016/j.phytochem.2019.112066
- Tabti, H., Hajji, K., En-Nahli, F., Bouamrane, S., Lakhlifi, T., Ajana, M. A., & Bouachrine, M. (2021). Effects of partial substitution of rosemary distillation residues to oat hay on digestive aspects, milk production, and metabolic statute of Tunisian local goats. Tropical Animal Health and Production, 53(5). https://doi.org/10.1007/s11250-021-02908-8
- Tapsell, L. C. (2014). Foods and food components in the Mediterranean diet: Supporting overall effects. BMC Medicine, 12(1), 1–3. https://doi.org/10.1186/1741-7015-12-100
- Tong, J., Zhang, X., Luo, D., & Bian, S. (2021). Molecular design, molecular docking and ADMET study of cyclic sulfonamide derivatives as SARS-CoV-2 inhibitors. Chinese Journal of Analytical Chemistry, 49(12), 63–73. https://doi.org/10.1016/j.cjac.2021.09.006
- Tshibangu, D. S. T., Matondo, A., Lengbiye, E. M., Inkoto, C. L., Ngoyi, E. M., Kabengele, C. N., Bongo, G. N., Gbolo, B. Z., Kilembe, J. T., Mwanangombo, D. T., Mbadiko, C. M., Mihigo, S. O., Tshilanda, D. D., Ngbolua, K.-T.-N., & Mpiana, P. T. (2020). Possible effect of aromatic plants and essential oils against COVID-19: Review of their antiviral activity. Journal of Complementary and Alternative Medical Research, 11(1), 10–22. https://doi.org/10.9734/jocamr/2020/v11i130175
- Vicidomini, C., Roviello, V., & Roviello, G. N. (2021). Molecular Basis of the therapeutical potential of clove (Syzygium aromaticum L.) and clues to its anti-COVID-19 utility. Molecules 2021, 26(7), 1880. https://doi.org/10.3390/molecules26071880
- Villa-Rodriguez, J. A., Kerimi, A., Abranko, L., Tumova, S., Ford, L., Blackburn, R. S., Rayner, C., & Williamson, G. (2018). Acute metabolic actions of the major polyphenols in chamomile: An in vitro mechanistic study on their potential to attenuate postprandial hyperglycaemia. Scientific Reports, 8(1), 1–14. https://doi.org/10.1038/s41598-018-23736-1
- Wang, S., Yao, J., Zhou, B., Yang, J., Chaudry, M. T., Wang, M., Xiao, F., Li, Y., & Yin, W. (2018). Bacteriostatic effect of quercetin as an antibiotic alternative. Journal of Food Protection, 81(1), 68–78. https://doi.org/10.4315/0362-028X.JFP-17-214
- Wani, T. A., Masoodi, F. A., Gani, A., Baba, W. N., Rahmanian, N., Akhter, R., Wani, I. A., & Ahmad, M. (2018). Olive oil and its principal bioactive compound: Hydroxytyrosol–A review of the recent literature. Trends in Food Science & Technology, 77, 77–90. https://doi.org/10.1016/j.tifs.2018.05.001
- WHO, W.G.R. on T. and C. No Title. Retrieved from https://apps.who.int/iris/handle/10665/312342.
- Wu, C.-C., Fang, C.-Y., Cheng, Y.-J., Hsu, H.-Y., Chou, S.-P., Huang, S.-Y., Tsai, C.-H., & Chen, J.-Y. (2017). Inhibition of Epstein-Barr virus reactivation by the flavonoid apigenin. Journal of Biomedical Science, 24(1), 1–13. https://doi.org/10.1186/s12929-016-0313-9
- ZeinEldin, R. A., Ahmed, M. M., Hassanein, W. S., Elshafey, N., Sofy, A. R., Hamedo, H. A., & Elnosary, M. E. (2023). Diversity and distribution characteristics of viruses from soda lakes. Genes, 14(2), 14. https://doi.org/10.3390/genes14020323
- Zhang, W., Qiao, H., Lv, Y., Wang, J., Chen, X., Hou, Y., Tan, R., Li, E., & Qiu, J. (2014). Apigenin inhibits enterovirus-71 infection by disrupting viral RNA association with trans-acting factors. PloS One, 9(10), e110429. https://doi.org/10.1371/journal.pone.0110429
- Zumla, A., Chan, J. F. W., Azhar, E. I., Hui, D. S. C., & Yuen, K.-Y. (2016). Coronaviruses—drug discovery and therapeutic options. Nature Reviews Drug Discovery, 15(5), 327–347. https://doi.org/10.1038/nrd.2015.37