Abstract
Psychological stress-induced hyperthermia (PSH) is a basic physiological stress response to increase physical performances to defend homeostasis and life from stressors, such as natural enemies. However, excessive and long-lasting stressors can lead to chronic hyperthermia, particularly recognized in humans as a psychosomatic symptom called “psychogenic fever.” The sympathetic and neuroendocrine responses that can contribute to PSH include brown adipose tissue (BAT) thermogenesis, cutaneous vasoconstriction, tachycardia and glucocorticoid secretion. Research on the central circuits underlying these stress responses has recently revealed several fundamental circuit mechanisms including hypothalamomedullary pathways driving the sympathetic stress responses. Psychological stress activates a monosynaptic glutamatergic excitatory neurotransmission from the dorsomedial hypothalamus (DMH) to sympathetic premotor neurons in the rostral medullary raphe region (rMR) to drive BAT thermogenesis and tachycardia, leading to the development of PSH. This glutamatergic neurotransmission could be potentiated by orexin neurons in the lateral hypothalamus through their projections to the rMR. Psychological stress also activates another monosynaptic pathway from the DMH to the paraventricular hypothalamic nucleus to stimulate the hypothalamo-pituitary-adrenal axis for the secretion of glucocorticoids. PSH is independent from the prostaglandin-mediated trigger mechanism for inflammation-induced fever, and several forebrain regions are considered to provide stress-driven inputs to the DMH to activate the sympathetic- and neuroendocrine-driving neurons. The circuit mechanism of PSH based on animal experiments would be relevant to understandings of the etiology of psychogenic fever in humans. This review describes the current understandings of the central circuit mechanism of PSH with recent important progress in research.
Abbreviations
BAT | = | brown adipose tissue |
CNS | = | central nervous system |
CTb | = | cholera toxin b-subunit |
DMH | = | dorsomedial hypothalamus |
HPA | = | hypothalamo-pituitary-adrenal |
PGE2 | = | prostaglandin E2 |
POA | = | preoptic area |
PSH | = | psychological stress-induced hyperthermia |
PVH | = | paraventricular hypothalamic nucleus |
rMR | = | rostral medullary raphe region |
VGLUT | = | vesicular glutamate transporter. |
Introduction
Psychological stress elicits a variety of autonomic responses in mammals, such as hyperthermia, tachycardia and hypertension. These stress responses all contribute to boosting physical performances: the hyperthermia warming up muscles and the central nervous system (CNS) by a few degrees CelsiusCitation1 and the cardiovascular responses increasing blood flows in muscles and the CNS to supply more oxygen and fuels. Therefore, these stress-induced physiological responses are thought to be beneficial in surviving the “fight or flight” situations when animals confront enemies. In contrast to these acute responses, however, long-lasting, intense stressors can cause chronic elevations in physiological parameters including body temperature and blood pressure. The stress-induced chronic hyperthermia is often called “psychogenic fever” as a psychosomatic symptom.Citation2,3 A clinical study has reported that approximately half cases of “fever of unknown origin” (exhibiting no abnormality in diagnostic tests nor physical examination except high body temperature) are psychogenic.Citation4
While there are abundant animal experimental models that can evoke such autonomic stress responses mimicking those in humans, less attention has been given to the central mechanism for psychological stress-induced hyperthermia (PSH) than those for cardiovascular stress responses. However, remarkable progress of research on the central circuitries for body temperature regulation and fever in the last decade (see reviewCitation5) has just stimulated new research for the mechanism by which psychological stress affects the central thermoregulatory circuits to drive PSH. As described in this review, a large part of the elevation of body temperature in response to psychological stress is an outcome of enhanced sympathetic thermogenesis and metabolism as well as decreased heat loss from the body surface. These stress-evoked thermal responses are driven by central sympathetic pathways through neural projections from the hypothalamus to the medulla oblongata, in which sympathetic premotor neurons are stimulated. This hypothalamomedullary pathway appears to be shared with the central sympathetic drives for the development of inflammation-induced fever and cold defense. Although the central circuit mechanism that converts psychologically stressful events perceived to a neural “signal” is unknown, accumulated evidence suggests that corticolimbic structures function for such psychological and emotional processing and likely provide innervations to the central sympathetic pathways through their neural projections to the hypothalamus. Several candidates of the neural projections mediating such stress-driven signaling to the hypothalamus can be proposed based on available neuroscientific literature. The central circuit mechanism for acute PSH obtained from animal research would be helpful to reveal the etiology of psychogenic fever in humans. In this review, I describe the current understandings of the central circuit mechanism of PSH, incorporating recent important discoveries.
Sympathetic and neuroendocrine responses contributing to PSH
Studies using rodents have shown that psychological stress induces a prompt elevation of body temperature predominantly by increasing metabolic heat production and decreasing heat loss from the skin surface. For cold defense and inflammation-induced fev-er, brown adipose tissue (BAT) plays as a major organ to produce heat in rodents.Citation6,7 BAT thermogenesis also occurs in adult humans for the maintenance of body temperature in cold environments as well as for metabolic homeostasis to prevent obesity.Citation8-11 BAT thermogenesis is controlled by sympathetic innervation primarily through the β3-adrenoceptor, which is predominantly expressed in brown adipocytes.Citation12 Systemic administration of a β3-adrenoceptor antagonist in rats diminishes PSH,Citation13 and thermotelemetry recordings in free-moving rats can detect psychological stress-induced BAT thermogenesis, which is represented by a rapid increase in the temperature of the interscapular BAT by approximately 2°C that precedes a rise in body core temperature ().Citation14 These findings demonstrate that psychological stress induces BAT thermogenesis contributing to the development of PSH.
Figure 1. Social defeat stress-induced BAT thermogenesis and hyperthermia in rats. (A and B) Changes in BAT and abdominal (core) temperatures induced by social defeat stress (gray period with a horizontal bar). Changes from the temperature at time 0 are shown (n = 6). Temperature changes for 5 min after the beginning of the stress exposure are expanded in (B). (C) A site of muscimol nanoinjection in the rMR was labeled with fluorescent microspheres (arrow). py, pyramidal tract; RMg, raphe magnus nucleus; rRPa, rostral raphe pallidus nucleus. Scale bar, 500 µm. (D and E) Effect of muscimol or saline nanoinjection into the rMR (arrows) on social defeat stress-induced changes in BAT temperature (TBAT, D) and abdominal temperature (Tcore, E) (n = 5). Temperature changes from the baseline are shown (analyzed by 2-way ANOVA followed by Bonferroni's post-hoc test). **P < 0.01. All values are means ± SEM. Modified from Kataoka et al.Citation14 © Elsevier. Permission to reuse must be obtained from the rightsholder.
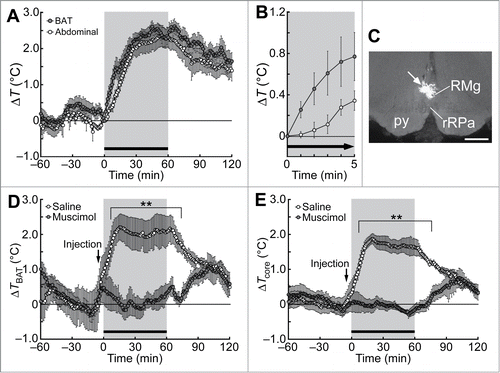
The decrease in radiative heat loss in response to psychological stress is mediated by cutaneous vasoconstriction,Citation15 an α-adrenoceptor-mediated sympathetic response to decrease skin blood flow. Stress-induced cutaneous vasoconstriction can be detected experimentally in the hairless skin of the tail and ears in rodents and rabbits by recording the blood flows with implanted Doppler ultrasonic probesCitation15,16 or by monitoring the skin temperature with infrared thermography.Citation17 The α1-adrenoblocker prazosin can attenuate PSH only at high doses in mice.Citation18 The strong inhibition of PSH with a β3-adrenoblockerCitation13 suggests that BAT thermogenesis makes a major contribution to the stress-induced rapid elevation of body temperature compared to cutaneous vasoconstriction.
Stress-induced tachycardia is an additional sympathetic response that can contribute to the development of PSH. Moderate tachycardia without decreasing stroke volume increases cardiac output and hence, can provide support for boosting the oxygen and fuel supplies to BAT and for the heat distribution from BAT to the rest part of the body. Furthermore, tachycardia may lead to increased thermogenic activity in the heart, proposed as “cardiac thermogenesis.”Citation19 In addition to the sympathetic responses, psychological stress activates the hypothalamo-pituitary-adrenal (HPA) neuroendocrine axis.Citation20 The activation of the HPA-axis increases the ACTH secretion from the pituitary and thereby, enhances the secretion of glucocorticoids from the adrenal cortex, which finally stimulate gluconeogenesis and lipolysis—2 catabolic events that can supply more fuels available to thermogenic organs.Citation21
Sympathetic premotor neurons driving PSH
Sympathetic premotor neurons integrate signals from various brain regions and provide excitatory commands to sympathetic preganglionic neurons in the spinal cord to drive effector responses. Sympathetic premotor neurons for BAT thermogenesis and cutaneous vasoconstriction are localized in the rostral medullary raphe region (rMR) including the rostral raphe pallidus and raphe magnus nuclei.Citation22,23 These neurons express the vesicular glutamate transporter (VGLUT) 3, potentially indicative of glutamatergic neurons, and directly innervate sympathetic preganglionic neurons in the thoracic spinal cord.Citation22,24 These neurons are labeled by transsynaptic retrograde tracing from rat interscapular BAT or tail skin blood vessels with a pseudorabies virus.Citation22 Many of VGLUT3-expressing neurons in the rMR express Fos, a marker for neuronal activation, following exposure to social defeat stress,Citation13 an animal model of psychological stress that is close to human social stress and induces PSH.Citation25 Supporting that this is psychogenic activation, the stress-induced activation of VGLUT3 neurons in the rMR is reversed by a systemic administration of diazepam, an anxiolytic agent.Citation13 These results raise the idea that psychogenic stress-driven central signals activate sympathetic premotor neurons in the rMR to drive BAT thermogenesis and cutaneous vasoconstriction.
Stimulation of neurons in the rMR with nanoinjection of neuroexcitatory drugs elicits BAT thermogenesis, cutaneous vasoconstriction and tachycardia.Citation26,27 These physiological responses suggest that the rMR contains sympathetic premotor neurons innervating the heart as well as those controlling BAT and skin blood vessels. Consistent with the idea that sympathetic premotor neurons in the rMR mediate the stress-induced sympathetic responses leading to PSH, inactivation of neurons in the rMR with nanoinjection of muscimol, a GABAA receptor aganist widely used as a neuronal inhibitor, suppresses psychological stress-induced BAT thermogenesis, hyperthermia () and cardiovascular responses.Citation14,28,29 Blockade of glutamate receptors in the rMR also inhibits the BAT thermogenesis, hyperthermia and tachycardia induced by social defeat stress.Citation14 These findings indicate that stress-driven glutamatergic inputs to the rMR, probably activating sympathetic premotor neurons, are required in the central circuit mechanisms for PSH and stress-induced tachycardia.
Hypothalamomedullary pathway driving PSH
We have recently investigated the upper brain regions that provide the stress-driven glutamatergic inputs to sympathetic premotor neurons in the rMR to drive PSH.Citation14 Functional neuroanatomy combining retrograde neural tracing and Fos immunohistochemistry revealed a population of neurons in the dorsomedial hypothalamus (DMH) that directly transmits stress-driven signals to the rMR. Injection of the retrograde neural tracer cholera toxin b-subunit (CTb) into the rat rMR labeled many neuronal cell bodies broadly distributed in dorsal areas of the hypothalamus, and subsequent exposure of the rats to social defeat stress induced Fos expression in the CTb-labeled neurons densely clustering in the dorsal part of the DMH ().Citation14 Supporting that this neuronal activation is psychogenic, social defeat stress-induced Fos expression in the DMH is reduced by systemic injection of diazepam.Citation30 Those stress-activated, rMR-projecting DMH neurons likely express the neuronal nitric oxide synthase.Citation31 Anterograde neural tracing showed that DMH neurons provide a direct projection to VGLUT3-expressing neurons in the rMR and that these DMH-derived nerve terminals apposed to VGLUT3-expressing neurons contain the glutamatergic neuronal marker VGLUT2.Citation14 These observations demonstrate that psychological stress activates a direct glutamatergic excitatory neurotransmission from the DMH to sympathetic premotor neurons in the rMR.
Figure 2. Social defeat stress activates rMR-projecting neurons and PVH-projecting neurons in the DMH. (A and B) Sites of injections of Alexa594-conjugated CTb (red) into the rMR (rMR-CTb, A) and Alexa488-conjugated CTb (green) into the PVH (PVH-CTb, B). Scale bars, 300 µm. (C and D) Fos immunoreactivity in CTb-labeled cells (arrows) in the dorsal part of the DMH (dDMH, C) and ventral part of the DMH (vDMH, D) following social defeat stress. Scale bars, 30 µm. (E and F) Distribution of rMR-CTb-labeled cells (red) and PVH-CTb-labeled cells (green) in the caudal hypothalamus of control (E) and stressed rats (F). Open and filled circles indicate cells negative and positive for Fos immunoreactivity, respectively. Very few cells were double-labeled with rMR-CTb and PVH-CTb (black circles). 3V, third ventricle; Arc, arcuate nucleus; cDMH, compact part of the DMH; f, fornix; ic, internal capsule; LH, lateral hypothalamic area; mt, mammillothalamic tract; ot, optic tract; VMH, ventromedial hypothalamic nucleus. Scale bar, 500 µm. Modified from Kataoka et al.Citation14 © Elsevier. Permission to reuse must be obtained from the rightsholder.
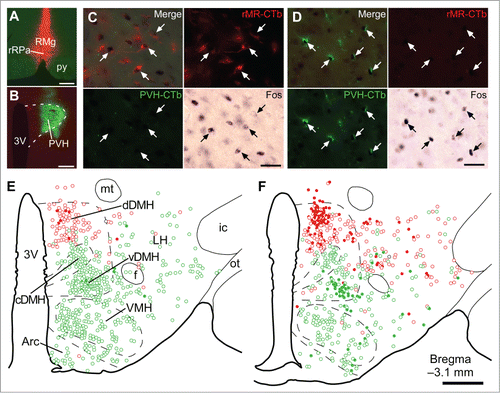
Inactivation of DMH neurons suppresses BAT thermogenic, hyperthermic, tachycardic and pressor responses to psychological stress.Citation14,32-34 Therefore, it was hypothesized that the monosynaptic pathway from the DMH to the rMR drives the stress-induced thermogenic and cardiovascular responses. To test this hypothesis, we selectively stimulated DMH-derived nerve endings in the rMR using an optogenetic technique ().Citation14 Viral transduction of DMH neurons with ChIEF, a channelrhodopsin-type cationic channel causing membrane depolarization and action potential when exposed to blue light,Citation35 resulted in the localization of this channel in many cell bodies in the DMH as well as in their nerve endings in the rMR ().Citation14 In vivo illumination of these nerve endings in the rMR consistently elicited BAT thermogenesis as well as increased heart rate and arterial pressure, mimicking stress-induced sympathetic responses ().Citation14 Photostimulation of the ChIEF-expressing cell bodies in the DMH also elicited similar thermogenic and cardiovascular responses, among which the BAT thermogenesis and tachycardia were abolished by blockade of glutamate receptors in the rMR.Citation14 Although ChIEF-containing nerve endings of DMH neurons were also distributed in the periaqueductal gray and the paraventricular hypothalamic nucleus (PVH), photostimulation of these nerve endings did not elicit any of the sympathetic responses.Citation14 These results are consistent with the notion that the stress-activated, glutamatergic monosynaptic neurotransmission from the DMH to sympathetic premotor neurons in the rMR drives the BAT thermogenenic and tachycardic responses to develop PSH ().
Figure 3. Optogenetic stimulation of DMH–rMR projection neurons elicits BAT thermogenesis and cardiovascular responses. (A) In vivo optogenetic experiment to selectively stimulate DMH-derived nerve endings in the rMR. (B and C) Cell bodies transduced with ChIEF-tdTomato (B, inset) in the DMH (mapped with circles) and their nerve endings containing ChIEF-tdTomato in the rMR (C). Scale bars, 500 µm (B); 30 µm (inset); 100 µm (C). (D) Effect of laser illumination of DMH-derived, ChIEF-tdTomato-containing nerve endings in the rMR on BAT thermogenic and cardiovascular activities. Horizontal bar, 30 sec. AP, arterial pressure; BAT SNA, BAT sympathetic nerve activity; HR, heart rate. Modified from Kataoka et al.Citation14 © Elsevier. Permission to reuse must be obtained from the rightsholder.
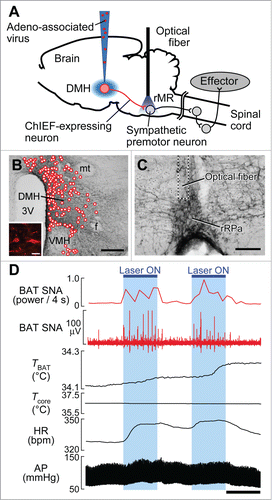
Figure 4. Schematic central circuits for sympathetic and neuroendocrine stress responses. Forebrain stress signals activate 2 groups of DMH neurons: dDMH neurons provide a direct glutamatergic input to sympathetic premotor neurons in the rMR to drive BAT thermogenesis and tachycardia contributing to PSH, and vDMH neurons provide a direct input to the PVH to drive a neuroendocrine outflow through the HPA axis to release stress hormones including glucocorticoids. Plus signs indicate excitatory neurotransmission. IML, intermediolateral nucleus. Modified from Kataoka et al.Citation14 © Elsevier. Permission to reuse must be obtained from the rightsholder.
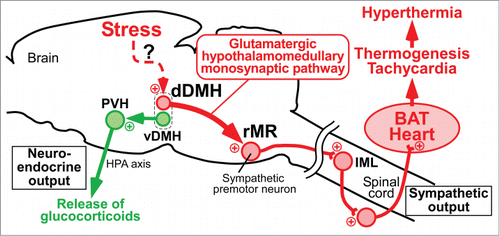
Although the stress-induced pressor response can be inhibited by inactivation of rMR neurons,Citation28,29 blockade of glutamate receptors in the rMR exerts limited inhibitory effects on the pressor responses to social defeat stress and to the photostimulation of DMH neurons.Citation14 These contrasting results suggest that a non-glutamatergic pathway from the DMH to the rMR partly mediates the stress-induced pressor response. Alternatively, in addition to the DMH–rMR pathway, a pathway from the DMH to the cardiovascular sympathetic premotor region, rostral ventrolateral medulla, could partly mediate the pressor response.Citation36
In addition to the DMH–rMR neurons, there is another population of hypothalamomedullary neurons that could be involved in the mechanism of PSH. They are orexin neurons, which are mostly distributed in the perifornical area of the lateral hypothalamusCitation37,38 and provide an axonal projection to the rMR.Citation39,40 Mice and rats with genetic ablation of orexin neurons exhibit attenuated hyperthermic and BAT thermogenic responses to handling stressCitation41 or to psychological stress from a resident-intruder paradigm.Citation42 In support of stress-induced activation of rMR-projecting orexin neurons, handling stress increases Fos expression in orexin neuronsCitation41 and social defeat stress induces Fos expression in some rMR-projecting neurons in the perifornical area, potentially including orexin neurons.Citation14 Injection of orexin into the rMR can increase BAT thermogenesis under slightly cooled conditions in which a basal level of small ongoing BAT sympathetic nerve activity is observed.Citation40 Orexin injection into the rMR also increases heart rate and arterial pressure, but does not elicit cutaneous vasoconstriction,Citation43 suggesting that orexin may selectively activate sympathetic premotor neurons controlling BAT and the heart.
Although these findings all support the involvement of orexin in stress-induced BAT thermogenesis and hyperthermia, inconsistent results have also been reported. Mice lacking orexin gene exhibit intact PSH,Citation41 suggesting a more important role of cotransmitters released from orexin neurons than orexin. Injection of a putative orexin receptor antagonist into the rMR elicits, instead of inhibiting, BAT thermogenesis and an increase in heart rate.Citation40 It should be noted that orexin neurons contribute to many central functions through their projections to diverse areas in the brain and the spinal cord,Citation44,45 and therefore, genetic and pharmacological manipulations of the orexin system can affect a wide range of fundamental physiological functions. For example, genetic ablation of orexin neurons disturbs the basic rest-activity cycles of BAT thermogenesis, body core temperature and feeding behavior,Citation42 making difficulties in the interpretation of the attenuated PSH in such genetically modified animals. Furthermore, the essential role of orexin in the development and functional differentiation of BATCitation46 provides a possibility that the thermoregulatory phenotypes observed in the animals with global genetic modifications of the orexin system are outcomes of a defect in the development of BAT.
The lateral hypothalamus provides fewer stress-activated projections to the rMR than the DMH does, and selective optogenetic stimulation of the nerve endings in the rMR that are derived from lateral hypothalamic neurons including orexin neurons elicits only weak BAT thermogenesis even under cooled conditions.Citation14 Therefore, orexin neurons seem to provide a subsidiary, modulatory signal to the rMR, likely by potentiating the glutamatergic stress signal transmission from the DMH to sympathetic premotor neurons in the rMR. In light of the diverse projections of orexin neurons into forebrain regions,Citation44 it is also possible that they contribute to the development of PSH by affecting the forebrain mechanisms for processing emotion and stress.
The DMH–rMR pathway — not only for PSH
As with PSH, inflammation-induced fever is also a hyperthermic response developed through BAT thermogenesis and cutaneous vasoconstriction associated by increases in heart rate and arterial pressure. A similar set of sympathetic responses is also evoked for the maintenance of body temperature in cold environments. These febrile and cold-defensive sympathetic responses are suppressed by inactivation of neurons in either rMR or DMH,Citation47–52 suggesting that the DMH–rMR neurotransmission drives the thermal and cardiovascular responses for fever and cold defense as well as for PSH. Although there is an exception that inactivation of DMH neurons does not inhibit febrile or spontaneous (temperature dependent) cutaneous vasoconstriction, stimulation of DMH neurons elicits cutaneous vasoconstriction.Citation53 Therefore, DMH neurons might be able to drive the cutaneous vasoconstriction for the development of PSH.
Either pyrogenic stimulation or cold exposure activates VGLUT3-expressing sympathetic premotor neurons in the rMR.Citation22 Cold exposure or lipopolysaccharide-mediated pyrogenic stimulation also activates DMH neurons projecting to the rMR.Citation31,54 Taken together with the thermogenic and cardiovascular responses elicited by the optogenetic stimulation of DMH-derived nerve endings in the rMR (),Citation14 these findings indicate that the DMH–rMR monosynaptic pathway can drive the febrile and cold-defensive sympathetic responses as well as the stress responses, by transmitting excitatory signals to sympathetic premotor neurons in the rMR probably through the VGLUT2-positive glutamatergic synapses.Citation14 In addition to these sympathetic responses, the DMH–rMR monosynaptic pathway may also mediate febrile and cold-defensive shivering in skeletal muscles.Citation55 The basal activity of the DMH–rMR monosynaptic pathway for normal thermoregulation is likely controlled by a tonic GABAergic inhibitory input from the thermoregulatory center, preoptic area (POA).Citation5 The tone of the inhibitory input from the POA to the DMH is thought to be decreased by an action of the pyrogenic mediator, prostaglandin E2 (PGE2) (see below) or by a cool-sensory input from the skin and increased by a warm-sensory input from the skin.Citation5,56,57
DMH–PVH pathway for stress-induced activation of the HPA axis
Psychological stress stimulates the HPA axis through activating a neuroendocrine subset of neurons in the PVH, resulting in enhanced secretion of glucocorticoids from the adrenal cortex, which then causes a variety of alterations in cells throughout the body to defend homeostasis from stressors.Citation20 The brain structures that provide stress-driven inputs to the PVH to stimulate the HPA axis have long been sought.Citation58 DiMicco and colleagues reported that inactivation of neurons in the DMH with muscimol injections reduces air-jet stress-induced ACTH release and Fos expression in the PVH.Citation33,59 These findings indicate that the DMH is involved in the stress signaling to the PVH to drive the neuroendocrine response. Supporting this view, our functional neuroanatomy has revealed that DMH neurons directly projecting to the PVH are activated by social defeat stress (),Citation14 showing a stress-driven direct neurotransmission from the DMH to the PVH. An intriguing observation in this tracing study, which used 2 types of CTb conjugated with different fluorophores to simultaneously trace from the PVH and the rMR, is that these stress-activated, PVH-projecting neurons are clustering in the ventral part of the DMH with no overlap with the stress-activated, rMR-projecting population clustering in the dorsal part of the DMH ().Citation14 Optogenetic stimulation of the DMH–PVH neurotransmission elicits neither BAT thermogenesis nor cardiovascular responses.Citation14 These findings raise the view that the DMH serves as a hub for the central stress signaling by providing the sympathetic efferent through the rMR to drive BAT thermogenesis and tachycardia and by providing the neuroendocrine efferent through the PVH to stimulate the HPA axis (). Curiously, leptin activates PVH-projecting neurons in the ventral part of the DMH,Citation60 suggesting that the stress-activated, PVH-projecting DMH neurons are also involved in the endocrine responses dependent on serum leptin levels reflecting the energy balance.Citation61 Also, a part of these DMH neurons might be those expressing the RFamide prolactin-releasing peptide and involved in thermogenic responses to leptin.Citation62
Difference between PSH and inflammation-induced fever
As mentioned above, the DMH–rMR monosynaptic pathway driving the sympathetic responses for PSH appears to be shared with the development of inflammation-induced fever. However, PSH and fever employ different mechanisms for the activation of the DMH–rMR pathway. The trigger of the central febrile circuit mechanism is an action of the pyrogenic mediator PGE2 on prostaglandin EP3 receptors located on neurons in the POA.Citation5,63-65 PGE2 is produced in endothelial cells of brain blood vessels during infection by the actions of PGE synthesising enzymes including cyclooxygenase-2, whose expression is induced in response to inflammatory cytokine signals from the immune system.Citation66–68 Systemic administration of cyclooxygenase inhibitors suppresses fever induced by lipopolysaccaride or inflammatory cytokines, but has no effect on PSH, Citation13,69-72 and mice lacking EP3 receptors fail to exhibit fever, but show intact PSH.Citation73 On the other hand, anxiolytic drugs including diazepam mitigate PSH but not fever.Citation13,18,72,74 Therefore, PSH does not involve the PGE2–EP3 receptor mechanism, different from inflammation-induced fever, but must be initiated by a mechanism that triggers the activation of the DMH neurons driving the sympathetic and neuroendocrine responses. Consistent with the dissociation of the mechanism of PSH from that of fever, a patient with psychogenic fever was reported to exhibit undetectable plasma levels of pyrogenic cytokines.Citation75
Stress-driven signaling to activate DMH neurons
How stress-driven signals are transmitted to the DMH is still unknown. There are 2 possibilities regarding the brain regions providing stress-driven inputs to the DMH. The first candidates are in the extrahypothalamic structures involved in the stress and emotional signal processing, such as the limbic system and the cerebral cortex. The DMH receives projections from limited extrahypothalamic regions, such as the bed nucleus of the stria terminalis, prefrontal cortex, ventral hippocampus, amygdala and septum.Citation76 Among these regions, the medial prefrontal cortex and the ventral hippocampus are the only sources of glutamatergic excitatory inputs to the DMH, while the other limbic regions provide GABAergic inhibitory projections.Citation77 Social defeat stress increases Fos expression in the medial prefrontal cortex and the ventral hippocampus, which is reduced by diazepam treatment,Citation30 although whether these Fos-expressing neurons project to the DMH is unknown. These anatomical observations leave open the possibility that these regions provide excitatory stress-driven signals to the DMH through the direct projections. However, inactivation of neurons in the medial prefrontal cortex has no inhibitory effect on tachycardic or pressor response to restraint stress.Citation34 On the contrary, inactivation or lesions in the medial prefrontal cortex intensify stress-induced Fos expression in the DMH and PVH.Citation34,78 Therefore, the medial prefrontal cortex might provide a signal to attenuate the cardiovascular stress responses potentially through an indirect pathway(s) to the DMH, although the role of the medial prefrontal cortex in the development of PSH has yet to be studied.
The other possible mechanism for the stress afferent to the DMH is innervations from intrahypothalamic structures. Neurons projecting to the DMH are broadly distributed within the hypothalamusCitation76 and provide both glutamatergic and GABAergic innervations.Citation77 The POA particularly contains many GABAergic neurons projecting to the DMH, including those expressing EP3 receptors.Citation51,79 However, the involvement of POA neurons in PSH has yet to be determined. EP3 receptor-expressing neurons in the POA are proposed to send tonic GABAergic inhibitory signals to the DMH to continuously regulate the activity of the sympathoexcitatory DMH neurons that drive the outflows to BAT and other thermal effectors.Citation5 Whether psychological stress reduces the tone of the GABAergic transmission from the POA to the DMH to elicit PSH is an interesting question awaiting to be addressed.
The anterior hypothalamic area has recently been proposed as a site mediating stress signaling for neuroendocrine responses through the PVH and for behavioral responses potentially through the periaqueductal gray,Citation80 although whether it can also provide a stress-driven input to the DMH is unknown. The anterior hypothalamic area has been shown to receive a GABAergic input from lateral septal neurons expressing the type 2 corticotropin-releasing factor receptor.Citation80 Optogenetic stimulation of this GABAergic transmission promotes, while inhibition attenuates, persistent anxious behaviors as well as circulating corticosteroid levels.Citation80 Social defeat stress induces Fos expression in many neurons of the lateral septum in a diazepam-sensitive manner.Citation30 With these findings, a disinhibiton mechanism of stress responsesCitation80 can be raised: stress-induced activation of the GABAergic transmission from the lateral septum decreases tonic inhibitory transmission from the anterior hypothalamic area to neurons in the PVH and in the periaqueductal gray, whose consequent disinhibition can lead to endocrine and behavioral stress responses. However, whether the anterior hypothalamic area provides tonic inhibition to the PVH and the periaqueductal gray is unknown.
Conclusions and perspectives
In conclusion, psychological stress induces hyperthermia through eliciting sympathetic and neuroendocrine responses including BAT thermogenesis, cutaneous vasoconstriction, tachycardia and glucocorticoid release. In the central circuit mechanism for driving the stress-induced sympathetic responses, the hypothalamomedullary glutamatergic monosynaptic pathway from the dorsal part of the DMH to sympathetic premotor neurons in the rMR plays an important role (). This pathway appears to be shared with the febrile and cold-defensive thermogenic and cardiovascular responses. Orexin neurons can contribute to this descending stress transmission likely through a potentiation of the glutamatergic transmission to sympathetic premotor neurons in the rMR. Another monosynaptic pathway from the ventral part of the DMH to the PVH likely contributes to stress-induced activation of the HPA axis to drive the stress hormone release (). The brain regions antecedent to the DMH in the stress circuit have yet to be identified, and it is an intriguing open question how these brain regions activate the 2 separate neuronal populations in the dorsal and ventral parts of the DMH to drive the sympathetic and neuroendocrine responses, respectively, in the coordinated manner. Many more brain structures than introduced here may be involved in the mechanism of PSH, in light of a recent curious finding that stimulation of the lateral habenula elicits BAT thermogenesis, cutaneous vasoconstriction and cardiovascular responses mimicking stress responses.Citation81 Interestingly, neurons distributed medially within the lateral habenula are activated by social defeat stress in a diazepam-sensitive manner.Citation30
Although the presence of functional BAT in adult humans has been proven,Citation8–11 its contribution to PSH in humans has yet to be determined. In the modern society, psychogenic fever is becoming a major issue in psychosomatic medicine and the knowledge on the mechanism of PSH obtained from animal research may be relevant to the etiology of psychogenic fever in humans.Citation3 Furthermore, a study using an animal model of panic disorder, a severe anxiety disorder characterized by recurrent panic attacks, has shown that NMDA receptor-mediated activation of DMH neurons is responsible for the development of panic-like responses, such as tachycardia, tachypnea, hypertension and increased anxiety.Citation82 The DMH–rMR pathway presented in this review might mediate the sympathetic part of these panic-like responses. Human studies using state-of-the-art technologies for noninvasive imaging, such as fMRI and PET-CT scanning, could bridge the gap between the basic findings from animal research and clinical evidence on psychogenic fever and other psychosomatic symptoms including panic disorder.
Disclosure of Potential Conflicts of Interest
No potential conflicts of interest were disclosed.
Funding
The author was supported by the Funding Program for Next Generation World-Leading Researchers from the Japan Society for the Promotion of Science (LS070), by Grants-in-Aids for Scientific Research (15H05932, 21890114, 22689007, 26118508 and 26713009) and a Special Coordination Fund for Promoting Science and Technology from the Ministry of Education, Culture, Sports, Science and Technology of Japan, and by grants from the Nakajima Foundation, Takeda Science Foundation, Brain Science Foundation, and Kowa Life Science Foundation.
References
- Bishop D. Warm up I: potential mechanisms and the effects of passive warm up on exercise performance. Sports Med 2003; 33:439-54; PMID:12744717; http://dx.doi.org/10.2165/00007256-200333060-00005
- Timmerman RJ, Thompson J, Noordzij HM, van der Meer JW. Psychogenic periodic fever. Neth J Med 1992; 41:158-60; PMID:1470287
- Oka T, Oka K. Mechanisms of psychogenic fever. Adv Neuroimmune Biol 2012; 3:3-17; http://dx.doi.org/10.3233/NIB-2012-011030
- Nozu T, Uehara A. The diagnoses and outcomes of patients complaining of fever without any abnormal findings on diagnostic tests. Intern Med 2005; 44:901-2; PMID:16157998; http://doi.org/10.2169/internalmedicine.44.901
- Nakamura K. Central circuitries for body temperature regulation and fever. Am J Physiol Regul Integr Comp Physiol 2011; 301, R1207-28; PMID:21900642; http://dx.doi.org/10.1152/ajpregu.00109.2011
- Nicholls DG, Locke RM. Thermogenic mechanisms in brown fat. Physiol Rev. 1984; 64:1-64; PMID:6320232
- Rothwell NJ. Eicosanoids, thermogenesis and thermoregulation. Prostaglandins Leukot Essent Fatty Acids 1992; 46:1-7; PMID:1321446; http://dx.doi.org/10.1016/0952-3278(92)90051-J
- van Marken Lichtenbelt WD, Vanhommerig JW, Smulders NM, Drossaerts JMAFL, Kemerink GJ, Bouvy ND, Schrauwen P, Teule GJJ. Cold-activated brown adipose tissue in healthy men. N Engl J Med 2009; 360:1500-8; PMID:19357405; http://dx.doi.org/10.1056/NEJMoa0808718
- Cypess AM, Lehman S, Williams G, Tal I, Rodman D, Goldfine AB, Kuo FC, Palmer EL, Tseng YH, Doria A, Kolodny GM, Kahn CR. Identification and importance of brown adipose tissue in adult humans. N Engl J Med 2009; 360:1509-17; PMID:19357406; http://dx.doi.org/10.1056/NEJMoa0810780
- Virtanen KA, Lidell ME, Orava J, Heglind M, Westergren R, Niemi T, Taittonen M, Laine J, Savisto NJ, Enerbäck S, et al. Functional brown adipose tissue in healthy adults. N Engl J Med 2009; 360:1518-25; PMID:19357407; http://dx.doi.org/10.1056/NEJMoa0808949
- Saito M, Okamatsu-Ogura Y, Matsushita M, Watanabe K, Yoneshiro T, Nio-Kobayashi J, Iwanaga T, Miyagawa M, Kameya T, Nakada K, et al. High incidence of metabolically active brown adipose tissue in healthy adult humans: effects of cold exposure and adiposity. Diabetes 2009; 58:1526-231; PMID:19401428; http://dx.doi.org/10.2337/db09-0530
- Cannon B, Nedergaard J. Brown adipose tissue: function and physiological significance. Physiol Rev 2004; 84:277-359; PMID:14715917; http://dx.doi.org/10.1152/physrev.00015.2003
- Lkhagvasuren B, Nakamura Y, Oka T, Sudo N, Nakamura K. Social defeat stress induces hyperthermia through activation of thermoregulatory sympathetic premotor neurons in the medullary raphe region. Eur J Neurosci 2011; 34:1442-52; PMID:21978215; http://dx.doi.org/10.1111/j.1460-9568.2011.07863.x
- Kataoka N, Hioki H, Kaneko T, Nakamura K. Psychological stress activates a dorsomedial hypothalamus-medullary raphe circuit driving brown adipose tissue thermogenesis and hyperthermia. Cell Metab 2014; 20:346-58; PMID:24981837; http://dx.doi.org/10.1016/j.cmet.2014.05.018
- Ootsuka Y, Blessing WW, Nalivaiko E. Selective blockade of 5-HT2A receptors attenuates the increased temperature response in brown adipose tissue to restraint stress in rats. Stress 2008; 11:125-33; PMID:18311601; http://dx.doi.org/10.1080/10253890701638303
- Yu YH, Blessing WW. Cutaneous vasoconstriction in conscious rabbits during alerting responses detected by hippocampal theta-rhythm. Am J Physiol 1997; 272:R208-16: PMID:9039011
- Vianna DM, Carrive P. Changes in cutaneous and body temperature during and after conditioned fear to context in the rat. Eur J Neurosci 2005; 21:2505-12; PMID:15932607; http://dx.doi.org/10.1111/j.1460-9568.2005.04073.x
- Zethof TJJ, Van der Heyden JAM, Tolboom JTBM, Olivier B. Stress-induced hyperthermia as a putative anxiety model. Eur J Pharmacol 1995; 294:125-35; PMID:8788424; http://dx.doi.org/10.1016/0014-2999(95)00520-X
- Morrison SF, Nakamura K. Central neural pathways for thermoregulation. Front Biosci (Landmark Ed) 2011; 16:74-104; PMID:21196160; http://dx.doi.org/10.2741/3677
- Charmandari E, Tsigos C, Chrousos G. Endocrinology of the stress response. Annu Rev Physiol 2005; 67:259-84; PMID:15709959; http://dx.doi.org/10.1146/annurev.physiol.67.040403.120816
- Silva JE. Thermogenic mechanisms and their hormonal regulation. Physiol Rev 2006; 86:435-64; PMID:16601266; http://dx.doi.org/10.1152/physrev.00009.2005
- Nakamura K, Matsumura K, Hübschle T, Nakamura Y, Hioki H, Fujiyama F, Boldogköi Z, König M, Thiel H-J, Gerstberger R, et al. Identification of sympathetic premotor neurons in medullary raphe regions mediating fever and other thermoregulatory functions. J Neurosci 2004; 24:5370-80; PMID:15190110; http://dx.doi.org/10.1523/JNEUROSCI.1219-04.2004
- Nakamura K, Matsumura K, Kobayashi S, Kaneko T. Sympathetic premotor neurons mediating thermoregulatory functions. Neurosci Res 2005; 51:1-8; PMID:15596234; http://dx.doi.org/10.1016/j.neures.2004.09.007
- Stornetta RL, Rosin DL, Simmons JR, McQuiston TJ, Vujovic N, Weston MC, Guyenet PG. Coexpression of vesicular glutamate transporter-3 and gamma-aminobutyric acidergic markers in rat rostral medullary raphe and intermediolateral cell column. J Comp Neurol 2005; 492:477-94; PMID:16228993; http://dx.doi.org/10.1002/cne.20742
- Björkqvist K. Social defeat as a stressor in humans. Physiol Behav 2001; 73:435-42; PMID:11438372; http://dx.doi.org/10.1016/S0031-9384(01)00490-5
- Morrison SF. RVLM and raphe differentially regulate sympathetic outflows to splanchnic and brown adipose tissue. Am J Physiol Regul Integr Comp Physiol 1999; 276:R962-73; PMID:10198373
- Blessing WW, Nalivaiko E. Raphe magnus/pallidus neurons regulate tail but not mesenteric arterial blood flow in rats. Neuroscience 2001; 105:923-9; PMID:11530230; http://dx.doi.org/10.1016/S0306-4522(01)00251-2
- Zaretsky DV, Zaretskaia MV, Samuels BC, Cluxton LK, DiMicco JA. Microinjection of muscimol into raphe pallidus suppresses tachycardia associated with air stress in conscious rats. J Physiol 2003; 546, 243-50; PMID:12509492; http://dx.doi.org/10.1113/jphysiol.2002.032201
- Pham-Le NM, Cockburn C, Nowell K, Brown J. Activation of GABAA or 5HT1A receptors in the raphé pallidus abolish the cardiovascular responses to exogenous stress in conscious rats. Brain Res Bull 2011; 86, 360-6; PMID:21982918; http://dx.doi.org/10.1016/j.brainresbull.2011.09.018
- Lkhagvasuren B, Oka T, Nakamura Y, Hayashi H, Sudo N, Nakamura K. Distribution of Fos-immunoreactive cells in rat forebrain and midbrain following social defeat stress and diazepam treatment. Neuroscience 2014; 272:34-57; PMID:24797330; http://dx.doi.org/10.1016/j.neuroscience.2014.04.047
- Sarkar S, Zaretskaia MV, Zaretsky DV, Moreno M, DiMicco JA. Stress- and lipopolysaccharide-induced c-fos expression and nNOS in hypothalamic neurons projecting to medullary raphe in rats: a triple immunofluorescent labeling study. Eur J Neurosci 2007; 26:2228-38; PMID:17927775; http://dx.doi.org/10.1111/j.1460-9568.2007.05843.x
- Stotz-Potter EH, Willis LR, DiMicco JA. Muscimol acts in dorsomedial but not paraventricular hypothalamic nucleus to suppress cardiovascular effects of stress. J Neurosci 1996; 16:1173-9; PMID:8558246
- Stotz-Potter EH, Morin SM, DiMicco JA. Effect of microinjection of muscimol into the dorsomedial or paraventricular hypothalamic nucleus on air stress-induced neuroendocrine and cardiovascular changes in rats. Brain Res 1996; 742:219-24; PMID:9117398; http://dx.doi.org/10.1016/S0006-8993(96)01011-6
- McDougall SJ, Widdop RE, Lawrence AJ. Medial prefrontal cortical integration of psychological stress in rats. Eur J Neurosci 2004; 20:2430-40; PMID:15525283; http://dx.doi.org/10.1111/j.1460-9568.2004.03707.x
- Lin JY, Lin MZ, Steinbach P, Tsien RY. (2009). Characterization of engineered channelrhodopsin variants with improved propertiesand kinetics. Biophys J 2009; 96:1803-14; PMID:19254539; http://dx.doi.org/10.1016/j.bpj.2008.11.034
- Horiuchi J, McAllen RM, Allen AM, Killinger S, Fontes MAP, Dampney RAL. Descending vasomotor pathways from the dorsomedial hypothalamic nucleus: role of medullary raphe and RVLM. Am J Physiol Regul Integr Comp Physiol 2004; 287, R824-32; PMID:15205184; http://dx.doi.org/10.1152/ajpregu.00221.2004
- de Lecea L, Kilduff TS, Peyron C, Gao XB, Foye PE, Danielson PE, Fukuhara C, Battenberg ELF, Gautvik VT, Bartlett FS 2nd, et al. The hypocretins: hypothalamus-specific peptides with neuroexcitatory activity. Proc Natl Acad Sci USA 1998; 95:322-7; PMID:9419374
- Sakurai T, Amemiya A, Ishii M, Matsuzaki I, Chemelli RM, Tanaka H, Williams SC, Richardson JA, Kozlowski GP, Wilson S, et al. Orexins and orexin receptors: a family of hypothalamic neuropeptides and G protein-coupled receptors that regulate feeding behavior. Cell 1998; 92:573-85; PMID:9491897; http://dx.doi.org/10.1016/S0092-8674(00)80949-6
- Berthoud HR, Patterson LM, Sutton GM, Morrison C, Zheng H. Orexin inputs to caudal raphé neurons involved in thermal, cardiovascular, and gastrointestinal regulation. Histochem Cell Biol 2005; 123:147-56; PMID:15742197; http://dx.doi.org/10.1007/s00418-005-0761-x
- Tupone D, Madden CJ, Cano G, Morrison SF. An orexinergic projection from perifornical hypothalamus to raphe pallidus increases rat brown adipose tissue thermogenesis. J Neurosci 2011; 31:15944-55; PMID:22049437; http://dx.doi.org/10.1523/JNEUROSCI.3909-11.2011
- Zhang W, Sunanaga J, Takahashi Y, Mori T, Sakurai T, Kanmura Y, Kuwaki T. Orexin neurons are indispensable for stress-induced thermogenesis in mice. J Physiol 2010; 588:4117-29; PMID:20807795; http://dx.doi.org/10.1113/jphysiol.2010.195099
- Mohammed M, Ootsuka Y, Yanagisawa M, Blessing W. Reduced brown adipose tissue thermogenesis during environmental interactions in transgenic rats with ataxin-3-mediated ablation of hypothalamic orexin neurons. Am J Physiol Regul Integr Comp Physiol 2014; 307:R978-89; PMID:25324552; http://dx.doi.org/10.1152/ajpregu.00260.2014
- Luong LN, Carrive P. Orexin microinjection in the medullary raphe increases heart rate and arterial pressure but does not reduce tail skin blood flow in the awake rat. Neuroscience 2012; 202:209-17; PMID:22178985; http://dx.doi.org/10.1016/j.neuroscience.2011.11.073
- Peyron C, Tighe DK, van den Pol AN, de Lecea L, Heller HC, Sutcliffe JG, Kilduff TS. Neurons containing hypocretin (orexin) project to multiple neuronal systems. J Neurosci 1998; 18:9996-10015; PMID:9822755
- van den Pol AN. Hypothalamic hypocretin (orexin): robust innervation of the spinal cord. J Neurosci 1999; 19:3171-82; PMID:10191330
- Sellayah D, Bharaj P, Sikder D. Orexin is required for brown adipose tissue development, differentiation, and function. Cell Metab 2011; 14:478-90; http://dx.doi.org/10.1016/j.cmet.2011.08.010; PMID:21982708
- Nakamura K, Matsumura K, Kaneko T, Kobayashi S, Katoh H, Negishi M. The rostral raphe pallidus nucleus mediates pyrogenic transmission from the preoptic area. J Neurosci 2002; 22:4600-10; PMID:12040067
- Morrison SF. Raphe pallidus neurons mediate prostaglandin E2-evoked increases in brown adipose tissue thermogenesis. Neuroscience 2003; 121:17-24; PMID:12946696; http://dx.doi.org/10.1016/S0306-4522(03)00363-4
- Zaretskaia MV, Zaretsky DV, DiMicco JA. Role of the dorsomedial hypothalamus in thermogenesis and tachycardia caused by microinjection of prostaglandin E2 into the preoptic area in anesthetized rats. Neurosci Lett. 2003; 340:1-4; PMID:12648744; http://dx.doi.org/10.1016/S0304-3940(03)00047-8
- Madden CJ, Morrison SF. Excitatory amino acid receptors in the dorsomedial hypothalamus mediate prostaglandin-evoked thermogenesis in brown adipose tissue. Am J Physiol Regul Integr Comp Physiol 2004; 286:R320-5; PMID:14563660; http://dx.doi.org/10.1152/ajpregu.00515.2003
- Nakamura Y, Nakamura K, Matsumura K, Kobayashi S, Kaneko T, Morrison SF. Direct pyrogenic input from prostaglandin EP3 receptor-expressing preoptic neurons to the dorsomedial hypothalamus. Eur J Neurosci 2005; 22:3137-46; PMID:16367780; http://dx.doi.org/10.1111/j.1460-9568.2005.04515.x
- Nakamura K, Morrison SF. Central efferent pathways mediating skin cooling-evoked sympathetic thermogenesis in brown adipose tissue. Am J Physiol Regul Integr Comp Physiol 2007; 292:R127-36; PMID:16931649; http://dx.doi.org/10.1152/ajpregu.00427.2006
- Rathner JA, Madden CJ, Morrison SF. Central pathway for spontaneous and prostaglandin E2-evoked cutaneous vasoconstriction. Am J Physiol Regul Integr Comp Physiol 2008; 295:R343-54; PMID:18463193; http://dx.doi.org/10.1152/ajpregu.00115.2008
- Yoshida K, Li X, Cano G, Lazarus M, Saper CB. Parallel preoptic pathways for thermoregulation. J Neurosci 2009; 29:11954-64; PMID:19776281; http://dx.doi.org/10.1523/JNEUROSCI.2643-09.2009
- Nakamura K, Morrison SF. Central efferent pathways for cold-defensive and febrile shivering. J Physiol 2011; 589:3641-58; PMID:21610139; http://dx.doi.org/10.1113/jphysiol.2011.210047
- Nakamura K, Morrison SF. A thermosensory pathway that controls body temperature. Nat Neurosci 2008; 11 :62-71; PMID:18084288; http://dx.doi.org/10.1038/nn2027
- Nakamura K, Morrison SF. A thermosensory pathway mediating heat-defense responses. Proc Natl Acad Sci U S A 2010; 107:8848-53; PMID:20421477; http://dx.doi.org/10.1073/pnas.0913358107
- Herman JP, Cullinan WE. Neurocircuitry of stress: central control of the hypothalamo-pituitary-adrenocortical axis. Trends Neurosci 1997; 20:78-84; PMID:9023876; http://dx.doi.org/10.1016/S0166-2236(96)10069-2
- Morin SM, Stotz-Potter EH, DiMicco JA. Injection of muscimol in dorsomedial hypothalamus and stress-induced Fos expression in paraventricular nucleus. Am J Physiol Regul Integr Comp Physiol 2001; 280:R1276-84; PMID:11294744
- Elmquist JK, Ahima RS, Elias CF, Flier JS, Saper CB. Leptin activates distinct projections from the dorsomedial and ventromedial hypothalamic nuclei. Proc Natl Acad Sci USA 1998; 95:741-6; PMID:9435263
- Ahima RS, Prabakaran D, Mantzoros C, Qu D, Lowell B, Maratos-Flier E, Flier JS. Role of leptin in the neuroendocrine response to fasting. Nature 1996; 382:250-2; PMID:8717038; http://dx.doi.org/10.1038/382250a0
- Dodd GT, Worth AA, Nunn N, Korpal AK, Bechtold DA, Allison MB, Myers MG Jr, Statnick MA, Luckman SM. The thermogenic effect of leptin is dependent on a distinct population of prolactin-releasing peptide neurons in the dorsomedial hypothalamus. Cell Metab 2014; 20:639-49; PMID:25176149; http://dx.doi.org/10.1016/j.cmet.2014.07.022
- Nakamura K, Kaneko T, Yamashita Y, Hasegawa H, Katoh H, Ichikawa A, Negishi M. Immunocytochemical localization of prostaglandin EP3 receptor in the rat hypothalamus. Neurosci Lett 1999; 260:117-20; PMID:10025713; http://dx.doi.org/10.1016/S0304-3940(98)00962-8
- Nakamura K, Kaneko T, Yamashita Y, Hasegawa H, Katoh H, Negishi M. Immunohistochemical localization of prostaglandin EP3 receptor in the rat nervous system. J Comp Neurol 2000; 421:543-69; PMID:10842213; http://dx.doi.org/10.1002/(SICI)1096-9861(20000612)421: 4<543::AID-CNE6>3.0.CO;2-3
- Lazarus M, Yoshida K, Coppari R, Bass CE, Mochizuki T, Lowell BB, Saper CB. EP3 prostaglandin receptors in the median preoptic nucleus are critical for fever responses. Nat Neurosci 2007; 10:1131-1133; PMID:17676060; http://dx.doi.org/10.1038/nn1949
- Matsumura K, Cao C, Ozaki M, Morii H, Nakadate K, Watanabe Y. Brain endothelial cells express cyclooxygenase-2 during lipopolysaccharide-induced fever: light and electron microscopic immunocytochemical studies. J Neurosci 1998; 18:6279-89; PMID:9698320
- Yamagata K, Matsumura K, Inoue W, Shiraki T, Suzuki K, Yasuda S, Sugiura H, Cao C, Watanabe Y, Kobayashi S. Coexpression of microsomal-type prostaglandin E synthase with cyclooxygenase-2 in brain endothelial cells of rats during endotoxin-induced fever. J Neurosci 2001; 21:2669-77; PMID:11306620
- Wilhelms DB, Kirilov M, Mirrasekhian E, Eskilsson A, Kugelberg UÖ, Klar C, Ridder DA, Herschman HR, Schwaninger M, Blomqvist A, et al. Deletion of prostaglandin E2 synthesizing enzymes in brain endothelial cells attenuates inflammatory fever. J Neurosci 2014; 34:11684-90; PMID:25164664; http://dx.doi.org/10.1523/JNEUROSCI.1838-14.2014
- Wilkinson MF, Kasting NW. Vasopressin release within the ventral septal area of the rat brain during drug-induced antipyresis. Am J Physiol 1993; 264:R1133-8; PMID:8322966
- Zampronio AR, Silva CAA, Cunha FQ, Ferreira SH, Pelá IR, Souza GEP. Indomethacin blocks the febrile response induced by interleukin-8 in rabbits. Am J Physiol 1995; 269:R1469-74; PMID:8594951
- Steiner AA, Branco LGS. Central CO-heme oxygenase pathway raises body temperature by a prostaglandin-independent way. J Appl Physiol 2000; 88:1607-13; PMID:10797120
- Vinkers CH, Groenink L, van Bogaert MJV, Westphal KGC, Kalkman CJ, van Oorschot R, Oosting RS, Olivier B, Korte SM. Stress-induced hyperthermia and infection-induced fever: two of a kind? Physiol Behav 2009; 98:37-43; PMID:19375439; http://dx.doi.org/10.1016/j.physbeh.2009.04.004
- Oka T, Oka K, Kobayashi T, Sugimoto Y, Ichikawa A, Ushikubi F, Narumiya S, Saper CB. Characteristics of thermoregulatory and febrile responses in mice deficient in prostaglandin EP1 and EP3 receptors. J Physiol 2003; 551:945-54; PMID:12837930; http://dx.doi.org/10.1111/j.1469-7793.2003.00945.x
- Olivier B, Zethof T, Pattij T, van Boogaert M, van Oorschot R, Leahy C, Oosting R, Bouwknecht A, Veening J, van der Gugten J, Groenink L. Stress-induced hyperthermia and anxiety: pharmacological validation. Eur J Pharmacol 2003; 463:117-32; PMID:12600705; http://dx.doi.org/10.1016/S0014-2999(03)01326-8
- Hiramoto T, Oka T, Yoshihara K, Kubo C. Pyrogenic cytokines did not mediate a stress interview-induced hyperthermic response in a patient with psychogenic fever: a case report. Psychosom Med 2009; 71:932-6; PMID:19875636; http://dx.doi.org/10.1097/PSY.0b013e3181bfb02b
- Thompson RH, Swanson LW. Organization of inputs to the dorsomedial nucleus of the hypothalamus: a reexamination with Fluorogold and PHAL in the rat. Brain Res Brain Res Rev 1998; 27:89-118; PMID:9622601; http://dx.doi.org/10.1016/S0165-0173(98)00010-1
- Myers B, Mark Dolgas C, Kasckow J, Cullinan WE, Herman JP. Central stress-integrative circuits: forebrain glutamatergic and GABAergic projections to the dorsomedial hypothalamus, medial preoptic area, and bed nucleus of the stria terminalis. Brain Struct Funct 2014; 219:1287-303; PMID:23661182; http://dx.doi.org/10.1007/s00429-013-0566-y
- Gerrits M, Westenbroek C, Fokkema DS, Jongsma ME, Den Boer JA, Ter Horst GJ. Increased stress vulnerability after a prefrontal cortex lesion in female rats. Brain Res Bull 2003; 61:627-35; PMID:14519460; http://dx.doi.org/10.1016/j.brainresbull.2003.08.004
- Nakamura Y, Nakamura K, Morrison SF. Different populations of prostaglandin EP3 receptor-expressing preoptic neurons project to two fever-mediating sympathoexcitatory brain regions. Neuroscience 2009; 161:614-20; PMID:19327390; http://dx.doi.org/10.1016/j.neuroscience.2009.03.041
- Anthony TE, Dee N, Bernard A, Lerchner W, Heintz N, Anderson DJ. Control of stress-induced persistent anxiety by an extra-amygdala septohypothalamic circuit. Cell 2014; 156:522-36; PMID:24485458; http://dx.doi.org/10.1016/j.cell.2013.12.040
- Ootsuka Y, Mohammed M. Activation of the habenula complex evokes autonomic physiological responses similar to those associated with emotional stress. Physiol Rep 2015; 3:e12297; PMID:25677551; http://dx.doi.org/10.14814/phy2.12297
- Johnson PL, Shekhar A. Panic-prone state induced in rats with GABA dysfunction in the dorsomedial hypothalamus is mediated by NMDA receptors. J Neurosci 2006; 26:7093-104; PMID:16807338; http://dx.doi.org/10.1523/JNEUROSCI.0408-06.2006