Abstract
Purpose
Metabolic-chronotropic relationship is the only concept that assesses the entire chronotropic function during exercise, as it takes into account individual fitness. To better understand interrelationships between chronotropic incompetence (CI), dynamic hyperinflation (DH) and exercise limitation among Global initiative for chronic Obstructive Lung Disease (GOLD) stages of chronic obstructive pulmonary disease (COPD) disease severity, we evaluated cardiopulmonary responses to symptom-limited cycle exercise in stable patients.
Patients and methods
We prospectively studied 47 COPD patients classified by GOLD stage severity. Pulmonary function tests and cardiopulmonary responses to symptom-limited incremental exercise were studied. CI was defined by regression line between percent heart rate (HR) reserve and percent oxygen uptake (V’O2) reserve, ie, chronotropic-metabolic index (CMI). DH was defined from the knot resulting from the nonlinear regressions of inspiratory capacity changes from rest to peak (dynamic inspiratory capacity (ICdyn)) with percentage of maximal HR and CMI.
Results
Aerobic capacity (median interquartile ranges) peak V’O2, 24.3 (23.6; 25.2), 18.5 (15.5; 21.8), 17.5 (15.4; 19.1) mL·kg−1·min−1 and CMI worsened according to GOLD severity. The optimal knot of ICdyn was equal to −0.34 L. The multivariate logistic regression showed a strong relationship between CI (outcome) and DH (odds ratio [confidence interval 95]) 25 (3.5; 191.6).
Conclusion
COPD patients with DH have a poor cardiovascular response to exercise, which may be attributed to CI.
Introduction
Patients with chronic obstructive pulmonary disease (COPD) have limited exercise tolerance, which has been related to reduced maximal voluntary ventilation (MMV), impaired diffusion capacity of the lung and lung hyperinflation.Citation1–Citation3 The major consequence of lung hyperinflation is the association of increased ventilatory workload and decreased inspiratory muscle pressure generating capacity, which contributes to dyspnea and poor exercise tolerance.Citation2,Citation4 Beyond the lungs, deleterious cardiovascular consequences of dynamic hyperinflation (DH) have been consistently identified in COPD patients during incremental exercise.Citation5–Citation7 In these observations, DH was associated with reduced oxygen (O2) uptake efficiency slope and O2 pulse, which noninvasively reflect cardiac stroke volume during submaximal exercise.Citation6,Citation8,Citation9 Interestingly, lung volume reduction surgery has been shown to improve exercise tolerance through cardiac stroke volume increases.Citation10
Along with cardiac stroke volume impairment is the concept that a contributing factor of reduced cardiac output leading to poor exercise tolerance might be the limitation to increase heart rate (HR), so called chronotropic incompetence (CI).Citation11 Chronotropic regulation involves cardiac interactions with the autonomic nervous system, which influences HR response to exercise, HR recovery and multiple components of HR variability.Citation12,Citation13 HR increases upon initiation of exercise are principally mediated by the withdrawal of cardiac parasympathetic activity, with the sympathetic contribution being manifested at a longer latency. Since vagal withdrawal with the initiation of exercise can result to an increase of 30 to 50 beats per minute (BPM) in HR, further increases are thought to be related to sympathetic activation. Recovery involves reactivation of the parasympathetic system and deactivation of sympathetic activity, causing a decline in HR. Interestingly, both impaired HR increases in proportion to the metabolic demand and HR recovery kinetics are associated with poor outcome.Citation13
An inability of HR to increase appropriately in proportion to the metabolic demands of exercise has been termed CI, which has been originally defined as an inability to reach 80% of age-predicted maximum HR (HRmax) or HR reserve utilization.Citation11 More adequately, the only concept that allows assessment of the entire chronotropic function is metabolic-chronotropic relationship, which takes into account individual fitness. Indeed, to ensure that reduced peak HR is not solely related to low exercise capacity, CI must be defined by the regression line between percent HR reserve and percent oxygen uptake (V’O2) reserve, ie, chronotropic-metabolic index (CMI).Citation11,Citation14 Using this approach, reduced CMI has clearly identified subgroups of patients largely dependent upon an increased HR for an increase in cardiac output during incremental exercise (HR becoming the limiting factor).Citation11 Furthermore, in heart failure patients, CI has been consistently associated with increased mortality, independently of confounding factors, such as age, gender, physical fitness, and traditional cardiovascular risk factors.Citation15,Citation16
Mechanisms of CI are thought to be secondary to chronic overactivation of the sympathetic system and subsequent downregulation of cardiac β-adrenoreceptor densities.Citation17,Citation18 Consistent studies have shown that acute lung hyperinflation in breath-hold maneuvers can induce pulmonary vessels and heart compression leading to sympathetic activity increase.Citation19,Citation20 It is thus likely that chronic hyperinflation in COPD patients would lead to chronic sympathetic overactivation and blunted cardiac chronotropic response.Citation21,Citation22 Recent findings supported that CI is common in patients with severe COPD and may be improved after lung volume reduction surgery.Citation23,Citation24 CI was also found as an independent and powerful outcome predictor in severe COPD patients.Citation25 Of note, whether CI as evaluated by CMI was related to lung hyperinflation has not been reported previously. Our aim was thus to test whether CI could be related to lung DH in exercising patients. We also aimed to determine the different factors, such as breathing pattern, DH and CI, which can influence exercise intolerance in COPD patients.
Patients and methods
Patients
This study was conducted in accordance with the amended Declaration of Helsinki (http://www.wma.net/en/30publications/10policies/b3/). The Institutional Review Board of Lille University Hospital (Lille, France) approved this study (2014-0111-b), which only included patients referred to our department for routine functional evaluation. Written informed consent was obtained from all patients. We prospectively enrolled all consecutive patients suffering from COPD, defined according to Global Initiative for Chronic Obstructive Lung Disease (GOLD) criteria.Citation26 The diagnosis of COPD was confirmed by forced spirometry, when the patient was considered clinically stable. All the procedures and their risks were explained to the patients, who gave their verbal informed consent to enter the study. Pulmonary function testing as well as exercise studies had been undertaken for clinical reasons at the request of the patients’ clinicians.
Inclusion and exclusion criteria
Caucasian subjects, either current or former smokers, with spirometrically confirmed COPD in GOLD stages were included. They had to have been free of COPD exacerbations during the last 3 months prior to inclusion. Patients with a history of left ventricular disease, left ventricular ejection fraction <45%, severe pulmonary hypertension (mean pulmonary artery pressure (PAP) >35 mmHg) were excluded. Patients treated for hypertension with blood pressure >160/90 mmHg, with arrhythmias, with significant coronary artery disease or using β-blockers were also excluded. None of the subjects had noninvasive positive-pressure ventilation support or long-term ambulatory O2 treatment.
Pulmonary function
Standard forced expiratory spirometry (forced expiratory volume in the first second (FEV1) and forced expiratory vital capacity (FVC)) and body plethysmography (residual volume (RV), functional residual capacity (FRC), total lung capacity (TLC)), and diffusing capacity of the lung for carbon monoxide (DL,CO) were performed (MasterScreen™ body plethysmograph, Viasys, France) using European and American Thoracic Society guidelines.Citation27
Cardiopulmonary testing
Cardiopulmonary exercise testing was performed according to standardized procedures using an electromagnetic braked cycle ergometer.Citation28 Exercise protocol involved an initial 2 minutes of rest, followed by 2 minutes of unloaded cycling with a progressive increment every minutes (10 W·m−1) until exhaustion at a pedaling frequency of 60–65 rpm. Subjects were continuously monitored by 12-lead electrocardiogram (Cardiosoft, CareFusion, France). Blood pressure assessed by sphygmomanometry was recorded every 2 minutes. Arterial blood (240 μL) was sampled at rest and at peak exercise and immediately analyzed using a blood gas analyzer/co-oximeter (ABL700, Radiometer, France).
Subjects respired through an oro-nasal mask (Hans Rudolf 7450 SeriesV2™ Mask, CareFusion, France). Breath-by-breath cardiopulmonary data (Vyntus, CareFusion, France) were measured at rest, warm up and incremental exercise testing. Before each test, O2 and carbon dioxide (CO2) analyzers and flow mass sensor were calibrated using available precision gas mixture and a 3-L syringe, respectively. Minute ventilation (V’E), V’O2, carbon dioxide output (V’CO2) were recorded as concurrent 10 seconds moving averages, as was determined ventilation anaerobic threshold (AT) by the V-slope method. Ventilatory reserve was calculated as (MVV − peak V’E)/MVV * 100 where MVV is maximal voluntary ventilation estimated as FEV1 multiplied by 35. Peak values were averaged over the last 30 seconds of exercise. Patient effort was considered to be maximal if two of the following occurred: predicted maximal work is achieved, predicted maximal HR is achieved, V’E/V’O2 >45, lactate level >6 mmol·L−1, RER >1.10 and pH drop >0.06, as recommended by the ATS/ACCP.Citation28 At peak exercise, subjects assessed Borg-perceived exertion ratings for both respiratory and leg discomfort. During the study period, mean values between qualified replicate tests performed weekly on control subjects were 5.1%±4.2%, 5.4%±3.2%, 6.1%±2.2%, for peak V’O2, V’CO2 and V’E, respectively.
Peak oxygen pulse (O2 pulse) was calculated and was expressed in mL per beat and as percentage of predicted value by dividing the predicted peak V’O2 by predicted peak HR. V’E/V’CO2 slope was calculated off-line as a linear regression function using 10-s averaged values and excluding the non-linear part of the relationship after the respiratory compensation point (where nonlinear rise in V’E occurred relative to V’CO2 in the presence of decrease of end-tidal pressure of CO2). The V’O2 efficiency slope (OUES) describes the relationship between V’O2 and V’E during incremental exercise, via a log transformation of V’E.Citation29 OUES was expressed as the slope of the linear relationship of log10 V’E to V’O2 (L·min−1) using V’O2 (L·min−1) = m (log10 V’E) + b, where m=OUES. Double-product (mmHg·bpm) was calculated as the product of systolic blood pressure by the HR.
DH
Changes in operational lung volumes were evaluated from measurements of inspiratory capacity (IC).Citation1–Citation3 End-expiratory lung volume (EELV) was assessed from IC maneuvers at rest, every 2 minutes during exercise and at peak exercise (Vyntus). In these maneuvers, after EELV was observed to be stable over 3–4 breaths, subjects were instructed to inspire maximally to TLC. For each measurement, EELV was calculated as resting TLC minus IC, using the plethysmographic TLC value. Dynamic IC (ICdyn) was defined as resting IC minus IC at peak exercise. DH as dichotomous variable was performed from optimal knot of ICdyn (see section “Statistical analysis”, “Results”, and ). An inflection in tidal volume (VT) relative to V’E (expressed as % peak V’E) was determined in each patient during exercise.
Figure 1 Relationship between dynamic inspiratory capacity (ICdyn) changes from rest to peak with chronotropic-metabolic index and percentage of age-predicted maximum heart rate (HRmax), (A and B), respectively.
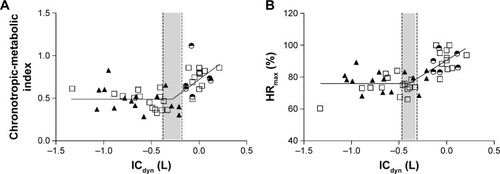
CMI
Chronotropic index was defined as an index of maximal predicted HR reserve achieved.Citation11 The percentage of HR reserve used at peak exercise referred to [(HRstage − HRrest)/(220 − age in years − HRrest)] * 100, where HR is heart rate. For any given stage of exercise, the percentage of V’O2 reserve used referred to [(V’O2 stage − V’O2 rest)/(V’O2 peak − V’O2 rest)] * 100. CMI was calculated off-line as the regression line slope between percent HR reserve and percent V’O2 reserve. A patient was referred to as having CI if the resulting slope index was <0.75 and HRmax <80%.Citation11,Citation14
Statistical analysis
The statistical analyses were performed with SAS software (SAS Institute Inc., Cary, NC, USA; version 9.3). A two-sided significance level of 0.05 was chosen for all tests. Descriptive statistics, with tests of normality (Shapiro–Wilk test) for values showed free distributions. Values were expressed as medians with interquartile ranges (IQR). Patients were divided according to GOLD classification.Citation30 Nonparametric analysis (Kruskal–Wallis test) was used to test for differences between classes. Post hoc comparisons were determined by Wilcoxon test with Bonferroni correction. Relationships between quantitative variables were assessed by the Spearman’s correlation coefficient (r). Bivariate comparisons for categorical variables were performed with Chi-squared test (with Fisher correction as appropriate).
Locally estimated scatterplot smoother SAS procedure showed a broken-line trend between CMI or HRmax and ICdyn changes. Linear mixed model procedure was used to analyze the change of CMI and HRmax versus a broken function of ICdyn that took 0 before the knot and subject’s ICdyn after the knot; the knot position was fixed by the smallest Akaike information criterion (AIC) using Gauss–Newton method.Citation31,Citation32 Thus, mean and 95% confidence interval of optimal knot, intercept (no change), and slope (increase) were estimated by nonlinear mixed model. As these two variables are used interchangeably in the literature to define CI, we took the middle of the overlap area of the ICdyn knot from these two relationships. ICdyn converted to DH as dichotomous variables indicating ICdyn < optimal knot (DH=1) or ICdyn ≥ optimal knot (DH=0). The association between CI and DH with covariables (age, body mass index (BMI), TLC, IC/TLC, RV/TLC, GOLD stage) was estimated as an odds ratio (OR) and 95% confidence intervals by using multivariate logistic regression analyses.
Results
We included 47 consecutive stable COPD patients (age 55 (49; 61) years, BMI 26 (22; 30), female n=14). All patients were maximally treated with bronchodilators. At study entry, patients were receiving inhaled steroids (75%), long-acting β2-agonists (75%) and tiotropium (65%); all of them were active or ex-smokers. Overall, a wide range of airflow obstruction (FEV1/FVC from 26% to 69%; 56 (50; 64) %), static hyperinflation (IC/TLC from 24% to 61% predicted; 40 (36; 46) % predicted), diffusing capacity (DL,CO from 17% to 98% predicted; 73 (49; 85) % predicted) was found. Nine out of 42 patients (21%) suffered from well-controlled arterial hypertension and were on diuretics (72%), ACE-inhibitors (52%), calcium-antagonists (36%) to control the disease.
According to objective ATS/ACCP criteria, exercise was considered maximal in all patients.Citation28 Mean peak workload and V’O2 was 90 (65; 95) watts (range 55–170 watts) and 18.5 (15.6; 22.6) mL·kg−1·min−1 (67 (61; 78) % predicted), respectively. AT V’O2 was 12.1 (11.0; 14.4) mL·kg−1·min−1. Peak VT to FEV1 ratio was 37 (30; 48) % and plateau of VT increase occurred at 70.8 (61.1; 77.8) % of maximum V’E. Breathing reserve at peak exercise was reduced (24 (3; 33) %). ICdyn change measured from rest to peak exercise was −0.39 (−0.67; −0.07) L and peak EELV (% TLC) was 64 (56; 72) %. HRmax was 134 (119; 150) bpm (80 (73; 88) % predicted) with HR reserve utilization of 60 (43; 76) %. Peak O2 pulse was 10.2 (8.6; 12.4) mL·bpm−1 (84 (71; 97) % predicted). Peak systemic arterial partial pressure of O2 (Pa,O2) and CO2 (Pa,CO2) were 91 (70; 99) and 39 (35; 41) mmHg, respectively. Arterial lactate was 5.6 (4.7; 7.0) mmol·L−1 at peak exercise.
Functional characteristics of patients classified by GOLD scaling system are shown in . Static hyperinflation significantly decreased incrementally from stage I to stage III–IV among baseline pulmonary function parameters (). – (main features of CPET, ventilatory and cardiovascular response details, respectively) display all results and significant differences of medians of exercise parameters between each group (GOLD stage I, stage II and stage III–IV) after adjustments. Peak V’O2 (% of predicted values) was not significantly different between GOLD stages (). At peak exercise (), EELV (% TLC), VD/VT(%) and Pa,CO2 significantly increased incrementally with patient’s COPD severity. ICdyn significantly decreased incrementally with patient’s COPD severity ().
Table 1 Subject characteristics
Table 2 Cardiopulmonary exercise testing (peak values, except AT)
Table 3 Ventilatory response during submaximal exercise (peak values, except VT/V’E inflexion point)
Table 4 Cardiovascular response during submaximal exercise (peak values, except recovery)
The nonlinear procedure established between ICdyn change and CMI () or HRmax () has converged to broken-line models. The best fit, which was defined by the smallest AIC, allowed us to obtain the values of the following knots ICdyn (mean ± standard error): −0.28±0.1 L and −0.39±0.08 L, respectively. The middle of the overlap area allowed us to determine the DH threshold with ICdyn change equal to −0.34 L.
The prevalence of DH was higher in GOLD III–IV patients than in GOLD I–II patients (). Patients with lung hyperinflation had more CI than patients without DH (). CI status was not related to GOLD score (P=0.1).
Table 5 Relationships between dynamic hyperinflation, GOLD stage and chronotropic incompetence
The logistic regression that analyzed the association between CI (outcome) and DH showed a strong relationship (OR [confidence interval 95%]) 20 [4.4–91.9] that was confirmed by multivariate analysis taking into account the following covariates: age, BMI, TLC, CI/TLC, RV/TLC and GOLD stage ().
Table 6 Multivariate analysis of chronotropic incompetence
Discussion
A new finding of this study is that lung hyperinflation in COPD patients is associated with impaired metabolic-chronotropic relationship, so-called CI, which may present as a limiting factor of exercise tolerance.
In our study, both static and dynamic lung volume measurements were performed to assess lung hyperinflation in COPD patients. Among baseline pulmonary function parameters, we used IC/TLC ratio as a static hyperinflation-derived variable reflecting mechanical inspiratory constraint.Citation1–Citation3 We found that over a wide range of airflow, IC/TLC decreased incrementally with FEV1/FVC obstruction. Such relationship was also observed with RV/TLC, which increased incrementally with FEV1/FVC. Next, we established exercise-induced DH by measuring ICdyn.Citation1–Citation3 ICdyn significantly decreased incrementally with patient’s GOLD score severity. DH was associated with increased VT constraint as VT/V’E inflection point expressed as peak V’E percent was decreased incrementally with patient’s COPD severity. DH was accompanied with poor cardiovascular response, which was evaluated in our study by noninvasive surrogate markers, such as OUES and O2 pulse.Citation5–Citation7 O2 pulse, a noninvasive measure of stroke volume, requires assumption that the arteriovenous O2 content difference remains constant during exercise. Physiologically, arterial O2 content can be altered by hemoglobin availability, arterial blood oxygenation and peripheral O2 extraction.Citation33 In our series of COPD patients, hemoglobin concentration and calculated arterial content were within the normal ranges. Furthermore, whether O2 extraction was normal or reduced in our patients, these changes may not account for O2 pulse reduction, since a lower O2 extraction would actually increase O2 pulse.Citation6,Citation10
Beside the negative effects of abnormal ventilatory mechanics on cardiac stroke volume, CI can also prevent the cardiovascular system from reaching its physiological limits before maximal exercise.Citation11 In healthy humans, HR increases virtually instantaneously upon the initiation of exercise. Involvement of a reduction in cardiac parasympathetic activity has been demonstrated in human studies showing that atropine can significantly attenuate the initial increase in HR in a variety of exercise conditions. In contrast, the magnitude of HR increase at the onset of exercise is not diminished by prior β-adrenergic blockade, implying a minimal influence of sympathetic activity at this time.Citation34,Citation35 Thereafter, there is an approximately linear relationship between HR and V’O2 during incremental dynamic exercise. At moderate-to-elevated exercise intensities β-adrenergic blockade significantly attenuates the size of the HR response, thus suggesting a key role for heightened sympathetic nervous system and elevated catecholamine levels in the circulation.Citation34–Citation36 At immediate cessation of exercise, HR abruptly declines, which is typically followed by a more gradual decline of HR over minutes depending on exercise conditions. Initial HR recovery is related to rapid restoration of cardiac parasympathetic activity, since it is virtually abolished by atropine administration, but unaffected by β-adrenergic blockade.Citation37
Blunted HR response is the primary cause of or a significant contributor to severe, symptomatic exercise intolerance in heart failure patients.Citation15,Citation16 Mechanisms of CI have been related to overstimulation of the sympathetic system, which induces downregulation of β-receptors and their desensitization in the presence of increased circulating catecholamine levels.Citation17,Citation18 In COPD patients, a striking elevation of sympathetic tone has been demonstrated and might thus provide a link between COPD and CI. Hypoxemia, chemoreflexes, impaired baroreflex sensitivity, muscle ergoreflex and lung hyperinflation are important factors contributing to neurohumoral activation in COPD.Citation20,Citation38 In normal subjects, sympathetic nerve firings are synchronized with central inspiratory motor activity and increase in lung volume during inspiration activates pulmonary vagal afferents that in turn inhibit sympathetic nerve discharge.Citation20,Citation38 The relative magnitude of these two opposing mechanisms determines the net effect of respiratory modulation of sympathetic nerve activity, which is in favor of sympathetic activation in COPD patients.Citation21,Citation22
Although lung hyperinflation may induce sympathetic overactivation in COPD patients, whether DH would lead to CI during submaximal exercise has not been previously tested. In normal subjects, CI has been most commonly diagnosed when HR fails to reach 80%–85% of the HRmax.Citation11 However, before one concludes that a patient has CI, it is important to consider the patient’s level of effort.Citation14 Whether attenuated HR in response to incremental exercise is related to low effort level or CI represents a major issue in this context. Hence, in order to more objectively evaluate CI, the relationship between HR and V’O2 during exercise should be measured.Citation11,Citation14 In this approach, the metabolic-chronotropic relationship is calculated from the ratio of the HR reserve to the metabolic reserve during submaximal exercise.Citation39,Citation40 If the assumption on which percentage of HR reserve equal V’O2 reserve is achieved in normal adults, a relationship slope less than 0.75–0.8 is considered indicative of CI in chronic heart disease patients.Citation39,Citation40 In our study, this metabolic-chronotropic relationship approach allowed us to define an association between CI and DH, which was further characterized by broken-line trend analysis with a reduced ICdyn of 0.34 L as a threshold level for CI.
In conclusion, we have shown that CI evaluated by the metabolic-chronotropic relationship is highly prevalent in a cohort of COPD patients. Adding evaluation of CI to standard pulmonary function parameters at rest and during incremental exercise allows to determine the level of lung hyperinflation as a potential mechanism of attenuated HR response.
Author contributions
All authors contributed toward data analysis, drafting and revising the paper and agree to be accountable for all aspects of the work.
Abbreviations
ACE inhibitor | = | angiotensin-converting-enzyme inhibitor |
AIC | = | Akaike information criterion |
AT | = | anaerobic threshold |
ATS/ACCP | = | American Thoracic Society/American College of Chest Physicians |
BMI | = | body mass index |
CI | = | chronotropic incompetence |
CMI | = | chronotropic-metabolic index |
COPD | = | chronic obstructive pulmonary disease |
CPET | = | cardiopulmonary exercise testing |
DH | = | dynamic hyperinflation |
DL,CO | = | diffusing capacity of the lung for carbon monoxide |
EELV | = | end-expiratory lung volume |
FEV1 | = | forced expiratory volume in the first second |
FRC | = | functional residual capacity |
FVC | = | forced expiratory vital capacity |
GOLD | = | Global Initiative for Chronic Obstructive Lung Disease |
HR | = | heart rate |
HRmax | = | age predicted maximum heart rate |
IC | = | inspiratory capacity |
ICdyn | = | dynamic inspiratory capacity |
IQR | = | interquartile ranges |
MVV | = | maximal voluntary ventilation |
O2 pulse | = | peak oxygen pulse |
OUES | = | oxygen uptake efficiency slope |
Pa | = | arterial partial pressure |
PAP | = | pulmonary artery pressure |
RER | = | respiratory exchange ratio |
RV | = | residual volume |
TLC | = | total lung capacity |
V’CO2 | = | carbon dioxide output |
V’E | = | minute ventilation |
V’O2 | = | oxygen uptake |
VD | = | death volume |
VT | = | tidal volume |
Acknowledgments
The authors thank all of the staff of the Pulmonary Functional Tests Department for their help in this work.
Disclosure
The authors report no conflicts of interest in this work.
References
- GagnonPGuenetteJALangerDPathogenesis of hyperinflation in chronic obstructive pulmonary diseaseInt J Chron Obstruct Pulmon Dis2014918720124600216
- GuenetteJAWebbKAO’DonnellDEDoes dynamic hyperinflation contribute to dyspnoea during exercise in patients with COPD?Eur Respir J201240232232922183485
- LangerDCiavagliaCENederJAWebbKAO’DonnellDELung hyperinflation in chronic obstructive pulmonary disease: mechanisms, clinical implications and treatmentExpert Rev Respir Med20148673174925159007
- VogiatzisIZakynthinosSFactors limiting exercise tolerance in chronic lung diseasesCompr Physiol2012231779181723723024
- ChuangMLHuangSFSuCHCardiovascular and respiratory dysfunction in chronic obstructive pulmonary disease complicated by impaired peripheral oxygenationInt J Chron Obstruct Pulmon Dis20151032933725709427
- TzaniPAielloMEliaDDynamic hyperinflation is associated with a poor cardiovascular response to exercise in COPD patientsRespir Res20111215022074289
- ViscaDAielloMChettaACardiovascular function in pulmonary emphysemaBiomed Res Int2013201318467824369007
- TeopompiETzaniPAielloMFat-free mass depletion is associated with poor exercise capacity irrespective of dynamic hyperinflation in COPD patientsRespir Care201459571872524170915
- VassauxCTorre-BouscouletLZeineldineSEffects of hyperinflation on the oxygen pulse as a marker of cardiac performance in COPDEur Respir J20083251275128218550609
- LammiMRCiccolellaDMarchettiNKohlerMCrinerGJIncreased oxygen pulse after lung volume reduction surgery is associated with reduced dynamic hyperinflationEur Respir J201240483784322267766
- BrubakerPHKitzmanDWChronotropic incompetence: causes, consequences, and managementCirculation201112391010102021382903
- FisherJPYoungCNFadelPJAutonomic adjustments to exercise in humansCompr Physiol20155247551225880502
- CooteJHRecovery of heart rate following intense dynamic exerciseExp Physiol201095343144019837772
- WilkoffBLMillerREExercise testing for chronotropic assessmentCardiol Clin19921047057171423382
- BenesJKotrcMBorlaugBAResting heart rate and heart rate reserve in advanced heart failure have distinct pathophysiologic correlates and prognostic impact: a prospective pilot studyJACC Heart Fail20131325926624621878
- WangJFangFYipGWImportance of chronotropic response and left ventricular long-axis function for exercise performance in patients with heart failure and preserved ejection fractionInt J Cardiol201620233934326426274
- BristowMRHershbergerREPortJDBeta-adrenergic pathways in nonfailing and failing human ventricular myocardiumCirculation1990822 SupplI12I252164894
- ColucciWSRibeiroJPRoccoMBImpaired chronotropic response to exercise in patients with congestive heart failure. Role of postsynaptic beta-adrenergic desensitizationCirculation19898023143232546698
- BreskovicTSteinbackCDSalmanpourAShoemakerJKDujicZRecruitment pattern of sympathetic neurons during breath-holding at different lung volumes in apnea divers and controlsAuton Neurosci20111641–2748121641877
- DempseyJASheelAWSt CroixCMMorganBJRespiratory influences on sympathetic vasomotor outflow in humansRespir Physiol Neurobiol2002130132012380012
- AndreasSAnkerSDScanlonPDSomersVKNeurohumoral activation as a link to systemic manifestations of chronic lung diseaseChest200512853618362416304321
- van GestelAJKohlerMClarenbachCFSympathetic overactivity and cardiovascular disease in patients with chronic obstructive pulmonary disease (COPD)Discov Med2012147935936823272688
- ArmstrongHFGonzalez-CostelloJJordeUPThe effect of lung volume reduction surgery on chronotropic incompetenceRespir Med2012106101389139522770683
- GuptaMBansalVChhabraSKAbnormal heart rate recovery and chronotropic incompetence on exercise in chronic obstructive pulmonary diseaseChron Respir Dis201310311712623785062
- González-CostelloJArmstrongHFJordeUPChronotropic incompetence predicts mortality in severe obstructive pulmonary diseaseRespir Physiol Neurobiol2013188211311823669496
- GuptaNPintoLMMoroganABourbeauJThe COPD assessment test: a systematic reviewEur Respir J201444487388424993906
- MillerMRHankinsonJBrusascoVStandardisation of spirometryEur Respir J200526231933816055882
- American Thoracic Society; American College of Chest PhysiciansATS/ACCP Statement on cardiopulmonary exercise testingAm J Respir Crit Care Med2003167221127712524257
- HollenbergMTagerIBOxygen uptake efficiency slope: an index of exercise performance and cardiopulmonary reserve requiring only submaximal exerciseJ Am Coll Cardiol200036119420110898434
- RabeKFHurdSAnzuetoAGlobal strategy for the diagnosis, management, and prevention of chronic obstructive pulmonary disease: GOLD executive summaryAm J Respir Crit Care Med2007176653255517507545
- RobbinsKRSaxtonAMSouthernLLEstimation of nutrient requirements using broken-line regression analysisJ Anim Sci200684SupplE155E16516582088
- NèveVEdméJLMatranREarlier decline in sniff nasal inspiratory pressure than peak expiratory flow in children with Duchenne muscular dystrophyEur Respir J20144451361136325142488
- WhippBJHiggenbothamMBCobbFCEstimating exercise stroke volume from asymptotic oxygen pulse in humansJ Appl Physiol (1985)1996816267426799018521
- RobinsonBFEpsteinSEBeiserGDBraunwaldEControl of heart rate by the autonomic nervous system. Studies in man on the interrelation between baroreceptor mechanisms and exerciseCirc Res19661924004115914852
- FagraeusLLinnarssonDAutonomic origin of heart rate fluctuations at the onset of muscular exerciseJ Appl Physiol1976405679682931893
- MacielBCGalloLJrMarin NetoJALima FilhoECMartinsLEAutonomic nervous control of the heart rate during dynamic exercise in normal manClin Sci (Lond)19867144574603757442
- ImaiKSatoHHoriMVagally mediated heart rate recovery after exercise is accelerated in athletes but blunted in patients with chronic heart failureJ Am Coll Cardiol1994246152915357930286
- JouettNPWatenpaughDEDunlapMESmithMLInteractive effects of hypoxia, hypercapnia and lung volume on sympathetic nerve activity in humansExp Physiol201510091018102926132990
- da CunhaFAFarinatti PdeTMidgleyAWMethodological and practical application issues in exercise prescription using the heart rate reserve and oxygen uptake reserve methodsJ Sci Med Sport2011141465720833587
- MezzaniACorràUGiordanoACafagnaMAdrianoEPGiannuzziPUnreliability of the %VO2 reserve versus %heart rate reserve relationship for aerobic effort relative intensity assessment in chronic heart failure patients on or off beta-blocking therapyEur J Cardiovasc Prev Rehabil2007141929817301633