Abstract
Objective. Dipeptidyl peptidase (DPP)-4 inhibitors inactivate glucagon-like peptide (GLP)-1, GLP-2, and other hormones by degradation. Administration of a DPP-4 inhibitor or GLP-2 analog stimulates intestinal growth and facilitates the restoration of mucosal damage caused by drug-induced colitis in mice. We studied the effect of GLP-2 deletion on morphological changes on gut and colitis recovery in mice deficient in proglucagon-derived peptides (PGDPs). Material and methods. Mice deficient in PGDPs were used. Eight-week-old male PGDPs−/− and PGDPs+/+ mice were sacrificed to examine the small intestine and colon morphology. To elucidate the effect of PGDP deletion on colitis, 12-week-old PGDPs−/− and PGDPs+/+ male mice were exposed to 2.5% dextran sulfate sodium (DSS) for 6 days. Colitis severity was assessed daily by disease activity index and body weight loss. Histological examinations were performed on days 7 and 21. Results. There were no differences in the height, width, and density of villous and the depth of crypt of small intestine, and the weight, length, and the crypt depth of colon between PGDPs−/− and PGDPs+/+. In DSS colitis, the decline and amelioration of disease activity index and body weight were not different between the PGDP−/− and PGDP+/+ mice. Histologically, the PGDP−/− mice showed significantly less recovery than showed by the PGDPs+/+ mice on day 21 (p < 0.05). Conclusion. Mice with PGDP deletion did not show morphological changes of gut. On colitis, PGDP deletion only caused less histological amelioration of colitis in the later phase of recovery.
Public Interest Statement
Glucagon-like peptide (GLP)-2 is an intestinal hormone which stimulates the growth of small intestine and decreases the intestinal injury. Therefore the role of GLP-2 is important for the treatment of the increasing inflammatory bowel diseases such as ulcerative colitis and Crohn’s disease. This study using the mice deletion with proglucagon derived peptides including GLP-2 is a first study aimed to explore two questions, what happens in small intestine if there were no GLP-2, and how colon recovers from drug induced colitis without GLP-2.
Competing Interests
The authors declare no competing interest.
1. Introduction
The proglucagon gene is expressed in both pancreatic A and intestinal L cells (Eissele et al., Citation1994; Mojsov et al., Citation1986). The prohormone convertases process proglucagon into different proglucagon-derived peptides (PGDPs) in each tissue in response to nutrient ingestion (Dhanvantari, Seidah, & Brubaker, Citation1996; Mojsov et al., Citation1986; Xiao, Boushey, Drucker, & Brubaker, Citation1999). The most notable pancreatic PGDP is glucagon, whereas L cells produce several structurally related peptides, including glucagon-like peptide (GLP)-1 and GLP-2, as well as glicentin and oxyntomodulin, which contain glucagon in their sequences (Brubaker, Izzo, Hill, & Drucker, Citation1997). It is well established that GLP-1 functions as a glucose-dependent insulinotrophic factor and as an enterogastrone (Mojsov, Weir, & Habener, Citation1987; Wettergren et al., Citation1993).
The physiological actions of other intestinal PGDPs have been identified, in particular for GLP-2, which acts as a potent stimulator of growth in the small intestine of mice (Drucker, Erlich, Asa, & Brubaker, Citation1996). Rats treated by GLP-2 showed significantly increased small intestinal length, height of the villous and the depth of crypts, however GLP-2 had no effect on the colon as measured by weight, length, and crypt depth (Kitchen et al., Citation2000). Dipeptidyl peptidase-4 (DPP-4) was initially identified as a therapeutic target for type 2 diabetes because it degraded an incretin, GLP-1. Inhibition of DPP-4 activity is, thus, capable of prolonging the insulinotrophic effects of GLP-1 (Ahrén, Citation2007). DPP-4 is a complex enzyme that exists as a membrane-anchored cell surface protein and cleaves N-terminal dipeptides from regulatory factors containing proline of alanine in the penultimate position (Drucker, Citation2007). A variety of growth factors, hormones, neuropeptides, and chemokines are known to be substrates of DPP-4. One substrate is GLP-2, and cleavage by DPP-4 causes GLP-2 to rapidly lose its bioactivity under physiological conditions (Yazbeck, Howarth, Geier, Demuth, & Abbott, Citation2008). DPP-4 inhibitor increases the intestinal weight, length, and morphometric data such as villous height and crypt depth of small intestine (Hartmann et al., Citation2000). Thus, inhibition of DPP-4 has been considered to have some curative efficacy against intestinal bowel diseases via enhancement of endogenous GLP-2 activity. The beneficial effect of DPP-4 inhibition on intestinal inflammation has been elucidated. Non-selective DPP-4 inhibitors (Yazbeck, Howarth, & Abbott, Citation2009), which also bind to two other members of the DPP family, DPP-8 and DPP-9, ameliorate dextran sulfate sodium (DSS)-induced colitis in mice (Bank et al., Citation2006; Yazbeck et al., Citation2008, Citation2010).
We also have reported that the selective DPP-4 inhibitor, anagliptin, facilitated the restoration of mucosal damage, which accelerated healing in an experimental colitis mouse model (Mimura et al., Citation2013).
These healing effects of DPP-4 inhibitors appear to depend on PGDPs. However, the intestinal influences of PGDPs deficiency under normal or stressed situations are unclear. To better understand the effect of absence of PGDPs, we examined the small intestine morphology and recovery from colitis in PGDPs knockout mice.
2. Methods
2.1. Animal studies
All mice were housed under a standard 12-h light/dark cycle with controlled humidity (60–80%) and temperature (22 ± 1°C). All animal care and experimental procedures were performed in strict accordance with the guidelines of the Institute for Laboratory Animal Research and the ethics committee of the Nagoya University School of Medicine.
We used transgenic mice to create proglucagon knockout mice (Hayashi et al., Citation2009). Proglucagon-liberating glucagon (Gcg), GLP-1, and GLP-2 were disrupted by insertion. The absence of Gcg and GLP-1 was confirmed in a previous study (Watanabe et al., Citation2012); however, the absence of GLP-2 was not verified. Therefore, confirmation of the absence of GLP-2 was required, so we determined the expression of mRNA of GLP-1 and GLP-2 by reverse transcription-polymerase chain reaction (Super Script III Reverse Transcriptase kit; Invitrogen, CA, USA) and the deletion of GLP-1 and GLP-2 mRNA in PGDPs−/− mice ().
2.2. Primers used for GLP-1 and GLP-2
The following primers were used: (mGLP-1 downstream; 5′-catgctgaagggacctttacc-3′, upstream; 5′-tcctcggcctttcaccag-3′) (mGLP-2 downstream; 5′-cacgctgatggctccttc-3′, upstream; 5′-gtcagtgatcttggtttgaatcagc-3′).
Small intestine and colon morphology; the villous height, villous width, villous density, and crypt depth of small intestine and the length, weight, and crypt depth of colon.
By performing tail tissue DNA genotyping, we classified 5–6 week-old mice into three groups: PGDPs+/+, PGDPs+/− and PGDPs−/− by using a Thermo Scientific Phire Animal Tissue Direct PCR kit (Finnzymes, Espoo, Finland). Male PGDPs+/+ and PGDPs−/− mice (five each) were sacrificed at 8 weeks of age, and their small intestines were examined microscopically using hematoxylin and eosin (H&E) staining. The villous height and crypt depth of the duodenum and upper, middle, and distal parts of the small intestine were examined. The villous width and villous density was defined as the average of four sections. The villous density was decided as the number of villous for the 1,000 μm segment of small intestine. The crypt depth of colon were measured on distal colon.
2.3. Induction of DSS colitis
Eight-to-12-week-old PGDPs+/+ and PGDPs−/− mice were individually housed with ad libitum access to drinking water containing 2.5% DSS for 6 days. DSS-containing water was replaced with filtered water without DSS on day 6, which the mice continued to drink for a maximum of 6 days. Severity of colitis was assessed daily by body weight (BW) loss and disease activity index (DAI).
2.4. Disease activity index
From day 0 to 22, the severity of colitis was assessed daily by the percentage of BW change and DAI. The percentage of BW change was calculated based on the BW of each mouse on Day 0. Calculation of DAI was performed according to a reported method (Cooper, Murthy, Shah, & Sedergran, Citation1993) by summarizing the score for BW loss (0 points, < 5% weight loss; 1 point, 5–10% weight loss; 2 points, 10–15% weight loss; 3 points, 15–20% weight loss; and 4 points, > 20% weight loss), stool consistency (0 points, formed pellets; 2 points, pasty/semiformed stool; and 4 points, liquid stool), and rectal bleeding (0 points, no rectal bleeding; 2 points, hemoccult positive; and 4 points, visible gross bleeding). Occult blood was detected daily by using the guaiac paper test (Shionogi, Osaka, Japan). Observation for water intake in each mouse was monitored by measuring left over water in bottles. Food intake was also monitored.
2.5. Histological examination
Histological examination was performed on day 7 and 22. The small intestine and colon were dissected, and the length and weight were measured as an indirect marker of inflammation. After measurement, a 1-cm segment from the distal small intestine and the distal colon were sectioned (Lakticova, Hutton-Thomas, Meyer, Gurkan, & Rice, Citation2006), fixed overnight in 4% paraformaldehyde, embedded in paraffin, cut into tissue slices, and stained with H&E (Mimura et al., Citation2013). Olympus AX80 microscope (Tokyo, Japan) was used for analysis.
Histological evaluation was performed by using a previously described histological scoring method (Bauer et al., Citation2010), which consisted of the following histological criteria: for cell infiltration, a focal increase in the number of inflammatory cells in the lamina propria was scored as 1, the confluence of inflammatory cells extending into the submucosa was scored as 2, and transmural extension of the infiltrate was scored as 3; for tissue damage, discrete lymphoepithelial lesions were scored as 1, mucosal erosion was scored as 2, and extensive mucosal damage and/or extension through deeper structures of the bowel wall was scored as 3. The two equally weighted subscores (cell infiltration and tissue damage) were then added to give a histological colitis severity score ranging from 0 to 6. All slides were scored in a blinded manner.
2.6. Statistical analysis
Statistical analysis was performed by using IBM SPSS Statistics Version 23 (IBM Inc., Armonk, NY, USA). Results are presented as the mean ± SD. Comparisons of DAI and histological scores were performed by using the Mann–Whitney U-test. Analysis of weight and length was performed by using Student’s t-test. For all analyses, p < 0.05 was regarded as indicating statistical significance.
3. Results
Small intestine and colon morphology; the villous height, villous width, villous density, and crypt depth of small intestine and the length, weight, and crypt depth of colon.
In the duodenum and three parts of the small intestine, deformities of the villous were not observed in the PGDPs−/− mice. The length of the small intestine was 334 ± 11 mm (mean ± SD) in the PGDPs+/+ mice and 367 ± 39 mm in the PGDPs−/− mice. The weight of the small intestine was 1,617 ± 258 mg in the PGDPs+/+ mice and 1,931 ± 387 mg in the PGDPs−/− mice. The differences were not significant.
Colon length were 7.8 ± 1.2 cm in PGDPs+/+ and 8.0 ± 0.6 cm in PGDPs−/−. Crypt depth of distal colon were 209 ± 19 μm in PGDPs+/+ and 201 ± 26 μm in PGDPs−/−. The differences were not significant.
In the duodenum and three parts of the small intestine, the average villus heights in the PGDPs−/− mice were lower than those in the PGDPs+/+ mice, but the differences were not significant ((A)). The average width of villous was 181 ± 40 μm in the PGDPs+/+ mice and 171 ± 32 μm in the PGDPs−/− mice. The average density of villous was 4.7 ± 0.7 villous for 1,000 μm section of small intestine in the PGDPs+/+ mice and 4.8 ± 0.4 villous for 1,000 μm section of small intestine in the PGDPs−/− mice. The differences in the widths and densities were not significant between the two groups.
Figure 2. (A) Villous height of the duodenum and three parts of the small intestine. No significant differences in villous height in each section are observed between the PGDPs−/− and PGDPS+/+ mice. A decrease in villous height is observed in both groups; the villous height of the small intestine is 34% of that in the duodenum in the PGDPs−/− mice and 40% in the PGDPS+/+ mice. (B) Crypt depth of the duodenum and three parts of the small intestine. No significant differences in crypt depth in each section are observed between the PGDPs−/− and PGDPS+/+ mice. Crypt depth shows no decline from the oral to anal side.
Each bar represents the mean height or depth and standard deviation.
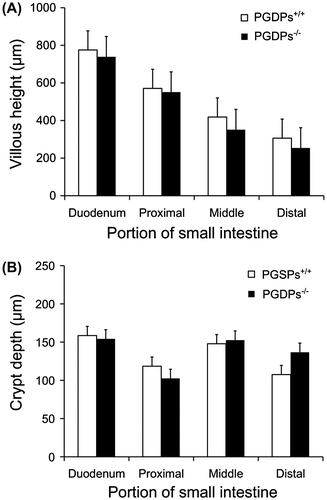
From the oral side to the anal side of the small intestine, decreases in the villus height were observed in both groups ((A)). The villous height of the distal small intestine was almost 40% of that in the duodenum in both groups. Crypt depth was not different by location and genotype ((B)).
3.1. Effect on colitis
3.1.1. Clinical data; changes in clinical scores and BW
No mice died following administration of 2.5% of DSS in both groups. Consumption of DSS water and food were not different between PGDPs+/+ and PGDPs−/− groups.
Administrations of DSS added to water were stopped on day 6. Increases in the DAI score continued until day 6 (6.9 ± 2.1 for PGDPs+/+ mice, 7.7 ± 3.4 for PGDPs−/− mice) Small intestine morphology and decreased to normal levels until day 13 ((A)). The differences between the groups were not statistically significant. Decreases in BW were observed until day 9 (82.4% ± 5.1% for PGDPs+/+ mice, 82.9% ± 4.6% for PGDPs−/− mice), and consistent recovery was observed until day 21 (97.5% ± 5.6% for PGDPs+/+ mice, 96.6% ± 4.6% for PGDPs−/− mice) ((B)). In the recovery phase, the increase in the average percent BW of the PGDPs−/− mice was higher from day 15 to 19, but the difference was not significant statistically ((B)).
Figure 3. (A) Time-dependent changes in disease activity index. The increases after the administration of dextran sodium for 5 days in the disease activity index until day 6 and the decline by day 12 are shown. Each point represents the mean ± SD of 7 PGDPs−/− and 7 PGDPS+/+ mice. (B) Time-dependent changes in BW. The decline in BW until day 9 and the recovery to 95% by day 20 are shown. Each point represents the mean ± SD of 7 PGDPs−/− and 7 PGDPS+/+ mice.
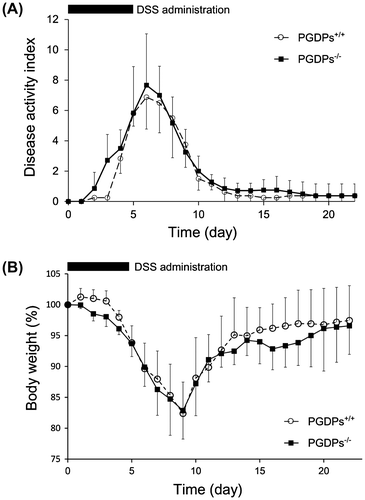
3.1.2. Histological examination
Colon length were 6.3 ± 1.0 cm in PGDPs+/+, 5.6 ± 0.8 cm in PGDPs−/− on day 7, and 7.6 ± 0.6 cm in PGDPs+/+, 6.9 ± 0.8 cm in PGDPs−/− on day 21. Colon weight were 654 ± 72 mg in PGDPs+/+, 657 ± 109 mg in PGDPs−/− on day 7, and 653 ± 103 mg in PGDPs+/+, 733 ± 99 mg in PGDPs−/− on day 21. There were no significant differences. In the distal small intestine, slight mucosal damages were found on day 7. Differences in the histological scores between PGDPs−/− and PGDPs+/+ mice were not observed on days 7 and 21 in the distal small intestine. More severe histological damage was observed in the distal colon in both groups, but the differences were not significant. However, the PGDPs−/− mice showed significantly less recovery than did the PGDPs+/+ mice on day 21 (p < 0.05) ((B)).
Figure 4. (A) Histological score of distal small intestine on colitis no significant differences were observed in histological score of distal small intestine between the PGDPs−/− and PGDPS+/+ mice. (B) Histological score of distal colon on colitis was not different on day 7 between the PGDPs−/− and PGDPS+/+ mice. On day 21, however, histological score of distal colon of PGDPs−/− was significantly higher than that of PGDPs−/−.
*p < 0.05.
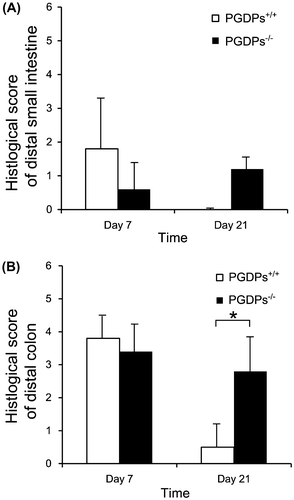
4. Discussion
4.1. Morphology
Vasoactive intestinal peptide (VIP) knockout mice have marked deformities of the small intestine, which may be related to the fact that VIP is a downstream mediator of GLP-2 (Yusta, Holland, Waschek, & Drucker, Citation2012). The administration of GLP-2 increases the villous height of the small intestine (Tsai, Hill, Asa, Brubaker, & Drucker, Citation1997).
However, PGDPs−/− transgenic mice with PGDP deletions, including glucagon, GLP-1, and GLP-2, did not show differences in the villous form, length, width, density, and crypt depth. These results indicated that PGDP deletion did not significantly affect the small intestine morphology.
4.2. Colitis
The damage caused by DSS colitis occurs primarily in the colon. Therefore, a DSS colitis model was used in the present study to elucidate the role of PGDPs on colitis to compare with previous studies that showed an intensifying effect of GLP-2 on drug-induced colitis (Sigalet et al., Citation2007; Xiao et al., Citation1999). To investigate other aspects, such as nutrient absorption of the small intestine and small intestinal injury in PGDP deletion, other experimental models would be required.
In DSS colitis, there were no significant difference in the DAI scores and BW in the active and recovery phases. From previous study results showing an amelioration effects of a GLP-2 analog and DPP-4 inhibitor administration (Drucker, Yusta, Boushey, DeForest, & Brubaker, Citation1999; Yazbeck et al., Citation2008, Citation2009, Citation2010), PGDP deletion had been thought to result in worse colitis activity and poor recovery, but this was not observed in our study. Some other chemical mediator, such as insulin-like growth factor may offset the adverse effects of PGDP deletion. Further research concerning signaling should be pursued.
In conclusion, this is the first reported study to have investigated the effect of PGDP deletion on the small intestine morphology and recovery after colitis. PGDPs were not found to adversely affect villous height, villous width, villous density, and crypt depth. However, PGDP deletion caused significantly less amelioration of colitis in the later phase of recovery.
Acknowledgements
We thank Dr Nobuaki Ozaki (Japanese Red Cross Nagoya Daiichi Hospital) and Dr Yoshitaka Hayashi (Department of Genetics, Research Institute of Environmental Medicine, Nagoya University) for technical supports and supplying mice deficient in PGDPs. We are also indebted to Dr Kazuhiro Ishiguro (Department of Gastroenterology and Hepatology, Nagoya University Graduate School of Medicine) for technical directions.
Additional information
Funding
Notes on contributors
Masanobu Matsushita
Masanobu Matsushita is a member of the Department of Gastroenterology and Hepatology of Nagoya University Graduate School of Medicine and Nagoya University Hospital, which are specialized in the basic, applied, and clinical research field. One of the basic and applied research themes is exploring the mechanism and the treatment of colitis using animal models and clinical samples. This study was planned following our previous study about the effect of DPP-4 inhibitor on drug induced colitis in mice.
References
- Ahrén, B. (2007). DPP-4 inhibitors. Best Practice & Research Clinical Endocrinology & Metabolism, 21, 517–533.10.1016/j.beem.2007.07.005
- Bank, U., Heimburg, A., Helmuth, M., Stefin, S., Lendeckel, U., Reinhold, D., ... Ansorge, S. (2006). Triggering endogenous immunosuppressive mechanisms by combined targeting of Dipeptidyl peptidase IV (DPIV/CD26) and Aminopeptidase N (APN/ CD13)—A novel approach for the treatment of inflammatory bowel disease. International Immunopharmacology, 6, 1925–1934.10.1016/j.intimp.2006.09.014
- Bauer, C., Duewell, P., Mayer, C., Lehr, H. A., Fitzgerald, K. A., Dauer, M., ... Schnurr, M. (2010). Colitis induced in mice with dextran sulfate sodium (DSS) is mediated by the NLRP3 inflammasome. Gut, 59, 1192–1199.10.1136/gut.2009.197822
- Brubaker, P. L., Izzo, A., Hill, M., & Drucker, D. J. (1997). Intestinal function in mice with small bowel growth induced by glucagon-like peptide-2. American Journal of Physiology, 272, E1050–8.
- Cooper, H. S., Murthy, S. N., Shah, R. S., & Sedergran, D. J. (1993). Clinicopathologic study of dextran sulfate sodium experimental murine colitis. Laboratory Investigation, 69, 238–249.
- Dhanvantari, S., Seidah, N. G., & Brubaker, P. L. (1996). Role of prohormone convertases in the tissue-specific processing of proglucagon. Molecular Endocrinology, 10, 342–355.
- Drucker, D. J. (2007). Dipeptidyl peptidase-4 inhibition and the treatment of type 2 diabetes: Preclinical biology and mechanisms of action. Diabetes Care, 30, 1335–1343.10.2337/dc07-0228
- Drucker, D. J., Erlich, P., Asa, S. L., & Brubaker, P. L. (1996). Induction of intestinal epithelial proliferation by glucagon-like peptide 2. Proceedings of the National Academy of Sciences, 93, 7911–7916.10.1073/pnas.93.15.7911
- Drucker, D. J., Yusta, B., Boushey, R. P., DeForest, L., & Brubaker, P. L. (1999). Human [Gly2]GLP-2 reduces the severity of colonic injury in a murine model of experimental colitis. American Journal of Physiology, 276, G79–91.
- Eissele, R., Goke, R., Weichardt, U., Fehmann, H. C., Arnold, R., & Goke, B. (1994). Glucagon-like peptide 1 immunoreactivity in gastroentero-pancreatic endocrine tumors: a light- and electron-microscopic study. Cell & Tissue Research, 276, 571–580.10.1007/BF00343955
- Hartmann, B., Thulesen, J., Kissow, H., Thulesen, S., Orskov, C., Ropke, C., ... Holst, J. J. (2000). Dipeptidyl peptidase IV inhibition enhances the intestinotrophic effect of glucagon-like peptide-2 in rats and mice. Endocrinology, 141, 4013–4020.
- Hayashi, Y., Yamamoto, M., Mizoguchi, H., Watanabe, C., Ito, R., Yamamoto, S., ... Murata, Y. (2009). Mice deficient for glucagon gene-derived peptides display normoglycemia and hyperplasia of islet α-cells but not of intestinal L-cells. Molecular Endocrinology, 23, 1990–1999.10.1210/me.2009-0296
- Kitchen, P. A., Fitzgerald, A. J., Goodlad, R. A., Barley, N. F., Ghatei, M. A., Legon, S., … Forbes, A. (2000). Glucagon-like peptide-2 increases sucrase-isomaltase but not caudal-related homeobox protein-2 gene expression. American Journal of physiology. Gastrointestinal Liver Physiology, 278, G425–8.
- Lakticova, V., Hutton-Thomas, R., Meyer, M., Gurkan, E., & Rice, L. B. (2006). Antibiotic-induced enterococcal expansion in the mouse intestine occurs throughout the small bowel and correlates poorly with suppression of competing flora. Antimicrobial Agents and Chemotherapy, 50, 3117–3123.10.1128/AAC.00125-06
- Mimura, S., Ando, T., Ishiguro, K., Maeda, O., Watanabe, O., Ujihara, M., ... Goto, H. (2013). Dipeptidyl peptidase-4 inhibitor anagliptin facilitates restoration of dextran sulfate sodium-induced colitis. Scandinavian Journal of Gastroenterology, 48, 1152–1159.10.3109/00365521.2013.832366
- Mojsov, S., Heinrich, G., Wilson, I. B., Ravazzola, M., Orci, L., & Habener, J. F. (1986). Preproglucagon gene expression in pancreas and intestine diversifies at the level of post-translational processing. Journal of Biological Chemistry, 261, 11880–11889.
- Mojsov, S., Weir, G. C., & Habener, J. F. (1987). Insulinotropin: glucagon-like peptide I (7-37) co-encoded in the glucagon gene is a potent stimulator of insulin release in the perfused rat pancreas. Journal of Clinical Investigation, 79, 616–619.10.1172/JCI112855
- Sigalet, D. L., Wallace, L. E., Holst, J. J., Martin, G. R., Kaji, T., Tanaka, H., & Sharkey, K. A. (2007). Enteric neural pathways mediate the anti-inflammatory actions of glucagon-like peptide 2. AJP: Gastrointestinal and Liver Physiology, 293, G211–G221.10.1152/ajpgi.00530.2006
- Tsai, C. H., Hill, M., Asa, S. L., Brubaker, P. L., & Drucker, D. J. (1997). Intestinal growth-promoting properties of glucagon-like peptide-2 in mice. American Journal of Physiology, 273, E77–84.
- Watanabe, C., Seino, Y., Miyahira, H., Yamamoto, M., Fukami, A., Ozaki, N., ... Hayashi, Y. (2012). Remodeling of hepatic metabolism and hyperaminoacidemia in mice deficient in proglucagon-derived peptides. Diabetes, 61, 74–84.10.2337/db11-0739
- Wettergren, A., Schjoldager, B., Mortensen, P. E., Myhre, J., Christiansen, J., & Holst, J. J. (1993). Truncated GLP-1 (proglucagon 78-107-amide) inhibits gastric and pancreatic functions in man. Digestive Diseases and Sciences, 38, 665–673.10.1007/BF01316798
- Xiao, Q., Boushey, R. P., Drucker, D. J., & Brubaker, P. L. (1999). Secretion of the intestinotropic hormone glucagon-like peptide 2 is differentially regulated by nutrients in humans. Gastroenterology, 117, 99–105.10.1016/S0016-5085(99)70555-X
- Yazbeck, R., Howarth, G. S., Geier, M. S., Demuth, H. U., & Abbott, C. A. (2008). Inhibiting dipeptidyl peptidase activity partially ameliorates colitis in mice. Frontiers in Bioscience, 13, 6850–6858.10.2741/3193
- Yazbeck, R., Howarth, G. S., & Abbott, C. A. (2009). Dipeptidyl peptidase inhibitors, an emerging drug class for inflammatory disease? Trends in Pharmacological Sciences, 30, 600–607.10.1016/j.tips.2009.08.003
- Yazbeck, R., Sulda, M. L., Howarth, G. S., Bleich, A., Raber, K., von Hörsten, S., ... Abbott, C. A. (2010). Dipeptidyl peptidase expression during experimental colitis in mice. Inflammatory Bowel Diseases, 16, 1340–1351.10.1002/ibd.21241
- Yusta, B., Holland, D., Waschek, J. A., & Drucker, D. J. (2012). Intestinotrophic glucagon-like peptide-2 (GLP-2) activates intestinal gene expression and growth factor-dependent pathways independent of the vasoactive intestinal peptide gene in mice. Endocrinology, 153, 2623–2632.10.1210/en.2012-1069