Abstract
Monitoring skin sensitivity is studied to clarify its relationship on balance. Measuring skin sensitivity is performed when subjects are sitting or lying, whereas balance tests are measured during standing. However, afferent signal processing and subsequent efferent responses can be altered by different body positions. Therefore, this study investigated whether vibration perception thresholds (VPTs) are influenced by body positions. Sixty-six healthy subjects (41♀; 25♂) participated in this study. Five measurements of VPTs were performed at each of the three analyzed anatomical locations (heel, first metatarsal head, hallux) of the right plantar foot under two randomized conditions: during sitting and standing. The contact force applied to the probe was measured and controlled within the five trials. Contact forces between the probe and the foot were higher during standing. However, no differences in VPTs were found between conditions. This indicates that VPTs are not different during standing compared to sitting, contrary to our expectations. We conclude that higher forces did not induce increased receptor activity. Since no differences were found between thresholds, future clinical studies can implement plantar VPT tests during sitting in association with balance tests during standing.
Public Interest Statement
The human skin of the foot soles contains various receptors which provide certain information like the perception of vibration. It is known that the sensitive information those receptors provide is an important contributor for human balance. Balance tests are performed while subjects are standing, but sensitivity tests are usually performed when sitting. Literature indicates that skin receptors answer differently depending on the pressure applied against the skin. Pressures against the foot soles are likely to be different when standing compared to sitting. Therefore, this study aimed in analyzing differences in perceiving vibration stimuli (vibration perception threshold, VPT) at the foot sole in sitting compared to standing. VPTs did not show differences comparing standing to sitting, although pressures were higher when standing. This is an important clinical finding since sensitivity tests conducted while sitting can be associated with balance tests in standing conditions.
Competing Interests
The authors declare no competing interest.
1. Introduction
It is known that afferent signal processing can be altered by different postures, which also affects efferent responses. For example, previous studies showed that the amplitude of the muscle reflex (H reflex) during standing was lower compared to other conditions (seated, lying, or prone) (Hayashi, Tako, Tokuda, & Yanagisawa, Citation1992; Koceja, Trimble, & Earles, Citation1993; Mynark & Koceja, Citation1997). Studies have reported the particular importance of plantar somatosensory information for balance control. For example, reduced somatosensation is associated with poorer balance abilities (Kavounoudias, Roll, & Roll, Citation1998; Perry, McIlroy, & Maki, Citation2000). In particular, various diseases, like diabetes mellitus, lead to reduced plantar sensitivity and deteriorated balance abilities (Leonard, Farooqi, & Myers, Citation2004). This emphasizes the importance of balance and sensory tests in clinical settings (Berg & Norman, Citation1996). However, sensory tests are usually performed when subjects are sitting or lying, whereas balance tests are measured during standing. In sensory vibration tests, the forces between the oscillating probe and the anatomical location are apparently greater during standing than during sitting conditions. In this regard, previous studies demonstrated that higher contact forces (by additionally applied masses) reduce vibration perception thresholds (VPTs) during sitting (Cassella, Ashford, & Kavanagh-Sharp, Citation2000; Hagander, Midani, Kuskowski, & Parry, Citation2000; Lowenthal & Derek, Citation1987). In contrast, another study did not find effects of increasing contact forces on VPTs when measuring dorsal foot areas during sitting (Hagander et al., Citation2000). However, these results may not directly be applied to standing conditions. First, contact forces might be higher when standing compared to sitting. Second, other than plantar aspects were investigated in the above mentioned studies. Furthermore, when standing, additional afferent inputs are required. Since those inputs are projected in the same afferent pathway, an alteration of the priority of plantar inputs is supposable, which may result in different VPTs. A recently published study (Mildren, Strzalkowski, & Bent, Citation2016) compared plantar VPTs during sitting and standing and found significant differences only at the metatarsal areas at 250 Hz. Note that they did not measure contact forces, and stimuli were applied by an array of many probes at the plantar metatarsal and heel areas. However, studies and clinical tests relating plantar sensitivity to balance usually investigate sensitivity thresholds using one single contact point and not an array.
Taking into account the above mentioned considerations, the purpose of this study was to compare plantar VPTs measured with subjects in a sitting and standing position. It was hypothesized that (a) contact forces against the probe are greater while standing compared to sitting and (b) consequently, VPTs measured during standing are lower than during sitting. These findings would certainly include observations of fundamental importance for future research as well as clinical diagnosis, in which perception of vibration is associated with body balance.
2. Materials and methods
2.1. Subjects
Sixty-six healthy subjects participated in this study (♂ 178.3 ± 4.7 cm; 75.1 ± 7.1 kg; 24.2 ± 4.1 years; ♀ 170.4 ± 5.9 cm; 62.3 ± 7.2 kg; 22.9 ± 3.2 years). The subjects had no history of lower leg injury or lower-extremity pain six months prior to testing and no peripheral neuropathy or other disorders that could affect sensitivity. Before the study began, the subjects were informed about the purpose of this study, gave informed written consent and were free to withdraw from it at any time. All procedures were conducted according the recommendations of the Declaration of Helsinki and were approved by the faculty’s ethics committee.
2.2. Equipment
A modified Tira Vib vibration exciter (model TV51075, Schalkau, Germany) powered by a Voltcraft oscillator (model FG 506, Hirschau, Germany) was used to measure vibration thresholds. The vibration from the exciter to the foot location was conducted by a metal probe (rounded, 7.8 mm diameter) protruding through a hole in a wooden box, which was internally covered by an acoustic insulation material. The tip of the probe was adjusted 2 mm higher than the level of the box (Nurse & Nigg, Citation1999). The measurements were performed at 200 Hz, since the optimal response of the vibration receptors (Vater–Pacini corpuscles) is reported to be at frequencies between 200 and 250 Hz (Verrillo, Citation1985). The vibration exciter was duly calibrated resulting in a constant with which the data was converted from V to μm. Room temperature was measured and controlled at 23 ± 2°C (EN ISO/IEC17025) using a thermometer (C28 Hand Held Digital Type K, Comark, UK) and the plantar foot temperature was measured using an infrared-thermometer (Mini Flash, TFA, Germany).
2.3. Testing procedure
Prior to measuring, the participants were allowed a period of ten min barefoot, during which they could adapt to the room temperature. The temperature of the foot (heel) and room were measured before each test.
Vibration thresholds were measured in two conditions: sitting and standing. While seated, the subjects should keep their feet rested on the box, knees and hips at 90° angles, not exert any pressure against the probe, and keep their upper limbs hanging down. During standing, the subjects should distribute the body weight evenly on both feet, stand with feet shoulder-width apart and upper limbs hanging down. Subjects were barefoot throughout the measurements and were instructed to wear their individual sports clothes (shorts and t-shirt) in order to feel most comfortable. Additionally, subjects used hearing protectors to avoid distracting noise. Five measurements were performed at each of the three analyzed anatomical locations of the right plantar foot: heel, first metatarsal head (Met I), and hallux. The vibration amplitude was gradually increased (at different speeds) from zero until vibratory stimulation was perceived by subjects. Body position (sitting and standing) and anatomical locations were randomized between the subjects.
Due to a possible influence of contact force between the foot and the probe during data collection, forces were controlled throughout the measurements, whereby it should not vary more than 1 N (Cassella et al., Citation2000).
2.4. Statistical analysis
The mean value of the five measurements was used to determine the VPT for each subject at each condition. By virtue of non-parametrical data tested using the Shapiro–Wilk test, differences between genders were compared with a Mann–Whitney test (α = 0.05) and differences between the conditions were analysed with a Wilcoxon-Test. Bonferroni correction was used in order to adjust the initial level of significance (from α = 0.05 to α = 0.05/3 = 0.016), by taking account of the three analyzed anatomical locations. SPSS version 18.0 was used for all statistical tests. Based on data from a previous study (Schlee, Sterzing, & Milani, Citation2009), differences between the conditions were considered relevant when >2.5 μm.
3. Results
Plantar temperatures at the heel were 26.8 ± 2.1 and 26.8 ± 2.0°C for standing and sitting conditions, respectively. Statistical analysis revealed no significant differences.
The contact forces between the probe and the location were measured for both body positions, and were higher for standing in comparison to sitting for all analyzed locations. As shown in , the contact forces increase in the standing position (%) compared to the sitting position. The contact force on the heel during standing was 62.6% higher than during sitting. On the first metatarsal head (Met I) and hallux the contact forces for the standing position were also higher than while sitting.
Figure 1. Increase in contact forces in the standing position compared to sitting.
Note: Met I = first metatarsal head.
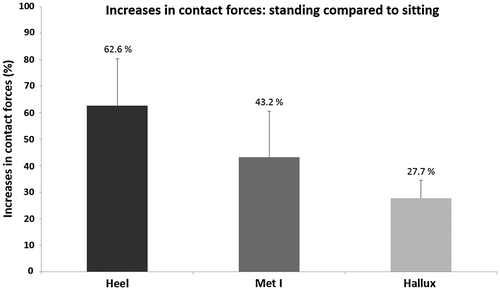
There were no significant differences in vibration thresholds when comparing men and women at all analyzed anatomical locations and both conditions. Therefore, the results were analyzed over the whole group (n = 66) (). The comparison of VPTs between standing and sitting conditions showed no significant differences in any of the analyzed anatomical locations ().
4. Discussion
Plantar temperatures pre and post trials only varied by 0.4°C on average. Guaranteeing this uniformity is important since skin sensitivity depends on skin temperature (Schlee et al., Citation2009).
Not surprisingly, contact forces during standing were higher compared to sitting. During standing, the body weight resulted in higher force applied against the probe, especially at the heel, which carries 60% of the weight-bearing load (Cavanagh, Rodgers, & liboshi, Citation1987).
Contact force is an influencing factor for VPT measurements. Hagander et al. (Citation2000) investigated the effects of vertically applied masses (30–100 g) on dorsal VPTs at 100 Hz and did not find any differences. Cassella et al. (Citation2000) varied masses (0–100 g) applied to the head of a neurothesiometer and found lower VPTs as masses increased. Likewise, using 200 and 400 g, the VPTs of healthy subjects and subjects with diabetic neuropathy showed reduced values as contact forces increased (Lowenthal & Derek, Citation1987). However, neither study analyzed plantar aspects. Another issue is that the neurothesiometer lacks repeatability due to non-linear behavior when expressing VPTs in micrometer (Schlee, Schleusener, & Milani, Citation2012). Although Casella et al. (Citation2000) presented VPTs in volts, comparing this data is still difficult since the common and preferred unit of VPTs is micrometer (Schlee et al., Citation2012).
In our study, with even higher forces applied towards the probe during standing, VPTs showed no significant or relevant (<2.5 μm) differences between conditions. This means that the perception of vibratory stimuli was not better during standing compared to sitting, which contradicts our hypothesis. Therefore, higher forces do not seem to induce increased receptor activity.
To our knowledge, the study by Mildren et al. (Citation2016) is the only investigation that compared plantar VPTs during standing and sitting. Similar to our study, they also did not find significant differences at the heel array at 250 Hz, but rather increased VPTs at the metatarsal array during standing. This contrary finding may result from the different probe configurations. Their probe alignment led to a greater area of stimulated skin. Possibly, this changed skin properties like stiffness (Fontanella, Carniel, Forestiero, & Natali, Citation2014), inducing alterations of the transmission of mechanical stimuli towards the receptor (Mildren et al., Citation2016). We presumably did not find the same effects as Mildren et al. (Citation2016) did due to the smaller area of stimulated skin in our study.
5. Conclusions
In conclusion, no differences between VPTs were present in our study, despite higher forces for standing compared to sitting. These results have potentially important implications for future research as well as for clinical diagnosis, in which plantar perception and balance control are closely related and implemented in various measuring setups, especially for patient populations or older adults. Future studies should objectively investigate whether vibrational firing rates are the same already at the receptor level when comparing various measuring positions.
Additional information
Funding
Notes on contributors
Andresa M.C. Germano
The research activities of the present group are related to the interaction of the human sensory and motor system. In particular, basic research related to plantar mechanoreceptors is one research focus (for example, determining sensory thresholds, influencing factors of cutaneous sensitivity). Furthermore, the contribution of plantar mechanoreceptors towards balance is another research emphasis. In this context, various experiments based on quasi-static and dynamic balance tasks are performed. Additionally, clinical studies with various patient groups (e.g. Alzheimer dementia) are implemented with focus on sensory and motor behavior.
References
- Berg, K., & Norman, K. (1996). Functional assessment of balance and gait. Clin Geriatr Med, 12, 705–723.
- Cassella, J. P., Ashford, R. L., & Kavanagh-Sharp, V. (2000). Effect of applied pressure in the determination of vibration sensitivity using the neurothesiometer. The Foot, 10, 27–30.10.1054/foot.2000.0575
- Cavanagh, P., Rodgers, M., & liboshi, A. (1987). Pressure distribution under symptom-free feet during barefoot standing. Foot & Ankle International, 7, 262–278.10.1177/107110078700700502
- Fontanella, C., Carniel, E., Forestiero, A., & Natali, A. (2014). Investigation of the mechanical behaviour of the foot skin. Skin Research and Technology, 20, 445–452.10.1111/srt.2014.20.issue-4
- Hagander, L., Midani, H., Kuskowski, M., & Parry, G. J. (2000). Quantitative sensory testing: Effect of site and skin temperature on thermal thresholds. Clinical Neurophysiology, 111, 17–22.10.1016/S1388-2457(99)00192-3
- Hayashi, R., Tako, K., Tokuda, T., & Yanagisawa, N. (1992). Comparison of amplitude of human soleus H-reflex during sitting and standing. Neuroscience Research, 13, 227–233.10.1016/0168-0102(92)90062-H
- Kavounoudias, A., Roll, R., & Roll, J. P. (1998). The plantar sole is a “dynamometric map” for human balance control. NeuroReport, 9, 3247–3252.10.1097/00001756-199810050-00021
- Koceja, D. M., Trimble, M. H., & Earles, D. R. (1993). Inhibition of the soleus H-reflex in standing man. Brain Research, 629, 155–158.10.1016/0006-8993(93)90495-9
- Leonard, D., Farooqi, M., & Myers, S. (2004). Restoration of sensation, reduced pain, and improved balance in subjects with diabetic peripheral neuropathy: A double-blind, randomized, placebo-controlled study with monochromatic near-infrared treatment. Diabetes Care, 27, 168–172.10.2337/diacare.27.1.168
- Lowenthal, L., Derek, T., & Hockaday, R. (1987). Vibration sensory thresholds depend on pressure of applied stimulus. Diabetes Care, 10, 100–102.10.2337/diacare.10.1.100
- Mildren, R. L., Strzalkowski, N. D. J., & Bent, L. R. (2016). Foot sole skin vibration perceptual thresholds are elevated in a standing posture compared to sitting. Gait Posture, 43, 87–92.10.1016/j.gaitpost.2015.10.027
- Mynark, R. G., & Koceja, D. M. (1997). Comparison of soleus H-reflex gain from prone to standing in dancers and controls. Electroencephalography and Clinical Neurophysiology/Electromyography and Motor Control, 105, 135–140.10.1016/S0924-980X(96)96096-8
- Nurse, M., & Nigg, B. (1999). Quantifying a relationship between tactile and vibration sensitivity of the human foot with plantar pressure distributions during gait. Clinical Biomechanics, 14, 667–672.10.1016/S0268-0033(99)00020-0
- Perry, S. D., McIlroy, W. E., & Maki, B. E. (2000). The role of plantar cutaneous mechanoreceptors in the control of compensatory stepping reactions evoked by unpredictable, multi-directional perturbation. Brain Research, 877, 401–406.10.1016/S0006-8993(00)02712-8
- Schlee, G., Schleusener, M., & Milani, T. L. (2012). Repeatability of vibration thresholds measured with the neurothesiometer: Is it a valid and reliable research tool? Clinical Neurophysiology, 123, 1053–1054.10.1016/j.clinph.2011.09.021
- Schlee, G., Sterzing, T., & Milani, T. L. (2009). Foot sole skin temperature affects plantar foot sensitivity. Clinical Neurophysiology, 120, 1548–1551.10.1016/j.clinph.2009.06.010
- Verrillo, R. (1985). Psychophysics of vibrotactile stimulation. The Journal of the Acoustical Society of America, 77, 225–232.10.1121/1.392263