Abstract
The aim of this study was to identify the influence of maturation on sprinting and jump performances, whilst quantifying injury markers in 95 youth males (age 13.2–15.7y). Comparative statistics were performed between maturation groups, identifying significant differences (p < 0.05) in all measured anthropometric variables. Sprint and jump performances were positively influenced (ES = trivial to large) by maturation status, whereas injury markers revealed no significant differences between groups. Increases in limb length, muscle size and muscular force output due to corresponding neuromuscular maturation, may help account for these improvements. Individuals’ within the circa PHV group exhibit a larger variability in sprint and jump performance when compared to the pre or post maturation groups. This variability may be attributed to the under-researched phenomenon of “adolescent awkwardness”. To conclude, performance characteristics of youth of similar chronological age vary considerably based on biological maturation, which may have consequences for long-term athlete development.
Keywords:
PUBLIC INTEREST STATEMENT
Adolescent maturity is a process we all experience and the physical changes that occur mean that it is likely something we remember. The unique individualised process presents itself differently from person to person, meaning the overall impact can be equally varied. This statement is confirmed by this research which showed sprint and jump performances improved significantly as maturation occurred, but these improvements may not necessarily match with the chronological age of the individuals. Markers of injury did not change significantly throughout maturation, suggesting the risk during this time is still of concern even before, or after, the adolescent growth spurt. Coaches, trainers and parents can use this information to help inform sporting and physical activity preferences and team selection for children around this period. By understanding the individual nature of growth, more care can be used to provide effective training and movement experiences to promote long term development for all.
Competing interests
The authors report no conflict of interest.
1. Introduction
During the adolescent growth phase, it is common to see variability within a variety of physical characteristics such as height, weight, and limb length when comparing individuals’, even those from a similar population or ethnicity (Mirwald, Baxter-Jones, Bailey, & Beunen, Citation2002; Philippaerts et al., Citation2006). These variations can be tentatively measured by quantifying the individuals’s level of biological maturity, also known as their biological age (Meyers, Oliver, Hughes, Lloyd, & Cronin, Citation2017; Sherar, Esliger, Baxter-Jones, & Tremblay, Citation2007; Van Der Sluis et al., Citation2014). Age at peak height velocity (PHV) is a term used to describe the period where the maximum rate of growth occurs during the adolescent growth spurt. By calculating time to, or from PHV, it is possible to provide a non-invasive quantitative estimate of an individual’s level of biological maturation (Philippaerts et al., Citation2006; Van Der Sluis et al., Citation2014). The timing and intensity of this PHV period differs between individuals’ and is genetically determined by a wide range of inherited variables (Mao et al., Citation2013; Sovio et al., Citation2009). Research has shown that as an individual enters their adolescent growth spurt, there are often resultant implications for their motor control and coordination (Lloyd, Radnor, De Ste Croix, Cronin, & Oliver, Citation2016a). The term “adolescent awkwardness” has been used to describe this phenomenon, which is caused by an increase in bone length, typically within the trunk and limbs, prior to the corresponding muscular growth and is primarily evident during the peak of vertical growth (Van Der Sluis et al., Citation2014). This occurrence is accompanied by an imbalance between strength and flexibility, which also increases the risk of both structural and soft tissue injuries (Hägglund & Waldén, Citation2016; Van Der Sluis et al., Citation2014), but is still a poorly understood concept in maturation literature (Meyers et al., Citation2017). Despite these physiological fluctuations in coordination and growth, some literature suggests adolescent awkwardness does not correspond with a decrease in performance in activities requiring high force outputs or multiple segment sequencing such as sprinting and jumping (Lloyd et al., Citation2016a).
Sprinting is an activity that is present in a variety of youth sporting activities, and is underpinned by several key kinetic and kinematic determinants (Hunter, Marshall, & McNair, Citation2004). Aspects such as ground reaction force (GRF) (Kawamori, Nosaka, & Newton, Citation2013), contact time, flight time (Cronin & Hansen, Citation2006), step length, and step frequency (Meyers, Citation2016) have been shown to strongly impact sprint performance. These variables are directly influenced by discrete physiological attributes such as strength, power and flexibility (Cavagna & Franzetti, Citation1986; Franzetti & Heglund, Citation1988; Perrier, Pavol, & Hoffman, Citation2011). Both Murtagh et al. (Citation2017), and Meyers (Citation2016), suggest jump performance is similarly influenced by muscle size, motor unit activation and force output, as well as the discrete physical characteristics of the individual. As non-linear physical growth and neural maturation occur during the adolescent growth phase, the determining factors of sprinting and jumping may be positively influenced, suggesting those of the same chronological age will likely vary in ability depending on their own level of biological maturation (Meyers, Citation2016).
Due to the current lack of depth in findings within this area, the aim of this study was to identify the influence of PHV on maximal sprint and unilateral jump performance, whilst assessing the corresponding markers of injury in a school-based male youth setting, as opposed to an athletically driven cohort which has frequently been utilized within relevant literature. Variability of individual responses within each maturation group was sought, in an attempt to identify “adolescent awkwardness”. Based on the findings of Meyers et al. (Citation2017) and Philippaerts et al. (Citation2006) it was hypothesised that increases in height, seated height, and weight will be evident as maturation increases. These physical characteristics will lead to improvements in performance variables, with a higher occurrence of injury markers and variability during the circa period of PHV, as per the suggestions of Hägglund and Waldén (Citation2016), and Van Der Sluis et al. (Citation2014).
2. Methods
2.1. Study design
This study utilised a cross-sectional study design to compare descriptive data from three distinct representative groups within the targeted male youth population. Comparative groups were allocated post testing with the use of a sex-specific maturity offset calculation (Mirwald et al., Citation2002) which utilises height, seated height and limb length, to measure maturity offset (pre < −0.50 y, circa −0.49 y to +0.49 y, post> +0.5 y) (Meyers et al., Citation2017). Despite this equation having a reported error of ± 0.592 y (Meyers et al., Citation2017), the allocations were made in accordance with similar studies (Meyers, Citation2016; Meyers et al., Citation2017), and to allow better distribution across maturation groups within this population.
2.2. Participants
A total of 95 youth males (age 13.2–15.7 y; maturity offset −1.0 to 2.6 y) volunteered for this study. There were no performance or sporting pre-requisites for this study, as a representation of general youth ability was sought. Participants were required to demonstrate their suitability via a completed health questionnaire (with no contraindications present) and guardian consent. Ethical approval was granted for all procedures from the Waikato Institute of Technology human ethics research group.
2.3. Experimental procedures/data collection
Participants were required to attend one single testing session lasting approximately one hour within an indoor gym facility. All tests were performed in bare feet whilst wearing appropriate active-wear. A standardised warm up was undertaken prior to the session, which lasted approximately 12 min and consisted of dynamic, progressive exercises targeting the whole body initially, then the lower limbs specifically. Familiarisation occurred prior to the commencement of each test via verbal instruction and a visual demonstration. Each participant was provided the opportunity to practice each movement prior to the recorded trials.
Anthropometrics (height, seated height and weight) were measured on testing day to provide information for the maturity offset calculation (Mirwald et al., Citation2002). Standing height was measured via a free-standing stadiometer, with participants feet shoulder width apart and the chin and line of sight parallel to the floor. The headpiece was lowered firmly on the centre of the participants head whilst they were standing with erect posture. Seated height was measured whilst sitting on a 30cm anthropometric box placed against a wall with a tape measure aligned vertically from centre of the box. Participants had their legs together and hands rested on their knees. The lower back was firmly against the wall at the rear of the box and the chin and eye line were parallel to the floor. The headpiece was lowered firmly on to the participants head, ensuring a right angle was kept with the wall. Both standing and seated heights were measured to the nearest millimetre. Weight was taken on a set of electronic scales which were zeroed prior to each participants measurement.
Participants performed three maximal effort 20m sprints (2 min rest between each trial), utilising a standing split stance with their preferred foot placed on the starting line 0.5m back from first timing light (White & Gunter, Citation2002). A dual-beam-modulated SWIFT timing light system (Wacol, Australia), captured performance times using four sets of lights placed at the zero, 5 m, 10 m and 20 m marks, at a height of 0.85m (to top of tripod), with the lane width approximately 3m. The initial set of timing lights was set lower (65cm to the top of tripod) than the other tripods to account for the likely hunched start positions of the participants. Each trial began with a forward movement of the torso, as opposed to a rocking motion where momentum could be generated prior to first foot movement. Once instructed to step up to the line, the participant was free to commence the trial in their own time to remove any variability in reaction times.
Maximal unilateral horizontal jump performance was obtained via three jumps for distance from each leg (take-off one leg and land with two), with approximately 2 min rest between trials (alternating legs each trial). Measurements were taken from the rear-most heel on a successful landing. An unsuccessful landing consisted of an individual falling backwards, stepping backwards, or putting their hands down behind the rear-most heel (these trials were repeated). Hands were free to move throughout the movement and no coaching or technical cues were given.
A single 10s bilateral tuck jump (TJ) assessment was performed and qualitatively marked against a modified rubric (Table ) (Fort-Vanmeerhaeghe, Montalvo, Lloyd, Read, & Myer, Citation2017), which provides insight into specific injury markers such as quadricep dominance, trunk dominance, leg dominance, ligament dominance, feedforward mechanisms and neuromuscular fatigue. Intra-rater reliability statistics (ICC) for the modified TJ assessment was calculated at 0.971 (substantial) and a 93% PEA, with Kappa scores ranging from 0.615 to 1.00 (p < 0.05) for each of the 10 individual variables within the rubric, which are in alignment with previous reliability studies surrounding this model (Herrington, Myer, & Munro, Citation2013; Read, Oliver, de Ste Croix, Myer & Lloyd, Citation2017). On the gym surface where the test was to be performed, tape was used to create a box with edges 41cm in length and 35cm wide, which the participants were instructed to remain on if possible (Fort-Vanmeerhaeghe et al., Citation2017). This assessment required the participant to perform continuous tuck jumps for a period of 10s within the specified area (if possible). Instructional cues consisted of the following; “bring knees to chest”, “continuous jumps for 10s”, “jump as high as you feel comfortable”. Two high-speed cameras (Casio Exilim, ex-zr200) capturing at 120fps on fixed tripods (set at 0.8m to base of tripod) provided frontal and sagittal views of the participant during their tuck jump assessment. Scores were allocated via post-session video analysis and compared against a severity based kinematic marking criteria (Table ).
Table 1. Modified TJ rubric derived from Fort-Vanmeerhaeghe et al. (Citation2017)
2.4. Statistical analysis
The two best individual sprint and jump trials were averaged for each participant and used as their comparative score, as per the recommendations of Maulder, Bradshaw, and Keogh (Citation2008). A one-way ANOVA with Bonferroni post hoc test was used to assess between group differences in sprint times, jump performance and tuck jump scores (SPSS Version 22, IBM, Armonk, NY). Cohens effect sizes and percentage differences with 90% confidence limits were calculated between maturation status and performance variables with qualitative inferences used to describe effect magnitudes (Hopkins, Citation2002), with p-values (p < 0.05) were used to indicate significance.
3. Results
Anthropometric data revealed significant differences (p < 0.05) in all measured variables (height, seated height and weight) between maturation groups (see Table ).
Table 2. Anthropometric variables compared between maturation groups
Significant group differences (p < 0.01) revealed increased maturation status positively influenced sprint performances. The circa group displayed faster sprint times (0.1%, 0.7% and 1.8%) than the pre group over 5m, 10m and 20m distances, respectively (see Table ). Significantly faster (p < 0.01) mean sprint times were demonstrated by the post group over all distances in comparison to both circa and pre groups (see Figure ), with statistics inferring “small” to “large” effect sizes (see Table ).
Table 3. Comparative statistics between maturation groups and performance variables
Figure 1.: 5 m, 10 m, and 20 m individual sprint times across maturation groups.
Note: A = 5 m times; B = 10 m times; C = 20 m times; # = significant difference to pre; * = significant difference to circa.
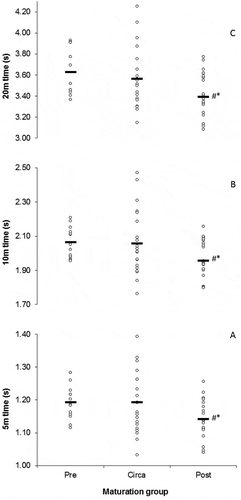
Dominant (HJD) and non-dominant (HJND) horizontal jump tests demonstrated greater jump performance with increased maturation. The circa group had mean jump distances 3.5% and 3.7% larger than pre groups (ES = 0.29–0.30), when comparing respective dominant and non-dominant legs (see Table ). Post groups revealed greater dominant and non-dominant jump distances (1.67m ± 0.22; 1.61m ± 0.22) in comparison to the circa group (1.62m ± 0.22; ±; 1.54m ± 0.22), with comparative statistics inferring trivial to moderate effect sizes (see Table ). Post group had significantly further jump distances (p < 0.01) than pre group in the HJD (see Figure ).
Figure 2. Mean horizontal jump performance on dominant and non-dominant legs across maturation groups.
Note: Figure = HJD; Figure = HJND; # = significantly different to pre.
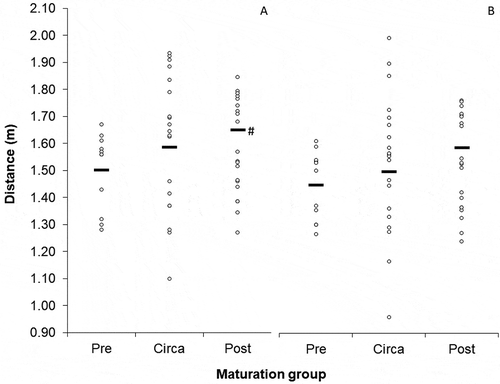
Tuck jump total scores (TJS) identified no significant differences between pre (12.8 ± 2.0), circa (12.5 ± 2.6) or post (12.3 ± 3.3) group means. Effect sizes of 0.25–0.83 depict moderate to unclear statistical differences between groups (see Table ). There were no significant differences between any specific injury markers when group comparisons were made.
4. Discussion
The purpose of this study was to identify the influence of maturation on maximal sprinting, jumping performances and injury markers in adolescent males. Current findings from literature within this field led to the hypothesis that; as maturation occurred, increases in physical characteristics such as height, seated height, and weight would be evident within the population; these findings would also be coupled with corresponding improvements in sprint and jump performance. Secondly, markers of injury and increased performance variability would be most prevalent within the circa stage of maturation due to the rapid onset of physical growth and the associated neurological changes. Results of this study partially supported these hypotheses, with anthropometric and performance variables demonstrating comparable findings to previous literature (Asadi, Ramirez-Campillo, Arazi, & Sáez de Villarreal, Citation2018; Meyers et al., Citation2017) whereas injury marker data revealed dissimilar trends to those originally hypothesised.
Anthropometric testing of height, seated height and weight, displayed significantly different values between maturation groups (see Table ), which supports both the hypothesis of this study and previous literature investigating this concept (Meyers et al., Citation2017; Murtagh et al., Citation2017). The adolescent growth phase is characterised by an alteration in hormonal activity, namely with increases in androgens such as testosterone, growth hormone and thyroid hormone (Ford et al., Citation2011). Increases in these androgen concentrations aid the facilitation of growth in muscular length and cross-sectional area, as well as differential bone growth throughout the appendicular limbs and trunk (Ford et al., Citation2011; Meyers, Citation2016; Van Der Sluis et al., Citation2014). The concomitant mass that accompanies this growth can be detrimental to performance and/or the underlying mechanisms related to performance (Meyers et al., Citation2017); although it is believed that the associated increases in physical size may also negate these potential decrements (Meyers, Citation2016; Meyers et al., Citation2017).
Articles by Meyers (Citation2016), and Meyers et al. (Citation2017), discuss how alterations in anthropometric measures (including standing height and limb length) and the corresponding body tissues may elicit improvements in motor activities such as sprinting. These findings were supported by the current study, where mean sprint times at 5 m, 10 m and 20 m improved as biological maturation (and anthropometric measures) increased (see Figure ). Lower limb length aids in increasing step length (Meyers, Citation2016), as well as decreasing ground contact time in some populations (Lloyd et al., Citation2016a), consequently improving sprint performance if other variables such as step frequency and flight time are not detrimentally altered in the process (Hunter et al., Citation2004). The larger percentage increases observed in the circa to post transition, compared to the pre to circa stage, are likely due to the increases in muscular strength and force output of individuals’ as they mature physically post PHV (Ford et al., Citation2011; Meyers, Citation2016; Van Der Sluis et al., Citation2014). The elevated testosterone and growth hormone levels allow for greater muscular development, therefore increasing the potential force and power output which are vital for early sprint speed success (Spinks, Murphy, Spinks, & Lockie, Citation2007). Despite comparable performance trends to current literature, the underlying kinematic mechanisms could not be confirmed in this study as the measuring of such variables was outside the intended scope.
Similarly, jump data displayed improved unilateral jump distance (both dominant and non-dominant legs) with increased maturation (see Figure ). Literature pertaining to this topic share analogous conclusions suggesting there are both physical and neural adaptations that occur with physical maturation and therefore improve subsequent jump performance, as observed in sprinting (Asadi et al., Citation2018; Murtagh et al., Citation2017). Murtagh et al. (Citation2017), specifies adaptations such as increases in muscle size, motor unit activation, and therefore the potential muscular output may all contribute to the improvements observed in jump performances. This statement is supported by Meyers (Citation2016), who displayed increases in absolute vertical and horizontal forces as maturation increased when sprinting, likely due to the neural and muscular advancements associated with maturation. The improvements in mean sprint times and jump distance that occurred congruently with increased maturation were not significant. It is hypothesised that this lack of significance is due to the increased variation and spread within the circa data set.
The concepts of neuromuscular disconnect and adolescent awkwardness discussed by Hägglund and Waldén (Citation2016), and Van Der Sluis et al. (Citation2014), did not have a notable detrimental effect on sprint or jump performance, as supported by the findings of Lloyd et al. (Citation2016a) and Meyers (Citation2016). Despite these comparable outcomes, both the sprint and jump data revealed a larger individual spread of performances within the circa maturation group in comparison to the pre and post groups (see Figures and ); suggesting performance within this circa group is more variable. It is hypothesised this variability is due to the highly genetic and variable nature of individual growth which is maximised during this maturation period due to the onset of PHV. The concept of adolescent awkwardness is currently under-researched and there is little definitive evidence to suggest any true implications (Ford et al., Citation2011; Meyers et al., Citation2017). The phenomenon is characterised by a rapid increase in physical growth, specifically within the bones of the trunk and limbs, causing a temporary disconnect between the associated musculature and nervous system, resulting in a disruption in motor coordination (Philippaerts et al., Citation2006). It could be assumed, that those who exhibit more rapid, and/or greater physical growth, may in fact be more susceptible to this perceived disconnect, which would help explain the larger variability within the circa data. Further investigation into this concept is warranted to provide insight into the possible implications of this phenomenon on both performance and injury. It is important to note that the participants who exhibited the greatest variability from the circa group mean, were not those individuals’ within the maturity offset calculations ±0.592 y error, as indicated by Meyers et al. (Citation2017).
Current literature states that during the adolescent growth phase (circa period), incidence of traumatic injuries (a single identifiable event) increases, with mechanisms such as joint stiffness, decreased bone density and abnormal movement mechanics likely contributing to the change (Ford, Myer, & Hewett, Citation2014; Van Der Sluis, Elferink-Gemser, Brink, & Visscher, Citation2015; Van Der Sluis et al., Citation2014). Overuse injuries (repeated micro trauma with no identifiable single event) were seen to increase post PHV, with Van Der Sluis et al. (Citation2015), suggesting that the increased susceptibility to injury is due to changes in muscular properties and tendon strength occurring after the maturational growth of bones and increase in muscular strength. The TJ test used within this study did not provide a rate of injury occurrence, but it could be reasonably assumed that those who are more prone to injury would display higher injury markers than other populations (Fort-Vanmeerhaeghe et al., Citation2017). The TJ scores revealed no significant differences between total scores, or any specific injury marker between maturation groups. It is believed that these findings can be attributed to the large variability within abilities across all maturation groups. The singular modified test, although deemed reliable during this study (ICC = 0.971), which is accordance with the findings of similar studies (Fort-Vanmeerhaeghe et al., Citation2017; Herrington et al., Citation2013), may lack applicability due to the complex, individualised, and variable nature of the injury markers (Read, Oliver, de Ste Croix, Myer, & Lloyd, Citation2017). It is recommended that a more robust testing battery be implemented when trying to quantify the markers of injury, or injury incidence within a non-athletic male youth population.
5. Conclusion
The results of this study suggest that biological maturation has a significant influence on anthropometric variables and sprint performance, whilst also positively influencing horizontal jump performance. These findings are consistent with literature surrounding these concepts (Asadi et al., Citation2018; Meyers, Citation2016; Meyers et al., Citation2017; Murtagh et al., Citation2017) and are likely due to the influence of the musculoskeletal growth that occurs during the adolescence (Meyers, Citation2016; Murtagh et al., Citation2017). Data suggests that individuals’ within their adolescent growth spurt (circa group) exhibit a larger variability in sprint and jump performance when compared to those in the pre or post PHV stages. It is hypothesised this is due to genetic factors that dictate the rate and intensity of musculoskeletal growth. Those who exhibit a steeper growth curve may be more prone to exhibiting a phenomenon known as adolescent awkwardness (Van Der Sluis et al., Citation2014). It is recommended that further research surrounding this neuromuscular disconnect is performed to identify its impact on the adolescent population, and the possible implications this disconnect may have on specific injury markers. It would also be beneficial to identify the effects of neuromuscular training interventions and their effectiveness of decreasing the prevalence of injury markers within youth. Results of this study display the key anthropometric and performance differences between children of similar ages. Further research into the kinematic variables such as step length, step frequency and contact times within the non-athletic youth setting would help to identify the underlying mechanisms behind these observed differences. It is also recommended that future research into school-based male youth include a measure of habitual physical activity to allow conclusions to be made about the over-arching activity levels of the cohort. Finally, investigations into the timing (pre, circa post PHV) of specific training interventions to improve sprint and jump performance is also warranted due to the differences observed within this study, and the implications these may have for motor skill acquisition and motor refinement.
Additional information
Funding
Notes on contributors
Regan J. Standing
This research article is part of a wider project investigating how coaches, trainers and parents can influence youth in a practical and applied manner in order to attain the most effective outcomes for both competitive and non-competitive sporting pursuits. This includes the need to understand what, when, and why maturity occurs, and how meaningful interactions through strength and conditioning, coaching, psychology and biomechanical interventions can maximise success long-term. This project provides insight into how maturation differs between individuals and the corresponding effects this may have on various modalities of performance. The researchers involved in this project have expertise in a range of sport science areas including coaching, biomechanics, ergogenic aids and physiology and are intent on applying this knowledge within the youth sector.
References
- Asadi, A., Ramirez-Campillo, R., Arazi, H., & Sáez de Villarreal, E. (2018). The effects of maturation on jumping ability and sprint adaptations to plyometric training in youth soccer players. Journal of Sports Sciences, 36(21), 1–11.
- Cavagna, G. A., & Franzetti, P. (1986). The determinants of the step frequency in walking in humans. The Journal of Physiology, 373(1), 235–242. doi:10.1113/jphysiol.1986.sp016044
- Cronin, J., & Hansen, K. T. (2006). Resisted sprint training for the acceleration phase of sprinting. Strength and Conditioning Journal, 28(4), 42–51. doi:10.1519/00126548-200608000-00005
- Ford, K. R., Myer, G. D., & Hewett, T. E. (2014). Longitudinal effects of maturation on lower extremity joint stiffnes in adolescente athletes. The American Journal of Sports Medicine, 38(9), 1829–1837.
- Ford, P., De Ste Croix, M., Lloyd, R., Meyers, R., Moosavi, M., Oliver, J., … Williams, C. (2011). The long-term athlete development model: Physiological evidence and application. Journal of Sports Sciences, 29(4), 389–402. doi:10.1080/02640414.2010.536849
- Fort-Vanmeerhaeghe, A., Montalvo, A. M., Lloyd, R. S., Read, P., & Myer, G. D. (2017). Intra- and inter-rater reliability of the modified tuck jump assessment. Journal of Sports Science and Medicine, 16(1), 117–124.
- Franzetti, P., & Heglund, N. C. (1988). Trotting and hopping in man and other vertebrates. Physiology, 81–92.
- Hägglund, M., & Waldén, M. (2016). Risk factors for acute knee injury in female youth football. Knee Surgery, Sports Traumatology, Arthroscopy : Official Journal of the ESSKA, 24(3), 737–746. doi:10.1007/s00167-015-3922-z
- Herrington, L., Myer, G. D., & Munro, A. (2013). Intra and inter-tester reliability of the tuck jump assessment. Physical Therapy in Sport, 14(3), 152–155. doi:10.1016/j.ptsp.2012.05.005
- Hopkins, W. (2002). A scale of magnitudes for effect statistics. Retrieved from http://www.sportsci.org
- Hunter, J. P., Marshall, R. N., & McNair, P. J. (2004). Interaction of step length and step rate during sprint running. Medicine and Science in Sports and Exercise, 36(2), 261–271. doi:10.1249/01.MSS.0000113664.15777.53
- Kawamori, N., Nosaka, K., & Newton, R. (2013). Relationships between ground reaction impulse and sprint acceleration performance in team sport athletes. The Journal of Strength and Conditioning Research, 27(3), 568–573. doi:10.1519/JSC.0b013e318257805a
- Lloyd, R. S., Radnor, J. M., De Ste Croix, M. B. A., Cronin, J. B., & Oliver, J. L. (2016a). Changes in sprint and jump performance after traditional, plyometric and combined resistance training in male youth pre- and post- peak height velocity. Strength And Conditioning Research, 30(5), 1239–1247. doi:10.1519/JSC.0000000000001216
- Mao, S., Xu, L., Zhu, Z., Qian, B., Qiao, J., Yi, L., & Qiu, Y. (2013). Association between genetic determinants of peak height velocity during puberty and predisposition to adolescent idiopathic scoliosis. Spine, 38(12), 1034–1039. doi:10.1097/BRS.0b013e31828cb001
- Maulder, P. S., Bradshaw, E. J., & Keogh, J. W. L. (2008). Kinematic alterations due to different loading schemes in early acceleration sprint performance from starting blocks. Journal of Strength and Conditioning Research, 22(6), 1992–2002. doi:10.1519/JSC.0b013e31818746fe
- Meyers, R. (2016). The influence of age, growth and maturation upon maximal sprint speed in male youth ( Doctoral dissertation, Cardiff Metropolitan University).
- Meyers, R. W., Oliver, J. L., Hughes, M. G., Lloyd, R. S., & Cronin, J. B. (2017). The influence of age, maturity and body size on the spatiotemporal determinants of maximal sprint speed in boys. Journal of Strength and Conditioning Research, 31(4), 1. doi:10.1519/JSC.0000000000002383
- Mirwald, R. L., Baxter-Jones, A. D. G., Bailey, D. A., & Beunen, G. P. (2002). An assessment of maturity from anthropometric measurements. Medicine and Science in Sports and Exercise, 34(4), 689–694.
- Murtagh, C. F., Brownlee, T. E., O’Boyle, A., Morgans, R., Drust, B., & Erskine, R. M. (2017). The importance of speed and power in elite youth soccer depends on maturation status. Journal of Strength and Conditioning Research, 44, 1. doi:10.1519/JSC.0000000000002367
- Perrier, E. T., Pavol, M. J., & Hoffman, M. A. (2011). The acute effects of a warm-up including static or dynamic stretching on countermovement jump height, reaction time, and flexibility. Journal of Strength and Conditioning Research, 25(7), 1925–1931. doi:10.1519/JSC.0b013e3181e73959
- Philippaerts, R. M., Vaeyens, R., Janssens, M., Van Renterghem, B., Matthys, D., Craen, R., … Malina, R. M. (2006). The relationship between peak height velocity and physical performance in youth soccer players. Journal of Sports Sciences, 24(3), 221–230. doi:10.1080/02640410500189371
- Read, P., Oliver, J. L., de Ste Croix, M. B. A., Myer, G. D., & Lloyd, R. S. (2017). Reliability of the tuck jump injury risk screening assessment in elite male youth soccer players. Journal of Strength and Conditioning Research, 30(6), 1510–1516. doi:10.1519/JSC.0000000000001260
- Sherar, L. B., Esliger, D. W., Baxter-Jones, A. D. G., & Tremblay, M. S. (2007). Age and gender differences in youth physical activity: Does physical maturity matter? Medicine and Science in Sports and Exercise, 39(5), 830–835. doi:10.1249/mss.0b013e3181453546
- Sovio, U., Bennett, A. J., Millwood, L. Y., Molitor, J., O’Reilly, P. F., . J., Timpson, N., … Jarvelin, M. R. (2009). Genetic determinants of height growth assessed longitudinally from infancy to adulthood in the northern finland birth cohort 1966. PLoS Genetics, 5(3), 1–8. doi:10.1371/journal.pgen.1000409
- Spinks, C., Murphy, A., Spinks, W., & Lockie, R. (2007). The effects of resisted sprint training on acceleration performance and kinematics in soccer, rugby union, and Australian football players. Journal of Strength and Conditioning Research, 21(1), 77–85. doi:10.1519/00124278-200702000-00015
- Van Der Sluis, A., Elferink-Gemser, M. T., Brink, M. S., & Visscher, C. (2015). Importance of peak height velocity timing in terms of injuries in talented soccer players. International Journal of Sports Medicine, 36(4), 327–332. doi:10.1055/s-00000028
- Van Der Sluis, A., Elferink-Gemser, M. T., Coelho-E-Silva, M. J., Nijboer, J. A., Brink, M. S., & Visscher, C. (2014). Sport injuries aligned to peak height velocity in talented pubertal soccer players. International Journal of Sports Medicine, 35(4), 351–355.
- White, K., & Gunter, K. (2002). The quick step: A new test for measuring reaction time and lateral stepping velocity. Journal of Applied Biomechanics, 18, 271–277. doi:10.1123/jab.18.3.271