Abstract
The peach-potato aphid Myzus persicae (Sulzer) and the black bean aphid Aphis fabae Scopoli are polyphagous and cosmopolitan hemipterans, therefore they can infest grapevines in all areas of cultivation. Electrical Penetration Graph (EPG) technique was applied to monitor the probing behavior of A. fabae and M. persicae on Vitis amurensis Rupr., Vitis riparia Michaux, and Vitis vinifera L. The content of major flavonoids and stilbenoids in grapevine leaves and epidermal thickness, distance between abaxial leaf surface and phloem, and the simulated shortest pathway from epidermis to phloem that might have affected aphid probing were also analyzed. Aphid probing was limited mainly to non-vascular tissues on the three studied grapevine species. Phloem phase occurred in 32%, 14%, and 6% of A. fabae and in 76%, 39%, and 74% of M. persicae on V. amurensis, V. riparia and V. vinifera, respectively. Phloem phase consisted of only salivation into sieve elements and lasted less than 2.5 minutes on average in all aphids. The time to reach the first phloem phase on grapevines was 5.0 hours in A. fabae and 2.6–3.6 hours in M. persicae. Of the analyzed flavonoids, catechin, epicatechin, and quercetin occurred in all grapevine species, while rutin – in V. amurensis and V. riparia and isorhamnetin only in V. amurensis. Of the analyzed stilbenoids, piceid occurred in all grapevines, resveratrol in V. amurensis and V. vinifera, and ε-viniferin only in V. vinifera. Aphid behavior demonstrated that V. amurensis, V. riparia and V. vinifera are not attractive host plants to A. fabae and M. persicae. It is likely that the content of flavonoids and stilbenoids contributes to the limited susceptibility of the three grapevine species to A. fabae and M. persicae, while the observed slight differences in the anatomical structure of the leaves seem not significant in this context.
Introduction
Grapevines (Vitaceae Juss). include about 950 species, usually shrubs or lianas, assigned to 16 genera, most of which occur in tropical or subtropical zones (Wen et al. Citation2018). On the other hand, species of the genus Vitis occur mainly in the temperate climate zones of the northern hemisphere – in Europe and Asia (Eurasian species, e.g. Vitis amurensis Rupr.) and North and Central America (American species, e.g. Vitis riparia Michaux). The genus Vitis comprises about 60 species. Among them, one of the most important, especially in economic terms, is Vitis vinifera L., which gave rise to the vast majority of grapevines cultivated today. Grapevine species differ in terms of morphology and preferred habitat, however, they can easily interbreed, creating interspecies hybrids (Keller Citation2015a). The term “hybrid” is used for grapevines that originate from the crossing of Vitis species (e.g. V. vinifera and V. riparia), and the hybrid grape-based wine is called a “hybrid wine” (Burns et al. Citation2002). Many attempts of cross-species breeding have been made to combine the positive qualities of some while eliminating the negative of the other. Hybrid varieties were produced to control diseases (downy mildew, powdery mildew caused by Plasmopara viticola (Berk. & M.A. Curtis) Berl. & De Toni and Erysiphe necator Schwein. In addition, some species are tolerant to environmental factors, such as V. amurensis, which is highly frost resistant (native to cold areas in Northeast China and Russian Siberia) (Kedrina-Okutan et al. Citation2019). Despite attempts to obtain varieties resistant to biotic and abiotic factors at the same time without losing the quality of grapes, grapevines are still a food source for many insect species including Coleoptera, Diptera (Sekrecka et al. Citation2015), Hemiptera (Omer et al. Citation1999; Ocete et al. Citation2008; Myśliwiec Citation2009; Baronio et al. Citation2014), Thysanoptera (Ocete et al. Citation2008), Lepidoptera (Ocete et al. Citation2008; Vogelweith et al. Citation2014), as well as Arachnidae (James & Whitney Citation1993). Among the grapevine-related herbivores, the occurrence of various species of Aphididae has been observed on various grapevine species, especially V. vinifera, e.g., the peach-potato aphid Myzus persicae (Sulzer) and the black bean aphid Aphis fabae Scopoli (Blackman & Eastop Citation2006). The presence of M. persicae and A. fabae on grapevines, including V. vinifera, in various areas of Europe was also indicated by Holman (Citation2009), who referred to research by many authors. The occurrence of M. persicae on vines was reported in Germany, while A. fabae - in Spain, Portugal, France, Serbia, and also outside Europe, in Turkey and Tajikistan (Holman Citation2009). However, considering the polyphagy and the worldwide distribution of A. fabae and M. persicae, grapevines can be infested by these aphid species in all areas of cultivation (CABI Citation2022a, Citation2022b).
Herbivores with sucking-piercing mouthparts, e.g., Coccoidea, Aphididae, Phylloxeridae, due to the way of feeding, can be vectors of various plant viruses, also transmitted to various species of the genus Vitis sp. In the scientific literature, a lot of attention has been paid to the interactions in the grapevine-Phylloxera system, also in terms of defense against viruses (Keller Citation2015b). At the same time, studies on the interactions between grapevines and aphids are scarce. Aphids transmit Alfalfa Mosaic Virus (AMV), Cucumber Mosaic Virus (CMV), Bean Common Mosaic Virus (BCMV), Broad Bean Wilt Virus (BBWV), Grapevine Vein Clearing Virus (GVCV) or Grapevine Enamovirus 1 (GEV1) (Basso et al. Citation2016; Fuchs Citation2020). The Sowbane Mosaic Virus (SoMV) on Vitis sp. is transmitted only by M. persicae (Chan et al. Citation1991). Virus transmission is related to the behavior of aphids during the selection of host plants (transmission of non-persistent and semi-persistent viruses during the test punctures of plant tissues) and to the specificity of aphid feeding (aphids uptake the phloem sap directly from the sieve tubes, which enables the transmission of persistent viruses) (Martin et al. Citation1997). It is therefore important to obtain cultivars resistant to the feeding of the aphids, as they are therefore less likely to be infected by viruses transmitted by these insects. Vitis amurensis, V. riparia and V. vinifera are important basic species in breeding hybrid grapevine varieties (De la Fuente Lloreda Citation2018; Teissedre Citation2018). The probing behavior of aphids on grapevines has not been studied in detail, hitherto.
The resistance of given species or cultivar to the feeding of herbivores may be based on the morphological and anatomical structure of the plant and the presence of allelochemicals (Harborne Citation1997; Powell et al. Citation2006; Stout Citation2013). Secondary metabolites in grapevines are mainly phenolic compounds – flavonoids, phenolic acids, and stilbenoids, as well as phenol derivatives such as tannins (De Rosso et al. Citation2014; Kedrina-Okutan et al. Citation2019). The content of phenolic compounds varies depending on the species and cultivar and is related to the variation in the climatic conditions, weather variability, irrigation, soil or environmental stresses (Petrussa et al. Citation2013). Flavonoids determine the color of plants, the taste of fruits, and are natural repellents for herbivores (Jasiński et al. Citation2009; Majewska & Czeczot Citation2009; Goławska et al. Citation2010, Citation2014; Golonko et al. Citation2015). Stilbenoids are derivatives of stilbene (Makowska-Wąs & Janeczko Citation2008; Jeszka et al. Citation2010). Depending on the species or cultivar, stilbenoids occur in the entire plant or its individual organs: in fruits, leaves, stems, and roots (Pawlus et al. Citation2012). Stilbenoids are involved in allelopathic effects and in the plant’s response to oxidative stress generated by UV radiation, they can also inhibit the growth of bacteria and fungi (disease-resistant species produce stilbenoids in higher concentrations) and show herbivore repellent properties (Chong et al. Citation2009; Pawlus et al. Citation2012; Nopo-Olazabal et al. Citation2014; Kozłowska & Czekała Citation2017). The choice of a plant as a food source may depend also on physical barriers. In the case of aphids, the thickness of the sclerenchyma layer surrounding the vascular bundles, which can constitute a barrier during penetration, may be important: the thicker the sclerenchyma layer, the more difficult it is for the aphids to reach the vascular bundle, especially the phloem from which the aphids take up the sap (Kidd Citation1976; Boczek Citation1988; Malinowski Citation2008; Ammar et al. Citation2014).
The aim of the present study was to assess the susceptibility of selected grapevine species V. amurensis, V. riparia and V. vinifera to M. persicae and A. fabae. We monitored aphid stylet penetration activities in plant tissues with the use of the Electrical Penetration Graph (EPG, known also as electropenetrography) technique, which is crucial in determining the impact of antixenosis factors on individual phases of aphid probing in peripheral as well as in vascular plant tissues. In addition, we analyzed the quantitative and qualitative variation in the content of selected major flavonoids and stilbenoids in the leaves of the grapevine species and we measured leaf anatomical parameters that might have affected aphid probing.
Material and methods
Cultures of plants and aphids
Grapevines
The grapevines used in the experiments were derived from Instituto Nacional de Investigacion y Technologia Agraria y Alimentaria (INIA), Madrid, Spain (Vitis vinifera L.) and Global Flowers Ltd, Rotherham, UK, through Sklep Ogrodniczy Plumeria (V. amurensis Rupr. and V. riparia Michx.). Previous studies showed that some natural populations of V. vinifera ssp. sylvestris in the Iberian Peninsula showed small infestations caused by aphids. Therefore, we have chosen the genotype with assumed tolerance to aphid infestation from Spain. All plants were grown in commercial soil in plastic pots (vol. 4 dm3), in the laboratory at 20°C, 65% r.h., and L16:8D photoperiod. The plants were watered regularly and no fertilizers were applied. For all experiments, 1-2-years old plants were used.
Aphids
Laboratory cultures of Aphis fabae and Myzus persicae were maintained as multiclonal colonies on Vicia faba L. cv. White Windsor and Brassica pekinensis (Lour.) Rupr. cv. Hilton, respectively, in the laboratory at 20°C, 65% r.h., and L16:8D photoperiod in a growing chamber Sanyo MLR-351 H (Sanyo Electronics Co. Ltd.).
Electronic monitoring of aphid probing behavior
Probing behavior of Aphis fabae and Myzus persicae on Vitis amurensis, V. riparia and V. vinifera was monitored using the technique of electronic registration of aphid behavior known as Electrical Penetration Graph (EPG) technique or electropenetrograhy that is frequently employed in insect–plant relationship studies (Will et al. Citation2007; Philippi et al. Citation2015). In the experimental set-up, aphid and plant are made parts of an electric circuit, which is completed when the aphid inserts its stylets into the plant. Weak voltage is supplied in the circuit, and all changing electric properties are recorded as EPG waveforms that can be correlated with aphid activities and stylet position in plant tissues (Tjallingii Citation1994). The parameters describing aphid behavior during probing and feeding, such as total time of probing, proportion of phloem patterns E1 and E2, number of probes, etc., are good indicators of plant suitability or interference of probing by chemical or physical factors in plant tissues (Mayoral et al. Citation1996). Based on previous research on aphid behavior during probing on susceptible and resistant plant species and cultivars (Gabryś & Pawluk Citation1999; Kordan et al. Citation2018, Citation2019, Citation2021; Stec et al. Citation2021), we established the following levels of grapevine susceptibility: (I) susceptible (phloem phase present and contains phloem sap ingestion); (II) relatively susceptible (over 90% of aphids reach the phloem phase but the phloem phase represented only by the E1 waveform related to the secretion of watery saliva); (III) moderately susceptible (40–75% of aphids reach the phloem phase, the phloem phase represented only by the E1 waveform related to the secretion of watery saliva); (IV) relatively resistant (5–35% of aphids reach the phloem phase, the phloem phase represented only by the E1 waveform related to the secretion of watery saliva); (V) resistant (no phloem phase).
In the present study, aphids for EPG experiments were 2–3 days old (2–3 days after the final molt) viviparous apterous A. fabae and M. persicae, which were attached to a golden wire electrode with silver paint and starved for 1 h prior to the experiments. The experiments were repeated 24 times for each species of grapevine and each replicate consisted of a freshly prepared aphid and a plant. Incomplete recordings (i.e., recordings that terminated due to aphid falling from the plant or where EPG signal was unclear) were rejected from analysis, so the final number of replications used for analyses was: A. fabae/V. amurensis, n = 19; A. fabae/V. riparia, n = 21; A. fabae/V. vinifera, n = 18 and M. persicae/V. amurensis, n = 17; M. persicae/V. riparia, n = 18; M. persicae/V. vinifera, n = 19. Probing behavior of aphids was monitored for 8 h continuously and all bioassays started at 10:00–11:00 h MEST (Middle European Summer Time). Giga-8 DC EPG system with a 1 GΩ of input resistance (EPG Systems, Wageningen, The Netherlands) was used to record EPGs. EPGs were recorded and analyzed using Stylet+ Software (EPG Systems, Wageningen, The Netherlands). Signals were saved on the computer and the various behavioral phases were labeled manually using the Stylet+ software.
The following EPG waveform patterns were distinguished: “np” (no penetration, when aphid stylets did not have contact with plant tissues), “ABC” (pathway phase – penetration of parenchyma/mesophyll), “E1e” (salivation into apoplast), “E1” (salivation into sieve elements), “E2” including “E1/E2” transitions (ingestion of phloem sap), “F” (derailed stylet activities representing undetermined difficulties in probing) and “G” (ingestion of xylem sap). The waveform patterns that were not terminated before the end of the experimental period (8 h) were included in the calculations. In sequential parameters, when time to waveforms related to phloem phase (E1 or E2) or xylem phase (G) was calculated, only individuals that showed either of the phases were included in calculations and statistical analysis. In non-sequential parameters, when a given waveform had not been recorded for an individual, the duration of that waveform was given the value of 0.
High-performance liquid chromatography of flavonoids and stilbenoids
The dried grapevine leaves of Vitis amurensis, V. riparia, and V. vinifera (1.2 g of each) were homogenized in an aqueous ethanol solution (80%) using a Diax 900 homogenizer. The resulting suspension was centrifuged (12,000 rpm, 10 min) and the supernatant solution was collected in a graduated flask and the pellet was reconditioned. This operation was repeated three times, and the obtained extracts were combined. The homogenization procedure in combination with the extraction was carried out in such a way that the final volume of the extract was 100 ml. From the prepared ethanol extracts, 10 ml was taken and evaporated to dryness in a rotary evaporator under reduced pressure at 40°C. The dry extracts were dissolved in 100% methanol to a volume of 1 ml. Resulting methanolic extracts containing flavonoid compounds were analyzed by HPLC–ESI-MS/MS.
The contents of flavonoids apigenin, catechin, epicatechin, hesperetin, isorhamnetin, kaempferol, luteolin, naringin, quercetin, rutin, taxifolin and stilbenoids ampelopsin, piceid, resveratrol, ε-viniferin were determined. The selection of the phenolic compounds for analysis was based on literature data that reported major phenolic constituents of vegetative parts of grapevines (Castillo-Munoz et al. Citation2010; Pawlus et al. Citation2012; Portu et al. Citation2015; Biais et al. Citation2017; Aliaño-González et al. Citation2020; Goufo et al. Citation2020; Baroi et al. Citation2022).
Individual pure flavonoids and stilbenoids were purchased from Sigma–Aldrich (Poland). Ethanol, HPLC gradient grade methanol and acetonitrile were supplied by Merck (Germany). Formic acid was purchased from Sigma-Aldrich (Poland). Stock standard solutions of individual phenolic compounds (50 mg/l) were prepared by dissolving appropriate amounts of solid reagents in methanol. Mixed working standard solutions of flavonoid compounds at 20, 10, 5, 2.5 and 1 mg/l concentrations were prepared by appropriate dilutions of stock standard solutions.
The chromatographic analysis was carried out with a Shimadzu LC system, comprising a LC20-AD binary pump, a DGU-20A5 degasser, a CTO-20AC column oven and a SIL-20AC autosampler, connected to a 3200 QTRAP hybrid triple quadrupole (Applied Biosystem, MDS SCIEX, USA) with electrospray ionization source (ESI) operated in negative-ion mode. Phenolic compounds were separated on a Phenomenex Luna C-18 column (100 × 2.0 mm × 3.0 µm) with a pre-column, both maintained at 30°C. A 7.4 mmol/l solution of formic acid (pH 2.8, eluent A) and acetonitrile (eluent B) were used. The mobile phase was delivered at 0.2 ml/min in a linear gradient mode as follows: 0–2 min 10% B, 30 min 60% B, 40 min 100% B, 55 min 10% B. Flavonoids and stilbenoids were identified by comparing their retention times and m/z values of precursor and resulting fragmentation product ions in their MS and MS/MS spectra, respectively, to those obtained for respective standard solutions analyzed under the same conditions. The quantification of flavonoids was done using calibration curves obtained in the SRM (single reaction mode) (Biesaga & Pyrzyńska Citation2013; Sergiel et al. Citation2014).
Analysis of leaf anatomy
For anatomical measurements, 0.5 mm thick pieces from distal and proximal parts of the leaves (Fig. S1) were cut and fixed for 24 hours in Karmovsky’s fixative containing 10% paraformaldehyde and 25% glutaraldehyde in 0.2 M phosphate buffer (pH 7.2). The fixed material was rinsed three times (15 min. each time) in 0.1 M phosphate buffer (pH 7.4), dehydrated in a graded ethanol series (for 10 min. in 30%, 50%, 70%, and 96% ethanol and 2 × 10 min. in 99.8% ethanol), and placed in 100% polypropylene oxide (2 × 10 min.). The slices were then placed overnight in a 1:1 mixture of epon resin (5.25 ml Poly/Bed 812, 3.25 ml DDSA, 0.75 ml MNA, 0.175 DMP) and propyl oxide. Next day, the plant samples were embedded in 100% epon resin. Transverse slices (0.5 μm) from distal and proximal sections of the leaves were obtained using a rotary automatic microtome Leica HistoCore Nanocut R. Slices were stained with toluidine blue, mounted on slides and examined with a light microscope Carl Zeiss Axio Imager 2 coupled with Zeiss AxioCam ERc 5 s and ZEN Lite computer programme.
Anatomical evaluation of grapevine leaves included measurements of the abaxial epidermal cell thickness, the distance from the abaxial leaf surface to phloem and the simulation of the shortest aphid stylet penetration pathway from abaxial leaf surface to phloem. The stylet pathway was expressed as intercellular area within epidermis and mesophyll (μm2). Three measurements at three different sites of the leaf midrib at proximal and distal sections of the leaf were taken (Fig. S2). The average of the three measurements was considered one replication. The number of replications (=analyzed slices) was n = 9.
Statistical analysis
EPG parameters describing aphid probing behavior were calculated manually and individually for every aphid and the mean and standard errors were subsequently calculated using the EPG analysis Excel worksheet created by the authors especially for this study. The data thus obtained were analyzed for the significance of differences among grapevine species using Kruskal–Wallis test and post-hoc multiple comparisons of mean ranks for all groups (Dunn’s test). Values of p < 0.05 were considered statistically significantly different.
For anatomical measurements, dual comparisons were applied. First, differences between values obtained from distal and proximal parts were evaluated within each grapevine species by Mann-Whitney U-test. Second, differences among grapevine species using values obtained from distal and proximal parts of the leaves were evaluated by Kruskal–Wallis test and post-hoc multiple comparisons of mean ranks for all groups (Dunn’s test). Values of p < 0.05 were considered statistically significantly different.
All statistical calculations were performed using StatSoft, Inc. (2014) STATISTICA (data analysis software system), version 12.
Results
Aphid probing behavior
The 8-h EPG monitoring of Aphis fabae and Myzus persicae behavior on grapevines Vitis amurensis, V. riparia and V. vinifera revealed activities defined as no-probing and probing. Probing activities embraced pathway phase, xylem phase, and phloem phase. Pathway phase included progressive stylet movements in apoplast with intracellular punctures and watery salivation into apoplast. Phloem phase occurred rarely in both aphid species and in all grapevines studied. When present, the phloem phase consisted only of watery salivation into sieve elements. No phloem sap ingestion was recorded in any aphid on any grapevine species (, ).
Figure 1. Visualization of characteristic aphid probing activities derived from Electrical Penetration Graphs (EPGs) technique. The illustration is composed of representative samples from EPG recordings in the present study. The images for panels a, c and d were obtained from EPG recordings of Aphis fabae on Vitis amurensis and the image for panel b was obtained from EPG recording of Myzus persicae on V. amurensis. “np” – no probing, “C” – pathway activity in apoplast with intracellular punctures, “E1e” – watery salivation into apoplast, “E1” – watery salivation into sieve elements, “F” – derailed stylet activities in apoplast, “G” – sap ingestion from xylem vessels.
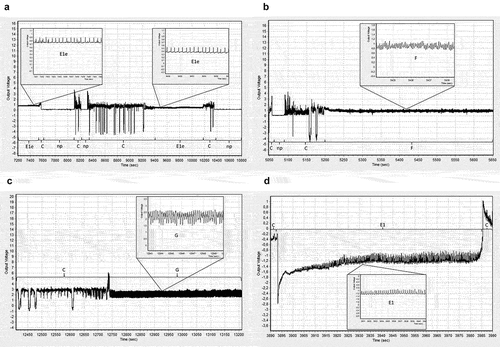
Figure 2. Temporal changes in probing behavior of Aphis fabae and Myzus persicae on grapevines Vitis amurensis, Vitis riparia and Vitis vinifera. G = xylem sap ingestion; E1e = watery salivation into apoplast; F = unidentified difficulties in penetration, E1 = watery salivation into sieve elements, E2 = phloem sap ingestion, C = pathway, np = no-probing. “Total” = proportion of all aphid activities during 8-hour EPG monitoring.
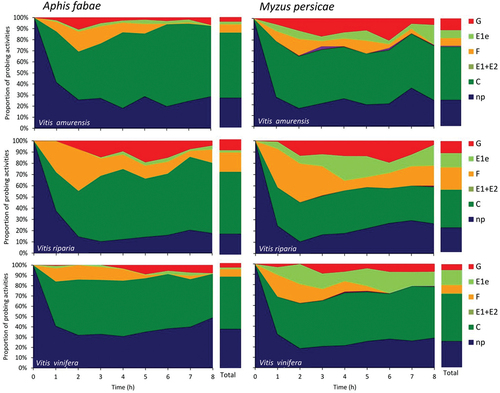
Table I. Probing behavior of Aphis fabae on Vitis amurensis, Vitis riparia and Vitis vinifera.
Table II. Probing behavior of Myzus persicae on Vitis amurensis, Vitis riparia and Vitis vinifera.
In A. fabae, probing activities predominated on all grapevine species during the entire 8-h monitoring period and occupied from 62.6% of the experimental time in V. vinifera to 82.0% in V. amurensis (, ). The average duration of a probe, i.e., the time when aphid stylets were in plant tissues, ranged from 12 minutes on V. vinifera to 47 min. in V. riparia. In V. vinifera, short, less than 2 minutes long, probes were the most common (51% of all probes), while in V. amurensis and V. riparia, the most common were probes longer than 10 minutes (40% and 38%, respectively). The proportion of probes that included phloem phase ranged from 0.4% of the probes in V. vinifera to 1.6% in V. amurensis. Probing activity included pathway phase, which occurred in all studied grapevines, and activities in vascular tissues, which occurred with different frequencies, depending on grapevine species. Within non-vascular tissues, the pathway (= the progressive stylet movements within epidermis and mesophyll apoplast with short intracellular punctures) predominated over other probing activities in all grapevine species (from 49.7% in V. vinifera to 58.9% in V. amurensis). Other activities, i.e., salivation into apoplast of peripheral tissues occupied from 1.4% to 2.3% on V. vinifera and V. amurensis, respectively, and derailed stylet movements – from 7.2% on V. vinifera and V. amurensis to 16.9% on V. riparia. The vascular tissues were reached within 3–5 hours from the onset of the first probe within the experiment, which was represented by the appearance of xylem phase (3–4 hours) and phloem phase (5 hours). Xylem phase was the most common activity within vascular tissues and it occurred in 76.2%, 36.8%, and 22.2% of aphids on V. riparia, V. amurensis and V. vinifera, respectively () and occupied from 4.1% to 9.5% of probing time in V. amurensis and V. riparia, respectively. Phloem phase was rare: it consisted only of watery salivation and occurred in 1 of the 18 studied aphids on V. vinifera (6%), 3 of 21 aphids on V. riparia (14%) and in 6 of 19 aphids on V. amurensis (32%). In V. amurensis, where the phloem phase was the most common, individual periods of phloem phase were short, 36 seconds on average and the average proportion of phloem phase in all aphid probing activities was 0.02% (, ).
Figure 3. Proportion of Aphis fabae and Myzus persicae that reached vascular tissues, phloem and xylem, in the leaves of Vitis amurensis, Vitis riparia and Vitis vinifera.
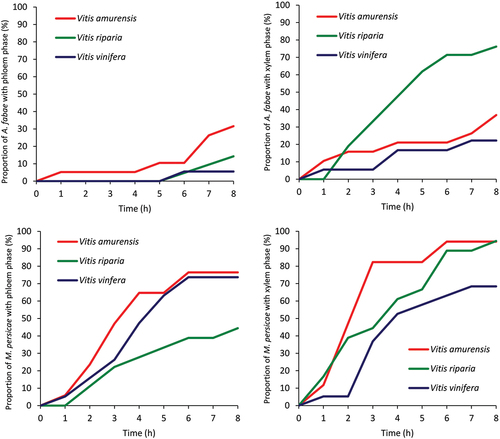
In M. persicae, probing activities occupied from 74.6% of the 8-h experiment in V. vinifera to 77.9% in V. riparia (, ). The average duration of the probe ranged from 17 minutes in V. amurensis and V. vinifera to 27 min in V. riparia. Short, less than 2 minutes long, probes were the most common, from 42% in V. riparia to 54% in V. amurensis. The proportion of probes that included phloem phase ranged from 3.0% in V. riparia to 4.3% in V. vinifera. Probing activity included pathway phase, which occurred in all studied grapevines, and activities in vascular tissues, which occurred with different frequencies, depending on grapevine species. Within non-vascular tissues, the pathway activity predominated in all grapevine species and it occupied from 33.9% to 49.5% of all activities in V. riparia and V. amurensis, respectively. Other activities, i.e., salivation into apoplast of non-vascular tissues, occupied from 7.3% to 14.4% in V. amurensis and V. vinifera, respectively, and derailed stylet activities – from 7.2% to 20.2% on V. amurensis and V. riparia, respectively. The vascular tissues were reached within 2.2–3.6 hours from the onset of the first probe within the experiment, which was represented by the appearance of xylem phase (2.2–3.1 hours) and phloem phase (2.6–3.6 hours). Xylem phase was the most common activity within vascular tissues and it occurred in 94.4%, 94.1%, and 68.4% aphids on V. riparia, V. amurensis, and V. vinifera, respectively () and occupied from 5.7% to 10.9% of the activities on V. vinifera, and V. amurensis and V. riparia, respectively. The phloem phase, which consisted of only watery salivation, occurred in 76%, 74%, and 44% aphids on V. amurensis, V. vinifera, and V. riparia, respectively. Individual bouts of the phloem phase were 1–2 minutes long in all grapevine species and the average proportion of phloem phase in all aphid activities ranged from 0.3% in V. riparia to 1.0% in V. amurensis (, ).
Flavonoids and stilbenoids in grapevine leaves
In grapevine leaves studied, both, flavonoids and stilbenoids, were identified. Of the analyzed flavonoids, the flavan-3-ols catechin and epicatechin and flavonols isorhamnetin, kaempferol, quercetin and rutin, which occurred in different amounts and proportions in individual grapevine species, were detected (Table S1, ). Catechin, epicatechin, and quercetin occurred in all species, kaempferol and rutin were detected in V. amurensis and V. riparia, and isorhamnetin occurred only in V. amurensis. Quercetin was the most abundant flavonoid in V. amurensis and V. riparia (52% and 58% of all flavonoids, respectively), while in V. vinifera – catechin was the most common (71%) (). Of the analyzed stilbenoids, piceid, resveratrol and ε-viniferin were detected. Piceid occurred in all grapevine species, while resveratrol in V. amurensis and V. vinifera and ε-viniferin only in V. vinifera. Piceid was the most abundant stilbenoid in all grapevine species (76%, 100%, and 64% of all analyzed stilbenoid compounds in V. amurensis, V. riparia, and V. vinifera, respectively) (Table S1, ).
Anatomy of grapevine leaves
The anatomical structure of grapevine leaves was analyzed based on transverse sections of proximal and distal parts of the leaves, at the region of the midrib (Fig. S1). The visual examination revealed adaxial epidermis, collenchyma, parenchyma, and the vascular bundle surrounded by a sheath (), which was typical for grapevines (Keller Citation2015a). No visible differences in the anatomical organization of leaf tissues occurred among the studied grapevine species (). The thickness of abaxial epidermis in grapevine leaves ranged from 5.1 μm to 6.1 μm in proximal parts of the leaf and from 5.0 μm to 8.1 μm in distal parts in V. amurensis and V. riparia, respectively (). The distance from abaxial leaf surface to the closest phloem vessel ranged from 62.3 μm to 86.6 μm in proximal parts in V. vinifera and V. riparia, respectively, and from 58.1 μm to 109.3 μm in distal parts in V. vinifera and V. amurensis, respectively. The length of the simulated shortest aphid stylet pathway from abaxial leaf surface to phloem, expressed as intercellular area within epidermis and mesophyll, ranged from 181.6 μm2 in V. vinifera to 279 μm2 in V. riparia in proximal parts of the leaf and from 146.8 μm2 in V. vinifera to 384,7 μm2 in V. amurensis in distal parts ().
Figure 5. Representative samples of transverse sections of grapevine leaves at main veins of the leaves of Vitis amurensis, V. riparia and V. vinifera observed under light microscope Carl Zeiss Axio Imager 2 coupled with Zeiss AxioCam ERc 5s and ZEN Lite computer programme (400x magnification). 1 – adaxial epidermis; 2 – collenchyma; 3 – parenchyma; 4 – vascular bundle sheath; 5 – phloem; 6 – xylem.
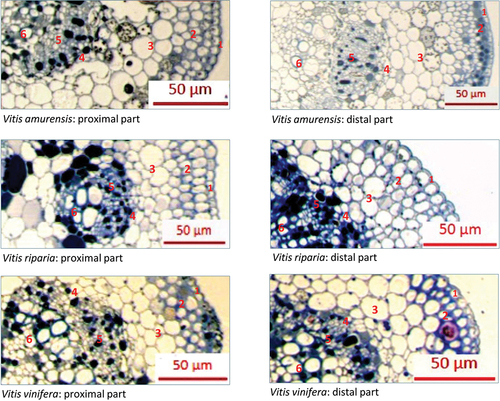
Table III. Anatomical measurements of leaves of Vitis amurensis, Vitis riparia and Vitis vinifera.
Discussion
The analysis of parameters describing the behavior of A. fabae and M. persicae during stylet penetration in leaf tissues showed that the two aphid species responded to the studied grapevine species differently. The recorded significant differences were related both to general aspects of probing and when probing was analyzed individually in non-vascular and vascular tissues.
The total time of no-probing did not differ in the case of M. persicae on each of the examined vines, where it amounted to 2 h in total during the 8-h experiment. In contrast, in A. fabae, no-probing was shortest on V. riparia (1.4 hr) and twice as long on V. riparia and V. vinifera. Consequently, the total duration of A. fabae probing was longest on V. riparia as opposed to the two other grapevine species. The prolonged no-probing times may indicate the presence of factors that discourage the continuation of probing, such as secondary metabolites (Mayoral et al. Citation1996; Prado & Tjallingii Citation1997).
The main activity in non-vascular tissues in all aphids on all grapevines was the progressive movement of stylets towards vascular tissues, classified as “C”: 50–60% in A. fabae and 34–50% in M. persicae. This activity is mainly extracellular, with brief, 5–10 sec punctures of cells adjacent to stylet path (Tjallingii Citation1978). During these punctures, samples of cell contents are ingested for gustatory purposes (Tjallingii & Esch Citation1993; Mayoral et al. Citation1996; Pettersson et al. Citation2007). Aphids use the information gathered during these punctures to continue or terminate the probes (Tjallingii Citation2001, Citation2006). In the present study, the probing activity of aphids on grapevines was frequently interrupted: during the 8-h EPG monitoring, there were from 18.2 to 28.8 probes per aphid in A. fabae and from 22.9 to 39.8 probes per aphid in M. persicae. In A. fabae, significantly more probes (1.5 times more) occurred in V. vinifera than in V. riparia and in M. persicae – 1.5 times more probes occurred in V. amurensis than in V. riparia. It must be stressed that in both M. persicae and A. fabae, short penetrations lasting less than 2 minutes prevailed on all plants. The occurrence and frequency of short probes are good indicators of plant suitability. As the time required by aphid stylets to pass one layer of cells is approximately 2–3 minutes (Van Hoof Citation1958), it means that a large number of short penetrations in relation to the total number of probes may indicate the presence of factors in the epidermis and outer mesophyll that discouraged the continuation of penetration in the tissues of the studied grapevines. Such relationship is typical of incompatible plant-aphid associations (Tjallingii Citation2001; Alvarez et al. Citation2006; Halarewicz & Gabryś Citation2012; Tetreault et al. Citation2019; Souza & Davis Citation2020). The presence of factors limiting probing within mesophyll is also indicated by the time from the beginning of penetration to the first phloem or xylem phase, i.e. the time to reach the vascular bundle in the leaves (Tjallingii Citation2001; Alvarez et al. Citation2006). During probing in grapevine leaf tissues, the phloem and xylem phases appeared in EPG recordings 2.6–3.6 and 2.2–3.1 hours, respectively, after the start of the first penetration in M. persicae, and after 5 and 3.4–4 hours, respectively, in A. fabae. On suitable hosts, aphids reach phloem usually after 2.4 hours of probing in non-vascular tissues (Wróblewska-Kurdyk et al. Citation2019). Moreover, the prolonged penetration time in the non-phloem compartments in relation to the penetration of phloem tissues may result from negative factors present in the phloem itself (Mayoral et al. Citation1996). Studies on the susceptibility of the varieties of two lupine species – yellow lupine Lupinus luteus L. and narrow-leaf lupine Lupinus angustifolius L. to the feeding of the pea aphid Acyrthosiphon pisum Harris have shown that the presence of various alkaloids in the tissues of lupines might have caused the extension of the duration of no-probing and an increase in the number of short probes (Kordan et al. Citation2008; Wróblewska et al. Citation2012). On the other hand, the reason for the occurrence of a large number of short penetrations may be the specificity of the method used to assess the behavior of aphids during the penetration of plant tissues (EPG technique), in which the aphids attached to an electrode of a certain length cannot leave the plant that is not suitable for them (Tjallingii Citation1986; Halarewicz & Gabryś Citation2012). Nevertheless, the EPG technique is indispensable to monitor aphid stylet penetration within plant tissues, as this activity is hidden from the human eye (Dancewicz et al. Citation2016; Zhang et al. Citation2017; Stec et al. Citation2021). This technique allows also a separate analysis of aphid behavior at pre-ingestive (within non-phloem tissues before the first phloem or xylem phase) and ingestive (within the phloem or xylem) phases of probing and localization of natural plant resistance factors (Pettersson et al. Citation2007). Apart from typical pathway activity “C”, aphids on grapevine leaves also showed two other activities in non-vascular tissues which occurred quite commonly: “F” and “E1e”. Activity “F” visualizes probably the “derailment” of the stylets in plant tissues (Alvarez et al. Citation2006; Silva-Sanzana et al. Citation2020) and its occurrence is usually linked to the aphid or plant physiological state or plant resistance level (Alvarez et al. Citation2007; Marchetti et al. Citation2009; Pompon & Pelletier Citation2012; Machado-Assefh & Alvarez Citation2018). In the present study, the significant increase in the duration of “F” was noted in M. persicae on V. riparia in relation to other species (more than 2 times longer duration of “F”). In A. fabae, no significant differences in the duration of “F” were observed, but on V. riparia, A. fabae also showed a tendency to increase the incidence of “F” in comparison to other grapevine species. The occurrence of “E1e” in EPG recordings is rare, and this activity is not fully understood. Most likely, it is associated with the secretion of watery saliva in the apoplast (Huang et al. Citation2012; Wu et al. Citation2013). Watery saliva contains a variety of detoxifying enzymes (Klinger et al. Citation1998; Miles Citation1999), hence the presence of this extracellular salivation activity and its increased proportion in aphid probing may indicate the presence of factors in epidermis and mesophyll that do not favor aphid penetration of plant tissues (Huang et al. Citation2012; Wu et al. Citation2013). In the present study, “E1e” occurred quite commonly in all aphids on all grapevines.
The success rate in reaching vascular bundles varied and it depended on both the aphid and the grapevine species. The proportion of A. fabae reaching xylem was highest on V. riparia (76%) and lowest on V. vinifera (22%) and phloem – highest on V. amurensis (31.6%) and lowest on V. vinifera (5.6%). The proportion of M. persicae reaching xylem was equally high on V. amurensis and V. riparia (94%) and lower on V. vinifera (68.4%) and phloem – comparable on V. amurensis and V, vinifera (76.5% and 73.7%, respectively) and lower on V. amurensis (44.4%). The duration of xylem phase “G” varied depending on the species of grapevine. In A. fabae, the duration of “G” was significantly longer on V. riparia in comparison to V. amurensis and V. vinifera. In M. persicae, the duration of “G” did not differ significantly among grapevine species. The increased proportion of xylem phase occurs in fasted individuals and may be caused by the presence of negative factors in the phloem tissues, which make it impossible to use phloem resources (Spiller et al. Citation1990; Leszczyński Citation1996). On the other hand, the increased xylem phase activity may also be caused by the inability to use phloem resources due to the presence of negative factors in the plant at the stage before the aphids reach the phloem (Liang et al. Citation2012; Souza & Davis Citation2020). The incidence of phloem phase in the present study was low and no phloem sap ingestion was recorded in any aphid that did reach sieve elements; phloem phase was represented of only watery salivation “E1”. A low percentage of aphids showing the phloem or xylem phase during probing may be due to the presence of negative factors in mesophyll, indicating difficulties in reaching vascular elements (Jiang et al. Citation2001; Kordan et al. Citation2019). The reduced uptake of phloem sap or complete absence of sap ingestion is the main feature related to plant resistance to aphid feeding: on susceptible plants, aphids have the ability to quickly initiate phloem sap uptake and achieve the continuous feeding phase (Sauge et al. Citation1998). As a rule, in susceptible (or host) plants, sap ingestion occurs shortly after the start of the phloem phase, moreover, in susceptible plants, the ingestion of phloem sap may last for many hours continuously (Akbar et al. Citation2014; Kordan et al. Citation2019). The presence of only E1 model may indicate the presence of factors in the phloem that negatively affect the behavior and feeding of aphids (Alvarez et al. Citation2006). These factors may make it difficult or impossible for aphids to take phloem sap. As stated earlier, saliva secreted by aphids may play a role in responses to plant defense mechanisms present in sieve elements, e.g. take part in detoxification of phenolic compounds produced by plants (Klinger et al. Citation1998; Canassa et al. Citation2020).
The studied grapevines show the features of resistant plants, described in numerous studies. (Sauge et al. Citation1998; Kordan et al. Citation2008, Citation2021; Philippi et al. Citation2015; Sun et al. Citation2018; Stec et al. Citation2021). Aphids on the resistant plant species or cultivars have difficulties in finding phloem vessels and/or start and continue feeding in a sustained manner. Usually, the observed resistance is linked to the variation in the content of plant-specific allelochemicals (Kordan et al. Citation2008, Citation2021; Philippi et al. Citation2015; Stec et al. Citation2021). Grapevine resistance to A. fabae and M. persicae detected in the present study, is most likely related to the presence of the antixenosis mechanism at the level of mesophyll and also phloem tissues: the phloem phase constituted a negligible proportion in the entire probing activity, the ingestion of phloem sap was non-existent, not all aphids reached the phloem elements, and those that reached the phloem phase did that at the late stages of the experiment. A high proportion of pathway and xylem phases during the entire aphid activity was also demonstrated, and a high proportion of G, F, and E1e activities was also observed. Antixenosis-based plant resistance to aphid feeding may result from the presence and concentration of secondary metabolites in plant tissues (Smith & Boyko Citation2007; Dogimont et al. Citation2010; Smith & Clement Citation2012; Smith & Chuang Citation2014). Among secondary metabolites, phenolic compounds are frequently reported as associated with plant resistance to herbivores (Lattanzio et al. Citation2000; Simmonds Citation2003; Buer et al. Citation2008; Pawlus et al. Citation2012; Ahmed et al. Citation2019). The studied grapevines differed in the quantitative and qualitative content of the analyzed group of phenolic compounds, flavonoids and stilbenoids. In all grapevines, flavonoids predominated among the analyzed phenolic compounds: in V. amurensis and V. riparia flavonoids amounted to 90% of the major phenolic compounds analyzed while in V. vinifera – 76%. The flavonoids belonged to two groups: flavan-3-ols and flavonols. Flavan-3-ols - catechin and epicatechin were present in the tissues of all studied grapevines. Of the flavonols analyzed, kaempferol, quercetin and rutin occurred in V. amurensis and V. riparia, while isorhamnetin occurred only in V. amurensis, and in V. vinifera only quercetin occurred. The stilbenoids occurred in all grapevines. Of the stilbenoids analyzed, piceid, resveratrol and ε-viniferin were detected in V. vinifera, piceid and resveratrol occurred in V. amurensis, and only piceid was recorded in V. riparia. The relationship between the susceptibility/resistance to aphids and the content of quercetin, kaempferol and isorhamnetin in plants was reported by Lattanzio et al. (Citation2000), Togola et al. (Citation2020), Kozak et al. (Citation2015); Goławska and Łukasik (Citation2009). In addition, the application of flavonoids to artificial diets confirmed the deterrent effect of flavonoids on the probing and feeding of several aphid species (Goławska & Łukasik Citation2012; Goławska et al. Citation2014; Stec et al. Citation2021).
In the anatomical studies of grapevine leaves, the following characters were determined: epidermal thickness, distance between abaxial leaf surface and phloem, and the simulated shortest pathway from epidermis to phloem. The analysis of data showed differences in the anatomical structure of leaves of individual grapevine species and varieties. Measurements were made in two parts of the leaf: the proximal part and the distal part. Significant differences occurred in the distance between epidermis and phloem between distal and proximal part of the leaf in V. amurensis and in the epidermal thickness and the simulated pathway in V. riparia. The epidermal thickness was highest in the distal part of V. riparia and the distance between leaf surface and phloem, and the value of the simulated pathway were highest in the distal parts of V. amurensis leaves and lowest in V. vinifera distal parts of the leaves. Aphids were able to move across the plant for a distance determined by the length of the electrode attached to the body (about 3 cm); therefore, during the EPG monitoring, they could penetrate tissues in various parts of the leaf (proximal and distal parts). Considering the results of the EPG monitoring of aphid behavior on grapevines, it can be inferred that the anatomical parameters did not have a significant impact on the time to reach the phloem and xylem elements by M. persicae and A. fabae. The differences in aphid behavior on grapevines, especially those related to probing in non-phloem tissues did not correspond with the differences in plant anatomy. When analyzing the data in studies to assess the susceptibility of grapevine species to the feeding of A. fabae and M. persicae, the main EPG variables indicating difficulties in reaching the vascular tissue, both phloem and xylem, were the time from the beginning of the experiment to reaching phloem and/or xylem elements, or the percentage of aphids that reached the vascular elements. In V. amurensis, where a large distance between plant surface and phloem was noted and the simulated pathway was the longest, no significant differences in the values of EPG variables were observed. Similarly, no differences were found during the penetration of V. vinifera tissues, where the above two parameters had a lower value (the lowest epidermis-phloem distance, the shortest simulated pathway). The findings in the present study are in accordance with the results obtained by other authors who determined that the resistance of the studied plant cultivars did not depend on the anatomical structure of the leaves (Singh et al. Citation2020)
In conclusion, the present study revealed that all studied species of grapevines, V. amurensis, V. riparia and V. vinifera are relatively resistant to A. fabae and M. persicae (class “IV”). These grapevines showed features related to the activity of antixenosis factors at the level of mesophyll and phloem tissues, which was manifested in difficulties in tissue penetration by aphids, little success in reaching the phloem phase and its short duration. Vitis vinifera was the least susceptible grapevine species to A. fabae. No differences in the susceptibility among grapevines occurred against M. persicae. However, considering the higher success rate in reaching phloem phase and longer duration of probing, it can be inferred that M. persicae poses greater threat to the studied grapevines than A. fabae. The longer duration of probing increases the risk of virus transmission. Differences in anatomical structure of the leaves did not relate with aphid probing behavior. However, flavonoids and stilbenoids may have caused the reduction of probing in phloem tissues. The present study revealed that all studied species of grapevines, V. amurensis, V. riparia and V. vinifera showed features related to the activity of antixenosis factors at the level of mesophyll and phloem tissues, which was manifested in difficulties in leaf tissue penetration by aphids, little success in reaching the phloem phase and its short duration. It can be assumed that anatomical structure of the leaves did not affect aphid probing behavior but flavonoids and stilbenoids might have caused the reduction of probing in phloem tissues. However, unambiguous determination of the direct influence of individual flavonoids and stilbenoids and particularly, piceid, on the behavior of M. persicae and A. fabae requires further, more detailed studies involving the application of artificial diets.
Acknowledgements
The Authors express sincere thanks to Dr. W.F. Tjallingii for confirming ‘E1e’ EPG waveform pattern.
Disclosure statement
No potential conflict of interest was reported by the authors.
Additional information
Funding
References
- Ahmed M, Ji M, Qin P, Gu Z, Liu Y, Sikandar A, Iqbal MF, Javeed A. 2019. Phytochemical screening, total phenolic and flavonoids contents and antioxidant activities of Citrullus colocynthis L. and Cannabis sativa L. Applied Ecology And Environmental Research 17(3):6961–6979. DOI: 10.15666/aeer/1703_69616979.
- Akbar W, Showler AT, Reagan TE, Davis JA, Beuzelin JM. 2014. Feeding by sugarcane aphid, Melanaphis sacchari, on sugarcane cultivars with differential susceptibility and potential mechanism of resistance. Entomologia Experimentalis Et Applicata 150(1):32–44. DOI: 10.1111/eea.12136.
- Aliaño-González MJ, Richard T, Cantos-Villar E. 2020. Grapevine cane extracts: Raw plant material, extraction methods, quantification, and applications. Biomolecules 10(8):1195. DOI: 10.3390/biom10081195.
- Alvarez AE, Garzo E, Verbeek M, Vosman B, Dicke M, Tjallingii WF. 2007. Infection of potato plants with potato leafroll virus changes attraction and feeding behaviour of Myzus persicae. Entomologia Experimentalis Et Applicata 125(2):135–144. DOI: 10.1111/j.1570-7458.2007.00607.x.
- Alvarez AE, Tjallingii WF, Garzo E, Vleeshouwers V, Dicke M, Vosman B. 2006. Location of resistance factors in the leaves of potato and wild tuber-bearing Solanum species to aphid Myzus persicae. Entomologia Experimentalis Et Applicata 121(2):145–157. DOI: 10.1111/j.1570-8703.2006.00464.x.
- Ammar E-D, Richardson ML, Abdo Z, Hall DG, RG Jr. S. 2014. Differences in stylet sheath occurrence and the fibrous ring (Sclerenchyma) between x Citroncirus plants relatively resistant or susceptible to adults of the Asian Citrus Psyllid Diaphorina citri (Hemiptera: Liviidae). PLoS ONE 9(10):e110919. DOI: 10.1371/journal.pone.0110919.
- Baroi AM, Popitiu M, Fierascu I, Sărdărescu I-D, Fierascu RC. 2022. Grapevine wastes: A rich source of antioxidants and other biologically active compounds. Antioxidants 11(2):393. DOI: 10.3390/antiox11020393.
- Baronio CA, Andzeiewski S, Silva da Cunha U, Botton M. 2014. Biologia e tabela de vida de fertilidade do pulgao-preto em cultivares de videira. Pesquisa Agropecuaria Brasilieira Brasília 49(9):665–672. DOI: 10.1590/S0100-204X2014000900002.
- Basso MF, Fajardo TVM, Saldarelli P. 2016. Grapevine virus diseases: Economic impact and current advances in viral prospection and management. Revista Brasiliera de Fruticultura 39(1):e–411. DOI: 10.1590/0100-29452017411.
- Biais B, Krisa S, Cluzet S, Da Costa G, Waffo-Teguo P, Mérillon J-M, Richard T. 2017. Antioxidant and cytoprotective activities of grapevine stilbenes. Journal of Agricultural and Food Chemistry 65(24):4952–4960. DOI: 10.1021/acs.jafc.7b01254.
- Biesaga M, Pyrzyńska K. 2013. Stability of bioactive polyphenols from honey during different extraction methods. Food Chemistry 136(1):46–54. DOI: 10.1016/j.foodchem.2012.07.095.
- Blackman RL, Eastop VF. 2006. Host lists and keys. In: Aphids on the world’s herbaceous plants and shrubs. Chichester: John Wiley & Sons Ltd, Natural History Museum.
- Boczek J. 1988. Odporność roślin oraz hodowla i uprawa odmian odpornych jako metoda walki ze szkodnikami: 47-57. Nauka o szkodnikach. Państwowe Wydawnictwo Rolnicze i Leśne.
- Buer CS, Muday GK, Djordjevic MA. 2008. Implications of long-distance flavonoid movement in Arabidopsis thaliana. Plant Signaling & Behavior 3(6):415–417. DOI: 10.4161/psb.3.6.5440.
- Burns J, Mullen W, Landrault N, Teissedre P-L, Lean MEJ, Crozier A. 2002. Variations in the profile and content of anthocyanins in wines made from cabernet sauvignon and hybrid grapes. Journal of Agricultural and Food Chemistry 50(14):4096–4102. DOI: 10.1021/jf011233s.
- CABI. 2022a. Aphis fabae. In: CABI Compendium. Wallingford, UK: CAB International. Available: https://doi.org/10.1079/cabicompendium.6196. Accesseed 24 May 2022.
- CABI. 2022b. Myzus persicae. In: CABI Compendium. Wallingford, UK: CAB International. Available: https://doi.org/10.1079/cabicompendium.35642. Accessed: 24 May 2022.
- Canassa VF, Baldin ELL, Lourencao AL, Barros DRP, Lopes NP, Sartori MMP. 2020. Feeding behavior of Brevicoryne brassicae in resistant and susceptible collard greens genotypes: Interactions among morphological and chemical factors. Entomologia Experimentalis Et Applicata 168(3):228–239. DOI: 10.1111/eea.12897.
- Castillo-Munoz N, Gomez-Alonso S, Garcıa-Romero E, Hermosın-Gutierrez I. 2010. Flavonol profiles of Vitis vinifera white grape cultivars. Journal of Food Composition and Analysis 23(7):699–705. DOI: 10.1016/j.jfca.2010.03.017.
- Chan CK, Forbes AR, Raworth DA. 1991. Aphid-transmitted viruses and their vectors of the world. Technical Bulletin 1991-3E. Vancouver: Research Branch Agriculture Canada.
- Chong J, Poutaraud A, Hugueney P. 2009. Metabolism and roles of stilbenes in plants. Plant Science 177(3):143–155. DOI: 10.1016/j.plantsci.2009.05.012.
- Dancewicz K, Sznajder K, Załuski D, Kordan B, Gabryś B. 2016. Behavioral sensitivity of Myzus persicae to volatile isoprenoids in plant tissues. Entomologia Experimentalis Et Applicata 160(3):229–240. DOI: 10.1111/eea.12480.
- De la Fuente Lloreda M. 2018. Use of hybrids in viticulture. A challenge for the OIV. OENO One 52(3):231–234. DOI: 10.20870/oeno-one.2018.52.3.2312.
- De Rosso M, Tonidandel L, Larcher R, Nicolini G, Vedova AD, De Marchi F, Gardiman M, Giusta M, Flamini R. 2014. Identification of new flavonols in hybrid grapes by combined liquid chromatography–mass spectrometry approaches. Food Chemistry 163:244–251. DOI: 10.1016/j.foodchem.2014.04.110.
- Dogimont C, Bendahmane A, Chovelon V, Boissot N. 2010. Host plant resistance to aphids in cultivated crops: Genetic and molecular bases, and interactions with aphid populations. Comptes Rendus - Biologies 333(6–7):566–573. DOI: 10.1016/j.crvi.2010.04.003.
- Fuchs M. 2020. Grapevine viruses: A multitude of diverse species with simple but overall poorly adopted management solutions in the vineyard. Journal of Plant Pathology 102(3):643–653. DOI: 10.1007/s42161-020-00579-2.
- Gabryś B, Pawluk M. 1999. Acceptability of different species of Brassicaceae as hosts for the cabbage aphid. Entomologia Experimentalis Et Applicata 91(1):105–109. DOI: 10.1046/j.1570-7458.1999.00471.x.
- Goławska S, Łukasik I. 2009. Acceptance of low-saponin lines of alfalfa with varied phenolic concentrations by pea aphid. Biologia Section Zoology 64(2):377–382. DOI: 10.2478/s11756-009-0051-5.
- Goławska S, Łukasik I. 2012. Antifeedant activity of luteolin and genistein against the pea aphid, Acyrthosiphon pisum. Journal of Pest Science 85(4):443–450. DOI: 10.1007/s10340-012-0452-z.
- Goławska S, Łukasik I, Kapusta I, Janda B. 2010. Analysis of flavonoids content in alfalfa/ Analiza zawartości flawonoidów w lucernie. Ecological Chemistry and Engineering 17(2–3):261–267.
- Goławska S, Sprawka I, Łukasik I. 2014. Are naringenin and quercetin useful chemicals in pest-management strategies? Journal of Pest Science 87(1):173–180. DOI: 10.1007/s10340-013-0535-5.
- Golonko A, Kalinowska M, Świsłocka R, Świderski G, Lewandowski W. 2015. Zastosowanie związków fenolowych i ich pochodnych w przemyśle i medycynie. Civil and Environmental Engineering 6:161–179.
- Goufo P, Singh RK, Cortez I. 2020. A reference list of phenolic compounds (including stilbenes) in grapevine (Vitis vinifera L.) roots, woods, canes, stems, and leaves. Antioxidants 9(5):398. DOI: 10.3390/antiox9050398.
- Halarewicz A, Gabryś B. 2012. Probing behavior of bird cherry oat aphid Rhopalosiphum padi (L.) on native bird cherry Prunus padus L. and alien invasive black cherry Prunus serotine Erhr. In Europe and the role of cyanogenic glycosides. Anthropod-Plant Interactions 6(4):497–505. DOI: 10.1007/s11829-012-9228-x.
- Harborne JB. 1997. Ekologia biochemiczna. Warszawa: PWN.
- Holman J. 2009. Host plant catalog of aphids. Dordrecht: Springer. p. 1140.
- Huang F, Tjallingii WF, Zhang P, Zhang J, Lu Y, Lin J. 2012. EPG waveform characteristics of solenopsis mealybug stylet penetration on cotton. Entomologia Experimentalis Et Applicata 143(1):47–54. DOI: 10.1111/j.1570-7458.2012.01233.x.
- James DG, Whitney J. 1993. Mite populations on grapevines in south-eastern Australia: Implications for biological control of grapevine mites (Acarina: Tenuipalpidae, Eriophyidae). Experimental & Applied Acarology 17(4):259–270. DOI: 10.1007/BF02337275.
- Jasiński M, Mazurkiewicz M, Rodziewicz P, Figlerowicz M. 2009. Flawonoidy – Budowa, właściwości i funkcja ze szczególnym uwzględnieniem roślin motylkowatych. Biotechnologia 2(85):81–94.
- Jeszka M, Flaczyk E, Kobus-Cisowska J, Dziedzic K. 2010. Związki fenolowe – Charakterystyka i znaczenie w technologii żywności. Nauka Przyroda Technologie 4(2):1–13.
- Jiang YX, Nombela G, Muniz M. 2001. Analysis by DC-EPG of the resistance to Bemisia tabaci on an Mi -tomato line. Entomologia Experimentalis Et Applicata 99(3):295–302. DOI: 10.1046/j.1570-7458.2001.00828.x.
- Kedrina-Okutan O, Novello V, Hoffmann T, Hadersdorfer J, Schneider A, Schwab W, Ferrandino A. 2019. Polyphenolic diversity in Vitis sp. Leaves. Scientia Horticulturae 256:108569. DOI: 10.1016/j.scienta.2019.108569.
- Keller M. 2015a. Botany and anatomy: 1-57. In: The science of grapevines. 2nd ed. Oxford: Elsevier LTD. pp. 522.
- Keller M. 2015b. Living with other organisms: 343-367. In: The science of grapevines. 2nd ed. Oxford: Elsevier LTD. pp. 522.
- Kidd NAC. 1976. Factors influencing leaf vein selection in the lime aphid (Eucallipterus tiliae L.). Oecologia (Berl.) 23(3):247–254. DOI: 10.1007/BF00361239.
- Klinger J, Powell G, Thompson GA, Isaacs R. 1998. Phloem specific aphid resistance in Cucumis melo line AR5: Effects on feeding behaviour and performance of Aphis gossypii. Entomologia Experimentalis Et Applicata 86(1):79–88. DOI: 10.1046/j.1570-7458.1998.00267.x.
- Kordan B, Gabryś B, Dancewicz K, Lahuta LB, Piotrowicz-Cieślak A, Rowińska E. 2008. European yellow lupine, Lupinus luteus, and narrow-leaf lupine, Lupinus angustifolius, as hosts for the pea aphid, Acyrthosiphon pisum. Entomologia Experimentalis Et Applicata 128(1):139–146. DOI: 10.1111/j.1570-7458.2008.00702.x.
- Kordan B, Stec K, Słomiński P, Giertych M, Wróblewska-Kurdyk A, Gabryś B. 2018. Susceptibility of forage legumes to infestation by the pea aphid Acyrthosiphon pisum (Harris) (Hemiptera: Aphididae). Crop & Pasture Science 69(8):775–784. DOI: 10.1071/CP18065.
- Kordan B, Stec K, Słomiński P, Laszczak-Dawid A, Wróblewska-Kurdyk A, Gabryś B. 2019. Antixenosis potential in pulses against the pea aphid (Hemiptera: Aphididae). Journal of Economic Entomology 112(1):465–474. DOI: 10.1093/jee/toy349.
- Kordan B, Wróblewska-Kurdyk A, Bocianowski J, Stec K, Jankowski K, Gabryś B. 2021. Variation in susceptibility of rapeseed cultivars to the peach potato aphid. Journal of Pest Science 94(2):435–449. DOI: 10.1007/s10340-020-01270-2.
- Kozak A, Chrzanowski G, Sempruch C, Klewek A, Chwedczuk M. 2015. Effect of selected flavonoids on the behavior of the bird cherry-oat aphid (Rhopalosiphum padi L.) during the colonization of winter wheat. Progress In Plant Protection 55(2):202–206. DOI: 10.14199/ppp-2015-033.
- Kozłowska M, Czekała Ł. 2017. Stilbenes and their role in disease resistance. Progress In Plant Protection 57(1):27–35. DOI: 10.14199/ppp-2017-004.
- Lattanzio V, Arpaia S, Cardinali A, Di Venere D, Linsalata V. 2000. Role of endogenous flavonoids in resistance mechanism of Vigna to aphids. Journal of Agricultural and Food Chemistry 48(11):5316–5320. DOI: 10.1021/jf000229y.
- Leszczyński B. 1996. Kurs praktyczny w zakresie chemicznych interakcji owady – Rośliny na przykładzie mszyc (Aphidoidea). Siedlce: WSRP. pp. 16–63.
- Liang L, Liu L, Yu X, Han B. 2012. Evaluation of the resistance of different tea cultivars to tea aphids by EPG technique. Journal of Integrative Agriculture 11(12):2028–2034. DOI: 10.1016/S2095-3119(12)60460-2.
- Machado-Assefh CR, Alvarez AE. 2018. Probing behavior of aposymbiotic green peach aphid (Myzus persicae) on susceptible Solanum tuberosum and resistant Solanum stoloniferum plants. Insect Science 25(1):127–136. DOI: 10.1111/1744-7917.12372.
- Majewska M, Czeczot H. 2009. Flawonoidy w profilaktyce i terapii. Farmacja Polska 65(5):369–377.
- Makowska-Wąs J, Janeczko Z. 2008. Stilbeny naturalnego pochodzenia. Część I. Czasopismo Aptekarskie, Vol. 15, Suppl. Rośliny Lecznicze w Polsce i na Świecie 2:7–15.
- Malinowski H. 2008. Strategie obronne roślin drzewiastych przed szkodliwymi owadami. Leśne Prace Badawcze (Forest Research Papers) 69(2):165–173.
- Marchetti E, Civolani S, Leis M, Chicca M, Tjjalingii WF, Pasqualini E, Baronio P. 2009. Tissue location of resistance in apple to the rosy apple aphid established by electrical penetration graphs. Bulletin of Insectology 62(2):203–208.
- Martin B, Collar JL, Tjallingi WF, Fereres A. 1997. Intracellular ingestion and salivation by aphids may cause the acquisition and inoculation of non-persistently transmitted plant viruses. Journal of General Virology 78(10):2701–2705. DOI: 10.1099/0022-1317-78-10-2701.
- Mayoral AM, Tjallingii WF, Castanera P. 1996. Probing behavior of Diuraphis noxia on five cereal species with different hydroxyamic acid levels. Entomologia Experimentalis Et Applicata 78(3):341–348. DOI: 10.1111/j.1570-7458.1996.tb00799.x.
- Miles P. 1999. Aphid saliva. Biological Reviews 74(1):41–85. DOI: 10.1017/S0006323198005271.
- Myśliwiec R. 2009. Uprawa winorośli. Kraków: Plantpress. pp. 164.
- Nopo-Olazabal C, Condori J, Nopo-Olazabal L, Medina-Bolivar F. 2014. Differential induction of antioxidant stilbenoids in hairy roots of Vitis rotundifolia treated with methyl jasmonate and hydrogen peroxide. Plant Physiology and Biochemistry 74:50–69. DOI: 10.1016/j.plaphy.2013.10.035.
- Ocete R, Lopez MA, Gallardo A, Arnold C. 2008. Comparative analysis of wild and cultivated grapevine (Vitis vinifera) in the Basque Region of Spain and France. Agriculture, Ecosystems & Environment 123(1–3):95–98. DOI: 10.1016/j.agee.2007.05.009.
- Omer AD, Granett J, Shebelut CW. 1999. Effect of attack intensity on host utilization in grape phylloxera. Crop Protection 18(5):341–347. DOI: 10.1016/S0261-2194(99)00033-2.
- Pawlus AD, Waffo-Téguo P, Shaver J, Mérillon JM. 2012. Stilbenoid chemistry from wine and the genus Vitis, a review. Journal International Des Sciences de la Vigne Et du Vin 46(2):57–111.
- Petrussa E et al. 2013. Plant flavonoids–biosynthesis, transport and involvement in stress responses. International Journal of Molecular Sciences 14(7):14950–14973. DOI: 10.3390/ijms140714950.
- Pettersson J, Tjallingii WF, Hardie J. 2007. Host plant selection and feeding. In: van Emden H, Harrington R, editors. Aphids as crop pests. Wallingford UK: CAB International. pp. 87–114.
- Philippi J, Schliephake E, Jurgens HU, Jansen G, Ordon F. 2015. Feeding behavior of aphids on narrow-leafed lupin (Lupinus angustifolius) genotypes varying in the content of quinolizidine alkaloids. Entomologia Experimentalis Et Applicata 156(1):37–51. DOI: 10.1111/eea.12313.
- Pompon J, Pelletier Y. 2012. Changes in aphid probing behaviour as a function of insect age and plant resistance level. Bulletin of Entomological Research 102(5):550–557. DOI: 10.1017/S0007485312000120.
- Portu J, López-Alfaro I, Gómez-Alonso S, López R, Garde-Cerdán T. 2015. Changes on grape phenolic composition induced by grapevine foliar applications of phenylalanine and urea. Food Chemistry 180:171–180. DOI: 10.1016/j.foodchem.2015.02.042.
- Powell G, Tosh CR, Hardie J. 2006. Host plant selection by aphids: Behavioral, evolutionary, and applied perspectives. Annual Review of Entomology 51(1):309–330. DOI: 10.1146/annurev.ento.51.110104.151107.
- Prado E, Tjallingii WF. 1997. Effects of previous plant infestation on sieve element acceptance by two aphids. Entomologia Experimentalis Et Applicata 82(2):189–200. DOI: 10.1046/j.1570-7458.1997.00130.x.
- Sauge M-H, Kervella J, Rahbe Y. 1998. Probing behaviour of the green peach aphid Myzus persicae on resistant Prunus genotypes. Entomologia Experimentalis Et Applicata 89(3):223–232. DOI: 10.1046/j.1570-7458.1998.00403.x.
- Sekrecka M, Łabanowska BH, Piotrowski W. 2015. Integrowana metoda ograniczania szkodników winorośli. In: Lisek J, editor. Metodyka Integrowanej Ochrony Winorośli (Materiały dla producentów) – Opracowanie zbiorowe. Skierniewice: Instytut Ogrodnictwa. pp. 29–43.
- Sergiel I, Pohl P, Biesaga M. 2014. Characterisation of honeys according to their content of phenolic compounds using high performance liquid chromatography/tandem mass spectrometry. Food Chemistry 145:404–408. DOI: 10.1016/j.foodchem.2013.08.068.
- Silva-Sanzana C, Estevez JM, Blanco-Herrera F. 2020. Influence of cell wall polymers and their modifying enzymes during plant–aphid interactions. Journal of Experimental Botany 71(13):3854–3864. DOI: 10.1093/jxb/erz550.
- Simmonds MSJ. 2003. Flavonoid-insect interactions: Recent advances in our knowledge. Phytochemistry 64(1):21–30. DOI: 10.1016/S0031-9422(03)00293-0.
- Singh B, Simon A, Halsey K, Kurup S, Clark S, Aradottir GI. 2020. Characterisation of bird cherry-oat aphid (Rhopalosiphum padi L.) behaviour and aphid host preference in relation to partially resistant and susceptible wheat landraces. Annals Applied Biology 177(2):184–194. DOI: 10.1111/aab.12616.
- Smith CM, Boyko EV. 2007. The molecular bases of plant resistance and defense responses to aphid feeding: Current status. Entomologia Experimentalis Et Applicata 122(1):1–16. DOI: 10.1111/j.1570-7458.2006.00503.x.
- Smith CM, Chuang WP. 2014. Plant resistance to aphid feeding: Behavioral, physiological, genetic and molecular cues regulate aphid host selection and feeding. Pest Management Science 70(4):528–540. DOI: 10.1002/ps.3689.
- Smith CM, Clement SL. 2012. Molecular bases of plant resistance to arthropods. Annual Review of Entomology 57(1):309–328. DOI: 10.1146/annurev-ento-120710-100642.
- Souza MF, Davis JA. 2020. Detailed characterization of Melanaphis sacchari (Hemiptera: Aphididae) feeding behavior on different host plants. Environmental Entomology 49(3):683–691. DOI: 10.1093/ee/nvaa036.
- Spiller NJ, Koenders L, Tjallingii WF. 1990. Xylem ingestion by aphids – A strategy for maintaining water balance. Entomologia Experimentalis Et Applicata 55(2):101–104. DOI: 10.1111/j.1570-7458.1990.tb01352.x.
- Stec K, Kordan B, Sergiel I, Biesaga M, Mroczek J, Bocianowski J, Gabryś B. 2021. Antixenosis in Glycine max (L.) Merr against Acyrthosiphon pisum (Harris). Scientific Reports 11(1):15289. DOI: 10.1038/s41598-021-94703-6.
- Stout MJ. 2013. Reevaluating the conceptual framework for applied research on host-plant resistance. Insect Science 20(3):263–272. DOI: 10.1111/1744-7917.12011.
- Sun M, Voorrips RE, Steenhuis-Broers G, Van’t Westende W, Vosman B. 2018. Reduced phloem uptake of Myzus persicae on an aphid resistant pepper accession. BMC Plant Biology 18(1):138. DOI: 10.1186/s12870-018-1340-3.
- Teissedre P-L. 2018. Composition of grape and wine from resistant vines varieties. OENO One 52(3):211–217. DOI: 10.20870/oeno-one.2018.52.3.2223.
- Tetreault HM, Grover S, Scully ED, Gries T, Palmer NA, Sarath G, Louis J, Sattler SE. 2019. Global Responses of Resistant and Susceptible Sorghum (Sorghum bicolor) to Sugarcane Aphid (Melanaphis sacchari). Frontiers in Plant Science 10:145. DOI: 10.3389/fpls.2019.00145.
- Tjallingii WF. 1978. Electronic recording of penetration behavior by aphids. Entomologia Experimentalis Et Applicata 24(3):521–530. DOI: 10.1111/j.1570-7458.1978.tb02836.x.
- Tjallingii WF. 1986. Wire effects on aphids during electrical recording of stylet penetration. Entomologia Experimentalis Et Applicata 40(1):89–98. DOI: 10.1111/j.1570-7458.1986.tb02159.x.
- Tjallingii WF. 1994. Sieve element acceptance by aphids. European Journal of Entomology 91:47–52.
- Tjallingii WF. 2001. Plant penetration by aphids as revealed by electrical penetration graphs. Aphids and Other Homopterous Insects 8:105–120.
- Tjallingii WF. 2006. Salivary secretions by aphids interacting with proteins of phloem wound responses. Journal of Experimental Botany 57(4):739–745. DOI: 10.1093/jxb/erj088.
- Tjallingii WF, Esch THH. 1993. Fine structure of aphid stylet routes in plant tissues in correlation with EPG signals. Physiological Entomology 18(3):317–328. DOI: 10.1111/j.1365-3032.1993.tb00604.x.
- Togola A, Boukar O, Servent A, Chamarthi S, Tamo M, Fatokun C. 2020. Identification of sources of resistance in cowpea mini core accessions to Aphis craccivora Koch (Homoptera: Aphididae) and their biochemical characterization. Euphytica 216(6):88. DOI: 10.1007/s10681-020-02619-5.
- Van Hoof HA. 1958. An investigation of the biological transmission of a non-persistent virus. Doctoral thesis (Van Putten and Oortmijer.
- Vogelweith F, Thiéry D, Moret Y, Colin E, Motreuil S, Moreau J. 2014. Defense strategies used by two sympatric vineyard moth pests. Journal of Insect Physiology 64:54–61. DOI: 10.1016/j.jinsphys.2014.03.009.
- Wen J, Lu LM, Nie ZL, Liu XQ, Zhang N, Ickert-Bond S, Gerrath J, Manchester SR, Boggan J, Chen ZD.2018.A new phylogenetic tribal classification of the grape family (Vitaceae).Journal of Systematics and Evolution 56(4):262–272. Special Issue: Recent Advances in Systematics and Evolution of the Grape Family Vitaceae. DOI: 10.1111/jse.12427.
- Will T, Tjallingii WF, Thonnessen A, van Bel AJ. 2007. Molecular sabotage of plant defense by aphid saliva. Proceedings of the National Academy of Sciences of the United States of America 104(25):10536–10541. DOI: 10.1073/pnas.0703535104.
- Wróblewska A, Dancewicz K, Gabryś B, Kordan B. 2012. EPG-registered probing behaviour of the pea aphid (Acyrthosiphon pisum Harris) on selected varieties of yellow lupin (Lupinus luteus L.) and narrow-leaved lupin (Lupinus angustifolius L.). Progress In Plant Protection 52(2):244–247.
- Wróblewska-Kurdyk A, Gniłka R, Dancewicz K, Grudniewska A, Wawrzeńczyk C, Gabryś B. 2019. β-Thujone and its derivatives modify the probing behavior of the peach potato aphid. Molecules 24(10):1–16. DOI: 10.3390/molecules24101847.
- Wu D, Zeng L, Zhou A, Xu Y. 2013. Effects of Solenopsis invicta (Hymenoptera: Formicidae) tending on the probing behavior of Phenacoccus solenopsis (Hemiptera: Pseudococcidae). Florida Entomologist 96(4):1343–1349. DOI: 10.1653/024.096.0413.
- Zhang Y, Fan J, Francis F, Chen J. 2017. Watery saliva secreted by the grain aphid Sitobion avenae stimulates aphid resistance in wheat. Journal of Agricultural and Food Chemistry 65(40):8798–8805. DOI: 10.1021/acs.jafc.7b03141.