Abstract
Several important papers discussing phoretic dispersal in animals have appeared in recent years, but the nature and evolutionary significance of the phenomenon are still not fully understood. This article reviews the current knowledge of this behaviour in Uropodina mites, which disperse at the deutonymphal stage. We first examine the morphological and behavioural attributes of Uropodina deutonymphs involved in phoretic dispersal. We then provide a systematic review of the animals that carry Uropodina, and examine the carrier-specificity of 30 well-known species. Most species are associated with a single family of carriers, or at most, two families. The available information shows that phoretic deutonymphs of most species do not show any preference for male or female carriers. The spatial pattern of attachment of phoretic deutonymphs varies according to the identity of the carrier insect. The nature of deutonymph infestation depends on the size and morphology of both the mite and its carrier. Successful phoresy also depends on synchronisation of the life cycles of the mite and its carrier, but the temporal dynamics of phoresy remain particularly poorly studied. Phoresy has ecological and evolutionary consequences for both the Uropodina and their carriers. Future research on phoresy will benefit from studies of its evolutionary development, the reasons why beetles appear to be specially favoured as carriers, how phoretic mites affect the insect that carries them, and how the apparent global decline in insect populations is affecting their symbiotic mites.
1. Introduction
The term phoresy refers to a situation in which an individual of an animal species is carried by another species for a limited time for dispersal without any parasitic benefit. The major review of the subject by Binns (Citation1982) has recently been extended by Bartlow and Agosta (Citation2021), Noriega et al. (Citation2022), Seeman and Walter (Citation2023). Phoresy is widely distributed throughout the animal kingdom, but is most commonly seen in small invertebrates with limited natural mobility. It is especially common in mites and appears to have evolved independently several times (Hunter & Rosario Citation1988; Houck & OConnor Citation1991).
Despite many published articles, the true evolutionary significance of phoresy in mites remains largely unstudied. Phoresy was traditionally considered only as a means of dispersal, but many types of evidence now indicate a range of much deeper and more complex relationships that vary significantly between and within various mite groups (Noriega et al. Citation2022). In some mites, the phoretic interactions with insects turned out to be accidental, whereas in others, they were highly specific (Athias-Binche Citation1991). The effect of a mite on its carrier can be negative (Kotiaho & Simmons Citation2001), but there are also cases in which a symbiosis with phoretic mites can be beneficial for the carrier or its progeny (Springett Citation1968). Moreover, our knowledge of phoresy in many mite species is limited to their phoretic stages, and we often do not have a basic understanding of the interactions between mites and their carriers over their complete life cycle. More specific studies of phoresy are needed to identify its role in the evolution of many groups of invertebrates.
Studies of phoresy in Uropodina have a long history, but phoretic behaviour in this mite group was long regarded more like a scientific curiosity. The oldest record of phoresy in Uropodina is from Eocene (ca. 44–49 Ma) Baltic amber by Dunlop et al. (Citation2013). Fossil Uropodina, presumably representatives of the genus Uroobovella Berlese, Citation1903, were found to be attached to the longhorn beetles (Cerambycidae), and they are the oldest known representatives of this mite group.
Phoresy in Uropodina mites has been studied from taxonomic, faunistic, and ecological points of view. Most commonly, new species of Uropodina are described based on phoretic deutonymphs collected directly from their carriers, and faunistic articles have been compiled to list phoretic species with information on their carrier species in relation to a particular geographical area. Often, such articles include basic information on the attachment sites and deutonymph infestation. More recent studies have provided more detailed insight into the ecology of phoretic dispersal in Uropodina mites, particularly concerning the carrier range, infestation level, or seasonal dynamics.
Binns (Citation1982) summarised the situation in these terms “ … The problem, however, is to give form to the mass of information … ”. This comment was made about the wealth of available data, particularly concerning the enormous number of phoretic mite species and their carriers. Writing a review paper on phoretic dispersal in Uropodina is a real challenge, not only because of the availability of a large amount of information that needs synthesis but also because of the unstable and confusing taxonomy of this mite group (Halliday Citation2015, Citation2016). Another problem that hinders the analysis of phoresy in Uropodina is the constantly changing taxonomy of their carriers, which often makes it impossible to determine the extent of the carriers, even at the family level. Some of these difficulties regarding the studies on phoresy in mites have been recently indicated by Noriega et al. (Citation2022).
The present article is the first that reviews and summarises the current state of knowledge on the phoresy of Uropodina concerning species that disperse via deutonymphs. In the first part of this review, we summarise the current knowledge of the morphological and behavioural adaptations to phoresy. We then review various ecological aspects of phoresy in Uropodina, including their carrier specificity and attachment sites, and attempt to identify patterns of localisation on the carrier’s body. We identify three groups of factors that affect phoretic deutonymph infestation. We then examine the temporal dynamics of phoresy, which remain one of the most understudied aspects of this behaviour, and discuss the consequences of phoresy for Uropodina mites and their carriers. We also consider the possibility of practical applications of phoresy in Uropodina. Finally, we formulate some unanswered questions that are worth further research in this area.
The following discussion is based on a review of hundreds of papers on the ecology and taxonomy of the Uropodina. That body of literature is extremely variable in quality, and ranges from small taxonomic studies of one or a few species to extensive faunistic and behavioural studies and monographs. We do not present a rigorous meta-analysis of that literature, but instead we identify a series of general trends that help to clarify the nature of phoresy in the Uropodina, and its ecological and evolutionary consequences.
2. Uropodina mites
The Uropodina is a large and diverse species group of mites. The exact number of Uropodina species is difficult to determine and varies according to different authors, as a result of the confusing and unstable classification. The number of known Uropodina species could be estimated at nearly 2,300 worldwide (Beaulieu et al. Citation2011). A first step toward the development of stable classification in this mite group was made by Halliday (Citation2015, Citation2016), who provided details of 300 genus-group names and 47 family-group names that have been used in the Uropodina. The classification used here is based on Karg (Citation1989) and Mašán (Citation2001). That classification deals mainly with the European fauna and must be considered as provisional. We include the name Trichocyllibidae Hirschmann, Citation1979, which is a nomen nudum, but which we believe represents a useful taxonomic concept.
Uropodina mites can be found at any latitude except from polar regions, in various habitat types. Most species are edaphic and inhabit the upper surface of forest soil and forest litter. Soil is considered a primitive habitat of Uropodina, and from there, many species have adapted for living in ephemeral and patchily distributed microhabitats such as animal manure, carrion, compost heaps, decaying wood, tree holes, bird and mammal nests (Athias-Binche & Habersaat Citation1988; Athias-Binche Citation1993; Athias-Binche et al. Citation1993; Krištofík et al. Citation1993; Mašán Citation1993, Citation1994; Beaulieu Citation2012). Only a few species live in open habitats such as meadows and agricultural fields (Błoszyk Citation1999).
The body length of Uropodina ranges from 0.2 to 2 mm (Mašán Citation2001). The body is heavily sclerotised, triangular, round, oval, cylindrical, or fusiform. The legs are shorter than the body length and, in some species, can be withdrawn into special recesses, the so-called pedofossae. Due to their relatively short legs in comparison to their body size, Uropodina move slowly when compared to other mites, and in the literature they are often called tortoise mites.
Various types of feeding behaviour can be observed in Uropodina mites. Some species are saprophagous and feed on decomposing organic matter (Karg Citation1989). Other species are mycetophagous and feed on mushroom spores and mycelium (Faasch Citation1967; El-Banhawy et al. Citation1997; Constantinescu & Cristescu Citation2007). Predatory behaviour has been observed in a few species that feed on nematodes, fly larvae, other mites, or earthworms (Faasch Citation1967; El-Banhawy et al. Citation1997). Eggs and larvae of bark beetles are also included in a diet of several Uropodina species (Chaires-Grijalva et al. Citation2016). Some authors believe that predation in Uropodina is not a typical way of feeding because of their slow movement (Karg Citation1989; Mašán Citation2001). Faasch (Citation1967) stated that Uropodina feed mainly on weak and unwell individuals. It is also known that some species of Uropodina may use a variety of feeding resources (Faasch Citation1967; Karg Citation1989; Mašán Citation2001).
The life cycle of Uropodina includes five stages: an egg, larva, protonymph, deutonymph, and adult. Most species are bisexual with podospermic insemination. Sexual dimorphism is distinct and involves differences in the structure of the intercoxal region. Thelytokous parthenogenesis has been observed in some species. Species that reproduce without males are commonly those that inhabit soil and forest litter, whereas many species living in temporal microhabitats reproduce bisexually (Błoszyk et al. Citation2004).
Dispersal by phoresy is a critically important part of the life cycle of many species of Uropodina that inhabit ephemeral or patchily distributed microhabitats. Uropodina are tiny animals of limited mobility, and compensate for these limitations by using other organisms for dispersal. Phoresy allows them to colonise new microhabitats suitable for further development, feeding, breeding, and, consequently, species survival (Athias-Binche Citation1994). Phoresy allows mites to leave the colonised habitat when conditions become unfavourable due to overcrowding or physical deterioration. More information on the consequence of phoresy to mites and carriers is presented in chapter 14.
3. Morphological adaptations to phoresy
Phoresy at the deutonymphal stage is observed in representatives of the superfamilies Polyaspidoidea and Uropodoidea. In some species of Uropodina all deutonymphs are phoretic, for example Uropoda orbicularis (Müller, Citation1776). Other species, including Polyaspis patavinus Berlese, Citation1881, are polymorphic and can form two types of deutonymphs: a phoretic morph adapted for dispersal and a sedentary morph that lacks phoretic adaptations (Faasch Citation1967; Athias-Binche Citation1984). The most important morphological differences between these two deutonymphal forms concern the structure of the anal region and the structure of the pretarsus of the legs II–IV, as described in detail by Faasch (Citation1967) for Uroobovella marginata (Koch, Citation1840). The anal region in sedentary deutonymphs is similar to that of protonymphs and adults, whereas in phoretic deutonymphs, it is bigger and more heavily sclerotised, and can be closed by a flap. The flap is absent in sedentary deutonymphs. The pretarsus of legs II–IV is provided with a pair of claws and a pulvillus. In sedentary deutonymphs, the pulvillus is divided into lobes that enable deutonymphs to move around in the moist substrate. The same pretarsus structure is observed in larvae, protonymphs, and adults. In phoretic deutonymphs, the pulvillus is adapted for mite movement on the flat surface of its carrier’s body. The pulvillus forms a unitary lobe that can elevate in contact with the smooth surface of the carrier’s body and then flatten to improve attachment for the mite (Faasch Citation1967).
The most spectacular and unique morphological adaptation to phoresy in Uropodina is the ability to secrete an anal pedicel that allows the phoretic deutonymph to attach to the carrier (). The pedicel is a temporary structure whose only function is phoretic attachment, whereas mites from other groups attach themselves to their carriers using existing structures that also have other functions. For instance, females of Macrochelidae use their chelicerae, and deutonymphs of Parasitidae attach themselves by ambulacral claws. The anal pedicel of Uropodina functions in a similar way to the sucker plate in the specialised hypopus of some Astigmata (Farish & Axtell Citation1971). In most species of Uropodina, the pedicel looks like a straight stalk of variable length with flattened ends. One end of the pedicel adheres to the deutonymphal anus and the other to the carrier’s body surface. In some species, e.g., Uroobovella pulchella (Berlese, Citation1904b) (Bajerlein et al. Citation2013), Centrouropoda almerodai (Hiramatsu & Hirschmann, 1992) (in Wiśniewski et al. Citation1992b) (Farmahiny Farahani et al. Citation2016) or in Uropodina species collected from crustaceans (Rigby Citation1996; Pugh et al. Citation1997), the pedicels are very short and frequently look more like a clump of adhesive than a straight stalk. In contrast, pedicels of species in the Uroobovella nova (Oudemans, Citation1902) species complex can be helically coiled (), as observed by Faasch (Citation1967) for U. marginata. The pedicel shows a great inter- and intraspecific variability in its length. Among pedicels studied in four Uropodina species, the longest were secreted by deutonymphs of U. nova (av. 918.8 µm) and the shortest by U. pulchella (av. 13.0 µm), whereas deutonymphs of U. orbicularis (av. 330 µm) and Trichouropoda ovalis (Koch, Citation1840) (141.1 µm) formed pedicels of intermediate length (Bajerlein et al. Citation2013). Several factors have been identified as having an influence on pedicel length (Faasch Citation1967; Bajerlein & Witaliński Citation2014). Faasch (Citation1967) found that the initial length of the pedicel is affected by the ability of the mite to straighten its hind legs. During secretion of the pedicellar substance, the deutonymph of U. orbicularis gradually extends its hind legs as high as possible and forms a stalk-like pedicel. Eventually, the mite positions itself on the pedicel so that its legs do not make contact with the surface of the carrier. In turn, deutonymphs of U. marginata, after forming a pedicel, may run forward to extend its length. Other factors affecting pedicel length are the amount of produced pedicellar substance and food availability. Faasch (Citation1967) observed that the total produced secretions had been used for pedicel formation, and consecutive pedicels formed one by one by deutonymphs experimentally detached from carriers were shorter. If food is limited, the pedicels are shorter or are not produced at all. Pedicel length can also be affected by the location of the deutonymph on the carrier’s body (Bajerlein & Witaliński Citation2014). Deutonymphs of U. orbicularis attached to exposed body parts of an aphodiid beetle, e.g., the upper part of elytra, form longer pedicels when compared with pedicels produced by those fixed to a more concealed part of a beetle body, e.g., femora of the third pair of legs or apex of the elytra. Pedicel length in this species is also affected by the density of the attached deutonymphs (). The more attached deutonymphs to a limited surface area, the longer pedicels were produced. Longer pedicels maintain deutonymphs at a certain distance from the carrier surface, which enables more deutonymphs to disperse (Bajerlein & Witaliński Citation2014).
Figure 1. Phoretic Uropodina and their carriers. (a) deutonymph of Uropoda orbicularis Müller attached via pedicel to the hind femur of Onthophagus beetle (scale bar 500 µm). (b) detached deutonymph of Uroobovella sp., a species within a species complex of Uroobovella nova Oudemans, with helically coiled pedicel; a black arrow indicates carrier terminus of the pedicel (scale bar 500 µm). (c) pedicels and deutonymphs of U. orbicularis attached to the hind femur of Aphodius beetle; white arrows indicate pedicels of variable length (scale bar 1000 µm). (d) deutonymph of U. orbicularis; note the position of the mite - the legs of the second to fourth pair are hidden in the podofossae, and the legs of the first pair with elongated setae are exposed (scale bar 500 µm). (e) deutonymphs of U. orbicularis attached to a posterior part of the elytra of Aphodius fimetarius (Linnaeus). (f) deutonymphs of U. orbicularis attached to a posterior part of the elytra of Onthophagus coenobita (Herbst); note that the mites are attached close one to the other, although the remaining dorsal body side of the beetles is free (scale bar 2000 µm). (g) deutonymphs of Oodinychus ovalis (Koch) (= Trichouropoda ovalis (Koch)) attached to a lateral body part of the centipede (scale bar 1000 µm). (h) deutonymphs of Trichouropoda sociata (Vitzthum) attached to elytra of Plagionotus detritus (Linnaeus) (scale bar 2000 µm). (i) deutonymphs of T. sociata attached to the ventral surface of the prothorax of P. detritus (scale bar 1000 µm). (j) deutonymphs of Uroobovella sp. (a species within the complex species of Uroobovella nova) attached to the ventral prothorax of the Nicrophorus beetle (scale bar 2000 µm). All images were captured under the Olympus SZ61 stereomicroscope.
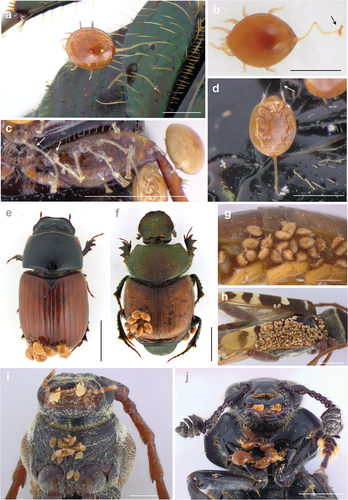
The substance that forms the pedicel is secreted by a pedicellar gland - a large, spherical, and slightly compressed organ, which is located in the posterior half of the body over the colon, between the ventriculus and postcolon. The gland bulges anteriad from the postcolon wall and consists of three types of glandular cells (A, B, and C types). Their apical parts are filled with secretion, whereas the basal parts contain nucleolus (Bajerlein & Witaliński Citation2012). The pedicel substance is fluid but hardens in contact with the air. Nevertheless, the pedicel is elastic, which enables the deutonymph to walk on the carrier’s body surface during the phoretic dispersal and to detach after reaching a new habitat. Faasch (Citation1967) has observed that pedicels may increase their length several times when stretched, and after the force has been removed, shrink back to their initial length. After reaching a new suitable habitat for further development, the deutonymph detaches from its carrier, and the pedicel usually remains on the carrier body. A single phoretic deutonymph may produce many pedicels in its life (Faasch Citation1967).
Some phoretic species do not form an anal pedicel. Phoretic deutonymphs of P. patavinus and Apionoseius infirmus (Berlese, Citation1887) (= Uroseius infirmus (Berlese, Citation1887) attach themselves using an anal disc (Athias Citation1976). Similarly, Gómez-Marco et al. (Citation2021) did not observe formation of a pedicel in Dinychus sp. phoretic on the South American palm weevil Rhynchophorus palmarum (Linnaeus, 1758). Uropodina associated with ants have developed some unusual methods of attachment to their carriers. Adults in the genera Planodiscus Sellnick, Citation1926, Circocylliba Sellnick, Citation1926, Trichocylliba Berlese, Citation1904c, Coxequesoma Sellnick, Citation1926, and Antennequesoma Sellnick, Citation1926 have developed effective holdfast mechanisms that enable them to attach to their army ant hosts. Elzinga (Citation1978) identified four types of holdfast mechanisms – (1) claws and empodial suckers, (2) toothed cuticular holdfasts, (3) ridged cuticular holdfasts, and (4) everted cuticular holdfasts. Claws and empodial suckers can be observed in all species in this group, and in Circocylliba and Trichocylliba they are the mite’s only means of attachment to the host (Elzinga Citation1978). Tarsal claws on legs II–IV are used for grasping the ant’s setae, whereas the empodia are used for attaching to other parts of the ant’s body. The toothed cuticular holdfast is found in Planodiscus and Coxequesoma. Species of Planodiscus possess short-toothed lateral lobes that are located anteriorly and attach to the ventral base of the ant’s tibia. The anterior dorsal prolongation of the idiosoma fits into the femoro-tibial joint of the carrier. In Coxequesoma, the entire lateral dorsum is expanded ventrally and creates a cavity that encloses structures on the ant’s body such as the coxae and nota. Mites in the genus Antennequesoma have evolved a ridged cuticular holdfast that can attach to the antennae or legs of ants. The dorsum has grown over the venter to make a hollowed area ventrally. The ridges that fasten the mite to its carrier extend from the anterior ventral border to beyond the terminus of the lateral flanges. The everted cuticular holdfast is similar to the claspers found in the hypopus of Labidophorus (Astigmata). Images of various types of the holdfast mechanism can be found in Elzinga (Citation1978).
Other morphological adaptations to phoresy are related to reducing the risk of water loss during dispersal and lack of food (Faasch Citation1967). The deutonymph withdraws its legs into the pedofossae, and also withdraws its gnathosoma into the camerostome. These are behavioural adaptations for phoresy, which reduce the animal’s surface area and therefore reduce water loss. They probably also reduce the risk of physical injury (Atalla & Hobart Citation1964; Athias-Binche Citation1981, Citation1984). Deutonymphs of Uropodina do not feed during phoretic dispersal. Experiments conducted by Faasch (Citation1967) showed that non-phoretic deutonymphs died after a 60-day period of starvation, while phoretic individuals not only survived but were also able to produce pedicels.
4. Behavioural adaptations to phoresy
Phoretic Uropodina have evolved adaptations for dispersal that include the following sequence of behaviours – active carrier seeking, carrier recognition, attachment to the carrier surface, and detachment (Athias-Binche Citation1994). Faasch (Citation1967) has observed that phoretic deutonymphs are more active than sedentary deutonymphs. Moreover, phoretic deutonymphs are less susceptible to sunlight, which allows them to gather on the surface of the drying substrate and wait for the carrier.
According to Faasch (Citation1967), smell is the phoretic deutonymph’s primary sensory cue for searching for a carrier. Olfactory organs are situated on the tarsi of the first pair of legs and on the pedipalps. After finding the carrier, the deutonymph climbs onto it and starts searching for an appropriate place for attachment. It then presses its anus onto the attachment surface and starts secreting the pedicel. During secretion of the pedicel material, the deutonymph straightens its hind legs as high as possible and the secreted substance forms a stalk-like pedicel. After forming the pedicel, the deutonymph withdraws its legs into the pedofossae and is transported into a new habitat. During the dispersal phase the deutonymph sits on the pedicel, which constitutes the link between the mite and its carrier. Sometimes, after secretion of the pedicel, the deutonymph runs forward to elongate the pedicel. In such cases, the pedicel is a kind of leash that connects the mite with its carrier but also enables the deutonymph to walk on its body (Faasch Citation1967).
When the carrier insect reaches a new habitat, the deutonymphs start the detachment process. According to Faasch (Citation1967), detachment depends on proper humidity. In low humidity, the pedicel loses its elasticity, making deutonymph detachment impossible. The deutonymph must also respond to olfactory stimuli from the new habitat. These stimuli must be more attractive for the mite than the stimuli coming from the carrier. When detaching from its carrier, the mite swings forward and straightens its legs, which makes contact with the carrier’s body surface possible. Then the deutonymph runs forward, which causes detachment of the pedicel from the deutonymph’s anus. Sometimes the pedicel detaches directly from the carrier surface, so the deutonymph remains joined with it. The detached deutonymph leaves its carrier and starts searching for food in the new habitat (Faasch Citation1967).
5. Phoretic species of Uropodina
It is very difficult to estimate the exact number of phoretic species of Uropodina. First, the confusing taxonomy of this mite group creates difficulties in species identification. The same mite species may be named differently by various authors using different nomenclature systems in their studies. Second, the ability to recognise phoresy in particular species could be confused by the use of different criteria. Many species are considered to be phoretic because their deutonymphs were collected directly from their carriers. Others are assumed to be phoretic because their deutonymphs show morphological adaptations for phoretic dispersal, although they were never collected from any carriers. In some species of Uropodina, authors indicate their associations with a given species of animal, but it is not clear whether the association involves the observation of phoretic deutonymphs on the surface of an insect’s body, or simply the presence of mites in the insect’s nest. Many species of Uropodina are associated with the galleries of wood-inhabiting beetles or the nests and burrows made by other insects but phoresy has not been observed directly, so the number of phoretic species of Uropodina is probably higher than is supposed.
Most species of Uropodina that have a phoretic deutonymphal stage are grouped within the family Trematuridae, followed by the families Urodinychidae and Uropodidae. Other families with species using deutonymphs for dispersal are Trachytidae, Dinychidae, Uroactiniidae, Polyaspididae, Metagynuridae, and Discourellidae. At the genus level, the highest number of phoretic Uropodina are found in Trichouropoda Berlese, Citation1916b (Knee et al. Citation2012a), Nenteria Oudemans, Citation1915 (Matos et al. Citation2023), Uroobovella Berlese, Citation1903 (Matos et al. Citation2023), and Uropoda Latreille, Citation1806 (Bajerlein & Witaliński Citation2014). Lower numbers of species with phoretic deutonymphs are found in the genera Dinychus Kramer, Citation1886 (Gómez-Marco et al. Citation2021), Discourella Berlese, Citation1910 (Mašán Citation1999), Metagynella Berlese (in Trouessart & Berlese Citation1919) (Napierała & Błoszyk Citation2013), Polyaspis Berlese, Citation1881 (Hirschmann & Kemnitzer Citation1989), Uroactinia Zirngiebl (in Sellnick Citation1958) (Domrow Citation1981), and Uroseius Berlese, Citation1888a (Gwiazdowicz et al. Citation2013).
Phoretic adults are occasionally observed in Uropodina, in species of Oplitis Berlese, Citation1884, Urodiscella Berlese, Citation1903 (Oplitidae), Circocylliba, Trichocylliba, Planodiscus, Coxequesoma, Antennequesoma (Trichocyllibidae), and Trachyuropoda Berlese, Citation1888b (Trachyuropodidae). All of these species appear to be associated with ants (Elzinga Citation1978; Lehtinen Citation1987; Moser & Blomquist Citation2011; Campbell et al. Citation2013), and may be involved in unusual complicated ecological relationships.
6. Carriers of Uropodina
Uropodine mites use various co-existing animals for phoretic dispersal, but mainly arthropods (Table S1). Phoretic deutonymphs have been observed on insects (e.g., Athias-Binche Citation1984; Athias-Binche et al. Citation1993; Knee et al. Citation2012a, Citation2013; Dunlop et al. Citation2013), arachnids (e.g., Poinar et al. Citation1998), crustaceans (e.g., Rigby Citation1996; Pugh et al. Citation1997), myriapods (e.g., Błoszyk et al. Citation2006), reptiles (e.g., Mertins & Hartdegen Citation2003), and mammals (e.g., Miko & Stanko Citation1991). Insects are the main carrier group and the other animals serve as carriers much less frequently. Among the insects, phoretic deutonymphs of Uropodina have been recorded most frequently on Coleoptera (e.g., Faasch Citation1967; Athias-Binche Citation1984; Kofler & Schmölzer Citation2000; Mašán Citation2001; Bajerlein & Błoszyk Citation2004; Knee et al. Citation2012a, Knee et al. Citation2013; Zach et al. Citation2016), then on Hymenoptera (e.g., Gordh & Barrows Citation1976; Pereira et al. Citation2016), and Diptera (e.g., Fain & Greenwood Citation1991; McGarry & Baker Citation1997; Rodrigueiro & Do Prado Citation2004). Phoretic deutonymphs have also occasionally been collected from other groups of insects, including Lepidoptera (Helson et al. Citation1975), Dermaptera (Hirschmann Citation1985), Isoptera (e.g., Phillipsen & Coppel Citation1978), Hemiptera (e.g., Athias-Binche Citation1983; Kofler & Schmölzer Citation2000), Orthoptera (e.g., Kofler & Schmölzer Citation2000), and Siphonaptera (e.g., Schwan & Corwin Citation1987; Błoszyk & Bajaczyk Citation1999).
For many reasons, it is difficult to determine the exact number of hosts that carry Uropodina. Many authors do not identify the carrier to the species level but give only its generic or family name. Even when a carrier is identified, its generic or family name may change because animal systematics is constantly changing and the same taxon may include different species at different times. An example is the use of the term “dung beetles” and “scarabaeid beetles”, as previously mentioned by Noriega et al. (Citation2022). In some systems of classification the family Scarabaeidae contains both dung beetles and strictly phytophagous species, but in others it contains only the true dung beetles. When a mite carrier has been determined as a representative of the family Scarabaeidae, that could refer to a species from different ecological groups. Moreover, it is often not known if the carrier has been identified by a beetle specialist or by the acarologist, which raises questions about the reliability of the identification. In this paper, we give the current carrier species name according to the Catalogue of Palaearctic Coleoptera (Löbl & Smetana Citation2007, Citation2010, Citation2011, Citation2013; Löbl & Löbl Citation2015, Citation2016, Citation2017; Danilevsky Citation2020; Iwan & Löbl Citation2020).
6.1. Coleoptera
Beetles are the primary carrier group of Uropodina, both in terms of the number of species of mites they carry, and the number of species of insects that carry mites. Among them, the bark beetles in the subfamily Scolytinae (family Curculionidae) play the most significant role in the dispersal of phoretic deutonymphs (e.g., Moser Citation1976a; Moser et al. Citation1989, Citation2005; Knee et al. Citation2012a, Citation2013; Paraschiv et al. Citation2018; Paraschiv & Isaia Citation2020). After the weevils, the long-horn beetles (Cerambycidae) (e.g., Kinn & Linit Citation1989; Knee et al. Citation2012c; Gwiazdowicz et al. Citation2013; Konwerski et al. Citation2017, Citation2019, Citation2020), and scarabaeid beetles, including dung and phytophagous beetles (e.g., Costa Citation1963; Faasch Citation1967; Ramsey Citation1967; Bajerlein & Błoszyk Citation2004; Mašán & Halliday Citation2009; Bajerlein Citation2011), are also significant carrier groups of Uropodina. Phoretic deutonymphs of Uropodina have been also found on beetles in the families Brentidae (Hirschmann & Wiśniewski Citation1985; Wiśniewski & Hirschmann Citation1991), Carabidae (Desender & Vaneechoutte Citation1984), Elateridae (Gordh Citation1985; Kofler & Schmölzer Citation2000), Histeridae (Gordh & Wills Citation1989; Bajerlein Citation2011), Hydrophilidae (Bajerlein & Przewoźny Citation2005), Staphylinidae including Silphinae (Athias-Binche et al. Citation1993; Knee et al. Citation2012b), Tenebrionidae (Moser & Roton Citation1971; Gordh & Wills Citation1989; Pfammatter et al. Citation2016a), and Trogidae (Stewart & Davis Citation1967; Philips Citation2009). Uropodines have also occasionally been found on beetles in the families Passalidae (Hirschmann Citation1981; Wiśniewski & Hirschmann Citation1992; Wiśniewski et al. Citation1992a), Lucanidae (Athias-Binche Citation1993; Mašán Citation2001), Buprestidae (Kofler & Schmölzer Citation2000) and Lymexylidae (Mašán Citation2001). The presence of phoretic Uropodina on other beetle families, such as Chrysomelidae (Kofler & Schmölzer Citation2000), Coccinelidae (Klausnitzer Citation2018), Monotomidae (Kofler & Schmölzer Citation2000), or Cleridae (Pfammatter et al. Citation2016a), is much less common and may be accidental.
6.2. Hymenoptera
The most conspicuous group of Hymenoptera associated with Uropodina is the ants in the family Formicidae. Phoresy of Uropodina on ants is especially interesting since in many mite species the deutonymph is phoretic, while in others it is the adult (e.g., Elzinga Citation1978; Eickwort Citation1990; Campbell et al. Citation2013).
Phoretic deutonymphs of Uropodina have been observed on Hymenoptera in the families Apidae, Braconidae, Crabronidae, Formicidae, Pteromalidae, and Scoliidae (Moser & Roton Citation1971; Hirschmann & Kemnitzer Citation1989; Kofler & Schmölzer Citation2000; Kamczyc & Gwiazdowicz Citation2013). Phoresy via deutonymphs on Hymenoptera has been observed in the genera Dinychus (Kofler & Schmölzer Citation2000), Discourella (Kamczyc & Gwiazdowicz Citation2013), Nenteria (Kofler & Schmölzer Citation2000; Kamczyc & Gwiazdowicz Citation2013), Oodinychus Berlese, Citation1917 (Wiśniewski & Hirschmann Citation1991), Phaulodinychus Berlese, Citation1904a (Lehtinen Citation1987), Trachytes Michael, Citation1894 (Kofler & Schmölzer Citation2000), Trichouropoda (Moser & Roton Citation1971; Kamczyc & Gwiazdowicz Citation2013), Uroobovella (Chmielewski Citation1998; Kuhlmann Citation1998), Urodiaspis Berlese, Citation1916a (Kofler & Schmölzer Citation2000), Uropoda (Kofler & Schmölzer Citation2000), and Polyaspis (Hirschmann & Kemnitzer Citation1989).
6.3. Diptera
Phoretic deutonymphs of Uropodina have been found on flies from the families Bibionidae, Fanniidae, Muscidae, Phoridae, Syrphidae, Spheroceridae, and Tachinidae (Karg Citation1989; Fain & Greenwood Citation1991; McGarry & Baker Citation1997; Fain Citation1998a, Citation1998b; Kofler & Schmölzer Citation2000; Mašán Citation2001; Rodrigueiro & Do Prado Citation2004; Perotti & Braig Citation2009). There are also recorded cases of phoresy of Uropodina on unidentified Diptera (Karg Citation1989; Kofler & Schmölzer Citation2000; Mašán Citation2001). The most detailed information on phoretic Uropodina on flies can be found for species of Uroseius Berlese, Citation1888a (Fain & Greenwood Citation1991; Rodrigueiro & Do Prado Citation2004). Phoretic interactions with Diptera have also been observed in representatives of the genera Dinychus (Karg Citation1989; Wiśniewski & Hirschmann Citation1993a; Kofler & Schmölzer Citation2000), Polyaspis (Kofler & Schmölzer Citation2000), Prodinychus Berlese, Citation1917 (McGarry & Baker Citation1997), Trachyuropoda (Karg Citation1989), Urodiaspis (Kofler & Schmölzer Citation2000), Uroobovella (Fain & Greenwood Citation1991; Wiśniewski & Hirschmann Citation1993a; Mašán Citation2001), and Uropoda (Kofler & Schmölzer Citation2000), but these were infrequent.
6.4. Why beetles?
The information listed above suggests two questions about phoresy in Uropodina – first why beetles are the primary carriers of Uropodina, and second, why are weevils and longhorn beetles the most important carriers among the beetles. The primary condition required for the evolution of any interactions between mites and their carriers is that they must co-occur temporally and spatially. Therefore, one of the possible explanations is simply that beetles are the most numerous animals that have adapted to living in a wide range of diverse habitats; hence the probability of meeting a beetle by a mite and using it as a carrier is high. Many species of Uropodina characterised by phoretic behaviour live in short-term temporary habitats such as animal manure or carrion, and long-term temporary resources, such as various kinds of wood habitats, including living, weakened, and dead wood. Beetles and flies are stable components of many invertebrate communities associated with these unstable microhabitats. Nevertheless, as previously suggested, Uropodina could prefer beetles for dispersal because flies are more difficult carriers, due to their fast movement and more heavily setose body surface that impedes the attachment of the pedicel (Faasch Citation1967; Bajerlein et al. Citation2016).
Differences in the number of carrier species of Uropodina between particular beetle families do not necessarily indicate their different roles in the dispersal of Uropodina, but may reflect a different state of knowledge in this area. For instance, the common observation of Scolytinae as carriers of Uropodina could be explained by the fact that bark beetles are one of the best-studied groups of Coleoptera, due to their economic importance. Knee et al. (Citation2013) studied phoretic associations between Uropodina and Scolytinae, and pointed out that previous studies have not paid attention to associations of Uropodina with other families of xylophagous beetles.
The significant role of xylophagous beetles in the dispersal of Uropodina may be related to the fact that most phoretic species are associated with wood habitats. Although the resources colonised by phoretic Uropodina are referred to as unstable microhabitats, they differ in size and duration. Weakened, damaged, dying, and dead trees are larger and last much longer than animal dung, carrion, or compost. They offer a much wider range of diverse niches, which may explain the high number of Uropodina species in this habitat.
Nevertheless, previous studies conducted worldwide suggest that the simple co-occurrence of animals that may serve as carriers is insufficient for the development of phoretic interactions. For instance, species within the Uroobovella nova species complex have adapted for dispersal on burying beetles (Silphinae: Nicrophorus spp.) but not on other silphid beetles, i.e., representatives of the genus Thanatophilus Leach, 1815, which also colonise small animal carrion (Kočárek Citation2003).
7. Carrier specificity
The occurrence of deutonymphs of Uropodina attached to insects is very commonly reported in the literature (e.g., Fain & Greenwood Citation1991; Rigby Citation1996; Pugh et al. Citation1997; Pernek et al. Citation2008; Philips Citation2009; Bahrami et al. Citation2011; Gwiazdowicz et al. Citation2011; Dunlop et al. Citation2013; Moraza et al. Citation2013; Paraschiv & Isaia Citation2020). However, conclusions about carrier specificity can be drawn only for some of the better known species of Uropodina (e.g., Moser et al. Citation1989, Schwarz et al. Citation1998; Philips Citation2009; Porcelli et al. Citation2009; Bajerlein Citation2011; Knee et al. Citation2013; Napierała et al. Citation2015; Pfammatter et al. Citation2016a; Zach et al. Citation2016). In this section we examine the carrier specificity of some of the more thoroughly studied phoretic species of Uropodina ().
Table I. Categories of phoresy in Uropodina mites according to the carrier range.
The classification of phoretic interactions between mites and their hosts as specific or non-specific may differ among individual authors and depends on the criteria adopted (Athias-Binche Citation1991, Citation1994; Camerik Citation2010; Noriega et al. Citation2022). In addition, conclusions about carrier specificity are often not drawn from an analysis of the full range of carriers associated with the habitat of a given mite species, but instead reflect the current state of knowledge of the species involved. Athias-Binche (Citation1991, Citation1994) classified phoretic relationships as euryxenous, eco-behavioural, stenoxenous, and oioxenous. Phoresy is euryxenous (non-specific) when the mite uses a wide variety of carriers for dispersal enabling its colonisation of various habitats. Phoresy is termed eco-behavioural or eco-ethological when the mite uses a variety of carriers that co-exist in a specific microhabitat. This category of phoresy is observed in mites inhabiting microhabitats such as cattle dung, carrion, animal nests, or mushrooms. Stenoxenous (specific) phoretic relationships are those in which a mite species uses multiple species of carriers that belong to a particular family or genus and occur in the same microhabitat. Finally, oioxenous (monospecific) phoresy refers to the situation where the mite uses only one or a few very closely related species for dispersal. According to Athias-Binche (Citation1991), the last two categories of phoresy include phylogenetic relationships, whereas eco-behavioural phoresy does not. Camerik (Citation2010), however, suggested including phylogenetic relationships in the eco-behavioural phoresy, since mite preference towards the carrier is restricted to one or few different insect orders. Therefore, a common evolutionary history may be expected even in this category of phoresy (Camerik Citation2010).
To determine carrier specificity in Uropodina mites we reviewed 30 species, including the Uroobovella nova species complex, according to criteria proposed by Athias-Binche (Citation1991). Among the Uropodina, only Uroobovella marginata has been classified as a non-specific (or euryxenous) species since its deutonymphs were observed on carriers from various phylogenetically and ecologically diverse groups such as beetles, flies, hymenopterans, isopods, reptiles, and mammals (Table S1). It was frequently observed in animal manure and on the body surface of dung beetles by Faasch (Citation1967), but it is also commonly recorded as phoretic on the red palm weevil Rhynchophorus ferrugineus (Olivier, 1970) (e.g., Dilipkumar et al. Citation2015). These observations must be interpreted with care, because the name U. marginata has probably been applied to multiple different species (Wiśniewski & Hirschmann Citation1993a).
Phoresy in many Uropodina species is often eco-behavioural, with the mites using different sympatric carriers for dispersal. It should be noted, however, that deutonymphs of such species may occur much less rarely on other animals, particularly if they appear accidentally in their environments. Uropoda orbicularis is an example of a well-known mite species with eco-behavioural phoresy (Bajerlein & Błoszyk Citation2004; Bajerlein Citation2011). Long-term studies of phoresy of this mite conducted on a local pasture grazed by cattle in Poland have revealed that phoretic deutonymphs were found on almost all species of dung beetles (Scarabaeidae, Geotrupidae), as well as the most abundant species of Histeridae in the same habitat (Bajerlein & Błoszyk Citation2004; Bajerlein Citation2011). It was also found on hydrophilid beetles (Hydrophilidae) and rove beetles (Staphylinidae) (Bajerlein & Przewoźny Citation2005; Bajerlein Citation2007). Although various authors have recorded deutonymphs of U. orbicularis on beetles from other families, e.g., carabids, trogids, or passalids, on myriapods, and even on small mammals (Cricetidae, Muridae), their presence on representatives of these animal groups seems to be rather accidental (Wiśniewski Citation1979a; Mašán Citation1993; Kofler & Schmölzer Citation2000; Barrios-Torres & Villegas-Guzmán Citation2015). Similarly, we have classified Oodinychus ovalis (= Trichouropoda ovalis) as a species with eco-behavioural phoresy. Although this species was observed on animals from various taxonomic groups, its main carriers are beetles associated with different kinds of wood habitats.
The other most frequently observed ecological category of phoresy is stenoxenous phoresy. This type of phoretic interaction occurs in Trichouropoda polytricha (Vitzthum, Citation1923) and Uroobovella americana Hirschmann, Citation1972b, which use beetles from the family Curculionidae, or in Trichouropoda shcherbakae Hirschmann, Citation1972a which is phoretic on longhorn beetles (Cerambycidae).
We have identified two species of Uropodina that appear to show oioxenous phoresy. For a long time, the phoretic deutonymphs of Uropodina observed on the burying beetles (Nicrophorus spp.) were classified as a single species Uroobovella nova. However, Knee et al. (Citation2012b) found that U. nova represents at least five morphologically similar species with much narrower carrier ranges than previously thought. Another species with a highly specific phoresy is Centrouropoda almerodai, whose phoretic interactions with the red palm weevil R. ferrugineus have been best studied in Italy (e.g., Mazza et al. Citation2011). Monzenga Lokela et al. (Citation2021) also recorded deutonymphs of this species from Rhynchophorus phoenicis (Fabricius, 1801) collected in Africa. Although some authors have also identified deutonymphs of C. almerodai from Rhynchophorus palmarum, studies by Kontschán et al. (Citation2014b) and Gómez-Marco et al. (Citation2021) suggest that deutonymphs of the genus Centrouropoda found on this palm weevil represent other species.
Our review of phoretic Uropodina mites shows that only a few species are non-specific or highly-specific in their carrier range. The carrier range of most Uropodina includes species from a few families or a single family. If the main function of phoresy is dispersal, a strategy of using a wide range of co-existing carriers should enhance the probability of colonisation of new sites for feeding and reproduction. Knee et al. (Citation2013) found that many species of mites phoretic on bark beetles (Scolytinae) are generalists at a global scale but are host-specific at a local scale. A different situation is found in the coprophilous U. orbicularis, whose phoretic deutonymphs were locally found on 55 beetle species inhabiting cow dung (Bajerlein & Błoszyk Citation2004; Bajerlein Citation2007, Citation2011). The difference in carrier specificity between Uropodina associated with wood habitats and those colonising animal dung may be due to differences in the stability of the habitat. Decomposing wood offers more stable conditions for mites than the very ephemeral dung of herbivorous mammals. The larger number of carrier species used by U. orbicularis may allow it to respond quickly enough to rapidly changing environmental conditions.
The proposed classification of Uropodina according to their carrier specificity is not perfect, as it was developed solely based on the analysis of the carrier range. To differentiate main carriers from accidental carriers, another step is needed which requires the determination of the level of infestation of phoretic deutonymphs on different carriers. It should be emphasised, however, that mite infestation should be estimated as a result of long-term studies of a local animal community exploring a given type of habitat colonised by a mite. Such an approach will allow us to assess other basic characteristics of this community, such as temporal changes in carrier species composition and variation in the abundance of both mites and their carriers. Such information will be crucial for the interpretation of carrier infestation levels, which should be the next step in the recognition of carrier specificity. For instance, Bajerlein (Citation2011) has shown that differences in infestation of particular species of coprophilous beetles with phoretic deutonymphs of U. orbicularis should not be interpreted as a preference toward carrier species, because they are caused by seasonal changes in beetle abundance. The highest level of deutonymph infestation was observed for Onthophagus spp., simply because these beetles were present at a time when the abundance of beetles decreased but the abundance of phoretic deutonymphs was relatively high. Since phoretic deutonymphs had fewer carriers to use, beetles present at that time were more heavily infested. A general approach to the estimation of the carrier specificity in mites has been presented in Fig. S1.
This is the first attempt to determine the carrier specificity in so many diverse Uropodina species. Along with increasing knowledge, the ecological classification of phoresy of at least some of these species may be subject to change as the taxonomy of both the mites and their carriers is revised.
For example, Uroobovella nova was thought to be phoretic on multiple species of carrion beetles in the genus Nicrophorus. Knee et al. (Citation2012b) showed that the species known as “Uroobovella nova” is actually a complex of cryptic species, each of which has a very narrow host range. The same may be true for Uroobovella orri Hirschmann, Citation1972b, but is apparently not true for several other species of generalists examined by Knee et al. (Citation2012a).
8. The range of carried species of Uropodina
The carrier range of phoretic species of Uropodina is commonly discussed in the literature but studies of phoretic interactions from the perspective of the carrier are less common (Knee et al. Citation2012c; Zach et al. Citation2016). Knee et al. (Citation2013) studied oribatid and mesostigmatic mites phoretic on bark beetles in Canada and concluded that a single carrier species was usually associated with one, two, or more rarely three, species of dispersing Uropodina. The same was true for phoretic interactions between Uropodina and bark beetles in Europe, and for Uropodina that use other types of carriers. The best-known carriers are associated with only one species of Uropodina (e.g., Bajerlein & Błoszyk Citation2004; Philips Citation2009; Konwerski et al. Citation2020). Less often carriers hosted two, three, or four species of Uropodina (e.g., Gwiazdowicz & Gutowski Citation2012; Knee et al. Citation2012c, Citation2013; Błoszyk et al. Citation2013; Zach et al. Citation2016; Konwerski et al. Citation2017, Citation2020). Examples of a single carrier that serve as a means of transport for multiple species of Uropodina, with extreme cases of 10 species, are rare and were usually observed for bark beetles (Table S1). Nevertheless, high numbers of species of Uropodina transported by a single carrier species can be observed at a global scale. Many studies have revealed that local assemblages are less complicated and include most frequently up to four Uropodina species transported by a single carrier species (e.g., Gwiazdowicz et al. Citation2011; Čejka & Holuša Citation2014a; Zach et al. Citation2016; Paraschiv & Isaia Citation2020).
Previous studies have shown two patterns of dominance structure of phoretic assemblages of Uropodina (i.e., a group of species phoretically associated with a given carrier species). In the first case one species of Uropodina is very strongly dominant (≥90%). For instance, Zach et al. (Citation2016) and Kršiak et al. (Citation2010) observed four-species assemblages of phoretic Uropodina on Hylastes cunicularius Erichson, 1836 (Curculionidae) where deutonymphs of Trichouropoda pecinai Hirschmann and Wiśniewski, Citation1986 accounted for over 90% and Trichouropoda obscura (Koch, Citation1835) making up less than 10% of the mites carried. Other observed species such as Uroobovella ipidis (Vitzthum, Citation1923) and Uroobovella vinicolora (Vitzthum, Citation1926) were accidental or were absent in some localities. Interestingly, Zach et al. (Citation2016) observed a reversed dominance structure of T. obscura and T. pecinai, with 99.5% and 0.5%, respectively on Hylurgops palliatus (Gyllenhal, 1813) (Curculionidae). Khaustov et al. (Citation2016) found Trichouropoda polytricha and U. ipidis phoretically associated with Pityogenes chalcographus (Linnaeus, 1760) in Siberia, with deutonymphs of the first species reaching 99.3% of all collected deutonymphs. In Poland, 43 species of coprophilous beetles, including dung beetles, clown beetles, and rove beetles within a local community carried only deutonymphs of a single species, Uropoda orbicularis. Nine beetle species hosted deutonymphs of two Uropodina species, three beetle species carried deutonymphs of three species of Uropodina, and one beetle species was found to serve as a means of transport for four species of Uropodina (Bajerlein Citation2007). While over 36,000 cases of phoresy of U. orbicularis have been found, deutonymphs of the remaining Uropodina species were accidental and accounted for 0.1% of all Uropodina deutonymphs collected (Bajerlein Citation2007).
In the second pattern of dominance structure in assemblages of phoretic Uropodina the most numerous species makes up less than 85% of all collected deutonymphs. This type of dominance structure can be observed in Uropodina phoretic on the spruce bark beetle; Ips typographus (Linnaeus, 1758). This beetle can locally carry 2–6 species of Uropodina, but most frequently there are 3–4 species (Moser et al. Citation1997; Feketeová Citation2011b; Gwiazdowicz et al. Citation2011; Čejka & Holuša Citation2014a; Holuša & Čejka Citation2020; Paraschiv & Isaia Citation2020). Trichouropoda polytricha is the most stable component of assemblages of Uropodina phoretic on the spruce bark beetle. Depending on the research area it co-occurs with deutonymphs of or Uroobovella obovata (Canestrini & Berlese, Citation1884) (Gwiazdowicz et al. Citation2011, Citation2015) U. ipidis (Moser et al. Citation1989, Citation1997; Gwiazdowicz et al. Citation2012; Čejka & Holuša Citation2014a; Holuša & Čejka Citation2020; Paraschiv & Isaia Citation2020). Trichouropoda polytricha is usually more abundant than U. obovata where they occur together (Gwiazdowicz et al. Citation2011, Citation2015), while in the interactions with U. ipidis a predominance of one or the other species was found (Moser et al. Citation1997; Feketeová Citation2011a; Gwiazdowicz et al. Citation2012). In the Czech Republic (Čejka & Holuša Citation2014a), Slovakia (Feketeová Citation2011b), and Romania (Paraschiv & Isaia Citation2020), T. polytricha and U. ipidis were accompanied by deutonymphs of U. vinicolora, which was always the least abundant species. Less often, the phoretic assemblages of Uropodina associated with I. typographus included only T. polytricha and U. vinicolora (Penttinen et al. Citation2013; Zach et al. Citation2016).
Farmahiny Farahani et al. (Citation2016) observed a similar relationship in the dominance structure of phoretic Uropodina on the red palm weevil Rhynchophorus ferrugineus in Iran, where Centrouropoda almerodai made up 72.8% of collected deutonymphs, and Uroobovella marginata the other 27.2%. The South American palm weevil Rhynchophorus palmarum carried three Uropodina species – Centrouropoda sp. (60.8%), Dinychus sp. (10.5%), and Uroobovella marginata (28.7%) (Gómez-Marco et al. Citation2021).
Questions arise about the factors that shape the structure of assemblages of Uropodina phoretic on individual carrier species. In particular, why do some carrier species carry only one species of Uropodina locally, while others are associated with two or more phoretic species? Why do we observe differences in abundances between Uropodina species even within phoretic assemblages with species-stable composition?
Previous observations have shown that the locality and the year of the study affect assemblages of phoretic Uropodina (e.g., Knee et al. Citation2012c; Pfammatter et al. Citation2013; Vissa et al. Citation2019). For instance, most European studies have revealed that the numbers of deutonymphs of T. polytricha phoretic on I. typographus were higher when compared with the numbers of deutonymphs of U. ipidis, or that their abundances were similar. Moser et al. (Citation1997), however, have observed the presence of T. polytricha and U. ipidis on I. typographus in Japan, with the latter species reaching 94.4% of all collected deutonymphs of Uropodina. Knee et al. (Citation2012c) found differences in numbers between deutonymphs of Trichouropoda lamellosa Hirschmann, Citation1972a and Trichouropoda hirsuta Hirschmann, Citation1972a phoretic on a longhorn beetle Monochamus scutellatus (Say, 1824) in Canada, according to the locality and date of the study. In turn, Gómez-Marco et al. (Citation2021) suggested that the observed differences in abundance of F. marginata and Centrouropoda sp. phoretic on R. palmarum may result from their competitive behaviour. Feketeová (Citation2011b) suggested that the body size of mites is one of the factors affecting the structure of phoretic assemblages. According to her observations, larger individuals of U. vinicolora attach in lower numbers to smaller individuals of I. typographus, or several individuals of this mite species but smaller in size were found on a larger individual of I. typographus in the company of other mites, most often of deutonymphs of T. polytricha.
The numbers of Uropodina species carried probably depends primarily on their biodiversity within a given habitat. Bark beetles were observed to carry high numbers of Uropodina species, probably because more phoretic mite species are associated with wood habitats. Large sizes and longer duration of different kinds of wood habitats offer mites higher diversity of available niches when compared to other smaller, unstable microhabitats.
The body size of the carriers seems to be of less importance when explaining the number of hosted mite species. It was observed that the number of phoretic species of Uropodina associated with the long-horned beetles is similar to that hosted by the bark beetles although differences in body size between cerambycids and scolytines are significant (Moser & Roton Citation1971; Kinn & Linit Citation1989; Knee et al. Citation2012c; Konwerski et al. Citation2017, Citation2020). Finally, the number of mite species carried by a given carrier will depend on its abundance and habitat preferences. Phoretic mites will be more frequently observed on the high-abundant carrier species as the probability of encountering them increases (Bajerlein & Błoszyk Citation2004; Bajerlein Citation2007). Similarly, carrier species with broad environmental preferences can be used for dispersal by more phoretic mite species (Bajerlein Citation2007).
9. Carrier sex specificity
Most studies of whether phoretic deutonymph infestation is sex-biased have observed their equal occurrence on female and male carriers (Moser Citation1976a; Pugh et al. Citation1997; Atakan et al. Citation2009; Mazza et al. Citation2011; Knee et al. Citation2012c; Błoszyk et al. Citation2016; Paraschiv et al. Citation2018; Konwerski et al. Citation2019). Willingness of a mite to accept a carrier of either sex might be expected to increase the range of potential carriers and thus increase the probability of successful colonisation of a new habitat. Sex-biased phoresy was less frequently found and males were usually favoured as carriers (Gwiazdowicz et al. Citation2013; Konwerski et al. Citation2017; Gómez-Marco et al. Citation2021). Nevertheless, a higher mite load of deutonymphs on female carriers was observed by Monzenga Lokela et al. (Citation2021) for Centrouropoda almerodai and Uroobovella phoenicicola Kontschán et al., Citation2012 phoretic on Rhynchophorus phoenicis. Atakan et al. (Citation2009) have found females of Rhynchophorus palmarum to be more heavily infested with deutonymphs than males, but these inter-sexual differences were low and the values of prevalence were similar. Interestingly, it has been shown that Trichouropoda shcherbakae disperse more frequently on males of Tetropium castaneum (Linnaeus, 1758) and on females of Tetropium fuscum (Fabricius, 1787) (Cerambycidae) (Konwerski et al. Citation2017).
Previous studies suggest that differences in infestations with Uropodina between female and male carriers need not be interpreted as mite preferences towards either carrier sex, but may simply result from differences in biology between both sexes of the carrier. For instance, Monzenga Lokela et al. (Citation2021) have suggested that higher deutonymph infestation in females of R. phoenicis could reflect the fact that females remain longer on palm trees and are therefore easier to find than males. In turn, field experiments carried out by Gwiazdowicz et al. (Citation2013) and Konwerski et al. (Citation2019) during May-July in Białowieża Primeval forest (Poland) have revealed that the prevalence of Trichouropoda sociata (Vitzthum, Citation1923) on both sexes of Plagionotus detritus (Linnaeus, 1758) (Cerambycidae) varied across time. Gwiazdowicz et al. (Citation2013) who conducted their studies in 2011 have found more males carrying deutonymphs of this Uropodina species. In turn, Konwerski et al. (Citation2019) did not find significant differences in prevalence between females and males when analysing combined data, but the numbers of carriers within both sexes differed across the year of the study with more infested males in 2014 and 2015, and more infested females in 2016.
The presence or absence of dimorphism in body size is one of the main features that characterise females and males in insects. The relation between the body size of male and female carriers and the degree of infestation with phoretic Uropodina is not obvious. For instance, Gómez-Marco et al. (Citation2021) observed that the presence of Uropodina on R. palmarum was positively correlated with reduced pronotum width in males but not in females. In turn, Konwerski et al. (Citation2019) did not find differences in the proportion of males and females of P. detritus carrying deutonymphs of T. sociata, although females were significantly larger than males. As explained, the numbers of females and males carrying deutonymphs are similar because new trees are colonised by representatives of both sexes. Presumably, differences in body size between sexes are of less importance for deutonymphs.
Many authors recognised this problem and examined rates of deutonymph infestation in female and male carriers, taking into account mites from various taxonomic groups. It now seems clear that carrier sex specificity in Uropodina is a complex issue that depends on details of the biology of both the mites and the beetles, and will need careful study in separate mite/beetle combinations.
10. Deutonymph localisation on carriers
The localisation of Uropodina on the body of their carrier is one of the most conspicuous and frequently studied aspects of phoresy in this group, but the attachment patterns of deutonymphs have been analysed in detail only for a limited number of species (e.g., Schwarz et al. Citation1998; Bajerlein & Błoszyk Citation2004; Błoszyk et al. Citation2006, Citation2013; Feketeová Citation2011b; Mazza et al. Citation2011; Vrabec et al. Citation2012; Gwiazdowicz et al. Citation2013; Čejka & Holuša Citation2014b; Bajerlein et al. Citation2016; Pfammatter et al. Citation2016b; Konwerski et al. Citation2017, Citation2019, Citation2020; Paraschiv et al. Citation2018; Gómez-Marco et al. Citation2021).
10.1. Attachment sites
Different species of Uropodina have been shown to differ in their location on the body of their carrier (e.g., Schwarz et al. Citation1998; Bajerlein & Błoszyk Citation2004; Mazza et al. Citation2011; Knee et al. Citation2012c). Even deutonymphs of the same species may have different attachment patterns on different species of carrier (e.g., Bajerlein et al. Citation2016; Konwerski et al. Citation2017). The attachment sites of Uropodina have been best studied in beetles. Deutonymphs are often observed attached to the surface of the elytra, legs, and abdominal sternites () (e.g., Moser Citation1976b; Bajerlein & Błoszyk Citation2004; Feketeová Citation2011b; Vrabec et al. Citation2012; Bajerlein et al. Citation2016; Konwerski et al. Citation2017). In contrast, deutonymph attachment to the carrier’s head is much less common. In some species, deutonymphs avoid attaching to the pronotum, whereas in others they distinctly prefer this part of the beetle’s body (Knee et al. Citation2012c; Konwerski et al. Citation2020).
Deutonymphs of Uropodina usually occupy the external surfaces of their carrier’s body. Their occurrence under beetle elytra is not common, but exceptions occur. The underside of the elytra seems to be a primary location of deutonymphs of Centrouropoda almerodai (Porcelli et al. Citation2009; Mazza et al. Citation2011; Dilipkumar et al. Citation2015; Farmahiny Farahani et al. Citation2016; Slimane-Kharrat & Ouali Citation2019) on the Red Palm Weevil. The deutonymphs of Centrouropoda sp. are the biggest and most abundant mites in this niche. Other small species of mites can co-exist with Centrouropoda sp. under the elytra of a beetle, but moderate size deutonymphs such as those of Uroobovella sp. and Nenteria extremica Kontschán et al., Citation2014a cannot, and are displaced to less desirable attachment sites (Matos et al. Citation2023).
The use of the same location site was also observed in Trichouropoda lamellosa phoretic on Monochamus scutellatus by Knee et al. (Citation2012c) and in Trichouropoda sociata on Plagionotus detritus (Konwerski et al. Citation2019). Some authors have observed unattached deutonymphs under the elytra on the membranous wings which were explained by the “scooping” mechanism (Konwerski et al. Citation2019). As a result of the folding and unfolding of the membranous wings, some deutonymphs that were previously attached to the underside of elytra and abdominal tergites might be detached (Konwerski et al. Citation2019, Citation2020). Therefore, the presence of Uropodina within this area is rather accidental.
10.2. Attachment patterns
The attachment sites of Uropodine mites on their host may be considered as dorsal vs ventral and anterior vs posterior. Deutonymphs are often found attached to the dorsal/posterior (e.g., Moser et al. Citation1989), ventral/anterior (e.g., Schwarz et al. Citation1998), and ventral/posterior (e.g., Bajerlein et al. Citation2016) areas of their carrier (). They are found less often in the dorsal/anterior region (Konwerski et al. Citation2020), or evenly distributed (Konwerski et al. Citation2020). Phoretic deutonymphs are occasionally found under the elytra of a beetle but this is the case for only a small number of species (e.g., Porcelli et al. Citation2009; Feketeová Citation2011b; Vrabec et al. Citation2012; Konwerski et al. Citation2020).
Table II. Examples of patterns of attachments in selected species of phoretic Uropodina.*
Many authors have reported site-specificity in deutonymphs of Uropodina (e.g., Schwarz et al. Citation1998; Knee et al. Citation2012c; Konwerski et al. Citation2020). The attachment space on the carrier can be a limiting resource for mites and therefore different mite species may show specialisation in attachment site to reduce interspecific competition (Hofstetter et al. Citation2013; Pfammatter et al. Citation2016b; Paraschiv et al. Citation2018). Many species of Uropodina have been shown to be specific in their selection of attachment locations because deutonymphs occupy the same body parts in different individuals of the same carrier species. Authors who have studied two - or three-species assemblages of phoretic Uropodina have revealed that these co-occurring mites were highly site-specific. For instance, Knee et al. (Citation2012c) observed that most deutonymphs of T. lamellosa occupied the underside of the elytra of the longhorn beetle M. scutellatus, while most deutonymphs of Trichouropoda hirsuta were present on the prothorax of the same carrier. Many studies revealed a constant pattern of attachment sites in Trichouropoda polytricha and Uroobovella ipidis phoretic on Ips typographus. Although both species infested the elytral declivity and ventral thorax, T. polytricha was most abundant on the elytral declivity whereas U. ipidis on the ventral thorax (Moser et al. Citation1989; Feketeová Citation2011b; Vrabec et al. Citation2012; Čejka & Holuša Citation2014a). However, even in cases of a distinct attachment pattern some exceptions can be observed. Paraschiv and Isaia (Citation2020) found no significant differences between the numbers of deutonymphs of T. polytricha located on the thorax and the elytral declivity of I. typographus, whereas U. ipidis was more abundant on the elytral declivity than on the thorax. Paraschiv et al. (Citation2018) found that T. polytricha showed the same site preferences both on I. typographus and Ips sexdentatus (Börner, 1766). In contrast, Pityokteines chalcographus carried more deutonymphs of T. polytricha on its posterior abdomen and the area between its legs than on its elytra and thorax (Khaustov et al. Citation2016).
The specificity of Uropodina for attachment sites may also be reflected in the number of body parts infested. In some species, deutonymphs were found attached to many different body parts on the same carrier individual, while in other species, deutonymphs were restricted only to one or two sites. For instance, deutonymphs of Uroobovella nova sensu (Oudemans, Citation1902) were attached only to the ventral surfaces of the head and thorax, and on the forelegs of their Nicrophorus beetle carriers (Schwarz et al. Citation1998). Deutonymphs of C. almerodai usually attach themselves to the underside of the elytra of the red palm weevil Rhynchophorus ferrugineus and less frequently to other parts of its body (Mazza et al. Citation2011; Farmahiny Farahani et al. Citation2016). A similar localisation of phoretic deutonymphs has been shown for other species of the genus Centrouropoda collected from Rhynchophorus palmarum (Gómez-Marco et al. Citation2021) and Rhynchophorus phoenicis (Kontschán et al. Citation2012). In these species, high site-specificity reflects high carrier specificity. In contrast, deutonymphs of Uropoda orbicularis, a species with a broad carrier range, can attach to almost all body parts of their Aphodius beetle carriers, which can be interpreted as a low site-specificity (Bajerlein & Błoszyk Citation2004; Bajerlein et al. Citation2016). The hindlegs and posterior elytra of Aphodius species are more frequently and abundantly infested by U. orbicularis, which may indicate that these body parts are preferred. On the other hand, U. orbicularis shows different attachment patterns on different carrier species, showing a rather low attachment-site specificity. It seems that at least some species of Uropodina show some kind of flexibility in the selection of attachment sites in relation to the carrier species, which can minimise the risk of dislodgement, hence increasing the probability of successful dispersal (Gómez-Marco et al. Citation2021). Therefore, the attachment patterns seem to be carrier-specific at least in some species, since deutonymphs of the same species can take different positions on different carrier species ().
10.3. Attachment site selection
The mechanisms of selection of attachment sites in phoretic deutonymphs of Uropodina are not well known. Nevertheless, the fact that the same Uropodina species show different locations on different carrier species may suggest that their attachment patterns are strongly carrier dependent. According to Faasch (Citation1967), the choice of the attachment location can be affected by the presence of previously attached deutonymphs or even pedicels without deutonymphs. Mites preferred to produce their stalks when they came across already attached deutonymphs. If they were previously “excited” running around on the beetle, they immediately calmed down when they found attached deutonymphs, and often attached themselves close to them (Faasch Citation1967). Deutonymphs of many Uropodina species were observed to travel in clumps, such that a group of individuals were attached one close to the other even if the remaining area was not occupied with deutonymphs () (Faasch Citation1967; Bajerlein et al. Citation2013; Slimane-Kharrat & Ouali Citation2019). This arrangement suggests probable chemical communication between deutonymphs. Faasch (Citation1967) also showed that deutonymphs perceive their carriers using olfactory cues and that their search for phoretic carriers is stopped by stimuli emanating from fresh manure (main components are odour and moisture). Faasch (Citation1967) has also reported that deutonymphs usually attach themselves to smooth and hydrophobic surfaces. Bajerlein et al. (Citation2016) found that deutonymphs of U. orbicularis attach to smooth, hairy sites as well as flat and sculptured surfaces. Smooth body parts and body parts covered with sparse setae were most frequently and intensively occupied with deutonymphs, whereas surfaces with a dense cover of setae were avoided. Phoretic deutonymphs of U. orbicularis preferred flat surfaces of elytral intervals on Aphodius beetle carriers, but frequently occupied the densely punctate propygidium and pygidium of Margarinotus carbonarius (Hoffmann, 1803) (Histeridae).
Carrier surface morphology is important for the attachment of deutonymphs, but it cannot fully explain the observed location of Uropodina. The selection of attachment sites that will reduce the risk of being incidentally removed or damaged while the carrier is moving is one of the most frequently discussed factors affecting attachment patterns in Uropodina (e.g., Pugh et al. Citation1997; Błoszyk et al. Citation2006; Pfammatter et al. Citation2016b; Paraschiv et al. Citation2018). This may explain why deutonymphs phoretic on tunneling carriers often occupy the ventral surface and the disturbance-free elytral declivity or the apex of the elytra. More exposed surfaces such as the pronotum or lateral sides of the elytra where the removal is likely are avoided (Bajerlein et al. Citation2016; Paraschiv & Isaia Citation2020). Dilipkumar et al. (Citation2015) suggest that deutonymphs present under beetle elytra can be protected from hot environments and noticed that mites that were collected from this area were less desiccated. Interestingly, it was noticed that the occurrence of mites within the underside of elytra may be affected by the presence of nematodes on which they can feed (Pfammatter et al. Citation2013; Paraschiv et al. Citation2018). Konwerski et al. (Citation2017) have indicated that the selection of attachment sites by mites may result from differences in their climbing behaviour and that particular carrier body sites may differ in their accessibility to phoretic mites in different habitats. Kršiak et al. (Citation2010) concluded that some body parts of the carrier can be more available to mites due to their exposure and shape. Deutonymphs of Trichouropoda pecinai were found on tibiae of Hylastes cunicularius but were absent on tarsi and femora, which, according to Kršiak et al. (Citation2010) seemed to be too exposed to present suitable attachment sites. On the contrary, Konwerski et al. (Citation2017) found a high infestation of T. sociata on the femora of P. detritus.
The successful dispersal of mites can only be achieved when the carrier reaches a new habitat. Therefore many authors have pointed out that the localisation of phoretic deutonymphs on the carrier body cannot negatively affect its sensory, feeding, and movement (Athias-Binche Citation1994; Bajerlein & Błoszyk Citation2004). This would explain why anterior body parts of carriers, particularly the head, are rarely occupied by Uropodina. Another factor that is considered to affect the attachment sites in Uropodina is the grooming behaviour of the carrier, i.e., its ability to clean particular parts of its body (Rigby Citation1996; Błoszyk et al. Citation2013; Chaires-Grijalva et al. Citation2013; Konwerski et al. Citation2019). However, Rigby (Citation1996) pointed out that due to the firm mode of attachment, the removal of deutonymphs of Uropodina by the carrier cannot be possible.
For unidentified Uropodina phoretic on amphipods, chemotaxis was identified as a mechanism of attachment site selection, where different amounts of ammonia excreted by different gills affected its selection (Rigby Citation1996). Feketeová (Citation2011b), studied phoretic associations between Uropodina and I. typographus and found a relationship between mite body size and the site of attachment. Smaller deutonymphs were most frequently attached to the ventral part of the carrier’s body and strongly preferred the area between the first and the second pair of legs, whereas larger deutonymphs preferentially occupied the elytral declivity. Some authors have found temporal changes in the preferred attachment site for Uropodina. For instance, Konwerski et al. (Citation2017) recorded higher numbers of T. sociata on abdominal ventrites of P. detritus in 2014 and 2016, but in 2015 beetle abdominal tergites were more heavily infested with deutonymphs. Gómez-Marco et al. (Citation2021) found that Centrouropoda sp. preferentially occurred under the elytra of R. palmarum in 2017, but in 2018 it was more abundant on the external surfaces of the beetle. The change was associated with an increased abundance of Centrouropoda per beetle (Gómez-Marco et al. Citation2021), suggesting that the mites only spread to the external surfaces of the beetles when space under the elytra was overcrowded.
In summary, the observed attachment patterns in Uropodina are often interpreted as preferences for specific locations, but they can actually be the outcome of several interacting forces. The true ecological meaning of variation in attachment sites is not yet understood.
11. Phoretic deutonymph infestation
Uropodina infestation varies among carrier species. In most studies, the minimal number of deutonymphs carried by a single host individual was one, but the maximum mite load was very high, reaching over 100 deutonymphs (e.g., Schwarz et al. Citation1998; Philips Citation2009; Błoszyk et al. Citation2016). A case of extremely high infestation of 780 mite individuals on Plagionotus detritus was recorded by Gwiazdowicz et al. (Citation2013). Nevertheless, these observations have also shown that an extremely high mite load is rather uncommon. For instance, Konwerski et al. (Citation2020) observed that 81.5% of individuals of Monochamus sartor urussovii (Waldheim, 1806) (Cerambycidae) carried 1–20 deutonymphs of Oodinychus ovalis and only 7.4% of individuals carried over 100 deutonymphs of this mite species. Similarly, most individuals of P. detritus (76.1%) carried 1–50 deutonymphs of Trichouropoda sociata, whereas a high deutonymph load of over 200 deutonymphs was observed only for 1.4% of the beetles examined (Konwerski et al. Citation2020). The prevalence of phoretic deutonymphs, i.e., the proportion of infested carriers, may also vary widely from less than 1% to more than 90% (e.g., Mazza et al. Citation2011; Knee et al. Citation2012c; Gómez-Marco et al. Citation2021). During regular sampling of carriers the prevalence of mites was rather low and usually did not exceed 30% (e.g., Moser Citation1976b; Knee et al. Citation2012c; Błoszyk et al. Citation2013, Citation2016; Gwiazdowicz et al. Citation2013; Pfammatter et al. Citation2013; Konwerski et al. Citation2017, Citation2019). High values of Uropodina prevalence were usually observed seasonally, i.e., in a specific period or in case of highly-specific interactions in which the number of carriers was restricted to one or a few closely related species (Schwarz et al. Citation1998; Al-Deeb et al. Citation2011; Mazza et al. Citation2011; Gómez-Marco et al. Citation2021).
11.1. Factors affecting deutonymph infestation in Uropodina
We concluded that the factors that influence levels of Uropodina infestation can be identified as (1) carrier-dependent factors; (2) mite–dependent factors; and (3) other factors (). Carrier-dependent factors include physical properties of the carrier such as the carrier’s body size. During phoretic dispersal the carrier becomes a habitat for the mite, so the number of deutonymphs carried will be limited by the body size of the carrier. Bajerlein and Przewoźny (Citation2012) found that the body size of coprophilous hydrophilid beetles was critical for infestation with phoretic deutonymphs of Uropoda orbicularis, and species with body length below 2.3 mm were not infested with deutonymphs. However, Jałoszyński (Citation2015) observed deutonymphs of U. orbicularis attached to adults of Ptenidium pusillum (Gyllenhal, 1808) (Coleoptera: Ptiliidae), which is characterised by an average body length of 1 mm.
The effect of carrier body size on deutonymph infestation seems to depend on more than simply a crude measurement of the carrier’s size. Some authors found that carrier body size was not related to the Uropodina infestation (Pugh et al. Citation1997; Błoszyk et al. Citation2016), or although it was important, it was just one factor among others (Rigby Citation1996). Interestingly, Gómez-Marco et al. (Citation2021) observed that the presence of Uropodina mites on Rhynchophorus palmarum was positively correlated with the reduced pronotum width, but only in males. The authors suggested that phoretic mites associated with pre-pupal and pupal weevils before the infestation of adult carriers, could impose a fitness cost in terms of resulting male size (Gómez-Marco et al. Citation2021).
The effect of carrier body size on Uropodina infestation is usually involved in studies that intend to analyse the deutonymph infestation according to carrier sex. Many authors did not observe distinct differences in deutonymph infestation between female and male carriers, even if they differed in body size (e.g., Konwerski et al. Citation2019). Nevertheless, in some studies, representatives of a given sex were found to be more heavily infested with phoretic deutonymphs, but these between-sex differences were difficult to explain (Bajerlein et al. Citation2016; Konwerski et al. Citation2017; Gómez-Marco et al. Citation2021).
Carrier-dependent factors that influence deutonymph infestation also include the morphology of the carrier’s body surface. Previous studies have provided evidence that deutonymph infestation varied according to carrier body parts. Bajerlein et al. (Citation2016) observed that smooth body surfaces and surfaces with a low density of setae were most intensively occupied by deutonymphs of U. orbicularis. Similar relationships between the morphology of the carrier body surface and deutonymph infestation were observed by Konwerski et al. (Citation2020) for Uropodina phoretic on longhorn beetles and by Rubink et al. (Citation1991) for unidentified phoretic deutonymphs and Apis mellifera Linnaeus, 1758.
Another factor that was considered to potentially affect Uropodina infestation is the timing of exposure of the carrier to mites. Rigby (Citation1996) observed that large beach hoppers emerge to forage later and, therefore appeared to be less active, probably increasing their level of exposure to the slow-moving mites. The infestation level of phoretic deutonymphs may also be influenced by the self-grooming used by insects to reduce the mite or parasite loads (Delfinado-Baker et al. Citation1992; Konwerski et al. Citation2019). Delfinado-Baker et al. (Citation1992) suggested that grooming limits the number of phoretic mites and reduces their sites of attachment. On the contrary, Rigby (Citation1996) reported that firmly attached deutonymphs of Uropodina were not removed by the grooming behaviour of the beach hopper. Grooming appears to limit the attachment of deutonymphs and contribute to removal of those that are already attached. It will probably depend mainly on the biology and morphology of the carrier species and on which part of the body the deutonymph prefers to attach.
The level of phoretic deutonymph infestation on a host undergoes temporal variations. Differences in deutonymph prevalence and the mean number of carried individuals were observed both between study seasons and years (Rodrigueiro & Do Prado Citation2004; Bajerlein Citation2011; Błoszyk et al. Citation2013; Pfammatter et al. Citation2013; Konwerski et al. Citation2017, Citation2019; Paraschiv et al. Citation2018). These temporal changes in deutonymph infestation result from both carrier and mite phenologies, and their variations in abundance. This means that different sampling dates can provide completely different information about deutonymph infestation. This fact should be taken into account when conclusions about the carrier range and mite infestation are not drawn based on data obtained from long-term studies of a local community but based on several samplings made over a relatively short period.
An example of a mite-dependent factor affecting the infestation of Uropodina can also be the mode of attachment to the carrier, i.e., the ability to secrete an anal pedicel. Phoretic deutonymphs may modify the length of their pedicels, and as reported by Bajerlein and Witaliński (Citation2014), the variable length of pedicels allows many deutonymphs to be distributed in three-dimensional space instead of in one plane on the carrier’s surface. Mite load can also be affected by the body size of phoretic individuals. Feketeová (Citation2011b) has observed that larger individuals of Uroobovella vinicolora attach in lower numbers to smaller individuals of Ips typographus, or several individuals of this species, but smaller in size, occur on I. typographus in the company of deutonymphs of other species.
Uropodina infestation is also affected by factors that are not carrier or mite dependent. Holuša and Čejka (Citation2020) have examined the effect of the sampling method on the mite load and revealed that mean numbers of Trichouropoda polytricha and Uroobovella ipidis per beetle were higher on beetles captured in emergence traps than those collected from pheromone traps. Uroobovella vinicolora and O. ovalis were not found on I. typographus captured in emergence traps. Mazza et al. (Citation2011) found that the method of sampling adults of Rhynchophorus ferrugineus significantly affected the infestation of Centrouropoda almerodai. A higher proportion of infested beetles was captured with aggregation pheromone traps compared with those collected by hand from infested plants.
Different infestations of Uropodina on the same carrier species have been observed under various climatic conditions, localities, and habitats (Kršiak et al. Citation2010; Knee et al. Citation2012c; Pfammatter et al. Citation2013, Citation2016a; Vissa et al. Citation2019, Citation2021). Deutonymph infestations may also be affected by factors whose effects are difficult to predict, such as changes in habitat humidity, deutonymph overcrowding, or a sudden decrease in available carrier numbers (Athias-Binche Citation1994). These factors are likely to have the greatest impact on cases of an extremely high deutonymph load.
In summary, many factors have been identified that may shape the relationship between Uropodina and their carriers. Some of the available data are inconclusive or contradictory. It is clear that the level of infestation of mites on a carrier can be simultaneously affected by various factors, but there is very little scientific understanding of their interactions.
12. Temporal dynamics of phoresy
Temporal variation in phoresy by Uropodina has been very poorly studied. Studies in this area have been conducted by collecting carriers and determining the numbers of phoretic deutonymphs found on them, or by studying the population structure of a given species of Uropodina inhabiting a given habitat. In the latter case, conclusions on the temporal dynamics of phoresy were made based on changes in the numbers of non-phoretic and phoretic deutonymphs but without collecting their carriers (Athias-Binche Citation1993). Very rarely a combined approach has been used, including the observations of both the abundances of deutonymphs collected from carriers and those collected from the habitat (Lieutier Citation1978).
Most of the information on the temporal dynamics of phoresy in Uropodina comes from the study of mites associated with wood-inhabiting beetles, particularly scolytines and cerambycids (e.g., Knee et al. Citation2012c; Konwerski et al. Citation2017, Citation2019; Paraschiv & Isaia Citation2020). Less frequently, such observations included species colonising short-term temporary habitats such as dung of herbivorous mammals (Bajerlein Citation2011) or carrion (Barton et al. Citation2014).
Some basic questions on the temporal dynamics of phoresy are (1) when does phoretic dispersal start seasonally, and when does it end; (2) is the phoretic activity initiated by the carrier activity or by unfavorable environmental factors; (3) to what degree is phoresy affected by the carrier’s life cycle, particularly its phenology and abundance; (4) is the proportion of phoretic deutonymphs constant or does it change across time?
Previous studies have provided answers to at least some of these questions. Many authors have observed phoretic deutonymphs attached to overwintered beetles during spring swarming or to the newly emerged adults during their dispersal flight (Kinn & Linit Citation1989; Mašán & Halliday Citation2009; Błoszyk et al. Citation2013; Konwerski et al. Citation2017, Citation2019; Paraschiv & Isaia Citation2020; Holuša & Čejka Citation2020). In some species of Uropodina, phoretic deutonymphs remained in close associations with immature stages of their carriers, waiting until they became adults (Porcelli et al. Citation2009). The phoretic activity may decline at different periods. For instance, in Uropoda orbicularis, phoresy was observed until the beginning of November, but infestation of phoretic deutonymphs during autumn was extremely low (Bajerlein Citation2011). Gwiazdowicz et al. (Citation2013) found a distinct decrease in the dispersal activity of Trichouropoda sociata phoretic on Plagionotus detritus during July.
Temporal changes in the abundance of phoretic deutonymphs have been observed for species of Uropodina that occur in temperate regions, with the highest activity usually found during spring or early summer (Lieutier Citation1978; Athias-Binche Citation1993; Bajerlein Citation2011; Błoszyk et al. Citation2013; Konwerski et al. Citation2017, Citation2019; Paraschiv & Isaia Citation2020). These studies have also revealed that the temporal dynamics of phoretic deutonymphs are most of all affected by the biology of the carrier, especially its life cycle, phenology, and abundance. Changes in the number of deutonymphs corresponded to changes in the number of their carriers (Bajerlein Citation2011; Knee et al. Citation2012c; Konwerski et al. Citation2017, Citation2019). Nevertheless, some studies have found a decline in the phoretic activity despite the high numbers of available carriers (Bajerlein Citation2011; Gwiazdowicz et al. Citation2013; Konwerski et al. Citation2019), or phoretic deutonymph load in spite of a significant decline in the number of their carriers (Knee et al. Citation2012c). This may suggest that the phoretic activity of Uropodina depends not only on the biology of the carriers but on the mite’s own phenology as well as unpredictable environmental factors. Nevertheless, in some studies, a lack of phoretic activity was observed year after year despite the availability of carriers, which suggests that the decline in phoretic dispersal at a given period is constant in the mite’s life cycle (Bajerlein Citation2011).
Previous studies have also revealed that for some species of Uropodina, the temporal variations in their phoretic activity may differ according to the carrier species. This has been well illustrated by the example of Trichouropoda polytricha phoretic on Ips typographus and Ips sexdentatus. The study conducted in Romania revealed the maximum load of T. polytricha on I. typographus in May (Paraschiv & Isaia Citation2020). On the contrary, the highest load of T. polytricha on I. sexdentatus collected in Spain was found in early October and then in June and April, which suggested that the dispersal of T. polytricha on I. sexdentatus is related to the emergence of a new generation (Paraschiv et al. Citation2018). For T. polytricha phoretic on I. typographus, the highest dispersal activity was more related to the swarming of the overwintered adults, and phoretic deutonymphs were only observed by the end of the first generation of this bark beetle (Paraschiv & Isaia Citation2020).
In some cases the seasonal dynamics of phoresy have been observed where several species of mites are phoretic on the same carrier species. For instance, Paraschiv and Isaia (Citation2020) noticed a distinct shift in abundance peaks in T. polytricha and Uroobovella ipidis phoretic on I. typographus, with the first species reaching its highest numbers in the middle of May and the latter in early June. On the contrary, Holuša and Čejka (Citation2020) have observed peaks in abundance of T. polytricha and U. ipidis phoretic on I. typographus at the same time, at the beginning of May.
Athias-Binche (Citation1993) examined the behaviour of Allodinychus flagelliger (Berlese, Citation1910), which inhabits dead wood and is phoretic on xylophagous beetles. Immigration of phoretic deutonymphs occurs in autumn, when beetles carrying mites arrive at a dead log to build their galleries. The rate of immigration declines during the later stages of aging of the wood. Subsequent emigration of phoretic deutonymphs occurs in spring when the beetles disperse away from their place of origin. The rate of emigration of A. flagelliger increases with the progressive decomposition of the dead wood. As the wood decomposes it becomes more spongy and retains more water. The rate of emigration of mites was highly correlated with the increase in water content of the wood. Environmental factors did not affect the frequency of sedentary deutonymphs, and the number of the latter was genetically fixed. On the contrary, phoresy in Uroseius sp. on Musca domestica Linnaeus, 1758 turned out to be correlated with temperature but not with rainfall and relative humidity (Rodrigueiro & Do Prado Citation2004). Interestingly, Rodrigueiro and Do Prado (Citation2004) found that the phoresy of Uroseius sp. on M. domestica observed in poultry houses was seasonal because the numbers of flies were affected by low temperatures and the use of insecticides.
The observed dynamics of phoresy seem to be a result of the interaction of various factors, among which the carrier-dependent factors such as phenology, abundance, and life cycle, seem to play a crucial role. Multiannual observations of phoresy in some species of Uropodina have shown that the dynamics of this animal-mediated dispersal are relatively constant (e.g., Athias-Binche Citation1993; Bajerlein Citation2011; Konwerski et al. Citation2019).
Camerik (Citation2010) suggested a revision of the categories of phoresy described by Athias-Binche (Citation1991, Citation1994) and classified them in terms related to fluctuations or changes in the environment rather than the frequency of migration. As a result, Camerik (Citation2010) proposed to term the accidental phoresy as facultative and the facultative as obligatory. Both in long-term (e.g., decaying logs) and short-term temporary (e.g., dung, carrion) habitats, the phoresy is induced by fluctuations in the environment, so it is obligatory, and the frequency of mite migration will be a function of the environmental changes. According to Camerik (Citation2010) obligatory phoresy, which Athias-Binche (Citation1994) divided into seasonal and cyclical, should rather be divided into frequent and infrequent. Obligate frequent phoresy refers to species inhabiting short-term temporary microhabitats, whereas obligate infrequent phoresy will be observed in species colonising long-term temporary microhabitats.
Our observations of phoresy in Uropodina suggest that the categories proposed by Camerik (Citation2010) describe the frequency of phoretic dispersal better than those of Athias-Binche (Citation1994). Phoresy in most species of Uropodina is basically obligatory, and the differences concern only the frequency of its observations. In species inhabiting short-term temporary habitats, the dispersal occurs frequently because it is induced by changes in the environment, such as population overcrowding or a decrease in humidity. In the case of long-term temporary habitats such as dead wood, deterioration of habitat quality occurs more slowly, so phoresy is observed less often. Phoresy in Uropodina has often been reported as dispersal on beetles attacking living, weakened, or diseased trees. Phoresy of Uropodina on xylophagous beetles seems to be more affected by the life cycle of the carrier than by changes in the environment itself. It is a constant life strategy but occurs seasonally depending on the life cycle of the carrier. Therefore, it is usually observed during such events as the beetle spring swarm or the new generation flights.
13. On arrival
Three groups of mites have convergently evolved phoretic dispersal at the deutonymph stage – Uropodina, Astigmata, and Parasitidae. Binns (Citation1982) reviewed the available information about the transformation of Astigmatid hypopodes to adults, and briefly mentioned the same phenomenon in Parasitus coleoptratorum (Linnaeus, 1758). The issues faced by a parasitid deutonymph when it arrives at a new habitat patch were recently discussed by Yasui (Citation2023). In brief, a deutonymph must decide whether or not to moult to the adult stage and search for a mate. If it does so, it sacrifices any further opportunity for phoretic dispersal. Alternatively it may remain as a deutonymph and seek a carrier for another dispersal event. It would appear that a Uropodine deutonymph faces the same decision – proceed to adulthood or remain as a deutonymph and attach to another carrier. The sensory and behavioural processes that lie behind that decision remain completely unexplored.
14. Consequences of phoresy for Uropodina and their carriers
Phoresy enables the colonisation of new areas, islands, and habitats, particularly those ephemeral and patchily distributed (Athias-Binche Citation1991). The populations of phoretic mites are usually structured in scattered demes, and the phoretic behaviour enables not only the survival of the population, avoidance of inbreeding, or competition, but also gene flow (Athias-Binche Citation1993).
Phoresy is considered a starting point in the evolution of parasitism in some mites (Athias-Binche & Morand Citation1993), but parasitism has only rarely developed in the Uropodina. Ragusa et al. (Citation2008) found that deutonymphs of Centrouropoda almerodai were commonly phoretic on the Red Palm Weevil Rhynchophorus ferrugineus, and that deutonymphs could feed on dead weevils to obtain enough nutrition to complete their development to adults. However, deutonymphs do not feed while they are attached to their living carriers. True parasitism in the Uropodina appears to exist only in the genus Macrodinychus Berlese, Citation1917. Lachaud et al. (Citation2016), Brückner et al. (Citation2017), and Pérez-Lachaud et al. (Citation2019) have shown that some species of Macrodinychus act as true parasitoids of ants. They are referred to as parasitoids because the immature mites are parasites of ant pupae and the adult mites are free-living predators. The complexity of this relationship is becoming clear as detailed behavioural studies are carried out (Pérez-Lachaud et al. Citation2019). Macrodinychus does not have a deutonymph adapted for dispersal by phoresy, and the available information does not suggest that a phoretic deutonymph was a precursor to the development of parasitism.
If the main goal of phoresy by mites is the colonisation of a new habitat, it is not in the mites’ interest to reduce their carrier’s mobility. However, the presence of phoretic mites does sometimes appear to impose physical constraints on their carrier (Athias-Binche & Morand Citation1993). The effect of Uropodina on their carriers will depend on the size and number of attached deutonymphs, their localisation, and the body size of the carrier. It might be expected that the same number of phoretic mites may differently affect the mobility of small and large carriers. Some authors have observed extremely heavy deutonymph infestations on their carriers (Schwarz et al. Citation1998; Gwiazdowicz et al. Citation2013; Konwerski et al. Citation2020). In such cases, the presence of phoretic deutonymphs might reduce the mobility of their carrier. Phoretic deutonymphs have rarely been observed on the head of their carrier, probably because even a low number of deutonymphs attached to the carrier’s head would be harmful to its orientation (Athias-Binche & Morand Citation1993).
Only a few authors have studied how phoretic deutonymphs of Uropodina affect their carriers. Kinn and Witcosky (Citation1978) have observed that adults of Dendroctonus frontalis Zimmermann, 1868 trapped at 3 metres altitude have more pedicels of deutonymphs of Trichouropoda australis Hirschmann, Citation1972a than those trapped at 6 or 9 meters, which suggested that the flight of beetles is influenced by the presence of phoretic mites. Błoszyk et al. (Citation2013) used the term “transport parasitism” to describe the situation where a beetle is measurably hampered by a heavy load of mites. On the other hand, Mazza et al. (Citation2011) observed a high rate of C. almerodai infestation in trap-captured adults of R. ferrugineus, which successfully flown to reach the pheromone trap. Pfammatter and Raffa (Citation2015) concluded that deutonymphs of T. australis and other mites phoretic on Ips grandicollis (Eichhoff, 1868) did not have a significant impact on the beetle’s reproductive success, and other factors such as tree quality, beetle predation, and weather appeared to be more important.
In our view, the long-term effect of Uropodina on their carriers will probably differ between cases of non-specific and highly-specific phoretic interactions. In cases of non-specific interactions, a particular beetle may be harmed by an excessive load of mites. However, that harm is likely to be accidental and localised, the result of unpredictable conditions, and the probability of its occurrence will increase with decreasing number of carriers. The consequences of phoresy might be more serious in the case of a highly specific relationship in which a mite species uses only one or a few species of carriers. A permanent and specific relationship involving a high percentage of infested carriers and relatively high mite loads may not only involve dispersal but lead to the synchronisation of the life cycles of the mite and its carrier, and evolve into true parasitism (Athias-Binche & Morand Citation1993).
A highly specific phoretic association exists between C. almerodai and the Red Palm Weevil Rhynchophorus ferrugineus. Centrouropoda almerodai is attracted to beetles at their pupal stage and moves onto the adult beetles as they emerge (Longo et al. Citation2009). Adults of R. ferrugineus with a heavy load of attached mites have their lifespan reduced by one third when compared with non-infested beetles (Mazza et al. Citation2011). Additionally, Ragusa et al. (Citation2008) found that deutonymphs of C. almerodai can feed on dead bodies of Red Palm Weevil and use them as a source of the protein required for completion of their development, which may suggest a consistently negative impact on their weevil carriers. However, under some circumstances C. almerodai may actually be beneficial to R. ferrugineus. The Red Palm Weevil is attacked by several species of parasitic nematodes (Mazza et al. Citation2014; Ibrahim et al. Citation2021; Loulou et al. Citation2023). Morton and García-Del-Pino (Citation2011) showed that C. almerodai can feed on these entomopathogenic nematodes, to the extent that it can reduce the effectiveness of the nematodes as biological control agents directed against the Red Palm Weevil.
15. Practical applications of phoresy in Uropodina mites
Bark beetles are frequent carriers of Uropodina, and are also vectors of different species of fungi that cause serious tree diseases. Phoretic mites have therefore been examined to determine whether they can serve as vectors of fungal pathogens. Moser et al. (Citation1997) recorded spores of the fungus Ophiostoma bicolor Davidson & Wells, 1955 on the bodies of Trichouropoda polytricha, Trichouropoda hirsuta, Uroobovella ipidis, and an unidentified species of Uroobovella phoretic on Ips typographus japonicus in Japan. Moser et al. (Citation2010) studied the mites phoretic on the European elm bark beetles Scolytus spp. carrying Ophiostoma novo-ulmi, which causes Dutch elm disease (DED). Spores of the DED fungus were found on four of the ten species of mites examined, but not on the single species of Uropodina examined, Trichouropoda bipilis (Vitzthum, Citation1921).
Some authors have examined whether phoretic mites can be used as biological control agents for their carrier pests. Pernek et al. (Citation2008) concluded that mites phoretic on bark beetles of the genus Pityokteines Fuchs, 1911 found in Croatia appeared to have no potential to be used for biological control of their carriers. Pfammatter and Raffa (Citation2015) have studied whether Trichouropoda australis and other mites phoretic on Ips grandicollis influence its reproductive system, and found that their effect wasn’t significant when compared to factors such as tree quality, beetle predation, and weather. Rodrigueiro and Do Prado (Citation2004) found phoretic deutonymphs of Uroseius sp. had little effect in controlling house fly (Musca domestica). Similarly, Athias-Binche and Habersaat (Citation1988) have revealed that Janetiella pyriformis (Berlese, Citation1920) (= Uroobovella pyriformis (Berlese, Citation1920)) living in decomposing organic matter has a modest impact on the control of nematodes. Fuscuropoda vegetans (De Geer, Citation1768) was found to be a predator of eggs of the little house fly, Fannia canicularis (Linnaeus, 1761) (O’Donnell & Nelson Citation1967), and eggs and first instar larvae of the house fly (O’Donnell & Axtell Citation1965). On the other hand, Raut and Panigrahi (Citation1991) have suggested that the well-known species Uroobovella marginata might contribute to the control of pest slugs.
Some authors have suggested that Centrouropoda almerodai and other species of Uropodina might contribute to the control of the Red Palm Weevil Rhynchophorus ferrugineus, which is widely considered to be the most damaging insect pest of palms in the world (reviewed by Dilipkumar et al. Citation2015). However, there is no convincing evidence that any species of Uropodina is an effective predator or parasite of R. ferrugineus (Halliday Citation2019).
Similarly, Kontschán et al. (Citation2012) speculated that Uroobovella phoenicicola might have a possible role as a biological control agent for the African Palm Weevil Rhynchophorus phoenicis, but did not present any evidence to support that suggestion. Chaires-Grijalva et al. (Citation2016) reviewed data showing some species of Uropodina feed on nematodes, fungi, and eggs and larvae of bark beetles, but their actual value in biological pest control has not been tested.
Phoretic dispersal is a primary way of colonising unstable and patchily distributed microhabitats such as animal carrion, including human cadavers. Therefore, mites can be potentially useful for forensic purposes as indicators of time elapsed since death (postmortem interval - PMI) and as indicators of their insect carriers. Perotti and Braig (Citation2009) have provided a review of mites, including Uropodina, phoretic on necrophilous insects, with information on their associations with a particular stage of carrion decomposition. Goff (Citation1989, Citation1991) has indicated the potential of soil mites, including Uropodina, as a valuable tool in the estimation of the PMI and emphasised the need for further research in this area.
Studies on phoresy as a dispersal strategy are essential to understanding the real nature of interactions between Uropodina and their carriers. Phoretic species of Uropodina may have practical applications in the biological control of pests, but the evidence to support that hypothesis is not yet available.
16. Conclusions and future perspectives
Phoresy is a vital life strategy in Uropodina. Although interactions between many mites and their carriers have been labelled phoretic, their true nature seems more complex than previously thought (Halliday Citation2019; Noriega et al. Citation2022). As recently discussed by Noriega et al. (Citation2022) in relation to mites and dung beetles, the true interactions between mites and their carriers will require experimental and field studies, which allow us to understand the mechanisms that shape these interactions. Such an approach to the studies of phoresy in Uropodina will certainly be a challenge because of the hidden lifestyle of many species and their carriers.
It will certainly not be possible to recognise the true nature of phoresy between mites and insects without collaboration between acarologists and entomologists. Noriega et al. (Citation2022) indicated that such collaboration is important for the correct species identification, although its absence can be partially compensated for by the use of molecular systematics techniques. However, understanding the mechanisms of phoresy requires not only taxonomic knowledge but also knowledge of the biology and ecology of the interacting species. This can only be ensured through scientific collaboration between different specialists.
Our knowledge of phoresy in Uropodina has increased significantly in recent years, but it is often difficult to interpret due to taxonomic ambiguity of both the mite and its carrier. The development of a stable classification of Uropodina is essential for any studies on this group of mites. Despite these difficulties, we believe we have drawn attention to the major aspects of phoresy in Uropodina, such as the carrier range, attachment patterns, infestation, and seasonal dynamics. Most of the conclusions we reached are vague and contradictory. The difficulties we pointed out in the studies of phoresy, frequent problems in the interpretation of the analysed findings, and doubts about the conclusions, are a permanent feature of this work and often end individual chapters leaving many questions open. The high level of uncertainty in our analysis may be an accurate reflection of the complexity of the subject – it is difficult to generalise about what is intrinsically heterogeneous.
Noriega et al. (Citation2022) formulated a list of questions to be resolved relating to the nature of mite-dung beetle interactions. Below we have added some further questions that are essential or fundamental to understand the ecology and evolution of phoresy in Uropodina.
What is the evolutionary pathway that led to the development of an anal pedicel instead of other methods of phoretic attachment; for example, the use of the chelicerae to grip a bristle on the carrier?
When did this dispersal strategy appear in Uropodina?
Why are beetles the primary carrier group of Uropodina? Is it simply because they are the largest group of terrestrial arthropods? If so, does this mean that phoresy via coleopterans is even more common than we now realise?
How does the presence of the deutonymphs of particular species affect the individual carrier specimen? Does it always cause a decrease in carrier fitness? Can the presence of phoretic deutonymphs be neutral or somehow beneficial to the carrier?
What consequences will the decline in insect biodiversity have on the phoretic dispersal in Uropodina?
What consequences will climate change have on phoretic Uropodina and their carrier insects? If climate change affects the geographical distribution of an insect, its symbiotic and parasitic mites may not be affected in the same way. Any such differential responses could lead to a breakdown in the phoretic relationship, with unpredictable effects on the functions of the surrounding ecological community (Vissa et al. Citation2021).
The search for answers to these questions will provide rich opportunities for research in evolutionary biology. Those future research projects must avoid a naive interpretation of the meaning of “phoresy”. The attachment of a mite to a carrier specimen probably never indicates a simple process of dispersal.
Supplemental Material
Download MS Word (455.5 KB)Acknowledgments
We would like to thank the reviewers for taking the time and effort necessary to review the manuscript. We sincerely appreciate all valuable comments and suggestions which helped us to improve the quality of the manuscript.
Disclosure statement
No potential conflict of interest was reported by the author(s).
Supplementary material
Supplemental data for this article can be accessed online at https://doi.org/10.1080/24750263.2023.2288847.
References
- Ahadiyat A, Ostovan H, Saboori A. 2004. Mites associated with Scolytus amygdali Guerin-Meneville, 1847 in Karaj region. Proceeding of the 16th Iranian Plant Protection Congress, Iran, University of Tabriz. 1: Pests. pp. 251.
- Al-Deeb MA, Muzaffar SB, Abuagla AM, Sharif EM. 2011. Distribution and abundance of phoretic mites (Astigmata, Mesostigmata) on Rhynchophorus ferrugineus (Coleoptera: Curculionidae). Florida Entomologist 94(4):748–755. DOI: 10.1653/024.094.0403.
- Atakan E, Çobanoğlu S, Yüksel O, Bal AD. 2009. Kırmızı palmiyeböceği [Rhynchophorus ferrugineus (Oliver, 1790) (Coleoptera: Curculionidae)] üzerinde foretik uropodid akarlar (Acarina: Uropodidae) (Phoretic uropodid mites (Acarina: Uropodidae) on the red palm weevil (Rhynchophorus ferrugineus) (Olivier, 1790) (Coleoptera: Curculionidae)). Türkiye Entomoloji Dergisi 33(2):93–105.
- Atalla EAR, Hobart J. 1964. The survival of some soil mites at different humidities and their reaction to humidity gradients. Entomologia Experimentalis et Applicata 7(3):215–228. DOI: 10.1111/j.1570-7458.1964.tb02441.x.
- Athias F. 1976. Observations morphologiques sur Polyaspis patavinus Berlese, 1881 (Acariens: Uropodides). I. Morphologie de l’idiosoma au cours du développement postembryonnaire. Acarologia 17(3):410–435.
- Athias-Binche F. 1981. Écologie des Uropodides édaphiques (Arachnides: Parasitifirmes) de trois écosystèmes forestiers. 1. Introduction, matériel, biologie. Vie Milieu 31(2):137–147.
- Athias-Binche F. 1983. Acari from Operation Drake in New Guinea 2. Uropodidae. Acarologia 24(4):361–371.
- Athias-Binche F. 1984. La phorésie chez les acariens uropodides (Anactinotriches), une stratégie écologique originale. Acta Oecologica/Oecologia Generalis 5:119–133.
- Athias-Binche F. 1991. Evolutionary ecology of dispersal in mites. In: Dusbábek F, Bukva V, editors. Modern acarology. Vol. 1. The Hague: Academia, Prague and SPB Academic Publishing bv. pp. 27–41.
- Athias-Binche F. 1993. Dispersal in varying environments: The case of phoretic uropodid mites. Canadian Journal of Zoology 71(9):1793–1798. DOI: 10.1139/z93-255.
- Athias-Binche F. 1994. La phorésie chez les acariens. Aspects adaptatifs et évolutifs. Perpignan, France: Editions du Castillet. p. 178.
- Athias-Binche F, Habersaat U. 1988. An ecological study of Janetiella pyriformis (Berlese, 1920), a phoretic Uropodina from decomposing organic matter (Acari: Anactinotrichida). Mitteilungen Der Schweizerischen Entomologischen Gesellschaft 61:377–390.
- Athias-Binche F, Morand S. 1993. From phoresy to parasitism: The example of mites and nematodes. Research and Reviews in Parasitology 53:73–79.
- Athias-Binche F, Schwarz HH, Meierhofer I. 1993. Phoretic association of Neoseius novus (Ouds., 1902) (Acari: Uropodina) with Nicrophorus spp. (Coleoptera: Silphidae): A case of sympatric speciation? International Journal of Acarology 19(1):75–86. DOI: 10.1080/01647959308683542.
- Bahrami F, Arbabi M, Shoushtari RV, Kazemi S. 2011. Mesostigmatic mites associated with Coleoptera and biodiversity calculation of these mites phoretic on dung beetles in Golestan Province (North of Iran). Middle-East Journal of Scientific Research 9(3):345–366.
- Bajerlein D. 2007. Foreza roztoczy z podrzędu Uropodina na koprofagicznych i koprofilnych chrząszczach [Phoresy of Uropodina mites on coprophagous and coprophilous beetles]. PhD- thesis. Poznań: Adam Mickiewicz University.
- Bajerlein D. 2011. Seasonal abundance and infestation of deutonymphs of Uropoda orbicularis (Müller, 1776) (Acari: Mesostigmata) phoretic on coprophilous beetles (Scarabaeidae, Geotrupidae, Aphodiidae, Hydrophilidae, Histeridae). International Journal of Acarology 37(3):216–227. DOI: 10.1080/01647954.2010.505579.
- Bajerlein D, Błoszyk J. 2004. Phoresy of Uropoda orbicularis by beetles (Coleoptera) associated with cattle dung in Poland. European Journal of Entomology 101(1):185–188. DOI: 10.14411/eje.2004.022.
- Bajerlein D, Przewoźny M. 2005. Coprophagous hydrophilid beetles (Coleoptera: Hydrophilidae) as carriers of phoretic deutonymphs of Uropoda orbicularis (Acari: Mesostigmata) in Poland. European Journal of Entomology 102(1):119–122. DOI: 10.14411/eje.2005.017.
- Bajerlein D, Przewoźny M. 2012. When a beetle is too small to carry phoretic mites? A case of hydrophilid beetles (Coleoptera: Hydrophilidae) and Uropoda orbicularis (Acari: Mesostigmata). Canadian Journal of Zoology 90(3):368–375. DOI: 10.1139/z2012-005.
- Bajerlein D, Witaliński W. 2012. Anatomy and fine structure of pedicellar glands in phoretic deutonymphs of uropodid mites (Acari: Mesostigmata). Arthropod Structure & Development 41(3):245–257. DOI: 10.1016/j.asd.2012.02.006.
- Bajerlein D, Witaliński W. 2014. Localization and density of phoretic deutonymphs of the mite Uropoda orbicularis (Parasitiformes: Mesostigmata) on Aphodius beetles (Aphodiidae) affect pedicel length. Naturwissenschaften 101(4):265–272. DOI: 10.1007/s00114-014-1150-x.
- Bajerlein D, Błoszyk J, Gwiazdowicz DJ, Ptaszyk J, Halliday B. 2006. Community structure and dispersal of mites (Acari, Mesostigmata) in nests of the white stork (Ciconia ciconia). Biologia, Bratislava 61(5):525–530. DOI: 10.2478/s11756-006-0086-9.
- Bajerlein D, Witaliński W, Adamski Z. 2013. Morphological diversity of pedicels in phoretic deutonymphs of Uropodina mites (Acari: Mesostigmata). Arthropod Structure & Development 42(3):185–196. DOI: 10.1016/j.asd.2013.02.002.
- Bajerlein D, Adamski Z, Kacalak W, Tandecka K, Wiesner M, Jurga S. 2016. To attach or not to attach? The effect of carrier surface morphology and topography on attachment of phoretic deutonymphs of Uropoda orbicularis (Acari). The Science of Nature 103(7–8):61. DOI: 10.1007/s00114-016-1385-9.
- Barrios-Torres PL, Villegas-Guzmán GA. 2015. Ácaros asociados a dos especies de Odontotaenius (Coleoptera: Passalidae) en México. Revista de biología tropical 63(3):659–671. DOI: 10.15517/rbt.v63i3.15745.
- Bartlow AW, Agosta SJ. 2021. Phoresy in animals: Review and synthesis of a common but understudied mode of dispersal. Biological Reviews 96(1):223–246. DOI: 10.1111/brv.12654.
- Barton PS, Weaver HJ, Manning AD. 2014. Contrasting diversity dynamics of phoretic mites and beetles associated with vertebrate carrion. Experimental and Applied Acarology 63(1):1–13. DOI: 10.1007/s10493-013-9758-7.
- Beaulieu F. 2012. Saproxyly in predatory mites? Mesostigmata in decaying log habitats versus litter in a wet eucalypt forest, Tasmania, Australia. International Journal of Acarology 38(4):313–323. DOI: 10.1080/01647954.2011.647072.
- Beaulieu F, Dowling APG, Klompen H, de Moraes GJ, Walter DE. 2011. Superorder Parasitiformes Reuter, 1909. In: Zhang Z-Q, editor. Animal biodiversity: An outline of higher-level classification and survey of taxonomic richness. Vol. 3148. Auckland, New Zealand: Magnolia Press. pp. 123–128.
- Berlese A. 1881. Indagini sulle metamorfosi di alcuni Acari insetticoli. Atti del Reale Istituto Veneto di Scienze. Lettere ed Arti Series 5(8):37–81.
- Berlese A. 1884. Acari, Myriopoda et Scorpiones hucusque in Italia reperta,11, 13 text pages + plates 1–10. Padova: Salmin.
- Berlese A. 1887. Acari, Myriapoda et Scorpiones hucusque in Italia reperta, 40: 13 text pages + 10 plates. Firenze: Tipographia del Seminario.
- Berlese A. 1888a. Acari, Myriopoda et Scorpiones hucusque in Italia reperta, 49, 15 text pages + plates 1–10. Firenze: Tipographia del Seminario.
- Berlese A. 1888b. Acari Austro-Americani quos collegit Aloysius Balzan. Manipulus primus. Species novas circiter quinquaginta complectens. Bollettino della Societa Entomologica Italiana 20:171–222. + plates V–XIII.
- Berlese A. 1903. Acari nuovi. Manipulus I.us. Redia 1:235–252.
- Berlese A. 1904a. Acari nuovi. Manipulus II.us. Redia 1:258–280.
- Berlese A. 1904b. Acari nuovi. Manipulus III. Redia 2:10–32. + plates I–II.
- Berlese A. 1904c. Illustrazione iconografica degli Acari mirmecofili. Redia 1:299–474. + plates VII–XX.
- Berlese A. 1910. Brevi diagnosi di generi e specie novi di Acari. Redia 6:346–388.
- Berlese A. 1916a. Centuria prima di Acari nuovi. Redia 12:19–67.
- Berlese A. 1916b. Centuria seconda di Acari nuovi. Redia 12:125–177.
- Berlese A. 1917. Intorno agli Uropodidae. Redia 13:7–16.
- Berlese A. 1920. Centuria quinta di Acari nuovi. Redia 14:143–195.
- Binns ES. 1982. Phoresy as migration - some functional aspects of phoresy in mites. Biological Reviews 57(4):571–620. DOI: 10.1111/j.1469-185X.1982.tb00374.x.
- Błoszyk J. 1999. Geograficzne i ekologiczne zróżnicowanie zgrupowań roztoczy z kohorty Uropodina (Acari: Mesostigmata) w Polsce. I. Uropodina lasów grądowych (Carpinion betuli). Poznań: Kontekst. p. 245.
- Błoszyk J, Bajaczyk R. 1999. The first record of Phaulodinychus borealis (Sellnick, 1940) phoresy (Acari: Uropodina) associated with fleas (Siphonaptera) in mole nests. In: Tajovský K, Pižl V, editors. Soil Zoology in Central Europe; Proceedings of the 5th Central European Workshop on Soil Zoology. České Budějovice, Czech Republic: Institute of Soil Biology, Academy of Sciences of the Czech Republic. pp. 13–17.
- Błoszyk J, Adamski Z, Napierała A, Dylewska M. 2004. Parthenogenesis as a life strategy among mites of the suborder Uropodina (Acari: Mesostigmata). Canadian Journal of Zoology 82(9):1503–1511. DOI: 10.1139/z04-133.
- Błoszyk J, Klimczak J, Leśniewska M. 2006. Phoretic relationships between Uropodina (Acari: Mesostigmata) and centipedes (Chilopoda) as an example of evolutionary adaptation of mites to temporary microhabitats. European Journal of Entomology 103(3):699–707. DOI: 10.14411/eje.2006.093.
- Błoszyk J, Gutowski JM, Gwiazdowicz DJ, Mądra A. 2013. Phoresy of Trichouropoda shcherbakae Hirschmann, 1972 (Acari: Mesostigmata) on beetles of the genus Tetropium Kirby, 1837 (Coleoptera: Cerambycidae) in Białowieża forest, Poland. Central European Journal of Biology 8(10):986–992. DOI: 10.2478/s11535-013-0226-5.
- Błoszyk J, Gutowski JM, Gwiazdowicz DJ, Mądra A, Konwerski S, Książkiewicz Z. 2016. Monochamus sartor (Coleoptera: Cerambycidae) contributes to alpha diversity of Uropodina mites (Acari: Mesostigmata) in first stage of wood decay in Białowieża Primeval forest. International Journal of Acarology 42(4):218–223. DOI: 10.1080/01647954.2016.1154890.
- Brückner A, Klompen H, Bruce AI, Hashim R, von Beeren C. 2017. Infection of army ant pupae by two new parasitoid mites (Mesostigmata: Uropodina). PeerJ 5:e3870. DOI: 10.7717/peerj.3870.
- Cai C, Tihelka E, Giacomelli M, Lawrence JF, Ślipiński A, Kundrata R, Yamamoto S, Thayer MK, Newton AF, Leschen RAB, Gimmel ML, Lü L, Engel MS, Bouchard P, Huang D, Pisani D, Donoghue PCJ. 2022. Integrated phylogenomics and fossil data illuminate the evolution of beetles. Royal Society Open Science 9(3):211771. DOI: 10.1098/rsos.211771.
- Camerik AM. 2010. Phoresy revisited. In: Sabelis M, Bruin J, editors. Trends in Acarology, Proceedings of the 12th International Congress of Acarology, Amsterdam, The Netherlands, Dordrecht Heidelberg London New York, Springer Science+Business Media B.V. pp. 333–336.
- Campbell KU, Klompen H, Crist TO. 2013. The diversity and host specificity of mites associated with ants: The roles of ecological and life history traits of ant hosts. Insectes Sociaux 60(1):31–41. DOI: 10.1007/s00040-012-0262-6.
- Canestrini G, Berlese A. 1884. Sopra alcune nuove specie di Acari Italiani. Atti della Societá Veneto-Trentina di Scienze Naturali 9:175–182 + plates 3–5.
- Čejka M, Holuša J. 2014a. Phoretic mites in uni - and bivoltine populations of Ips typographus: A 1-year case study. Turkish Journal of Zoology 38(5):569–574. DOI: 10.3906/zoo-1309-20.
- Čejka M, Holuša J. 2014b. Foretičtí roztoči lýkožrouta severského Ips duplicatus (Coleoptera: Curculionidae: Scolytinae) z oblasti recentní gradace ve střední Evropě. Lesnicky Časopis – Forestry Journal 60(4):240–243. DOI: 10.1515/forj-2015-0006.
- Chaires-Grijalva MP, Estrada-Venegas EG, Equihua-Martínez A, Moser JC, Sánchez-Martínez G, Vásquez-Rojas IM, Otero-Colina G, Romero-Nápoles J. 2013. Mesostigmados (Acari: Mesostigmata) asociados con Dendroctonus rhizophagus de Chihuahua, México [Mesostigmatid mites (Acari, Mesostigmata) associated to Dendroctonus rhizophagus from Chihuahua, Mexico]. Revista Mexicana De Biodiversidad 84(4):1235–1242. DOI: 10.7550/rmb.35723.
- Chaires-Grijalva MP, Estrada-Venegas EG, Equihua-Martinez A, Moser JC, Blomquist SR. 2016. Trophic habits of mesostigmatid mites associated with bark beetles in Mexico. Journal of the Acarological Society of Japan 25(S1):161–167. DOI: 10.2300/acari.25.Suppl_161.
- Chmielewski W. 1997 1998. Roztocze (Acarina) występujące na pszczołach społecznych (Hymenoptera: Apidae: Apinae, Bombinae). [Mites (Acarina) occurring on social bees (Hymenoptera: Apidae: Apinae, Bombinae)]. Wiadomości Entomologiczne 16(3–4):201–216.
- Chmielewski W. 2000. Powiązania roztoczy z chrząszczami (Acarina-Coleoptera (Insecta)). In: Ignatowicz S, editor. Akarologia polska u progu XXI wieku. Proceedings of the XXVI Acarological Syposium, Kazimierz Dolny, 24-26 October 1999, Warszawa, Poland. pp. 79–90.
- Cilbircioğlu C, Kovač M, Pernek M. 2021. Associations of phoretic mites on bark beetles of the genus Ips in the Black Sea Mountains of Turkey. Forests 12(5):516. DOI: 10.3390/f12050516.
- Constantinescu IC, Cristescu C. 2007. Studies on the trophic preferences of certain Uropodina mites (Acarina: Anactinotrichida, Uropodina) for some taxa of imperfect fungi. Analele Ştiinţifice ale Universităţii ,Al I Cuza” Iaşi, s Biologie animală 53:83–87.
- Cooreman J. 1963. Notes et observations sur quelques Acariens inféodés aux Coléoptères Scolytides de la faune Belge. Bulletin de l’Institut Royal des Sciences Naturelles de Belgique 39:1–48.
- Costa M. 1963. The mesostigmatic mites associated with Copris hispanus (L.) (Coleoptera, Scarabaeidae) in Israel. Journal of the Linnaean Society of London, Zoology 45(303):25–45. DOI: 10.1111/j.1096-3642.1963.tb00485.x.
- Costa M. 1966. The present stage of knowledge of mesostigmatic mites in Israel (Acari, Mesostigmata). Israel Journal of Zoology 15(2):69–82.
- Danilevsky M. 2020. Catalogue of Palaearctic Coleoptera. Volume 6/1. Chrysomeloidea I (Vesperidae, Disteniidae, Cerambycidae). Updated and revised second. Leiden, Boston: Brill. xxvii +. p. 712.
- De Geer C. 1768. Djur, som med en sträng i ändan äro säste vid andra lesvande djur [Animals that, with a string at the end, are attached to other living animals]. Kongliga Vetenskaps Academiens Handlingar 29:176–183 + Plate IV.
- Delfinado-Baker M, Rath W, Boecking O. 1992. Phoretic bee mites and honeybee grooming behavior. International Journal of Acarology 18(4):315–322. DOI: 10.1080/01647959208683966.
- Desender K, Vaneechoutte M. 1984. Phoretic associations of carabid beetles (Coleoptera, Carabidae) and mites (Acari). Revue d’Ecologie et Biologie du Sol 21:363–371.
- Dilipkumar M, Ahadiyat A, Mašán P, Chuah TS. 2015. Mites (Acari) associated with Rhynchophorus ferrugineus (Coleoptera: Curculionidae) in Malaysia, with a revised list of the mites found on this weevil. Journal of Asia-Pacific Entomology 18(2):169–174. DOI: 10.1016/j.aspen.2014.12.010.
- Domrow R. 1981. A small lizard stifled by phoretic deutonymphal mites (Uropodina). Acarologia 22(3):247–252.
- Dunlop JA, Kontschán J, Zwanzig M. 2013. Fossil mesostigmatid mites (Mesostigmata: Gamasina, Microgyniina, Uropodina) associated with longhorn beetles (Coleoptera: Cerambycidae) in Baltic amber. Naturwissenschaften 100(4):337–344. DOI: 10.1007/s00114-013-1031-8.
- Eickwort GC. 1990. Associations of mites with social insects. Annual Review of Entomology 35(1):469–488. DOI: 10.1146/annurev.en.35.010190.002345.
- El-Banhawy EM, El-Borolossy MA, El-Sawaf BM, Afia SI. 1997. Biological aspects and feeding behaviour of the predacious soil mite Nenteria hypotrichus (Uropodina: Uropodidae). Acarologia 38(4):357–360.
- Elzinga RJ. 1978. Holdfast mechanisms in certain Uropodine mites (Acarina: Uropodina). Annals of the Entomological Society of America 71(6):896–900. DOI: 10.1093/aesa/71.6.896.
- Faasch H. 1967. Beitrag zur Biologie der einheimischen Uropodiden Uroobovella marginata (C. L. Koch 1839) und Uropoda orbicularis (O. F. Müller 1776) und experimentelle Analyse ihres Phoresieverhaltens. Zoologische Jahrbücher, Abteilung für Systematik 94:521–608.
- Fain A. 1998a. Description of mites (Acari) phoretic on Phoridae (Insecta: Diptera) with description of four new species of the genus Uroseius Berlese (Parasitiformes, Uropodina, Polyaspididae). International Journal of Acarology 24(3):213–220. DOI: 10.1080/01647959808683586.
- Fain A. 1998b. New mites (Acari) phoretic on Phoridae and Ephydridae (Diptera) from Thailand. Bulletin de L’institut Royal Des Sciences Naturelles de Belgique Entomologie 68:53–61.
- Fain A, Greenwood MT. 1991. Notes on a small collection of mites (Acari), phoretic on Diptera, mainly Phoridae, from the British Isles. Bulletin de L’institut Royal Des Sciences Naturelles de Belgique Entomologie 61:193–197.
- Farish JD, Axtell RC. 1971. Phoresy redefined and examined in Macrocheles muscaedomesticae (Acarina: Macrochelidae). Acarologia 13(1):16–29.
- Farmahiny Farahani VR, Ahadiyat A, Mašán P, Dehvari MA. 2016. Phoretic uropodine mites (Acari: Mesostigmata) associated with the red palm weevil, Rhynchophorus ferrugineus (Coleoptera: Curculionidae) in Iran. Journal of Entomological and Acarological Research 48(3):5853. DOI: 10.4081/jear.2016.5853.
- Feketeová Z. 2011a. Lykožrút smrekový (Ips typographus) a jeho úloha vo forézii uropodných roztočov (Acari: Uropodina). Entomofauna carpathica 23(1):23–30.
- Feketeová Z. 2011b. Význam Uropodných roztočov (Acari: Uropodina) v ekológii lykožrúta smrekového (Ips typographus). Entomofauna carpathica 23(2):11–19.
- Fernández M, Diez J, Moraza ML. 2013. Acarofauna associated with Ips sexdentatus in northwest Spain. Scandinavian Journal of Forest Research 28(4):358–362. DOI: 10.1080/02827581.2012.745897.
- Goff ML. 1989. Gamasid mites as potential indicators of postmortem interval. In: Channabasavanna G, Viraktamath C, editors. Progress in acarology-volume 1. New Delhi, India: Oxford and IBH. pp. 443–450.
- Goff ML. 1991. Use of Acari in establishing a postmortem interval in a homicide case on the island of Oahu, Hawaii. In: Dusbábek F, Bukva V, editors. Modern acarology. Academia, Prague and SPB. Vol. 1. The Hague, Netherlands: Academic Publishing bv. pp. 439–442.
- Gómez-Marco F, Klompen H, Hoddle MS. 2021. Phoretic mite infestations associated with Rhynchophorus palmarum (Coleoptera: Curculionidae) in Southern California. Systematic & Applied Acarology 26(10):1913–1926. DOI: 10.11158/saa.26.10.6.
- Gordh G. 1985. Uropoda sp. phoretic on Elater lecontei Horn. Pan - Pacific Entomologist 6:154.
- Gordh G, Barrows EM. 1976. Uropoda phoretic on Ceratina (Acarina: Hymenoptera). Journal of the Kansas Entomological Society 49(3):344–345.
- Gordh G, Wills L. 1989. Anatomical notes on Uropoda sp., a phoretic mite infesting dung- inhabiting beetles in Southern California (Acari: Uropodidae; Coleoptera: Tenebrionidae, Histeridae). Pan-Pacific Entomologist 65(4):410–413.
- Gwiazdowicz DJ. 2000. Mites (Acari: Gamasida) associated with insects in the Białowieża National Park. Acta Parasitologica 45(1):43–47. DOI: 10.3161/00159301FF2000.43.8.091.
- Gwiazdowicz DJ, Gutowski JM. 2012. Records of phoretic mesostigmatid mites (Acari: Mesostigmata) on beetles (Coleoptera: Carabidae, Cerambycidae, Elateridae, Erotylidae, Scolytinae) in the Białowieża Primeval forest. Polish Journal of Entomology 81(4):305–310. DOI: 10.2478/v10200-012-0010-2.
- Gwiazdowicz DJ, Kamczyc J, Błoszyk J. 2011. The diversity of phoretic Mesostigmata on Ips typographus (Coleoptera: Scolytinae) caught in the Karkonosze forest. European Journal of Entomology 108(3):489–491. DOI: 10.14411/eje.2011.063.
- Gwiazdowicz DJ, Kamczyc J, Teodorowicz E, Błoszyk J. 2012. Mite communities (Acari, Mesostigmata) associated with Ips typographus (Coleoptera, Scolytidae) in managed and natural Norway spruce stands in Central Europe. Central European Journal of Biology 7:910–916. DOI: 10.2478/s11535-012-0070-z.
- Gwiazdowicz DJ, Gutowski JM, Kamczyc J, Teodorowicz E. 2013. Phoretic relationships between Plagionotus detritus (Coleoptera: Cerambycidae) and Trichouropoda sociata (Acari: Mesostigmata). Entomologica Fennica 24(1):59–64. DOI: 10.33338/ef.7868.
- Gwiazdowicz DJ, Błoszyk J, Gdula AK. 2015. Alpha diversity of mesostigmatid mites associated with the bark beetle Ips typographus (L.) in Poland. Insect Conservation and Diversity 8(5):448–455. DOI: 10.1111/icad.12122.
- Halliday RB. 2015. Catalogue of genera and their type species in the mite suborder Uropodina (Acari: Mesostigmata). Zootaxa 3972(2):101–147. DOI: 10.11646/zootaxa.3972.2.1.
- Halliday RB. 2016. Catalogue of families and their type genera in the mite suborder Uropodina (Acari: Mesostigmata). Zootaxa 4061(4):347–366. DOI: 10.11646/zootaxa.4061.4.2.
- Halliday B. 2019. The enemy of my parasite is my friend: The possible role of predatory mites as biological control agents of pest beetles in soil. International Journal of Acarology 45(4):189–196. DOI: 10.1080/01647954.2019.1574895.
- Helson GAH, Flower NE, Wood J. 1975. Phoretic association of a uropodid mite and a lepidopteran larva. New Zealand Journal of Zoology 2(2):223–226. DOI: 10.1080/03014223.1975.9517872.
- Hirschmann W. 1972a. Gangsystematik der Parasitiformes. Teil 92. Gänge, Teilgänge, Stadien von 13 neuen Trichouropoda-Arten (Trichouropodini, Uropodinae). Acarologie Schriftenreihe für Vergleichende Milbenkunde 17:3–8 + plates 1–3.
- Hirschmann W. 1972b. Gangsystematik der Parasitiformes. Teil 93. Gänge, Teilgang, Stadien von 7 neuen Uroobovella-Arten (Dinychini, Uropodinae). Acarologie Schriftenreihe für Vergleichende Milbenkunde 17:9–13 + plates 3–4.
- Hirschmann W. 1975. Gangsystematik der Parasitiformes. Teil 193. Teilgang einer neuen Trichouropoda-Art (Trichouropodini, Uropodinae). Acarologie Schriftenreihe für Vergleichende Milbenkunde 21:10–12. + plate 9.
- Hirschmann W. 1979. Stadiensystematik der Parasitiformes. Teil 1. Stadienfamilien und Stadiengattungen der Atrichopygidiina, erstellt im Vergleich zum Gangsystem Hirschmann 1979. Acarologie Schriftenreihe für Vergleichende Milbenkunde 26:57–70.
- Hirschmann W. 1981. Gangsystematik der Parasitiformes. Teil 420. Teilgang und Stadien von 4 neuen Uroobovella-Arten der Pulchella- und Carinata-Gruppe aus Belgien, Zaire, USA und Vietnam (Dinychini, Uropodinae) Acarologie. Schiftenreihe für vergleichende Milbenkunde 28:125–127.
- Hirschmann W. 1985. Gangsystematik der Parasitiformes. Teil 489. Stadien von 2 neuen Nenteria-Arten der rühmi-Gruppe an Pyragra (Dermaptera) aus Argentinien und Bolivien (Trichouropodini, Uropodinae) Acarologie. Schiftenreihe für vergleichende Milbenkunde 32:163–166.
- Hirschmann W. 1989. Gangsystematik der Parasitiformes. Teil 509. Die Ganggattung Uroobovella Berlese 1903 – Artengruppen – Bestimmungstabellen – Diagnosen – (Dinychini, Uropodinae). Acarologie Schiftenreihe für vergleichende Milbenkunde 36:84–196.
- Hirschmann W, Kemnitzer F. 1989. Polyaspis (Polyaspis)-Deutonymphen Polyaspis-Wandernymphentraube an Solitärwespe (Scoliidae) (Atrichopygidiina: Uropodina). Acarologia 30(1):3–12.
- Hirschmann W, Wiśniewski J. 1985. Gangsystematik der Parasitiformes. Teil 486. Die rühmi-Gruppe, eine neue Adulten-Gruppe der Ganggattung Nenteria Gang Teilgänge, Stadien von 12 neuen Nenteria-Arten aus Israel, Venezuela, Ekuador, Guatemala, Tanzania, Rwanda, Java, Sumatra, Laos, Neuguinea, Neu-Britannien. Neuzeichnungen und Ergänzungsbeschreibungen von 3 bekannten Nenteria-Arten (Trichouropodini, Uropodinae) Acarologie Schiftenreihe für vergleichende Milbenkunde 32:96–132.
- Hirschmann W, Wiśniewski J. 1986. Gangsystematik der Parasitiformes. Teil 495. Weltweite Revision der Ganggattung Trichouropoda Berlese 1916. V. Die obscura-Gruppe (Trichouropodini, Uropodinae). Acarologie Schriftenreihe für Vergleichende Milbenkunde 33:149–176.
- Hirschmann W, Wiśniewski J. 1987. Gangsystematik der Parasitiformes. Teil 497. Weltweite Revision der Ganggattung Trichouropoda Berlese, 1916 VII. Die sociata-Gruppe (Trichouropodini, Uropodinae). Acarologie Schiftenreihe für vergleichende Milbenkunde 34:51–132.
- Hirschmann W, Wiśniewski J. 1989. Gangsystematik der Parasitiformes. Teil 507-508. Weltweite Revision der Gattung Trichouropoda Berlese 1916 Teil IV. Acarologie Schiftenreihe für vergleichende Milbenkunde 36:1–72.
- Hirschmann W, Zirngiebl-Nicol I. 1961. Gangsystematik der Parasitiformes. Teil 4. Die Gattung Trichouropoda Berlese 1916 nov. comb., die Cheliceren und das System der Uropodiden. Acarologie Schriftenreihe für Vergleichende Milbenkunde 4:1–41 + plates 1–16.
- Hirschmann W, Zirngiebl-Nicol I. 1969. Gangystematik der Parasitiformes. Teil 73. Neuzeichung bekannter Uropodiden-Arten, Gänge, Teilgänge, Stadien, Chaetotaxie, Literatur, Synonyma, Fundorte, Grösse. Acarologie Schiftenreihe für vergleichende Milbenkunde 12:125–132.
- Hofstetter RW, Moser JC, Blomquist SR. 2013. Mites associated with bark beetles and their hyperphoretic ophiostomatoid fungi. In: Seifert K, De Beer Z, Wingfield M, editors. The ophiostomatoid fungi: Expanding frontiers. Vol. 12. Utrecht: CBS-KNAW Fungal Biodiversity Centre. pp. 165–176.
- Holuša J, Čejka M. 2020. Estimates of phoretic mite abundance on bark beetles as affected by beetle capture method: A case study with Mesostigmata mites and Ips typographus (Coleoptera: Curculionidae). Experimental and Applied Acarology 82(3):347–357. DOI: 10.1007/s10493-020-00560-9.
- Houck MA, OConnor BM. 1991. Ecological and evolutionary significance of phoresy in the Astigmata. Annual Reviews in Entomology 36(1):611–636. DOI: 10.1146/annurev.en.36.010191.003143.
- Hunter PE, Rosario RMT. 1988. Associations of Mesostigmata with other arthropods. Annual Review of Entomology 33(1):393–417. DOI: 10.1146/annurev.en.33.010188.002141.
- Hyatt KH. 1959. Mesostigmatic mites associated with Geotrupes stercorarius (L.) (Col., Scarabaeidae). Entomologist’s Monthly Magazine 95:22–23.
- Ibrahim M, Loulou A, Brouk A, Muller A, Machado RAR, Kallel S. 2021. Parasites rather than phoronts: Teratorhabditis synpapillata nematodes reduce lifespan of their Rhynchophorus ferrugineus host in a life stage-dependent manner. Ecology and Evolution 11(18):12596–12604. DOI: 10.1002/ece3.8004.
- Iwan D, Löbl I. 2020. Catalogue of Palaearctic Coleoptera. Volume 5. Tenebrionoidea. Revised and updated second. Leiden, Boston: Brill xxiv +. p. 945.
- Jałoszyński P. 2015. Ptenidium pusillum (Gyllenhal, 1808) from egg to pupa (Coleoptera: Ptiliidae). Zootaxa 3948(3):361–421. DOI: 10.11646/zootaxa.3948.3.3.
- Kamczyc J, Gwiazdowicz DJ. 2013. The diversity of soil mites (Acari: Mesostigmata) in yellow ant (Lasius flavus) nests along a gradient of land use. Biologia 68(2):314–318. DOI: 10.2478/s11756-013-0154-x.
- Kapuściński S, Wiśniewski J. 1985. Wandernymphen von Milben als Passagiere auf Käfern. Mikrokosmos 74(6):161–163.
- Karg W. 1989. Acari (Acarina), Milben. Unterordnung Parasitiformes (Anactinochaeta). Uropodina Kramer, Schlidkrötenmilben. Die Tierwelt Deutschlands 67:1–203.
- Khaustov AA, Trach VA, Bobylev AN. 2016. Mites (Acari) phoretic on six-toothed spruce bark beetle, Pityogenes chalcographus Linnaeus (Coleoptera: Curculionidae: Scolytinae), in western Siberia, Russia. Acarina 24(2):137–151. DOI: 10.21684/0132-8077-2016-24-2-137-151.
- Khaustov AA, Klimov PB, Trach VA, Bobylev AN, Salavatulin VM, Khaustov VA, Tolstikov AV. 2018. Review of mites (Acari) associated with the European spruce bark beetle, Ips typographus (Coleoptera: Curculionidae: Scolytinae) in Asian Russia. Acarina 26(1):3–79. DOI: 10.21684/0132-8077-2018-26-1-3-79.
- Kinn DN. 1982. Seasonal distribution of three common mite associates of the southern pine beetle (Coleoptera: Scolytidae) in central Louisiana. The Florida Entomologist 65(1):185–187. DOI: 10.2307/3494161.
- Kinn DN, Linit MJ. 1989. A key to phoretic mites commonly found on long-horned beetles emerging from Southern Pines. New Orleans, LA, USA: United States Department of Agriculture, Forest Service Southern Forest Experiment Station. Research Note SO-357. pp. 1–8. DOI: 10.2737/SO-RN-357.
- Kinn DN, Witcosky JJ. 1978. Variation in southern pine beetle attack height associated with phoretic uropodid mites. The Canadian Entomologist 110(3):249–251. DOI: 10.4039/Ent110249-3.
- Klausnitzer B. 2018. Schildkrötenmilben (Uropodina) an Calvia decemguttata (Linnaeus, 1767) (Coleoptera, Coccinellidae). Entomologische Nachrichten und Berichte 62:71–72.
- Knee W, Beaulieu F, Skevington JH, Kelso S, Cognato AI, Forbes MR, Shaw P. 2012a. Species boundaries and host range of tortoise mites (Uropodoidea) phoretic on bark beetles (Scolytinae), using morphometric and molecular markers. PloS One 7(10):e47243. DOI: 10.1371/journal.pone.0047243.
- Knee W, Beaulieu F, Skevington JH, Kelso S, Forbes MR. 2012b. Cryptic species of mites (Uropodoidea: Uroobovella spp.) associated with burying beetles (Silphidae: Nicrophorus): The collapse of a host generalist revealed by molecular and morphological analyses. Molecular and Phylogenetics and Evolution 65(1):276–286. DOI: 10.1016/j.ympev.2012.06.013.
- Knee W, Forbes MR, Beaulieu F. 2013. Diversity and host use of mites (Acari: Mesostigmata, Oribatida) phoretic on bark beetles (Coleoptera: Scolytinae): Global generalists, local specialists? Annals of the Entomological Society of America 106(3):339–350. DOI: 10.1603/AN12092.
- Knee W, Hartzenberg T, Forbes MR, Beaulieu F. 2012c. The natural history of mites (Acari: Mesostigmata) associated with the white-spotted sawyer beetle (Coleoptera: Cerambycidae): Diversity, phenology, host attachment, and sex bias. The Canadian Entomologist 144(5):711–719. DOI: 10.4039/tce.2012.57.
- Kočárek P. 2003. Decomposition and Coleoptera succession on exposed carrion of small mammal in Opava, the Czech Republic. European Journal of Soil Biology 39(1):31–45. DOI: 10.1016/S1164-5563(02)00007-9.
- Koch CL. 1835. Deutschlands Crustaceen, Myriapoden und Arachniden. Ein Beitrag zur Deutschen Fauna. G.A.W Herrich‐Schäffer, editor. Regensberg: Putset. 2:25 un-numbered text pages + 24 plates.
- Koch CL. 1840. Deutschlands Crustaceen, Myriapoden und Arachniden. Ein Beitrag zur Deutschen Fauna. G.A.W Herrich‐Schäffer, editor. Regensberg: Montag & Weiβ. 27:25 un-numbered text pages + 24 plates.
- Kofler A, Schmölzer K. 2000. Zur Kenntnis phoretischer Milben und ihrer Tragwirte in Österreich (Acarina: Gamasina: Uropodina). Berichte des Naturwissenschaftlich-medizinischen Vereins in Innsbruck 87:133–157.
- Kontschán J, Tambe JT, Riolo P. 2012. Uroobovella phoenicicola sp. n., a new Uropodina mite (Acari: Mesostigmata) associated with the African palm weevil (Rhynchophorus phoenicis Fabricius, 1801) from Cameroon. African Invertebrates 53(2):593–600. DOI: 10.5733/afin.053.0205.
- Kontschán J, Mazza G, Nannelli R, Roversi PF. 2014a. Nenteria extremica n. sp., a new Uropodina mites (Acari Mesostigmata) collected on Rhynchophorus ferrugineus in Italy, with notes on other Uropodina mites associated with red palm Weevil. Redia 97:63–69.
- Kontschán J, Mazza G, Nannelli R, Roversi PF. 2014b. The true identity of the red palm weevil associated Uropodina mite, Centrouropoda almerodai Hiramatsu & Hirschmann. Redia 97:83–88.
- Konwerski S, Gutowski JM, Książkiewicz-Parulska Z, Błoszyk J. 2017. Repeatability of the phoretic relationships between mites of the genus Trichouropoda Berlese (Parasitiformes: Uropodina) and longhorn beetles of the genus Tetropium Kirby (Coleoptera: Cerambycidae) in Białowieża Primeval forest, Central Europe. International Journal of Acarology 43(8):612–621. DOI: 10.1080/01647954.2017.1379558.
- Konwerski S, Gutowski JM, Błoszyk J. 2019. Analysis of the phoretic relationships between mites of the genus Trichouropoda Berlese (Parasitiformes: Uropodina) and the longhorn beetle Plagionotus detritus (Linnaeus) (Coleoptera: Cerambycidae) based on multiannual observations in Białowieża Primeval forest, Central Europe. International Journal of Acarology 45(1–2):29–40. DOI: 10.1080/01647954.2018.1538257.
- Konwerski S, Gutowski JM, Błoszyk J. 2020. Patterns of distribution of phoretic deutonymphs of Uropodina on longhorn beetles in Białowieża Primeval Forest, Central Europe. Diversity 12(6):239. DOI: 10.3390/d12060239.
- Kotiaho JS, Simmons LW. 2001. Effects of Macrocheles mites on longevity of males of the dimorphic dung beetle Onthophagus binodis. Journal of Zoology (London, England) 254(4):441–445. DOI: 10.1017/S0952836901000930.
- Kramer P. 1886. Ueber Milben. Archiv für Naturgeschichte 52:241–268. + plate XII. DOI: 10.5962/bhl.part.28438.
- Krištofík J, Mašán P, Šustek Z, Gajdoš P. 1993. Arthropods in the nests of penduline tit (Remiz pendulinus). Biologia, Bratislava 48(5):493–505.
- Kršiak B, Zach P, Kulfan J. 2010. The role of Hylastes cunicularius Erichson (Coleoptera: Scolytidae) in transferring uropodine mites in a mountain spruce forest. Journal of Forest Science 56(6):258–264. DOI: 10.17221/81/2009-JFS.
- Kuhlmann M. 1998. Nachweise mit Bienen und Wespen (Hymenoptera Aculeata) assoziierter Milben (Acari) und Fächerflügler (Strepsiptera). Linzer Biologische Beiträge 30(1):69–80.
- Lachaud J-P, Klompen H, Pérez-Lachaud G. 2016. Macrodinychus mites as parasitoids of invasive ants: An overlooked parasitic association. Scientific Reports 6:29995. DOI: 10.1038/srep29995.
- Latreille PA. 1806. Genera Crustaceorum et Insectorum secundum ordinem naturalem in famlias disposita, iconibus exemplisque plurimus explicita. Vol. 1. König, Paris. xviii + 302. p. + 16 plates. DOI: 10.5962/bhl.title.65741.
- Lehtinen PT. 1987. Association of uropodid, prodinychid, polyaspidid, antennophorid, sejid, microgynid, and zerconid mites with ants. Entomologisk Tidskrift 108:13–20.
- Levieux J, Lieutier F, Moser JC, Perry TJ. 1989. Transportation of phytopathogenic fungi by the bark beetle Ips sexdentatus Boerner and associated mites. Journal of Applied Entomology 108(1–5):1–11. DOI: 10.1111/j.1439-0418.1989.tb00425.x.
- Lieutier F. 1978. Les acariens associés a Ips typographus et Ips sedentatus (Coleoptera: Scolytidae) en région parisienne et les variations de leurs populations au cours du cycle annuel. Bulletin de la Société d’Ecologie 9(4):307–321.
- Löbl I, Löbl D. 2015. Catalogue of Palaearctic Coleoptera. Hydrophiloidea – Staphylinoidea. Volume 2. Revised and updated ed. Leiden, Boston: Brill. xxvi +. p. 1702.
- Löbl I, Löbl D. 2016. Catalogue of Palaearctic Coleoptera. Volume 3. Scarabaeoidea – Scirtoidea – Dascilloidea – Buprestoidea – Byrrhoidea. Revised and updated ed. Leiden, Boston: Brill. xxviii +. p. 983.
- Löbl I, Löbl D. 2017. Catalogue of Palaearctic Coleoptera. Volume 1. Archostemata – Myxophaga – Adephaga. Revised and updated ed. Leiden, Boston: Brill. xxxiv +. p. 1443.
- Löbl I, Smetana A. 2007. Catalogue of Palaearctic Coleoptera. Volume 4. Elateroidea, Derodontoidea, Bostrichoidea, Lymexyloidea, Cleroidea, Cucujoidea. Stenstrup: Apollo Books. p. 935.
- Löbl I, Smetana A. 2010. Catalogue of Palaearctic Coleoptera. Volume 6. Chrysomeloidea. Stenstrup: Apollo Books. p. 924.
- Löbl I, Smetana A. 2011. Catalogue of Palaearctic Coleoptera. Volume 7. Curculionoidea I. Stenstrup: Apollo Books. pp. 373.
- Löbl I, Smetana A. 2013. Catalogue of Palaearctic Coleoptera. Volume 8. Curculionoidea II. Leiden: Brill. p. 707.
- Longo S, Ragusa Di Chiara S. 2006. Presenza e diffusione in Italia dell’acaro Centrouropoda almerodai (Uroactiniinae, Uropodina). Bollettino di Zoologia Agraria e di Bachicoltura 38(3):265–269.
- Longo S, Suma P, Bella S, La Pergola A. 2009. Artropodi associate al Punteruolo rosso delle palme. Regione Siciliana—Assessorato Agricoltura e Foreste Dipartimento Interventi Infrastrutturali—Servizi allo Sviluppo, editor La ricerca scientifica sul Punteruolo rosso e gli altri fitofagi delle palme in Sicilia 1:61–64.
- Loulou A, Fakhraoui A, M’rabet‑Samaali B, Chihani‑Hammas N, Kallel S. 2023. Interactions between microorganisms associated with red palm weevil, Rhynchophorus ferrugineus, in Tunisia. Euro-Mediterranean Journal for Environmental Integration 8(3):493–505. DOI: 10.1007/s41207-023-00382-2.
- Majka CG, Behan-Pelletier V, Bajerlein D, Błoszyk J, Krantz GW, Lucas Z, OConnor B, Smith IM. 2007. New records of mites (Arachnida: Acari) from Sable Island, Nova Scotia, Canada. The Canadian Entomologist 139(5):690–699. DOI: 10.4039/n06-103.
- Makarova OL. 1995. Mesostigmatic mites (Parasitiformes, Mesostigmata) on the forest dung beetle Geotrupes stercorosus. Zoologicheskii Zhurnal. 74(12):16–23.
- Mašán P. 1993. Mites (Acarina) associated with species of Trox (Coleoptera: Scarabaeidae). European Journal of Entomology 90:359–364.
- Mašán P. 1994. The mesostigmatic mites (Acarina, Mesostigmata) associated with the dung beetles (Coleoptera, Scarabaeidae) in South Slovakia. Biologia, Bratislava 49:201–205.
- Mašán P. 1998. Description of the female of Uropoda copridis (Acarina, Mesostigmata, Uropodina). Biologia, Bratislava 53:651–653.
- Măsán P. 1999. New mite species of the cohort Uropodina (Acarina, Mesostigmata) from Slovakia. Biologia, Bratislava 54(2):121–133.
- Mašán P. 2001. Mites of the cohort Uropodina (Acarina, Mesostigmata) in Slovakia. Annotationes Zoologicae et Botanicae 223:1–321.
- Mašán P, Halliday B. 2009. Mesostigmatid mites associated with the dung beetle Copris lunaris (Coleoptera: Scarabaeidae). European Journal of Entomology 106(4):545–550. DOI: 10.14411/eje.2009.068.
- Matos I, Silva D, Oliveira J, Gonçalves C, Alves R, Pereira N, Catarino F, Ameixa OMCC, Sousa JA, Rangel LF, Santos MJ, Ayra-Pardo C. 2023. Body size-dependent effects on the distribution patterns of phoretic mite species assemblages on Rhynchophorus ferrugineus (Olivier, 1790). Ecology and Evolution 13(7):e10338. DOI: 10.1002/ece3.10338.
- Mazza G, Cini A, Cervo R, Longo S. 2011. Just phoresy? Reduced lifespan in red palm weevils Rhynchophorus ferrugineus (Coleoptera: Curculionidae) infested by the mite Centrouropoda almerodai (Uroactiniinae: Uropodina). Italian Journal of Zoology 78(1):101–105. DOI: 10.1080/11250003.2010.509135.
- Mazza G, Francardi V, Simoni S, Benvenuti C, Cervo R, Faleiro JR, Llácer E, Longo S, Nannelli R, Tarasco E, Roversi PF. 2014. An overview on the natural enemies of Rhynchophorus palm weevils, with focus on R ferrugineus. Biological Control 77:83–92. DOI: 10.1016/j.biocontrol.2014.06.010.
- McGarry JW, Baker AS. 1997. Observations on the mite fauna associated with adult Stomoxys calcitrans in the U.K. Medical and Veterinary Entomology 11(2):159–164. DOI: 10.1111/j.1365-2915.1997.tb00307.x.
- Mertins JW, Hartdegen RW. 2003. The ground skink, Scincella lateralis, an unusual host for phoretic deutonymphs of a uropodine mite, Fuscuropoda marginata with a review of analogous mite-host interactions. The Texas Journal of Science 55(1):33–42.
- Michael AD. 1894. Notes on the Uropodinæ. Journal of the Royal Microscopical Society 1894(3):289–319 + plates VI–VII. DOI: 10.1111/j.1365-2818.1894.tb00029.x.
- Michalski J, Ratajczak E, Wiśniewski J. 1985. Roztocze (Acarina: Mesostigmata) towarzyszące kornikom (Coleptera: Scolytidae) Gór Świętokrzyskich. Prace Komisji Nauk Rolniczych i Komisji Nauk Leśnych 60:85–92.
- Miko L, Stanko M. 1991. Small mammals as carriers of non-parasitic mites (Oribatida, Uropodina). In: Dusbábek F, Bukva V, editors. Modern acarology. Vol. 1. The Hague: Academia, Prague and SPB Academic Publishing bv. pp. 395–402.
- Monzenga Lokela JC, Le Goff GJ, Kayisu K, Hance T. 2021. Phoretic mites associated with Rhynchophorus phoenicis Fabricius (1880) (Coleoptera: Curculionidae) in the Kisangani region, D. R Congo Acarologia 61(2):291–296. DOI: 10.24349/acarologia/20214431.
- Moraza ML, Fernández M, Jurc M. 2013. Phoretic mites of the six-spined engraver beetle, Ips sexdentatus (Böerner, 1776) (Coleoptera, Scolytinae), on Pinus halepensis in Slovenia. International Journal of Acarology 39(8):597–599. DOI: 10.1080/01647954.2013.867900.
- Morton A, García-Del-Pino F. 2011. Possible interaction of the phoretic mite Centrouropoda almerodai on the control of Rhynchophorus ferrugineus by entomopathogenic nematodes. IOBC-WPRS Bulletin. 66:363–366.
- Moser JC. 1976a. Phoretic carrying capacity of flying southern pine beetles (Coleoptera: Scolytidae). The Canadian Entomologist 108(8):807–808. DOI: 10.4039/ent108807-8.
- Moser JC. 1976b. Surveying mites (Acarina) phoretic on the southern pine beetle (Coleoptera: Scolytidae) with sticky traps. The Canadian Entomologist 108(8):809–813. DOI: 10.4039/Ent108809-8.
- Moser JC, Blomquist SR. 2011. Phoretic arthropods of the red imported fire ant in Central Louisiana. Annals of the Entomological Society of America 104(5):886–894. DOI: 10.1603/AN11010.
- Moser JC, Bogenschütz H. 1984. A key to the mites associated with flying Ips typographus in South Germany. Zeitschrift für Angewandte Entomologie 97(1–5):437–450. DOI: 10.1111/j.1439-0418.1984.tb03774.x.
- Moser JC, Eidmann HH, Regnander JR. 1989. The mites associated with Ips typographus in Sweden. Annales Entomologici Fennici 55:23–27.
- Moser JC, Konrad H, Blomquist SR, Kirisits T. 2010. Do mites phoretic on elm bark beetles contribute to the transmission of Dutch elm disease? Naturwissenschaften 97:219–227. DOI: 10.1007/s00114-009-0630-x.
- Moser JC, Konrad H, Kirisits T, Carta LK. 2005. Phoretic mites and nematode associates of Scolytus multistriatus and Scolytus pygmaeus (Coleoptera: Scolytidae) in Austria. Agricultural and Forest Entomology 7(2):169–177. DOI: 10.1111/j.1461-9555.2005.00261.x.
- Moser JC, Perry TJ, Furuta K. 1997. Phoretic mites and their hyperphoretic fungi associated with flying Ips typographus japonicus Niijima (Col., Scolytidae) in Japan. Journal of Applied Entomology 121(1–5):425–428. DOI: 10.1111/j.1439-0418.1997.tb01429.x.
- Moser JC, Roton LM. 1971. Mites associated with southern pine bark beetles in Allen Parish, Louisiana. The Canadian Entomologist 103(12):1775–1798. DOI: 10.4039/Ent1031775-12.
- Müller OF. 1776. Zoologiae Danicae Prodromus, seu Animalium Daniae et Norvegicae Indigenarum. Characteres, Nomina, et Synonyma Imprimis Popularum. Hafniae, Copenhagen. p. 282. DOI: 10.5962/bhl.title.13268.
- Napierała A, Błoszyk J. 2013. Unstable microhabitats (merocenoses) as specific habitats of Uropodina mites (Acari: Mesostigmata). Experimental and Applied Acarology 60(2):163–180. DOI: 10.1007/s10493-013-9659-9.
- Napierała A, Książkiewicz Z, Leśniewska M, Gwiazdowicz DJ, Mądra A, Błoszyk J. 2015. Phoretic relationships between uropodid mites (Acari: Mesostigmata) and centipedes (Chilopoda) in urban agglomeration areas. International Journal of Acarology 41(4):250–258. DOI: 10.1080/01647954.2015.1029968.
- Noriega JA, Halliday B, Weston P, Thotagamuwa A, Gurr G. 2022. Beyond phoresy: Symbioses between dung beetles (Coleoptera: Scarabaeidae: Scarabaeinae) and mites (Acari). Entomologia Generalis 42(4):499–513. DOI: 10.1127/entomologia/2021/1406.
- O’Donnell AE, Axtell RC. 1965. Predation by Fuscuropoda vegetans (Acarina: Uropodidae) on the house fly (Musca domestica). Annals of the Entomological Society of America 58(3):403–404. DOI: 10.1093/aesa/58.3.403.
- O’Donnell AE, Nelson EL. 1967. Predation by Fuscuropoda vegetans (Acarina: Uropodidae) and Macrocheles muscaedomesticae (Acarina: Macrochelidae) on the eggs of the little house fly, Fannia canicularis. Journal of the Kansas Entomological Society 40(3):441–443.
- Oudemans AC. 1902. New list of Dutch Acari. Second part. Tijdschrift voor Entomologie 45:1–52 + plates 1–6.
- Oudemans AC. 1915. Acarologische Aanteekeningen. LVI. Entomologische berichten 4(83):180–188.
- Oudemans AC. 1916. Acarologische aanteekeningen. LX. Entomologische berichten 4(91):307–316. DOI: 10.5962/bhl.part.1130.
- Paraschiv M, Isaia G. 2020. Disparity of phoresy in mesostigmatid mites upon their specific carrier Ips typographus (Coleoptera: Scolytinae). Insects 11(11):771. DOI: 10.3390/insects11110771.
- Paraschiv M, Martínez‑Ruiz C, Fernández MM. 2018. Dynamic associations between Ips sexdentatus (Coleoptera: Scolytinae) and its phoretic mites in a Pinus pinaster forest in northwest Spain. Experimental & Applied Acarology 75(3):369–381. DOI: 10.1007/s10493-018-0272-9.
- Penttinen R, Viiri H, Moser JC. 2013. The mites (Acari) associated with bark beetles in the Koli National Park in Finland. Acarologia 53(1):3–15. DOI: 10.1051/acarologia/20132074.
- Pereira MCSDA, Kontschán J, Bernardi LFDO, Hermes MG. 2016. Description of a new species of Oplitis Berlese, 1884, and the first record of a phoretic uropodid mite (Acari: Oplitidae) on a Neotropical solitary wasp (Hymenoptera: Vespidae). Zootaxa 4067(3):394–400. DOI: 10.11646/zootaxa.4067.3.8.
- Pérez-Lachaud G, Klompen H, Poteaux C, Santamaría C, Armbrecht I, Beugnon G, Lachaud J-P. 2019. Context dependent life-history shift in Macrodinychus sellnicki mites attacking a native ant host in Colombia. Scientific Reports 9(1):1–10. DOI: 10.1038/s41598-019-44791-2.
- Pernek M, Hrasovec B, Matosevic D, Pilas I, Kirisits T, Moser JC. 2008. Phoretic mites of three bark beetles (Pityokteines spp.) on silver fir. Journal of Pest Science 81(1):35–42. DOI: 10.1007/s10340-007-0182-9.
- Pernek M, Wirth S, Blomquist SR, Avtzis DN, Moser JC. 2012. New associations of phoretic mites on Pityokteines curvidens (Coleoptera, Curculionidae, Scolytinae). Central European Journal Biology 7(1):63–68. DOI: 10.2478/s11535-011-0096-7.
- Perotti MA, Braig HR. 2009. Phoretic mites associated with animal and human decomposition. Experimental and Applied Acarology 49(1–2):85–124. DOI: 10.1007/s10493-009-9280-0.
- Pfammatter JA, Raffa KF. 2015. Do phoretic mites influence the reproductive success of Ips grandicollis (Coleoptera: Curculionidae)? Environmental Entomology 44(6):1498–1511. DOI: 10.1093/ee/nvv125.
- Pfammatter JA, Moser JC, Raffa KF. 2013. Mites phoretic on Ips pini (Coleoptera: Curculionidae: Scolytinae) in Wisconsin red pine stands. Annals of the Entomological Society of America 106(2):204–213. DOI: 10.1603/AN12122.
- Pfammatter JA, Coyle DR, Gandhi KJK, Hernandez N, Hofstetter RW, Moser JC, Raffa KF. 2016a. Structure of phoretic mites assemblages across subcortical beetle species at a regional scale. Environmental Entomology 45(1):53–65. DOI: 10.1093/ee/nvv150.
- Pfammatter JA, Malas KM, Raffa KF. 2016b. Behaviours of phoretic mites (Acari) associated with Ips pini and Ips grandicollis (Coleoptera: Curculionidae) during host-tree colonization. Agricultural and Forest Entomology 18(2):108–118. DOI: 10.1111/afe.12143.
- Philips JR. 2009. The mite (Acarina) fauna of trogid beetles (Coleoptera: Trogidae). International Journal of Acarology 35(1):1–17. DOI: 10.1080/01647950802709843.
- Phillipsen WJ, Coppel HC. 1978. Uroobovella formosana sp. n. associated with the Formosan subterranean termite, Coptotermes formosanus Shiraki (Acarina: Uropodidae—Isoptera: Rhinotermitidae). Journal of the Kansas Entomological Society 51(1):22–27.
- Poinar GO, Ćurčić BPM, Cokendolpher JC. 1998. Arthropod phoresy involving pseudoscorpion in the past and present. Acta Arachnologica 47(2):79–96. DOI: 10.2476/asjaa.47.79.
- Porcelli F, Ragusa E, D’Onghia AM, Mizzi S, Mifsud D. 2009. Occurrence of Centrouropoda almerodai and Uroobovella marginata (Acari: Uropodina) phoretic on the Red Palm Weevil in Malta. Bulletin of the Entomological Society of Malta 2:61–66.
- Pugh PJA, Llewellyn PJ, Robinson K, Shackley SE. 1997. The associations of phoretic mites (Acarina: Chelicerata) with sand-hoppers (Amphipoda: Crustacea) on the South Wales coast. Journal of Zoology 243(2):305–318. DOI: 10.1111/j.1469-7998.1997.tb02784.x.
- Ragusa E, Porcelli F, Di Palma A, Ragusa Di Chiara S. 2008. Una specie di acaro associata al Punteruolo rosso delle palme: Centrouropoda almerodai (Uroactiininae, Uropodina). Regione Siciliana—Assessorato Agricoltura e Foreste Dipartimento Interventi Infrastrutturali—Servizi allo Sviluppo, editor La ricerca scientifica sul Punteruolo rosso e gli altri fitofagi delle palme in Sicili 1:79–82.
- Ramsey GW. 1967. Phoretic mites associated with insects. New Zealand Entomologist 3(5):6–7. DOI: 10.1080/00779962.1966.9722853.
- Raut SK, Panigrahi A. 1991. The mite Fuscuropoda marginata (C. L. Koch) for the control of pest slugs Laevicaulis alte (Ferussac). In: Dusbábek F, Bukva V, editors. Modern acarology. Vol. 2. The Hague: Academia, Prague and SPB Academic Publishing bv. pp. 683–687.
- Rigby MC. 1996. Association of a juvenile phoretic uropodid mite with the beach hopper Traskorchestia traskiana (Stimpson, 1857) (Crustacea: Talitridae). Journal of Natural History 30(11):1617–1624. DOI: 10.1080/00222939600770941.
- Rodrigueiro TSC, Do Prado AP. 2004. Macrocheles muscaedomesticae (Acari, Macrochelidae) and a species of Uroseius (Acari, Polyaspididae) phoretic on Musca domestica (Diptera, Muscidae): effects on dispersal and colonization of poultry manure. Iheringia Série Zoologia 94(2):181–185. DOI: 10.1590/S0073-47212004000200011.
- Rubink WL, Delfinado-Baker M, Wilson WT, de Lourdes Gonzales-Gracia M, Gonzales-Cortés S. 1991. A phoretic uropodid mite associated with honey bee swarms of Northeastern Mexico. International Journal of Acarology 17(4):259–263. DOI: 10.1080/01647959108683916.
- Schwan TG, Corwin D. 1987. Uropodid mites phoretic on fleas of ground squirrels in California. Proceedings of the Entomological Society of Washington. 89:790–793.
- Schwarz HH, Starrach M, Koulianos S. 1998. Host specificity and permanence of associations between mesostigmatic mites (Acari: Anactinotrichida) and burying beetles (Coleoptera: Silphidae: Nicrophorus). Journal of Natural History 32(2):159–172. DOI: 10.1080/00222939800770101.
- Seeman OD, Walter DE. 2023. Phoresy and mites: More than just a free ride. Annual Review of Entomology 68:69–88. DOI: 10.1146/annurev-ento-120220-013329.
- Sellnick M. 1926. Alguns novos Acaros (Uropodidae) myrmecophilos e termitophilos. Archivos do Museu Nacional Rio de Janeiro 26:29–56.
- Sellnick M. 1958. Eine neue Milbe aus Fliedermauskot (sic) in einer Höhle Südafrikas (Acarina-Uropodina). Journal of the Entomological Society of Southern Africa 21(2):274–285.
- Slimane-Kharrat S, Ouali O. 2019. Mites associated with the red palm weevil (Rhynchophorus ferrugineus) in Tunisia. Tunisian Journal of Plant Protection 14(2):29–38.
- Springett BP. 1968. Aspects of the relationship between burying beetles, Necrophorus spp. and the mite Poecilochirus necrophori Vitz. Journal of Animal Ecology 37(2):417–424. DOI: 10.2307/2957.
- Stewart TB, Davis R. 1967. Notes on mites associated with coprophagous beetles. Journal of the Georgia Entomological Society 2:21–26.
- Trouessart E, Berlese A. 1919. Generi nuovi di Acari. Redia 14:4.
- Vissa S, Hofstetter RW, Bonifácio L, Khaustov A, Knee W, Uhey DA. 2019. Phoretic mite communities associated with bark beetles in the maritime and stone pine forests of Setúbal, Portugal. Experimental and Applied Acarology 77(2):117–131. DOI: 10.1007/s10493-019-00348-6.
- Vissa S, Soderberg DN, Hofstetter RW. 2021. Field translocation of mountain pine beetles suggests phoretic mite communities are locally adapted, and mite populations respond variably to climate warming. Insects 12(2):131. DOI: 10.3390/insects12020131.
- Vitzthum H. 1921. Acarologische Beobachtungen. 4. Reihe Archiv für Naturgeschichte 86(A, 10):1–69.
- Vitzthum H. 1923. Acarologische Beobachtungen. 7 Reihe. Kommensalen der Ipiden. Archiv für Naturgeschichte 89(A2, 2):97–181.
- Vitzthum H. 1926. Acari als Commensalen von Ipiden (Der Acarologischen Beobachtungen 11 Reihe). Zoologische Jahrbücher Abteilung für Systematik, Geographie und Biologie der Tiere 52:407–503.
- Vrabec M, Kalúz S, Ferenčík J. 2012. Foretické roztoče na lykožrútovi smrekovom (Ips typographus) na vybraných lokalitách vo Vysokých Tatrách. Entomofauna carpathica 24(1):1–14.
- Wiśniewski J. 1979a. Für die Fauna Polens neue Uropodina (Acari: Parasitiformes). Fragmenta Faunistica 25(5):73–76. DOI: 10.3161/00159301FF1979.25.5.073.
- Wiśniewski J. 1979b. Gangsystematik der Parasitiformes. Teil 339. Zur Kenntnis der Uropodiden-Fauna Polens. Acarologie Schiftenreihe für vergleichende Milbenkunde 26:68–74.
- Wiśniewski J. 1982. Für die Fauna Polens neue Uropodina (Acari: Parasitiformes). Teil. II. Fragmenta Faunistica 27(10):143–147. DOI: 10.3161/00159301FF1982.27.10.143.
- Wiśniewski J, Hirschmann W. 1991. Ergänzungsangaben über Verbreitung und Lebensräume der Trichouropoda-Arten (Acarina, Uropodina). Fragmenta Faunistica 35(11):173–177. DOI: 10.3161/00159301FF1991.35.11.173.
- Wiśniewski J, Hirschmann W. 1992. Neue Uropodina-Arten [Acarina] aus dem Staatlichen Museum fur Naturkunde in Lwow [Ukraine]. Bulletin of the Polish Academy of Sciences. Biological Sciences 41(1):43–65.
- Wiśniewski J, Hirschmann W. 1993a. Gangsystematik der Parasitiformes. Teil 548. Katalog der Ganggattungen, Untergattungen, Gruppen und Arten der Uropodiden der Erde (Taxonomie, Literatur, Grösse, Verbreitung, Vorkommen). Acarologie Schriftenreihe für Vergleichende Milbenkunde 40:1–220.
- Wiśniewski J, Hirschmann W. 1993b. Stadien von drei neuen Uroseius (Apionoseius)-Arten (Acarina, Uropodina) auf Trox-Arten (Coleoptera, Tenebrionidae) aus USA und Brasilien. Bulletin of the Polish Academy of Sciences Biological Sciences 41(1):85–97.
- Wiśniewski J, Michalski J. 1984. Gangsystematik der Parasitiformes. Teil 456. Stadien von 3 neuen Trichouropoda-Arten aus Sibirien, nebst einigen zoogeographischen Angaben über die Uropodiden-Fauna der Sowjetunion (Trichouropodini, Uropodinae). Acarologie Schiftenreihe für vergleichende Milbenkunde 31:75–80.
- Wiśniewski J, Hiramatsu N, Hirschmann W. 1992a. Gangsystematik der Parasitiformes. Teil 539. Gang und Stadien von 9 neuen Uroobovella-Arten der ipidis-Gruppe (Dinychini, Uropodinae). Acarologie Schiftenreihe für vergleichende Milbenkunde 39:109–128.
- Wiśniewski J, Hirschmann W, Hiramatsu N. 1992b. Neue Centrouropoda-Arten (Uroactiniinae, Uropodina) aus den Philippinen, aus Brasilien und Mittelafrika. Acarologia 33(4):313–320.
- Yasui Y. 2023. Mite dilemma: molting to acquire sexual maturity or not molting to ensure durability and dispersal ability in Phorytocarpais fimetorum (Parasitiformes; Gamasida; Parasitidae). Journal of Ethology 41(2):177–184. DOI: 10.1007/s10164-023-00783-4.
- Zach P, Kršiak B, Kulfan J, Parák M, Kontschán J. 2016. Mites Trichouropoda and Uroobovella spp. (Uropodoidea) phoretic on bark beetles (Scolytinae): A comparison from a declining mountain spruce forest in Central Europe. International Journal of Acarology 42(4):212–217. DOI: 10.1080/01647954.2016.1154107.