Abstract
The birds inhabiting the high-altitude mountains have several physiological adaptations to environmental conditions. In this study, we try to investigate if are the morphological changes in erythrocytes caused by adaptation to a different condition in Eurasian mountain ranges and molt period. In a comparison of morphology of erythrocytes in alpine accentors from three different regions (Central Tian Shan (Kyrgyzstan), the Rila Mountains (Bulgaria) and the High Tatras (Slovakia)) variations were found in size and shape. Birds from the High Tatras had the largest and most elliptical cells, while erythrocytes from Tian Shan´s birds have the smallest and roundest shape. We also found that individuals from spatially separated populations of alpine accentors vary in size. The largest birds are found in the High Tatras, with smaller birds in the Rila Mountains and the smallest in the Tian Shan. Changes in erythrocyte morphology were studied in birds during the molt period (September–October). The cells elongate during the molt and do not reach their pre-molt size even after the molt. These morphology differences likely reflect their adaptation to environmental conditions such as climate and temperature as well as individual metabolic processes during the molt and preparation for autumn migration. In addition to their essential function (respiratory gas transport), nuclear erythrocytes can also participate in the transcription and translation of proteins, extending their other functions. Thus, it seems that changes in morphology must not affect these functions.
Introduction
Environmental conditions in high mountain habitats cause adaptations in behaviour, morphology and physiology of living organisms. Physical features of the high montane environment (hypoxia, cold, intense solar radiation, low water vapour pressure, increased winds, etc.) vary with altitude as a secondary consequence of the variation in barometric pressure (Carey Citation1994) and the macroclimate in high mountains becomes progressively colder and windier with elevation (Mani Citation1990). The synergistic effect of these may result in an increased demand for organisms and leads to strong selective abiotic forces on organisms (Landmann & Winding Citation1995). Notwithstanding these severe environmental conditions, some bird species breed successfully in high alpine areas up to approximately 6500 m a.s.l. in the Himalayas (Rahn Citation1977) but migrate to lower elevations or further south during winter (Barve et al. Citation2016). Low atmospheric pressure and high radiation increase energy demands in birds, including the energy requirements for powered flight and the rate of dehydration (Altshuler & Dudley Citation2006). The time period that birds spend at higher elevations varies among species, depending on their life strategy or environmental conditions, cold tolerance in winter, food availability and the predation risk of particular species and regions (Carey & Morton Citation1976; Boyle Citation2008; Boyle et al. Citation2015). The physiological adaptation to high altitude in mammals and birds includes a high O2-hemoglobin affinity, a moderate or absent polycythemic response, low venous partial pressure of oxygen, and an absence of chronic mountain sickness (Monge & Leon-Velarde Citation1991). The transmission of respiratory gases by blood is closely related to the features of blood, which directly reflects external factors affecting the organism. Fair et al. (Citation2007) states that variation in hematocrit (Hct) may be attributable to factors such as age, sex, reproductive status and environment. Carey and Morton (Citation1976) found a trend of increased Hct at a higher elevation in a variety of wild bird species. In an experimental study of zebra finches (Taeniopygia guttata), hemoglobin (Hg), Hct and erythrocyte counts were on average significantly lower in birds acclimated to cold (Niedojadlo et al. Citation2018). Studies comparing the body size of individuals of identical species or close relatives of this species confirm that the weight of individuals increases with increased altitude (Dunson Citation1965; Carey & Morton Citation1976; Weinstein et al. Citation1985; Rodríguez-Cajarville et al. Citation2019). The results of the study by May et al. (Citation1971), however, point to a lower weight of chicks at low environmental temperatures. Thus, the environmental temperature seems to be an important factor influencing the weight of individuals.
The assumption of survival at high altitudes includes the proper allocation of time and energy among the most important cycles: migration, reproduction itself and molt, which are sharply partitioned temporally throughout the annual cycle (Morton et al. Citation1969). Feathers as a “dead“ structure become damaged and break down quickly from physical wear and exposure to the sun’s radiation and must be completely replaced every year. In many bird species molting begins after breeding, because both are processes have a high energy demand (Jenni & Winkler Citation1994; Newton & Rothery Citation2005). Reproductive hormones, such as testosterone, have an inhibitory effect on molt (Schleussner et al. Citation1985; Nolan et al. Citation1992; Dawson Citation1994, Citation2004). Molt tends to be completed before autumn migration starts (Pulido & Coppack Citation2004). Consequently, in individuals that breed late, raise more broods or breed at higher latitudes, molt progresses more rapidly (Hall & Fransson Citation2001). The endocrine changes that occur at molt begin with rising prolactin levels, inhibiting hypothalamic release of gonadotropin-releasing hormone and release of luteinizing hormone from the pituitary (Tabibzadeh et al. Citation1995). This rise in prolactin may also inhibit ovarian steroidogenesis (Rozenboim et al. Citation1993), leading to regression of the oviduct. Some studies mention the timing of molt is related to the time of peak prolactin concentrations, or when prolactin started to decrease and birds entered reproductive quiescence (Dawson & Goldsmith Citation1982, Citation1983; Dawson Citation2006, Citation2007). The changes that occurred in hematological and blood chemistry parameters of birds during molting were examined in studies by Brake and Thaxton (Citation1979), Brake et al. (Citation1979), Driver (Citation1981), and Dawson and Bortolotti (Citation1997). The most significant change occurs in Hct levels. During postnuptial molt, documented decreases in Hct (Chilgren & DeGraw Citation1977; DeGraw et al. Citation1979; Driver Citation1981; DeGraw & Kern Citation1985; Kasprzak et al. Citation2006; Mazzaro et al. Citation2013) were dependant on the number and size of erythrocytes. The process of molting is complex, and with the subsequent recovery from the molt, it may be viewed as a complex physiological constellation, influenced by environmental and nutritional cues, involving endocrine systems, reproductive tissue structure and function, lymphoid structure and immune function (Berry Citation2003).
In our previous study (Janiga et al. Citation2017), we examined the seasonal effects on erythrocyte morphology in alpine accentors (Prunella collaris, Scop. 1769). We found that the size and shape of red blood cells are seasonally very variable parameters and effectively change during a year. We also discussed variations that are conditioned by the high-altitude habitat of this bird species. Changes in erythrocyte morphology also occur upon exposure to the toxicological effects of the environment. With increased lead values in the blood of alpine accentors, shorter erythrocyte lengths were found (Janiga & Haas Citation2019). Morphometric changes in the nucleus and cytoplasm of erythrocytes have also been associated with compensatory mechanisms against the effects of pollutants (ZnO) in studies in birds (de Andrade Vieira et al. Citation2019) and turtles (da Costa Araújo et al. Citation2019; de Oliveira et al. Citation2020).
The aim of this study was to examine variations in erythrocytes from different Eurasian mountain ranges with regard to molting. We assume that changes in the size and shape of erythrocytes are induced by the adaptation of the species to the conditions of the alpine environment, but are also influenced by physiological status of a bird molting.
Materials and methods
Molting
We used data from 29 individuals examined over the years 2001–2006 in the High Tatra Mountains (Slovakia; the West Carpathians). The stage of molt was made by assigning a value: pre-molting (individuals before molt, complete plumage in birds), molting (individual in molt, with dropped or growing new feathers, at least one observed) and post-molting (individual with complete molt), for each individual.
Erythrocytes measurements
We investigated the erythrocyte of three populations of accentors during late summer and autumn living in different regions. The individuals from the first group are from the different localities of the High Tatras (West Carpathians; Slovakia): Kriváň peak (2495 m a.s.l.; N 49.16305556; E 20.00166667), Predné Solisko peak (2093 m a.s.l.; N 49.150079; E 20.037811); Téryho chata hut (2015 m a.s.l., N 49.1901; E 20.19922). The second group of individuals originates from Central Tian Shan Mountains (Kyrghyzia): Ala Archa national park, Ak-Sai camp (3370 m a.s.l.; N 42.53136111; E 74.52491667), and the third group was from Rila Mountains (Bulgaria): Malak Mechi Vrah (2474 m a.s.l., N 42.028826; E 23.428491).
The birds were captured using ornithological claptraps or mist nets and weighed. Blood samples were collected in the field from each individual, by puncturing the vena ulnaris cutanea. A drop of blood was transferred to a glass slide and a thin smear was made. Blood smears were air-dried and stained in the laboratory according to Pappenheim, two-phase staining using May-Grünwald and Giemsa-Romanowsky dyes (Doubek et al. Citation2003). The slides were examined by a microscope under 1000x magnification. For each individual, 50 nondeformed erythrocytes were used. The perimeter, length and width of erythrocytes and their nuclei were measured using LAS (Leica Application Suite; ver. 4.5.0; Leica Microsystems CMS GmbH, Switzerland). We measured cells from 29 individuals from the High Tatras (caught in September and October 2001–2006), eight individuals from Tian Shan (caught in September 2008) and six individuals from Rila (caught in October 2010).
Erythrocytes size and shape definition
In the analysis of morphological variations, it is important to conceive how shape varies with size. If size and shape variables can be defined precisely, then their association can be described. Mosimann (Citation1970; Citation1975a, Citation1975b) proposed a simple direct method of defining size and shape in a way that is geometrically meaningful and that allows the study of their joint statistical distribution. A size variable is any measurement of easily measurable biological characters, the sum of measurements or a combination of variables such as the geometric mean. Shape variables are either ratios or proportions. If the data follow a multivariate lognormal distribution, their logs will jointly follow a multivariate normal distribution, and parametric statistical methods can be used to study the relationship of size and shape (James & McCulloch Citation1985). The goal of size and shape analysis is not to produce uncorrelated size and shape variables, but to define the size and shape precisely and then analyze how they are related.
Bird erythrocytes are elliptical with a central-positioned oval-shaped nucleus (Glomski & Pica Citation2011). The size of erythrocytes and their nuclei is determined by measured values – length (major axis) and width (minor axis). The size also is expressed by the value of the perimeter.
Shape index
Length/width (L/W) ratio
The ratio of length and width values (L/W) expresses the shape of the erythrocyte or nucleus. If the values approach 1, the shape is less elliptical (i.e. value 1 defines the round shape). If the values are greater, the shape is more elliptical.
Index of the quantity of cytoplasm
Perimeter of erythrocyte/perimeter of nucleus ratio (PE/PN)
This index provides information about the quantity of cytoplasm. If the nucleus perimeter is high, the PE/PN ratio decreased and the cell contains a lower quantity of cytoplasm.
Index of cell and nucleus shape synchrony
Shape of cell/shape of nucleus (EL/EW)/(NL/NW)
The ratio expresses the similarity between the shape of the cell and the shape of the nucleus. If the values approach 1, the shape of the cell and its nucleus is equal, if the values are greater, the cell is more elliptical while the nucleus is more rounded, if the values are below 1 the nucleus is more elliptical than the cell.
Statistical analysis
The size and shape variables were reported as mean, SE and 95% confidence limits, and the significance of differences between groups of birds was tested in the One-way ANOVA and Tukey HSD test. For the assumption of homogeneity of variance, the Bartlett’s test was used. When p < 0.05, the data were considered to be significantly different. Statistical analysis was done using Statistica software ver. 12.
Results
Geographic variation in erythrocyte size
Alpine accentors differed in body weight among different mountain ranges. All individuals were in good health conditions at various stages of molt. In autumn, the more northerly distributed birds from the High Tatras were significantly heavier (m = 39.13 g; + - SE 1.5; n females = 11; n males = 18) than birds from Rila (m = 38.4 g; + -SE 1.3; n females = 3; n males = 3) or the lightest birds from Tian Shan (m = 33.45 g; + - SE 0.7; n females = 2; n males = 6). In September, Rila´s bird erythrocytes were significantly smaller than erythrocytes from the Tatra mountains, and in October, the Tian Shan erythrocytes were smaller than the cells from High Tatras (); ). The same applies to the size of the nucleus ()). The ratio between cell size and nucleus size remained more or less equal among the bird groups, but in October it started to significantly vary in the High Tatras; in some birds, the size of the nucleus increased when related to the size of the cell (); ).
Table I. Mean and SE of erythrocyte characters of alpine accentor.
Geographic variation in erythrocyte shape
The most elongated cells were found in birds from the High Tatras from September (); ). Cells became round in October, and they became comparable to the shape of cells from Tian Shan and Rila. This clearly indicates that birds from more arid, southern localities likely breed earlier than birds from the High Tatras. The habitat of alpine accentors in the West Carpathians is at the northern border of its distribution in Eurasia which is likely reflected by the special behaviour of cell shape. The same trend occurs in the shape behaviour of the nucleus (); ). Nuclei shape was more elliptical in September than in October in the High Tatras, and more elliptical in the High Tatras than in the Tian Shan or Rila.
Figure 2. Shape variation in erythrocytes (a), their nuclei (b) and index of cell and nucleus shape synchrony (c) in alpine accentor erythrocytes in Tian Shan, Rila Mountain and West Carpathians.
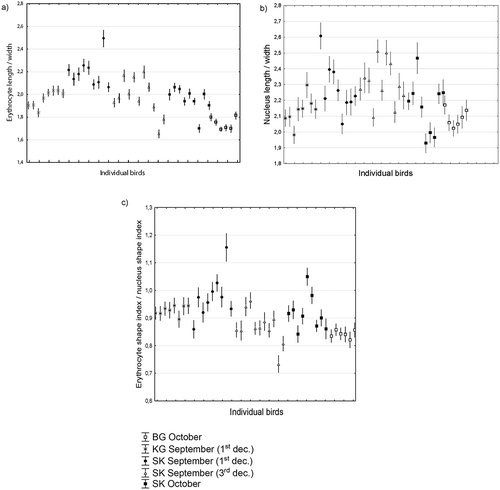
The shape of erythrocytes in alpine accentors changes toward round forms during the end of the molt and the period before the beginning of autumn migration. This phenomenon is observable in each of the studied populations but is separated in time and space. Tian Shan birds have round erythrocytes at the beginning of September, while individuals from the West Carpathians do not acquire this shape until October. This shape was observed in the population from Rila in early October.
The nucleus shape follows the shape of the cell to some extent, particularly in the Tian Shan and Rila bird populations. For Tatra birds of different periods, the nucleus shape is much more variable than the cell shape and reflects the nucleus processes in individual birds.
The shape of the nucleus is the most similar to the shape of the cell in September, while in October the nucleus shape tends to be the most different from the shape of cells (), this variability is probably less geographically dependent, and influenced to a greater extent by the physiological status of an individual bird ()).
Changes in erythrocytes size and shape before molt, during molting and after molt
The size and shape of the erythrocytes and their nuclei varied over the study period. At the end of the nesting period (July–August), the erythrocytes of pre-molt birds are smaller and have a round form. During molting, the erythrocytes change their size and shape. They are big and elliptic (; ). The nuclei behave similarly. In August and September, during molting, the cells and nuclei become more elongated. At the end of molting (October), the cells were more rounded than during molting but nuclei remained narrow, indicating a significant dissimilarity in shape between the cell and nucleus ().
Table II. Mean and SE of erythrocyte characters of alpine accentor in the molting period.
Discussion
Size of individuals from different populations (High Tatras, Rila, Tian Shan) and their erythrocytes
The study of congeneric and conspecific groups of bird species living in different altitudes shows that species living at higher elevations have a larger body size, heavier hearts and higher hematological parameters such as red blood cell count, Hg concentration and Hct (Dunson Citation1965; Carey & Morton Citation1976; Weinstein et al. Citation1985; Rodríguez-Cajarville et al. Citation2019). The increase in body size (which reduces heat loss by reduction of relative body surface – Bergmann’s rule) with increasing altitudes is, therefore, an optimal strategy for energy conservation in high-altitude bird species (Landmann & Winding Citation1995). However, our results show that birds from Tian Shan were smaller in size (m = 33.45 g; n = 8), despite their habitat having the highest altitude. Our finding is also opposite with the results of the study by May et al. (Citation1971), where the weight of birds was higher in warmer environments. This is likely due to the orographic position and climate of the mountains. The West Carpathians constitute the northern boundary of distribution for P. collaris, and during the summer, the High Tatras are colder than the continental Tian Shan or more southerly located Rila Mountains on the Balkan Peninsula.
The size of erythrocytes also behaves similarly. Alpine accentors from the High Tatras have larger erythrocytes than birds from Rila or Tian Shan, in the same time period, and the cells are significantly more elliptic. The size of erythrocytes and other cell types have been compared several times, and the correlation between body mass and cell size has been demonstrated (Gregory Citation2000, Citation2002; Kostelecka-Myrcha & Chołostiakow-Gromek Citation2001; Kozłowski et al. Citation2010). Moreover, in birds, there is a strong correlation between erythrocyte size and metabolic rate, as high metabolism requires smaller cells, which in turn require small DNA contents. This may also play a crucial role in size variations in cells responsible for oxygen supply (Gregory Citation2002). Smaller cells are more metabolically effective during periods of warming but require more energy to maintain and repair (Adrian et al. Citation2016). At a low temperature, where metabolism proceeds slowly, large cells should provide a sufficient area of membranes to transport the metabolites needed to sustain life. Erythrocytes are subject to a rule; when these cells are higher in number, they are smaller in size and have more hemoglobin (Pettingill Citation1985). However, erythrocyte productivity and Hg levels decrease with increasing air temperature (Rehder et al. Citation1982). In more southerly populations of accentors (Rila Mountains, Tian Shan), the average temperatures during summer and early autumn are higher and the climate is drier than in the West Carpathians. While latitude plays a role, so does altitude. Tian Shan populations of alpine accentors live at higher elevations than birds from the High Tatras or Rila Mountains. Blood cell size, therefore, depends more on altitude, and lower air pressure and oxygen content, than on air temperature. However, our data confirm that orography and colder local climates in the High Tatras likely have a crucial effect on erythrocyte morphology because cells from Tian Shan (4000 m a.s.l. breeding area) and Rila (2500 m a.s.l.) differ less than cells from the High Tatras (2000–2500 m a.s.l.).
Our previous study (Janiga et al. Citation2017) showed a trend whereby the adult birds have the largest cells in the winter and the smallest in the summer when they remain at higher elevations during nesting and weather conditions are stable. As the mean or the variance of temperature increases, smaller cells can be efficient in the increased metabolism, especially for oxygen supply (Czarnoleski et al. Citation2013, Citation2015). A large number of cells and a shorter diffusion pathway of smaller erythrocytes should enhance blood oxygen transport efficiency and thus potentially facilitate metabolic acclimatisation in environments of low oxygen availability (Hawkey et al. Citation1991; Breuer et al. Citation1995).
Our results clearly demonstrate changes in the size of erythrocytes and their nuclei in different populations of P. collaris. In the High Tatras, accentor erythrocytes are large and narrow, while smaller and rounder erythrocytes occur in birds living at higher elevations in the Tian Shan and Rila Mountains. The laboratory experiments showed that binding oxygen and flattening and deoxygenation of hemoglobin are highly correlated (Barrett & Scheinberg Citation1972) to the process of erythrocyte transformation in shape from flat to spherical. In low-pressure environments, under hypoxic conditions and with long-term low temperatures, it is probable that a mechanism activating erythrocytes to size and shape changes could evolve.
In highland birds, the alteration of the size of the erythrocyte occurs within the erythroid modifications, i.e. a reduction in the mean volume of the individual circulating red cell, since the percentage of increase in the erythrocyte count was threefold the comparable increment in the Hct (Hawkey et al. Citation1991; Glomski & Pica Citation2011). One of the consequences of acclimatisation to high altitudes is an increase of hemoglobin in the blood. With increasing hemoglobin, the amount of oxygen that can be carried to tissues also increases, but very high concentrations of hemoglobin lead to high blood viscosity. Besides whole blood viscosity, the most important parameter for erythrocyte function is also cytoplasmic viscosity, reflected by mean cellular hemoglobin concentration (MCHC). In birds, the cytoplasmic hemoglobin concentration is higher than in mammals, but Hct is lower and hence blood viscosity is reduced. On the other hand, increasing MCHC may lead to an increase in erythrocyte size resulting in reduced oxygen diffusion to tissues (Monge & Leon-Velarde Citation1991; Canals et al. Citation2007).
The ability of the erythrocytes to deform is influenced by the surface area to volume ratio, cell shape, membrane mechanical properties and their internal viscosity (Chien Citation1987; Baskurt & Meiselman Citation2003). Avian erythrocytes are able to deform in a specific way by folding along their major axis (Gaehtgens et al. Citation1981) and to orient in a shear field. The geometric characteristics of erythrocytes such as shape and membrane surface area to cell volume ratio (S/V ratio) can also affect rheology. However, the ability of the cell to deform depends critically on its S/V ratio as a sphere has the minimum surface area for the contained volume, and thus to deform a sphere it is necessary to enlarge its area (Baskurt & Meiselman Citation2003). This can also be a factor affecting the modification of shape of erythrocytes at high elevations. Blood flow properties change under conditions of increased pressure, low blood flow, heterothermy, water balance and other environmental and physiological stresses that are seen across the vertebrate groups. The size of the blood cells also changes in shape. During the study period, the cells of the High Tatras individuals changed their shape from oval/longitudinal cells to shorter rounder forms. Round form cells are found in Tian Shan individuals as early as September when birds in the High Tatras still have oval erythrocytes.
A suite of physiological adjustments informs erythroid modifications (e.g. increase in hemoglobin, changes in size and shape of erythrocytes), which may also lead to successful exploitation of montane habitats. Hemoglobin concentration does not correlate to high elevations in residents, these species exhibit higher mean cellular hemoglobin concentration (MCHC) than elevational migrants (Barve et al. Citation2016). Species that reside at high elevations regulate hemoglobin independent of increases in Hct and increase blood oxygen-carrying capacity without the concomitant increases in blood viscosity incurred by excessive erythropoiesis (Barve et al. Citation2016). The gene locus of erythropoiesis is activated by hypoxia-inducible transcription factors and permits response to the local oxygen levels in the peripheral blood; by decreasing Hct and hemoglobin levels, the kidneys begin to sense low oxygen levels (Jelkmann Citation2011).
Changes in the ratio of cytoplasm and nucleus
The widely accepted hypothesis that organisms with larger genomes tend to have larger cells (Horner & Macgregor Citation1983; Gregory Citation2001) is not sufficient to explain changes in the size and shape of the erythrocytes and their nuclei observed as part of this study. The amount of cytoplasm in the cell was derived from the ratio of the cell perimeter to the nucleus (PE/PN). Higher values represent cells with more cytoplasm, i.e. smaller nuclei. Lower values represent cells with less cytoplasm content, i.e. larger nuclei. This ratio is independent of cell size. To find out what causes these changes in the cytoplasm/nucleus ratio, we need to know the principles that affect the size and shape of the nucleus in cells. The nucleus is altered in two ways, by processes related to mitosis or by forces acting in the cytoplasm. These changes in the rigidity of the nucleus could be beneficial for cells that need to squeeze through tight spaces (Webster et al. Citation2009), such as erythrocytes.
In all types of eukaryotic cells, a relatively stable nuclear volume to cell volume ratio is maintained, referred to as a constant “karyoplasmic ratio” (Wilson Citation1925; Cavalier-Smith Citation2005). One of the pilot studies of cell and nucleus size (Conllins Citation1912) declares that the size of the nucleus is dependent, among other things, on the volume of the cytoplasm. Additionally, nuclei exposed to larger volumes of cytoplasm grew bigger than nuclei within less cytoplasm. Some studies documented that in higher eukaryotes, nuclear size (Newport et al. Citation1990; Yang et al. Citation1997) as well as shape (see Gruenbaum et al. Citation2005; Worman & Courvalin Citation2005) is strongly influenced by the nuclear lamina, but the molecular basis for the involvement of lamins in nuclear size and shape is still unclear (Huber & Gerace Citation2007). The ratio of nuclear to cellular volume remains constant throughout the cell cycle, even as cell volume increases (Jorgensen et al. Citation2007; Neumann & Nurse Citation2007). When cell size changes, the size of intracellular structures including the nucleus must be regulated in order to ensure proper physiological cell function (Jevtić & Levy Citation2014). Morphology, function and metabolism of erythrocytes suffer from continuous alterations in conjunction with the cell winding its way through the circulatory system (D´Alessandro Citation2013). Changes in cell size and shape directly affect changes in nucleus size and shape, while still maintaining optimal cell function. From the point of view of the functionality of erythrocytes, their essential characteristic is the exchange of respiratory gases, so it is necessary to maintain the hemoglobin concentration during these changes. However, the most characteristic feature of nonmammalian erythrocytes is the presence of a nucleus which allows them to transcribe and translate proteins and therefore intervene in additional functions different from delivery of oxygen to tissues (Morera et al. Citation2011)
Birds from Tian Shan have cells with higher cytoplasm content at the beginning of September, as do birds from Rila at the beginning of October. Changes in cytoplasmic quantity are visible in High Tatras individuals from September to October. At the beginning of September, the blood cells of the birds have nuclei slightly larger than at the end of the month when they have the smallest in the observed period. The change occurs at the beginning of October when the nuclei become larger and the cell has relatively little cytoplasm. One of the possible explanations is that the architecture of erythrocytes changes as a result of metabolic processes. Pis (Citation2008) observed changes in the size and shape of erythrocytes and their nuclei in erythrocytes of chickens after hatching. These changes were especially visible in the first days following hatching, which could reflect metabolic processes in the body.
Changes in the shape and size of erythrocytes before, during and after the molt in alpine accentors
Alpine accentors molt after breeding once per year. During a period of several weeks, the change in the size and shape of erythrocytes is evident (). In our research, we found the larger erythrocytes in molting birds, which does not support the hypothesis that cells are smaller during periods of increased metabolism. In the molt phase, which is an energetically-demanding process and increases metabolic rate (Cyr et al. Citation2008), the organism is exposed to acute stress. Molt is associated with the most energy – and nutrient – demanding periods in the annual cycle of birds (Murphy Citation1996), when the minimum resting metabolic rate (RMRmin) is significant increased (Buttemer et al. Citation2019). The physiological mechanism of molting is very complex, requiring substantial stores of nutrients, an increase in the metabolism of protein (Murphy & Taruscio Citation1995; Murphy Citation1996), a loss in body weight, cessation of egg production, involution of reproductive organs (oviducts, ovaries and follicles) and thermoregulatory setback owing to loss of feathers (Harrison et al. Citation1974; Sekimoto et al. Citation1987). During this phase of molting an increase in plasma and blood volume occurs (DeGraw & Kern Citation1985). While Hct, mean corpuscular volume (MCV) and mean corpuscular hemoglobin concentration (MCHC) are higher during the molt season, erythrocyte numbers did not increase significantly until the end of molt (DeGraw & Kern Citation1985; Arora & Vatsalya Citation2011).
When the number of erythrocytes decreases, their size increases, but the ratio of hemoglobin to the total surface area of erythrocytes (TSAE), despite the statistically significant differences in hemoglobin concentrations, remains unchanged (Kostelecka-Myrcha Citation1997; Kostelecka-Myrcha & Chołostiakow-Gromek Citation2001). This constant index results from the adaptation of the TSAE value to Hb concentration and guarantees permanent saturation of Hb with oxygen. The foundation of this mechanism is a negative correlation between the number and size of erythrocytes (Kostelecka-Myrcha & Chołostiakow-Gromek Citation2001; Pis Citation2008).
The erythrocyte surface-to-volume ratio, lower in small erythrocytes, may directly affect gas exchange (Lay & Baldwin Citation1999; Snyder & Sheafor Citation1999), which is related to the demands of metabolism. The “Cell Metabolism Hypothesis” (Davison Citation1955; Kozłowski et al. Citation2003) predicts that larger cells are metabolically more frugal, i.e. they have a lower metabolic rate per volume unit, due to their relatively smaller membrane surface to cell volume, which should determine the cell metabolic rate (Starostová et al. Citation2013). However, in our research, we found the largest erythrocytes occurred during the molting period, and a similar trend was observed in erythrocytes of alpine accentors in winter (Janiga et al. Citation2017). A possible explanation is given by an interpretation of a study from Buttemer et al. (Citation2019), wherein cells remain less active despite increased metabolism of an organism such as during the molt period. Their study noted that, during the molt, the basal metabolic rate increases (BMR), but aerobic scope is substantially reduced. A study on Zonotrichia leucophrys oriantha (Morton Citation1994), which examined Hct values in relation to reproductive schedule in ecologically similar species (breeding at high elevation) confirms that both sexes have the lowest Hct values during mid- and late-molt. Blood oxygen-carrying capacity is dependent on Hct, Hg concentration and oxygen affinity (Harrison & Harrison Citation1986). It is widely assumed that Hct is positively related to aerobic capacity (e.g. Ekblom Citation2000; Hammond et al. Citation2000). Yap et al. (Citation2018) confirmed that birds exercising at high altitudes showed decreased Hct, increased glucose mobilization and decreased antioxidant capacity, regardless of treatment. This could be a key consequence of the physiological adaptation in the conditions of high altitudes. While metabolism increased during molt, aerobic scope and thus Hct was decreased, which ultimately results in the increasing size of erythrocytes.
The changes in the size, volume and shape of erythrocytes during molt reflect the actual physiological demands of the organism. The variability in erythrocyte size, limited by their nuclei size and shape, is decisive for tissue perfusion, specifically for the distribution of oxygen. This morphology of erythrocytes may determine the architecture of the circulatory system, namely capillary diameter. This diameter affects all tissues and thus the total metabolic rate of an organism (Snyder & Sheafor Citation1999; Starostová et al. Citation2013), which has been found to significantly increase in wild birds undergoing molt (Kuenzel Citation2003). The structure of an erythrocyte affects its morphology, properties and consequently contributes to the ability for gas transfer and blood rheology.
Acknowledgements
We would like to thank to Dr. Martin Lukáň, Dr. Petar Shurulinkov, Peter Macuľa, Ivan Hanula, Martina Júnová, Elena Markuseková, Veronika Justová and Filip Faith, for help in the feldwork during traping the birds.
Disclosure statement
No potential conflict of interest was reported by the authors.
Additional information
Funding
References
- Adrian GJ, Czarnoleski M, Angilletta Jr MJ. 2016. Flies evolved small bodies and cells at high or fluctuating temperatures. Ecology and Evolution 6:7991–7996. DOI: 10.1002/ece3.2534.
- Altshuler DL, Dudley R. 2006. The physiology and biomechanics of avian flight at high altitude. Integrative and Comparative Biology 46:62–71. DOI: 10.1093/icb/icj008.
- Arora KL, Vatsalya V. 2011. Deleterious effects of molting on the morpho-physiology of Japanese quail layers (Coturnix japonica). International Journal of Poultry Science 10:120–124. DOI: 10.3923/ijps.2011.120.124.
- Barrett LA, Scheinberg SL. 1972. The development of avian red cell shape. Journal of Experimental Zoology 182:1–13. DOI: 10.1002/jez.1401820102.
- Barve S, Dhondt AA, Mathur VB, Cheviron ZA. 2016. Life-history characteristics influence physiological strategies to cope with hypoxia in Himalayan birds. Proceedings of the Royal Society B 283:2016–2201. DOI: 10.1098/rspb.2016.2201.
- Baskurt OK, Meiselman HJ 2003. Blood rheology and hemodynamics. Seminars in Thrombosis and Hemostasis 29. pp. 435–450. DOI: 10.1055/s-2003-44551.
- Berry WD. 2003. The physiology of induced molting. Poultry Science 82:971–980. DOI: 10.1093/ps/82.6.971.
- Boyle WA. 2008. Can variation in risk of nest predation explain altitudinal migration in tropical birds? Oecologia 155:397–403. DOI: 10.1007/s00442-007-0897-6.
- Boyle WA, Sandercock BK, Martin K. 2015. Patterns and drivers of intraspecific variation in avian life history along elevational gradients: A meta-analysis. Biological Reviews 91:469–482. DOI: 10.1111/brv.12180.
- Brake J, Thaxton P. 1979. Physiological changes in caged layers during a forced molt. 2. Gross changes in organs. Poultry Science 58:707–716. DOI: 10.3382/ps.0580707.
- Brake J, Thaxton P, Benton EH. 1979. Physiological changes in caged layers during a forced molt. 3. Plasma thyroxin, plasma triiodothyronine, adrenal cholesterol and total adrenal steroids. Poultry Science 58:1345–1350. DOI: 10.3382/ps.0581345.
- Breuer K, Lill A, Baldwin J. 1995. Hematological and body-mass changes of small passerines overwintering in south-eastern Australia. Australian Journal of Zoology 43:31–38. DOI: 10.1071/ZO9950031.
- Buttemer WA, Bauer S, Emmenegger T, Dimitrov D, Peev S, Hahn S. 2019. Moult-related reduction of aerobic scope in passerine birds. Journal of Comparative Physiology B 189:463–470. DOI: 10.1007/s00360-019-01213-z.
- Canals M, Donoso C, Figueroa D, Sabat P. 2007. Pulmonary hematological parameters, energetic flight demands and their correlation with oxygen diffusion capacity in the lungs. Revista Chilena de Historia Natural 80:275–284. DOI: 10.4067/S0716-078X2007000300002.
- Carey C. 1994. Structural and physiological differences between montane and lowland avian eggs and embryos. Journal of Biosciences 19:429–440. DOI: 10.1007/BF02703179.
- Carey C, Morton ML. 1976. Aspects of circulatory physiology of montane and lowland birds. Comparative Biochemistry and Physiology 54:61–74. DOI: 10.1016/S0300-9629(76)80073-4.
- Cavalier-Smith T. 2005. Economy, speed and size matter: Evolutionary forces driving nuclear genome miniaturization and expansion. Annals of Botany (London) 95:147–175. DOI: 10.1093/aob/mci010.
- Chien S. 1987. Red cell deformability and its relevance to blood flow. Annual Review of Physiology 49:177–192. DOI: 10.1146/annurev.ph.49.030187.001141.
- Chilgren JD, DeGraw WA. 1977. Some blood characteristics of white-crowned sparrows during molt. Auk 94:169–171. DOI: 10.1093/auk/94.1.169.
- Conllins EG. 1912. Cell size and nuclear size. Journal of Experimental Zoology 12:1–98. DOI: 10.1002/jez.1400120102.
- Cyr NE, Wikelski M, Romero LM. 2008. Increased energy expenditure but decreased stress responsiveness during molt. Physiological and Biochemical Zoology 81:452–462. DOI: 10.1086/589547.
- Czarnoleski M, Cooper BS, Kierat J, Angilletta MJ. 2013. Flies developed small bodies and small cells in warm and in thermally fluctuating environments. Journal of Experimental Zoology 216:2896–2901. DOI: 10.1242/jeb.083535.
- Czarnoleski M, Dragosz-Kluska D, Angilletta MJ. 2015. Flies developed smaller cells when temperature fluctuated more frequently. Journal of Thermal Biology 54:106–110. DOI: 10.1016/j.jtherbio.2014.09.010.
- D´Alessandro A 2013. Red blood cell ageing in vivo and in vitro: The integrated omics perspective. PhD Thesis. Ecological and Biological Science, University of Tuscia, Viterbo, Italy. pp. 394.
- da Costa Araújo AP, Lima VS, de Andrade Vieira JE, Mesak C, Malafaia G. 2019. First report on the mutagenicity and cytotoxicity of ZnO nanoparticles in reptiles. Chemosphere 235:556–564. DOI: 10.1016/j.chemosphere.2019.06.164.
- Davison J. 1955. Body weight, cell surface and metabolic rate in anuran Amphibia. Biological Bulletin 109:407–419. DOI: 10.2307/1539173.
- Dawson A. 1994. The effects of daylength and testosterone on the initiation and progress of moult in starlings, Sturnus vulgaris. Ibis 136:335–340. DOI: 10.1111/j.1474-919X.1994.tb01104.x.
- Dawson A. 2004. The effects of delaying the start of moult on the duration of moult, primary feather growth rates and feather mass in common starlings, Sturnus vulgaris. Ibis 146:493–500. DOI: 10.1111/j.1474-919x.2004.00290.x.
- Dawson A. 2006. Control of molt in birds: Association with prolactin and gonadal regression in starlings. General and Comparative Endocrinology 147:314–322. DOI: 10.1016/j.ygcen.2006.02.001.
- Dawson A. 2007. Seasonality in a temperate zone bird can be entrained by near equatorial photoperiods. Proceedings of the Royal Society B 274:721–725. DOI: 10.1098/rspb.2006.0067.
- Dawson A, Goldsmith AR. 1982. Prolactin and gonadotrophin secretion in wild starlings (Sturnus vulgaris) during the annual cycle and in relation to nesting, incubation, and rearing young. General and Comparative Endocrinology 48:213–221. DOI: 10.1016/0016-6480(82)90019-3.
- Dawson A, Goldsmith AR. 1983. Plasma prolactin and gonadotrophins during gonadal development and the onset of photorefractoriness in male and female starlings (Sturnus vulgaris) on artificial photoperiods. Journal of Endocrinology 97:253–260. DOI: 10.1677/joe.0.0970253.
- Dawson RD, Bortolotti GR. 1997. Are avian hematocrits indicative of condition? American kestrels as a model. Journal of Wildlife Management 61:1297–1306. DOI: 10.2307/3802129.
- de Andrade Vieira JE, de Oliveira Ferreira R, Dos Reis Sampaio DM, da Costa Araújo AP, Malafaia G. 2019. An insight on the mutagenicity and cytotoxicity of zinc oxide nanoparticles in Gallus gallus domesticus (Phasianidae). Chemosphere 231:10–19. DOI: 10.1016/j.chemosphere.2019.05.111.
- de Oliveira JSP, Vieira LG, Carvalho WF, de Souza MB, de Lima Rodrigues AS, Simões K, de Melo De Silva D, dos Santos Mendonca J, Luz Hirano LQ, Quagliatto Santos AL, Malafaia G 2020: Mutagenic, genotoxic and morphotoxic potential of different pesticides in the erythrocytes of Podocnemis expansa neonates. Science of The Total Environment 140304. DOI: org/10.1016/j.scitotenv.2020.140304
- DeGraw WA, Kern MD. 1985. Changes in the blood and plasma volume of Harris’ sparrows during postnuptial molt. Comparative Biochemistry and Physiology B 8lA:889–893. DOI: 10.1016/0300-9629(85)90925-9.
- DeGraw WA, Kern MD, King JR. 1979. Seasonal changes in blood composition of captive and free-living White-crowned Sparrows. Journal of Comparative Physiology 129B:151–162. DOI: 10.1007/BF00798180.
- Doubek J, Bouda J, Doubek M, Fürll M, Knotková Z, Pejřilová S, Scheer P, Svobodová Z, Vodička R. 2003. Veterinární hematologie. Brno, Noviko. pp. 124–127.
- Driver EA. 1981. Hematological and blood chemical values of mallard, Anas p. platyrhynchos, drakes before, during and after remige moult. Journal of Wildlife Diseases 17:413–421. DOI: 10.7589/0090-3558-17.3.413.
- Dunson WA. 1965. Adaptation of heart and lung weight to high altitude in the robin. The Condor 67:215–219. DOI: 10.2307/1365399.
- Ekblom BT. 2000. Blood boosting and sport. Baillieres Best Practice and Research Clinical Endocrinology and Metabolism 14:89–98. DOI: 10.1053/beem.2000.0056.
- Fair J, Whitaker S, Pearson B. 2007. Sources of variation in haematocrit in birds. Ibis 149:535–552. DOI: 10.1111/j.1474-919X.2007.00680.x.
- Gaehtgens P, Schmidt F, Will G. 1981. Comparative rheology of nucleated and non-nucleated red blood cells I. Microrheology of avian erythrocytes during capillary flow. Pflügers Archiv European Journal of Physiology 390:278–282. DOI: 10.1007/BF00658276.
- Glomski C, Pica A. 2011. The avian erythrocyte. Its phylogenetic Odyssey. 1st ed. Boca Raton, FL: CRC Press. pp. 113–125.
- Gregory TR. 2000. Nucleotypic effects without nuclei: Genome size and erythrocyte size in mammals. Genome 43:895–901. DOI: 10.1139/g00-069.
- Gregory TR. 2001. Coincidence, coevolution, or causation? DNA content, cell size, and the C-value enigma. Biological Reviews of the Cambridge Philosophical Society 76:65–101. DOI: 10.1017/s1464793100005595.
- Gregory TR. 2002. A bird’s-eye view of the C-value enigma: Genome size, cell size, and metabolic rate in the class Aves. Evolution 56:121–130. DOI: 10.1111/j.0014-3820.2002.tb00854.x.
- Gruenbaum Y, Margalit A, Goldman RD, Shumaker DK, Wilson KL. 2005. The nuclear lamina comes of age. Nature Reviews Molecular Cell Biology 6:21–31. DOI: 10.1038/nrm1550.
- Hall KSS, Fransson T. 2001. Wing moult in relation to autumn migration in adult common whitethroats Sylvia communis communis. Ibis 143:580–586. DOI: 10.1111/j.1474-919X.2001.tb04885.x.
- Hammond KA, Chappell MA, Cardullo RA, Lin R, Johnsen TS. 2000. The mechanistic basis of aerobic performance variation in red junglefowl. Journal of Experimental Zoology 203:2053–2064.
- Harrison GL, Harrison LR. 1986. Clinical avian medicine and surgery. London: W.B. Saunders.
- Harrison PC, Casey JM, Adair RL, Reeve JJ. 1974. Fluctuation of hypothalamic luteinizing releasing hormone and pituitary gonadotropins in laying and non-laying hens. Poultry Science 53:554–559. DOI: 10.3382/ps.0530554.
- Hawkey CM, Bennetts M, Gascoyne C, Hart G, Kirkwood JK. 1991. Erythrocyte size, number and haemoglobin content in vertebrates. British Journal of Haematology 77:392–397. DOI: 10.1111/j.1365-2141.1991.tb08590.x.
- Horner HA, Macgregor HC. 1983. C value and cell volume: Their significance in the evolution and development of amphibians. Journal of Cell Science 63:135–146.
- Huber MD, Gerace L. 2007. The size-wise nucleus: Nuclear volume control in eukaryotes. Journal of Cell Biology 179:583–584. DOI:10.1083/jcb.200710156.
- James FC, McCulloch CE. 1985. Data analysis and the design of experiments in ornithology. In: Johnston R, editor. Current ornithology Vol. 2. Boston, MA: Springer. pp. 1–52. DOI:10.1007/978-1-4613-2385-3_1.
- Janiga M, Haas M. 2019. Alpine accentors as monitors of atmospheric long-range lead and mercury pollution in alpine environments. Environmental Science and Pollution Research 26:2445–2454. DOI: 10.1007/s11356-018-3742-z.
- Janiga M, Haas M, Kufelová M. 2017. Age, sex and seasonal variation in the shape and size of erythrocytes of the alpine accentor, Prunella collaris (Passeriformes: Prunellidae). The European Zoological Journal 84(1):583–590. DOI:10.1080/24750263.2017.1403656.
- Jelkmann W. 2011. Regulation of erythropoietin production. Journal of Physiology 589:1251–1258. DOI: 10.1113/jphysiol.2010.195057.
- Jenni L, Winkler R. 1994. Moult and ageing of European passerines. London: Academic Press. pp. 323.
- Jevtić P, Levy DL. 2014. Mechanisms of nuclear size regulation in model systems and cancer. Advances in Experimental Medicine and Biology 773:537–569. DOI: 10.1007/978-1-4899-8032-8_25.
- Jorgensen P, Edgington NP, Schneider BL, Rupes I, Tyers M, Futcher B. 2007. The size of the nucleus increases as yeast cells grow. Molecular Biology of the Cell 18:3523–3532. DOI: 10.1091/mbc.e06-10-0973.
- Kasprzak M, Hetmański T, Kulczykowska E. 2006. Changes in hematological parameters in free-living pigeons (Columba livia f. urbana) during the breeding cycle. Journal of Ornithology 147:599. DOI: 10.1007/s10336-006-0084-2.
- Kostelecka-Myrcha A. 1997. The ratio of amount of haemoglobin to total surface area of erythrocytes in birds in relation to body mass, age of nestlings and season of the year. Physiological Zoology 70:278–282. DOI: 10.1086/639597.
- Kostelecka-Myrcha A, Chołostiakow-Gromek J. 2001. Body mass dependence of the haemoglobin content to surface area ratio of avian erythrocytes. Acta Ornithologica 36:123–128. DOI: 10.3161/068.036.0204.
- Kozłowski J, Czarnołęski M, François-Krassowska A, Maciak S, Pis T. 2010. Cell size is positively correlated between different tissues in passerine birds and amphibians, but not necessarily in mammals. Biology Letters 6:792–796. DOI: 10.1098/rsbl.2010.0288.
- Kozłowski J, Konarzewski M, Gawelczyk AT. 2003. Cell size as a link between noncoding DNA and metabolic rate scaling. Proceedings of the National Academy of Sciences USA 100:14 080–14 085. DOI: 101073/pnas.2334605100.
- Kuenzel WJ. 2003. Neurobiology of molt in avian species. Poultry Science 82:981–991. DOI: 10.1093/ps/82.6.981.
- Landmann A, Winding N. 1995. Guild organisation and morphology of high-altitude granivorous and insectivorous birds: Convergent evolution in an extreme environment. Oikos 73:237–250. DOI: 10.2307/3545914.
- Lay PA, Baldwin J. 1999. What determines the size of teleost erythrocytes? Correlations with oxygen transport and nuclear volume. Fish Physiology and Biochemistry 20:31–35. DOI: 10.1023/A:1007785202280.
- Mani MS. 1990. Fundamentals of high altitude biology. London: Aspect Pub. pp. 138.
- May JD, Deaton JW, Reece FN, Mitlin N, Kubena LF. 1971. The effect of environmental temperature on blood volume. Poultry Science 50:1867–1870. DOI: 10.3382/ps.0501867.
- Mazzaro LM, Meegan J, Sarran D, Romano TA, Bonato V, Deng S, Dunn JL. 2013. Molt-associated changes in hematologic and plasma biochemical values and stress hormone levels in African penguins (Spheniscus demersus). Journal of Avian Medicine and Surgery 27:285–293. DOI: 10.1647/2012-004.
- Monge C, Leon-Velarde F. 1991. Physiological adaptation to high altitude: Oxygen transport in mammals and birds. Physiological Reviews 71:1135–1172. DOI: 10.1152/physrev.1991.71.4.1135.
- Morera D, Roher N, Ribas L, Balasch JC, Donate C, Callol A, Boltana S, Roberts R, Goetz G, Goetz FW, MacKenzie SA. 2011. RNA-Seq reveals an integrated immune response in nucleated erythrocytes. PloS One 6:10. DOI: 10.1371/journal.pone.0026998.
- Morton ML. 1994. Hematocrits in montane sparrows in relation to reproductive schedule. The Condor 96:119–126. DOI: 10.2307/1369069.
- Morton ML, King JR, Farner DS. 1969. Postnuptial and postjuvenal molt in White-crowned Sparrows in central Alaska. The Condor 71:376–385. DOI: 10.2307/1365736.
- Mosimann JE. 1970. Size allometry: Size and shape variables with characterizations of the lognormal and generalized gamma distributions. Journal of the American Statistical Association 65:930–945. DOI: 10.2307/2284599.
- Mosimann JE. 1975a. Statistical problems of size and shape. I. Bio logical applications and basic theorems. In: Patil GP, Kotz S, Ord K, editors. Statistical distri butions in scientific work 2. Dordrecht-Holland: D. Reidel Publ. Co. pp. 187–217.
- Mosimann JE. 1975b. Statistical problems of size and shape. II. Characterizations of the lognormal and gamma dis tributions. In: Patil GP, Kotz S, Ord K, editors. Statistical distributions in scientific work 2. Dordrecht-Holland: D. Reidel Publ. Co. pp. 219–239.
- Murphy ME. 1996. Energetics and nutrition of molt. In: Carey C, editor. Avian energetics and nutritional ecology. New York: Plenum Press. pp. 158–198.
- Murphy ME, Taruscio TG. 1995. Sparrows increase their rates of tissue and whole-body protein synthesis during the annual molt. Comparative Biochemistry and Physiology A 111:385–396. DOI: 10.1016/0300-9629(95)00039-A.
- Neumann FR, Nurse P. 2007. Nuclear size control in fission yeast. Journal of Cell Biology 179:593–600. DOI: 10.1083/jcb.200708054.
- Newport JW, Wilson KL, Dunphy WG. 1990. A lamin-independent pathway for nuclear envelope assembly. Journal of Cell Biology 111:2247–2259. DOI: 10.1083/jcb.111.6.2247.
- Newton I, Rothery P. 2005. The timing, duration and pattern of moult and its relationship to breeding in a population of the European greenfinch Carduelis chloris. Ibis 147:667–679. DOI: 10.1111/j.1474-919X.2005.00439.x.
- Niedojadlo J, Bury A, Cichoń M, Sadowska ET, Bauchinger U. 2018. Lower haematocrit, haemoglobin and red blood cell number in zebra finches acclimated to cold compared to thermoneutral temperature. Journal of Avian Biology 49:jav–01596. DOI: 10.1111/jav.01596.
- Nolan V, Ketterson ED, Ziegenfus C, Cullen DP, Chandler CR. 1992. Testosterone and avian life Histories - effects of experimentally elevated testosterone on prebasic molt and survival in male dark-eyed juncos. The Condor 94:364–370. DOI: 10.2307/1369209.
- Pettingill OS. 1985. Ornithology in Laboratory and Field. 5th ed. London: Academic Press. pp. 403.
- Pis T. 2008. Resting metabolic rate and erythrocyte morphology in early development of thermoregulation in the precocial grey partridge (Perdix perdix). Comparative Biochemistry and Physiology - Part A: Molecular & Integrative Physiology 151:211–218. DOI: 10.1016/j.cbpa.2008.06.026.
- Pulido F, Coppack T. 2004. Correlation between timing of juvenile moult and onset of migration in the blackcap, Sylvia atricapilla. Animal Behaviour 68:167–173. DOI: 1016/j.anbehav.2003.11.006.
- Rahn H. 1977. Adaptation of the avian embryo to altitude: The role of gas diffusion through the eggshell. In: Paintal AS, Gill-Kumar P, editors. Respiratory adaptations, capillary exchange and reflex mechanism. Delhi: Vallabhbhai Patel Chest Institute, University of Delhi. pp. 94–105.
- Rehder NB, Bird DM, Laguë PC. 1982. Variations in blood packed cell volume of captive American Kestrels. Comp. Comparative Biochemistry and Physiology Part A: Physiology 72:105–109. DOI: 10.1016/0300-9629(82)90017-2.
- Rodríguez-Cajarville MJ, Calderón L, Tubaro PL, Cabanne GS. 2019. Body size and genetic variation in the White-tipped Plantcutter (Phytotoma rutila: Cotingidae) suggest ecological divergence across the Chaco–Andes dry forest belt. Journal of Ornithology 160:947–961. DOI: 10.1007/s10336-019-01694-3.
- Rozenboim I, Tabibzadeh C, Silsby JL, El Halawani ME. 1993. Effect of ovine prolactin administration on hypothalamic vasoactive intestinal peptide (VIP), gonadotropin releasing hormone I and II content, and anterior pituitary VIP receptors in laying turkey hens. Biology of Reproduction 48:1246–1250. DOI: 10.1095/biolreprod48.6.1246.
- Schleussner G, Dittami JP, Gwinner E. 1985. Testosterone implants affect molt in male European starlings, Sturnus vulgaris. Physiological Zoology 58:597–604. DOI:10.1086/physzool.58.5.30158586.
- Sekimoto K, Imai K, Suzuki M, Takikawa H, Hoshino N, Tosuka K. 1987. Thyroxin-induced molting and gonadal function in laying hens. Poultry Science 66:752–756. DOI: 10.3382/ps.0660752.
- Snyder GK, Sheafor BA. 1999. Red blood cells: Centerpiece in the evolution of the vertebrate circulatory system. American Zoologist 39:189–198. DOI: 10.1093/icb/39.2.189.
- Starostová Z, Konarzewski M, Kozłowski J, Kratochvíl L. 2013. Ontogeny of metabolic rate and red blood cell size in eyelid geckos: Species follow different paths. PLoS One 8:5. DOI: 10.1371/journal.pone.0064715.
- Tabibzadeh C, Rozenboim I, Silsby JL, Pitts GR, Foster DN, El Halawani ME. 1995. Modulation of ovarian cytochrome P450 17S-hydroxylase and cytochrome aromatase mRNA by prolactin in the domestic turkey. Biology of Reproduction 52:600–608. DOI: 10.1095/biolreprod52.3.600.
- Webster M, Witkin KL, Cohen-Fix O. 2009. Sizing up the nucleus: Nuclear shape, size and nuclear-envelope assembly. Journal of Cell Science 122:1477–1486. DOI: 10.1242/jcs.037333.
- Weinstein Y, Bernstein MH, Bickler PE, Gonzales DV, Samaniego FC, Escobedo MA. 1985. Blood respiratory properties in pigeons at high altitudes: Effects of acclimation. American Journal of Physiology-Regulatory, Integrative and Comparative Physiology 249:R765–R775. DOI: 10.1152/ajpregu.1985.249.6.R765.
- Wilson EB. 1925. The karyoplasmic ratio. In: The cell in development and heredity. 3th ed. The New York: Macmillan Company. pp. 727–733.
- Worman HJ, Courvalin JC. 2005. Nuclear envelope, nuclear lamina, and inherited disease. International Review of Cytology 246:231–279. DOI: 10.1016/S0074-7696(05)46006-4.
- Yang L, Guan T, Gerace L. 1997. Lamin-binding fragment of LAP2 inhibits increase in nuclear volume during the cell cycle and progression into S phase. Journal of Cell Biology 139:1077–1087. DOI: 10.1083/jcb.139.5.1077.
- Yap KN, Dick MF, Guglielmo CG, Williams TD. 2018. Effects of experimental manipulation of hematocrit on avian flight performance in high-and low-altitude conditions. Journal of Experimental Zooloogy 221:jeb191056.