Abstract
Background
Obesity is characterized by excessive body fat, insulin resistance and dyslipidemia, which increases the chances of developing chronic diseases like type 2 diabetes, cardiovascular diseases, hypertension, nonalcoholic fatty liver diseases, some types of cancers and neurodegenerative diseases. Kukoamine B (Kuk B) is a spermine alkaloid obtained from Lycium chinense, and it has been shown to possess antidiabetic, antioxidant and anti-inflammatory properties. In this study, we evaluated the therapeutic effect of Kuk B on high-fat diet/high-fructose (HFDFr)-induced insulin resistance and obesity in experimental rats.
Materials and Methods
Rats were fed with either normal rat diet or HFDFr for 10 consecutive weeks. The groups that were fed with HFDFr received Kuk B (25 and 50 mg/kg) from the beginning of the 6th week to the 10th week. After treatment, the effect of Kuk B on body weight, food, water intake, insulin, blood glucose, serum biochemical parameters, hepatic oxidative stress (malondialdehyde (MDA), superoxide dismutase (SOD), catalase (CAT), glutathione peroxidase (GSH-Px) and proinflammatory cytokine (interleukin (IL)-6, interleukin (IL)-1β and tumor necrosis factor alpha (TNF-α)) levels was determined. Histopathological analysis of the liver tissues was also performed.
Results
HFDFr-fed rats showed a significant increase in body weight, fasting blood glucose, insulin, lipid accumulation and liver function enzymes. In addition, HFDFr diet increased hepatic MDA, TNF-α, IL-1β and IL-6 and decreased hepatic SOD, CAT and GSH-Px activities. On the other hand, Kuk B significantly attenuated body weight, insulin resistance, lipid accumulation, oxidative stress and inflammation.
Conclusion
These results indicated that Kuk B showed protective effect against HFDFr-induced metabolic disorders by downregulating lipid accumulation, oxidative stress and inflammatory factors.
Introduction
The incidence of obesity has increased sporadically in the last two decades and it is gradually becoming a global epidemic that needs urgent attention. In 2014, the World Health Organization estimated that 600 million people were obese, while another 2 billion adults were considered overweight.Citation1 Obesity is a chronic metabolic disorder that bears the hallmark of excessive body fat accumulation and it is associated with the development of several chronic health conditions such as insulin resistance, type 2 diabetes, cardiovascular diseases, hypertension, nonalcoholic fatty liver diseases, some types of cancers and neurodegenerative diseases.Citation2,Citation3. Insulin resistance, a major prevalent factor in obesity, has been closely linked to the accumulation of lipids, oxidative stress and pro-inflammatory mediators including interleukin-6 and interleukin-1β, tumor necrosis factor alpha, nuclear factor kappa B, reactive oxygen species and free fatty acids. In addition, anomaly in the metabolism of lipids leading to hyperlipidemia is also a characteristic feature in obesity.Citation4–Citation6 Despite the advances in medical sciences, the majority of the treatments currently used for obesity have not achieved absolute success. Therefore, alternative therapies, especially from natural entities that can effectively combat obesity, are now gaining attention.Citation7–Citation9
Medicinal plants play an integral role in traditional medicinal system across the world, especially in nations where the availability and affordability of allopathic medicine are out of the reach of common people. The presence of an array of bioactive phyto-constituents in many of these plants has afforded them as promising valuable assets for the treatment of several diseases.Citation10 Lycium chinense is a famous traditional Chinese medicinal plant in the family Solanaceae, and it is widely consumed as a functional food as well as medicine.Citation11,Citation12 The dried root bark of L. chinense is frequently used in TCM for the treatment of metabolic diseases, notably diabetes and hypertension,Citation13,Citation14 and it has been proven to show potent antioxidant, anti-inflammatory and neuroprotective properties.Citation15,Citation16 One of the bioactive constituents isolated from L. chinense is kukoamine B (), a spermine alkaloid known for its wide range of therapeutic actions including antioxidant, anti-inflammatory and antidiabetic effects.Citation17 Although kukoamine B has many pharmacological effects associated with it, there is no report on its effect on obesity and insulin resistance. Thus, this study investigated the anti-obesity effect of kukoamine B in high-fat diet/fructose-fed obese rats.
Materials and Methods
Animals and Experimental Design
Adult male Wistar albino rats (150–180 g) were used for the study. The rats were housed under controlled conditions of temperature of 22 ± 2 °C, relative humidity of 55 ± 10% and a 12 h/12 h light–dark cycle. After 7 days of adaptation, the rats were fed with either normal rat diet with normal drinking water or high-fat diet with 15% fructose solution. Each group of rats except for the normal control group was fed with HFDFr for 5 weeks, and at the beginning of the 6th week, they were administered with kukoamine B (Kuk B) together with the HFDFr for an additional 5 weeks. The composition of the HDF was based on the previous reportCitation18 and is shown in .
Table 1 Composition of High-Fat Diet
The treatment groups were normal control group, HFDFr control group and Kuk B groups (gavage with 25 and 50 mg/kg). The dose of Kuk B used was according to previous studies.Citation17 After 10 weeks of treatment, the rats were fasted overnight and blood glucose levels were determined with the aid of a glucometer (Accu-Check Performa, Roche, Mannheim, Germany). The experimental procedure was conducted in line with the National Institute of Health guide for the care and use of laboratory animals (NIH Publication No. 80-23; revised 1978) and was reviewed and approved by the ethics committee of The First People’s Hospital of Yunnan Province (ethic number: 20181229).
Intra-Peritoneal Glucose Tolerance Test (IPGTT) and Insulin Tolerance Test (ITT)
After the 10th week of treatment, the animals were fasted for 12 h and intraperitoneally injected with 2.0 g/kg of glucose solution for the determination of IPGTT. Blood glucose levels were estimated at 0, 30, 60, 90 and 120 min after the injection with the aid of a blood glucose meter. For the ITT, the rats were fasted for 6 h and intraperitoneally injected with 0.75 U insulin/kg. Blood samples were taken at 0, 30, 60, 90 and 120 min for the determination of blood glucose level. The concentration of glucose in a time point plot was used to calculate the area under curves of IPGTT and ITT.
Sample Collection
After the experiments, the rats were fasted for 12 h and euthanized with sodium thiopental (150 mg/kg) and sacrificed by cervical dislocation. Blood was collected by cardiac puncture and centrifuged at 2500 rmp for 10 min (4 °C) to obtain the plasma. The liver, epididymal and retroperitoneal fat pads were carefully excised, washed with phosphate buffer and weighed. A part of the liver was preserved in 10% buffered formalin solution for histological analysis while 10% homogenate was prepared from the other part in phosphate buffer and centrifuged at 2500 rmp, 4°C for 10 min. The supernatant obtained was used for further biochemical assays.
Determination of Serum Biochemical Parameters
Serum total cholesterol (TC), triglycerides (TG), low-density lipoprotein cholesterol (LDL-C), high-density lipoprotein cholesterol (HDL-C), alanine transaminase (ALT), and aspartate transaminase (AST) were determined with the aid of an automatic biochemical analyzer. Serum insulin and free fatty acid (FFA) concentrations were measured by using a commercially available ELISA kit (Mlbio, Shanghai Enzyme-linked Biotechnology Co., Ltd, Shanghai, China) according to the manufacturer’s instructions. Homeostatic model assessment index (HOMA-IR) was calculated according to the following formula:Citation19
Determination of Hepatic Lipids
Hepatic lipids were extracted using the previously described protocol.Citation20 Briefly, the liver lipids were extracted by homogenizing liver tissues in chloroform/methanol solution (ratio 2:1) on an orbital shaker for 25 mins. Thereafter, the liver homogenate was centrifuged at 2500 rmp for 10 min and the supernatants obtained were dried with nitrogen gas. The extracted lipids were dissolved in 2-propanol containing 1% Triton X100 and used for the determination of TC and TG contents.
Determination of Oxidative Stress Parameters
The excised liver samples were homogenized in phosphate buffer (10% homogenate) and centrifuged at 2500 rpm for 10 min at 4 °C. The supernatant obtained from the liver homogenate was used for estimating hepatic oxidative stress marker (SOD, GSH-Px, CAT and MDA) levels using commercial assay kits (Nanjing Jiancheng Bioengineering Institute, Nanjing, China) based on the manufacturer’s directives.
Determination of Pro-Inflammatory Mediators
The serum levels of pro-inflammatory cytokines (IL-1β, TNF-α, IL-6 and NF-kB) were analyzed with the aid of commercially available ELISA kits (Mlbio, Shanghai Enzyme-linked Biotechnology Co., Ltd, Shanghai, China, and Elabscience Biotechnology, Wuhan, China).
Histopathological Analysis of Liver Tissues
The liver tissues were fixed in 10% buffered formalin solution, dehydrated with graded alcohol solution, embedded in paraffin and sliced and into 5-μm sections. The sections were further stained with hematoxylin and eosin (H&E), and histopathologic changes of the liver tissues were observed under an Olympus light microscope (Tokyo, Japan).
Statistical Analysis
The results are expressed as the mean ± standard deviation (SD). Differences among groups were analyzed by one-way ANOVA and Tukey’s multiple comparison tests using Prism 7.0 software (GraphPad Software Inc., San Diego, CA). Statistically significant was set at P < 0.05 in all cases.
Results
Effect of Kuk B on Food, Water Consumption and Organ Weight in HFDFr-Fed Rats
As shown in , compared with the normal control group there was a significant reduction in food intake and water intake of the HFDFr group (P< 0.05). On the other hand, there were no significant changes in the food and water intake between the Kuk B-treated groups and HFDFr groups. Furthermore, there was a significant increase in the weight of liver, epididymal fat and retroperitoneal fat in the HFDFr group compared with the normal control group. However, treatment with Kuk B reduced the weight of liver and fat compared with the HFDFr group ().
Table 2 Effect of Kuk B on Food Intake, Water Intake and Organ Weight in Obese Rats
Effect of Kuk B on Body Weight and Insulin Tolerance in HFDFr-Fed Rats
The effect of Kuk B on body weight is shown in . The body weight of HFDFr-fed rat group rapidly and significantly increased compared to the normal control group (P < 0.05). In Kuk B-treated groups, the results showed a significant reduction in the body weight of rats as compared with the HFDFr group (P < 0.05). The effect of Kuk B on fasting blood glucose, insulin, and insulin resistance index (HOMA-IR) is shown in –. The rats in the HFDFr group showed significantly increased fasting blood glucose () and insulin levels () when juxtaposed with the normal diet-fed rats (P < 0.05). On the other hand, insulin and fasting blood glucose levels in the Kuk B-treated groups (25 and 50 mg/kg) were significantly lowered compared to the levels in the HFDFr group. In addition, treating with Kuk B significantly improved HOMA-IR compared to HFDFr control group ()
Figure 2 Effect of Kuk B on (A) body weight, (B) fasting blood glucose, (C) insulin, (D) HOMA-IR in obese rats.
Notes: Data are expressed as the mean ± SD (n = 6). One-way ANOVA with Tukey’s post hoc test was used for statistical analysis. **P < 0.05 vs normal control, ***P < 0.05 vs HFDFr.
Abbreviations: Kuk B, kukoamine B; HOMA-IR, homeostatic model assessment of insulin resistance; SD, standard deviation; HFDFr, high-fat diet/high fructose
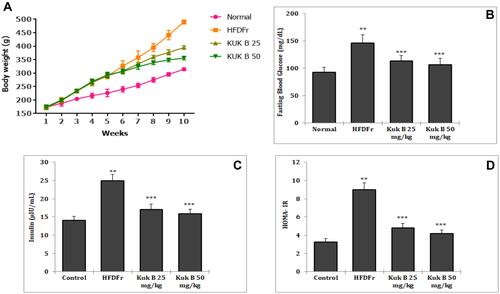
Effect of Kuk B on Glucose and Insulin Tolerance in HFDFr-Fed Rats
As indicated in , the HFDFr group showed significantly higher fasting blood glucose levels at all time points measured (0, 30, 60, 90 and 120 min) when compared to the normal control group (P < 0.05) in the IPGTT. On the other hand, the blood glucose levels of Kuk B-treated groups were significantly decreased at 30, 60, 90 and 120 min after the administration of glucose. Furthermore, the AUC in the Kuk B-treated groups was significant lower as compared to the HFDFr group (). In the ITT, the blood glucose levels in the normal control and Kuk B-treated groups (50 mg/kg) were markedly lower than the blood glucose levels in the HFDFr group (), suggesting that insulin sensitivity in the HFDFr group was reduced. Kuk B-treated group (25 mg/kg) had a lower level of blood glucose; however, the effect was not significant when compared to the HFDFr group (). Analysis of AUC summarized in indicated that the area under the curve for the ITT in the HFDFr group was significantly increased compared with the normal control group. However, treatment with Kuk B significantly reduced blood glucose levels.
Figure 3 (A) Effect of Kuk B on IPGTT. (B) Quantification of the area under the curve (AUC) from the IPGTT. (C) Effect of Kuk B on ITT. (D) Quantification of the area under the curve (AUC) from the ITT.
Notes: Data are expressed as the mean ± SD (n = 6). One-way ANOVA with Tukey’s post hoc test was used for statistical analysis. **P < 0.05 vs normal control, ***P < 0.05 vs HFDFr.
Abbreviations: Kuk B, kukoamine B; IPGTT, intraperitoneal glucose tolerance test; ITT, intraperitoneal insulin tolerance test; SD, standard deviation; HFDFr, high-fat diet/high fructose
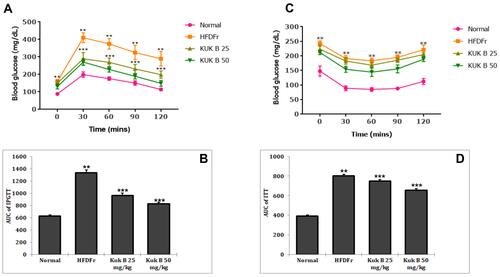
Effect of Kuk B on Biochemical Parameters in HFDFr-Fed Rats
The effect of Kuk B on serum lipid levels in HFDFr-fed rats is shown in . Marked increase in the serum levels of TG, TC (), LDL-C () and FFA () was noticed in the HFDFr group compared to the normal control group (P < 0.05). On the other hand, after 5 weeks of treatment with Kuk B, the lipid profiles were significantly improved compared to the HFDFr group (P < 0.05). HDL-C levels were significantly lowered in the HFDFr group compared with the normal control group, Kuk B insignificantly increased HDL-C level compared to the HFDFr group (). In addition, high levels of hepatic TG and TC were observed in the HFDFr group (), Kuk B remarkably decreased TG and TC levels in the liver tissues of treated rats (P < 0.05). As shown in , compared to the HFDFr group, treatment with Kuk B significantly decreased the serum levels of AST and ALT (P < 0.05). These results indicated that Kuk B attenuated anomalies of the liver function and lipid metabolism caused by HFDFr.
Figure 4 Effect of Kuk B on (A) serum TG and TC (B) serum LDL-C and HDL-C (C) hepatic TG and TC (D) serum AST and ALT (E) serum FFA in obese rats.
Notes: Data are expressed as the mean ± SD (n = 6). One-way ANOVA with Tukey’s post hoc test was used for statistical analysis. **P < 0.05 vs normal control, ***P < 0.05 vs HFDFr.
Abbreviations: Kuk B, kukoamine B; TG, triglycerides; TC, total cholesterol; LDL-C, low density lipoprotein cholesterol; HDL-C, high density lipoprotein cholesterol; AST, aspartate transaminase; ALT, alanine transaminase; FFA, free fatty acid; SD, standard deviation; HFDFr, high-fat diet/high fructose.
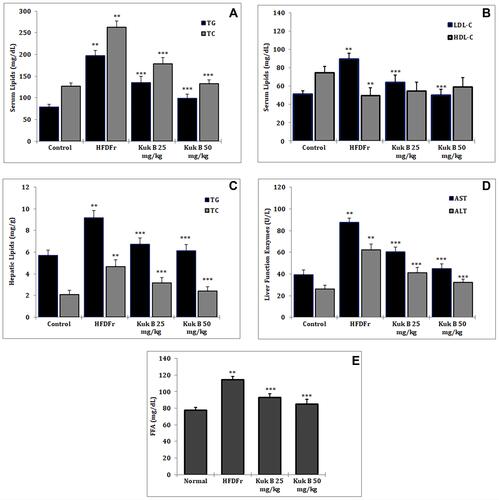
Effect of Kuk B on Inflammatory Cytokines in HFDFr-Fed Rats
Serum inflammatory cytokines (TNF-α, IL-1β, IL-6 and NF-kB) concentrations were elevated in HFDFr rats when compared to normal control. However, these parameters were significantly reduced in the Kuk B-treated group (P < 0.05) compared to the HFDFr group (–).
Figure 5 Effect of Kuk B on (A) TNF-α, (B) IL-6, (C) IL1-β, (D) NF-kB in obese rats in obese rats.
Notes: Data are expressed as the mean ± SD (n = 6). One-way ANOVA with Tukey’s post hoc test was used for statistical analysis. **P < 0.05 vs normal control, ***P < 0.05 vs HFDFr.
Abbreviations: Kuk B, kukoamine B; TNF-α,tumor necrosis factor alpha; IL-6, interleukin 6; IL1-β, interleukin 1 beta; NF-kB, nuclear factor kappa B; SD, standard deviation; HFDFr, high-fat diet/high fructose.
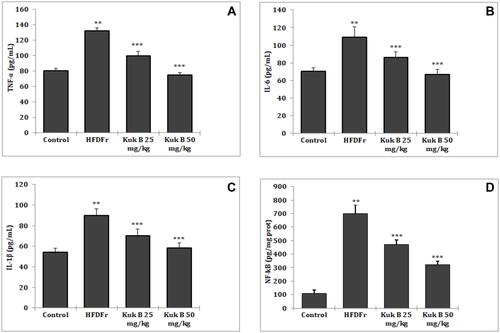
Effect of Kuk B on Oxidative Stress Markers in HFDFr-Fed Rats
We further examined the effect of Kuk B on oxidative stress parameters in HFDFr-fed rats by estimating the hepatic levels of MDA, SOD, GSH-Px and CAT. As shown in , MDA level in the HFDFr-fed rats was increased by 1.6-fold compared to the rats that were fed with the normal diet. In contrast, treatment with Kuk B attenuated MDA levels in the treated rats. Furthermore, in the HFDFr-fed group, the activities of SOD, GSH-Px and CAT were reduced; however, a significant increase was observed in the activities of these enzymes after 5 weeks of treatment with Kuk B (–).
Figure 6 Effect of Kuk B on (A) MDA, (B) SOD, (C) CAT, (D) GSH-Px in obese rats.
Notes: Data are expressed as the mean ± SD (n = 6). One-way ANOVA with Tukey’s post hoc test was used for statistical analysis. **P < 0.05 vs normal control, ***P < 0.05 vs HFDFr.
Abbreviations: Kuk B, kukoamine B; MDA, malondialdehyde; SOD, superoxide dismutase; CAT, catalase; GSH-Px, glutathione peroxidase; SD,standard deviation; HFDFr, high-fat diet/high fructose.
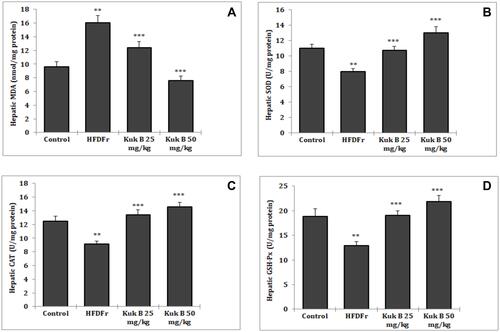
Effect of Kuk B on Pathological Lesions in HFDFr-Fed Rats
The effect of Kuk B on histopathological alterations in HFDFr-induced obesity was evaluated by histological analysis. As shown in , the normal control rats showed no abnormalities, whereas, hepatocyte lipid droplet accumulation as well as inflammatory cells were observed in the liver histology of the HFDFr group (). In the Kuk B-treated groups, there were obvious signs of alleviation of these pathological alterations (–).
Discussion
Kukoamine B (Kuk B) is a spermine alkaloid obtained from the root bark of L. chinense, and several studies have reported it as a potent antioxidant and anti-inflammatory agent, and its ability to inhibit oxidative stress–induced damages has been highlighted.Citation21–Citation24 Since oxidative stress and low-grade inflammation have been largely implicated in the pathogenesis of insulin resistance and obesity, natural substances with antioxidant and anti-inflammatory effects may be promising in the treatment of obesity and metabolic disorders. Therefore, this study investigated the effect of Kuk B on high-fat/fructose diet–induced obesity as well as its role in alleviating oxidative stress, lipid accumulation, inflammation and insulin resistance in obese rats.
The consumption of diets with high quantities of fats and fructose has been widely viewed as one of the key elements associated with obesity, insulin resistance and other metabolic disorders. Excessive lipid accumulation in the adipose tissue can result in the formation of lipid intermediates including fatty acyl-CoA, diacylglycerols and ceramides, which alters several processes in the body, especially in the muscle and liver, resulting in metabolic anomalies such as glucose intolerance and hepatic steatosis.Citation25–Citation28 The accumulation of free fatty acids initiates insulin resistance in skeletal muscle, which subsequently activates protein kinase C and inflammatory pathways such as JNK, IKK and NF-kβ.Citation29–Citation31 Furthermore, high fructose consumption has also been associated with insulin resistance due to its effect on elevating plasma insulin, FFA, fasting glucose and glucose intolerance.Citation32,Citation33
Insulin resistance is a condition that is associated with reduction in insulin sensitivity, utilization of insulin by peripheral tissues and glucose uptake, leading to excessive secretion and circulation of insulin in the blood (hyperinsulinemia). Hyperinsulinemia can result in several metabolic disorders such as type 2 diabetes mellitus, hypertension, coronary heart disease, cerebrovascular disease, obesity and dyslipidemia.Citation34,Citation35 Furthermore, insulin resistance is a requisite for the elevation of blood glucose level, serum lipids and cholesterol levels, thus creating a fertile ground for the development of cardiovascular disease and metabolic syndrome.Citation36,Citation37 The results of this study clearly demonstrated that Kuk B significantly ameliorated insulin resistance caused by HFDFr. Indeed, the administration of Kuk B reduced levels of blood glucose, insulin, serum and hepatic lipids, as well as liver function enzymes. These results suggest that Kuk B enhances insulin sensitivity, inhibits insulin resistance and alleviates other metabolic anomalies associated with insulin resistance.
The etiology of insulin resistance is multi-faceted and a full understanding of it is still lacking; however, certain parameters like oxidative stress and inflammation have been widely implicated in the etiology of insulin resistance and obesity. Numerous reports have shown the existing cordial relationship between oxidative stress, insulin resistance and obesity. The activities of cellular antioxidant enzymes are diminished and the generation of oxidative stress agents such as ROS and other oxidative modification products is increased in the serum, liver, adipose and brain tissues during insulin resistance.Citation6,Citation38-Citation40 Accumulation of excess fat, which is often the case in obesity, or HFDFr intake leads to increase in FFA oxidation, which subsequently triggers nicotinamide adenine dinucleotide (NADPH) oxidase to overgenerate ROS. In addition, previous reports have associated oxidative stress agents with high-fat accrual.Citation41–Citation43 Furthermore, it has been widely acknowledged that insulin resistance and oxidative stress can trigger inflammation and the release of cytokines by the liver and adipose tissue. Oxidative stress also has the ability to activate inflammatory response leading to insulin resistance through the upregulation of several inflammatory-related pathway signaling molecules such as serine kinases c-Jun N-terminal kinase (JNK) and I kappa B kinase (IKK-b) in the adipose tissues and liver that inhibit normal insulin signaling. In response to the instigation of chronic inflammation, several inflammatory mediators such as TNF-α, IL-6, IL-1β, COX-2 and MCP-1 are released, which can aggravate insulin resistance and further reduce the sensitivity of tissues to insulin, thus limiting glucose uptake.Citation44–Citation48 Previous reports have clearly indicated that HFDFr stimulates ROS, oxidative stress and inflammatory mediators.Citation4,Citation49 Our study indicated that the HFDFr-fed rats showed increased oxidative stress and inflammatory markers (MDA, TNF-α, IL-6, IL-1β and NF-kB) and decreased antioxidant enzyme activities (GSH-Px, SOD, and CAT). Kuk B attenuated oxidative stress and inflammation by enhancing antioxidant defense activity and reducing the release of inflammatory-related cytokine.
Numerous studies have clearly illustrated that lipid profiles are significantly altered in diet-induced obesity and metabolic abnormalities. Consumption of HFDFr enhances the accumulation of lipids in the liver and subsequently increased secretion in blood.Citation50,Citation51 High levels of TC, TG and LDL-C and reduced levels of HDL-C are vital indicators that have been linked to the incidence of diabetes mellitus, coronary heart diseases and cardiovascular diseases.Citation52 The results from this study demonstrated that the administration of Kuk B decreased serum and hepatic TC and TG as well as serum FFA and LDL-C. The Kuk B-treated groups also showed improvement in HDL-C levels. It was observed that the HFDFr group had high levels of serum AST and ALT, indicating liver damage. The release of these liver function enzymes has been linked to alterations in the plasmatic membrane of the hepatocytes. The decrease in hepatic lipid profiles and liver function enzymes in the Kuk B-treated groups correlates with the histopathology analysis of the liver, where a reduction in the lipid droplets (lipid vacuole content) was obviously seen in the Kuk B-treated groups when compared to the HFDFr group. These results are consistent with those of other studies.Citation51,Citation53
Conclusion
Kuk B protected against HFDFr-induced insulin resistance and metabolic dysfunction in rats through the regulation of dyslipidemia, antioxidant defenses and inflammatory response. Thus, L. chinense and its active components especially kukoamine B may have possible applications as dietary supplements in ameliorating metabolic disorders.
Author Contributions
All authors contributed to data analysis, drafting and revising of the article, gave final approval of the version to be published, and agree to be accountable for all aspects of the work.
Disclosure
The authors report no conflicts of interest in this work.
Additional information
Funding
References
- Mancini MC, Edna de Melo M. The burden of obesity in the current world and the new treatments available: focus on liraglutide 3.0 mg. Diabetol Metab Syndr. 2017;9:44. doi:10.1186/s13098-017-0242-0
- Berrington de Gonzalez A, Hartge P, Cerhan JR, et al. Body-mass index and mortality among 1.46 million white adults. N Engl J Med. 2010;363:2211–2219. doi:10.1056/NEJMoa1000367
- Kopelman PG. Obesity as a medical problem. Nature. 2002;404(6778):635–643. doi:10.1038/35007508
- Sah SP, Singh B, Choudhary S, Kumar Aff. Animal models of insulin resistance: a review. Pharmacol Rep. 2016;68(6):1165–1177. doi:10.1016/j.pharep.2016.07.010
- Hotamisligil GS. Endoplasmic reticulum stress and the inflammatory basis of metabolic disease. Cell. 2010;140:900–917. doi:10.1016/j.cell.2010.02.034
- Henriksen EJ, Diamond-Stanic MK, Marchionne EM. Oxidative stress and the etiology of insulin resistance and type 2 diabetes. Free Radic Biol Med. 2011;51(5):993–999. doi:10.1016/j.freeradbiomed.2010.12.005
- Kang JG, Park CY. Anti-obesity drugs: a review about their effects and safety. Diabetes Metab J. 2012;36(1):13–25. doi:10.4093/dmj.2012.36.1.13
- Rodgers RJ, Tschop MH, Wilding JPH, Rodgers RJ, Tschöp MH, Wilding JP. Anti-obesity drugs: past, present and future. Dis Model Mech. 2012;5:621–626. doi:10.1242/dmm.009621
- Sergent T, Vanderstraeten J, Winand J, Beguin P, Schneider YJ. Phenolic compounds and plant extracts as potential natural anti-obesity substances. Food Chem. 2012;135(1):68–73. doi:10.1016/j.foodchem.2012.04.074
- de Freitas Junior LM, de Almeida EB Jr. Medicinal plants for the treatment of obesity: ethnopharmacological approach and chemical and biological studies. Am J Transl Res. 2017;9:2050–2064.
- Potterat O. Goji (Lycium barbarum and L. chinense): phytochemistry, pharmacology and safety in the perspective of traditional uses and recent popularity. Planta Med. 2010;76:7–19. doi:10.1055/s-0029-1186218
- Yao X, Peng Y, Xu LJ, Li L, Wu QL, Xiao PG. Phytochemical and biological studies of Lycium medicinal plants. Chem Biodivers. 2011;8:976–1010. doi:10.1002/cbdv.201000018
- Yao R, Heinrich M, Weckerle CS. The genus Lycium as food and medicine: a botanical, ethnobotanical and historical review. J Ethnopharmacol. 2018;212:50–66. doi:10.1016/j.jep.2017.10.010
- Xie LW, Atanasov AG, Guo DA, et al. Activity-guided isolation of NF-κB inhibitors and PPARγ agonists from the root bark of Lycium chinense Miller. J Ethnopharmacol. 2014;152:470–477. doi:10.1016/j.jep.2014.01.029
- Cho SH, Park EJ, Kim EO, Choi SW. Study on the hypocholesterolemic and antioxidative effects of tyramine derivatives from the root bark of Lycium chinense Miller. Nutr Res Pract. 2011;5:412–420. doi:10.4162/nrp.2011.5.5.412
- Chen H, Olatunji OJ, Zhou Y. Anti-oxidative, anti-secretory and anti-inflammatory activities of the extract from the root bark of Lycium chinense (Cortex Lycii) against gastric ulcer in mice. J Nat Med. 2016;70:610–619. doi:10.1007/s11418-016-0984-2
- Li YY, Stewart DA, Ye XM, et al. A metabolomics approach to investigate Kukoamine B-A potent natural product with anti-diabetic properties. Front Pharmacol. 2019;9:1575. doi:10.3389/fphar.2018.01575
- Gheibi S, Bakhtiarzadeh F, Jeddi S, Farrokhfall K, Zardooz H, Ghasemi A. Nitrite increases glucose-stimulated insulin secretion and islet insulin content in obese type 2 diabetic male rats. Nitric Oxide. 2017;64:39–51. doi:10.1016/j.niox.2017.01.003
- Esteghamati A, Ashraf H, Khalilzadeh O, et al. Optimal cut-off of homeostasis model assessment of insulin resistance (HOMA-IR) for the diagnosis of metabolic syndrome: third national surveillance of risk factors of non-communicable diseases in Iran (SuRFNCD-2007). Nutr Metab (Lond). 2010;7:26. doi:10.1186/1743-7075-7-26
- Folch J, Lees M, Solane GM. A simple method for the isolation and purification of total lipids from animal tissues. J Biol Chem. 1957;226:497–509.
- Zheng X, Wang N, Yang Y, Chen Y, Liu X, Zheng J. Insight into the inhibition mechanism of kukoamine B against CpG DNA via binding and molecular docking analysis. RSC Adv. 2016;6:85756–85762.
- Yang D, Zheng X, Wang N, et al. Kukoamine B promotes TLR4-independent lipopolysaccharide uptake in murine hepatocytes. Oncotarget. 2016;7:57498–57513. doi:10.18632/oncotarget.11292
- Hu XL, Guo LP, Song Q, et al. Kukoamine B, an amide alkaloid, protects against NMDA-induced neurotoxicity and potential mechanisms in vitro. Neurochem Int. 2015;87:66–76. doi:10.1016/j.neuint.2015.06.001
- Li X, Lin J, Chen B, Xie H, Chen D. Antioxidant and cytoprotective effects of kukoamines A and B: comparison and positional isomeric effect. Molecules. 2018;23:4.
- Dandona P, Aljada A, Chaudhuri A, Mohanty P, Garg R. Metabolic syndrome: a comprehensive perspective based on interactions between obesity, diabetes, and inflammation. Circulation. 2005;111:448–1454. doi:10.1161/01.CIR.0000158483.13093.9D
- Zivkovic AM, German JB, Sanyal AJ. Comparative review of diets for the metabolic syndrome: implications for nonalcoholic fatty liver disease. Am J Clin Nutr. 2007;86(2):285–300. doi:10.1093/ajcn/86.2.285
- Perry RJ, Samuel VT, Petersen KF, Shulman GI. The role of hepatic lipids in hepatic insulin resistance and type 2 diabetes. Nature. 2014;510(7503):84–91. doi:10.1038/nature13478
- Petersen MC, Shulman GI. Roles of diacylglycerols and ceramides in hepatic insulin resistance. Trends Pharmacol Sci. 2017;38(7):649–665. doi:10.1016/j.tips.2017.04.004
- Shoelson SE, Lee J, Goldfine AB. Inflammation and insulin resistance. J Clin Invest. 2006;116(7):1793–1801. doi:10.1172/JCI29069
- Samuel VT, Shulman GI. Mechanisms for insulin resistance: common threads and missing links. Cell. 2012;148(5):852–871. doi:10.1016/j.cell.2012.02.017
- Ye J. Mechanisms of insulin resistance in obesity. Front Med. 2013;7(1):14–24. doi:10.1007/s11684-013-0262-6
- Naples M, Federico LM, Xu E, Nelken J, Adeli K. Effect of rosuvastatin on insulin sensitivity in an animal model of insulin resistance: evidence for statin-induced hepatic insulin sensitization. Atherosclerosis. 2008;198(1):94–103. doi:10.1016/j.atherosclerosis.2007.11.003
- Maithilikarpagaselvi N, Sridhar MG, Swaminathan RP, Zachariah B. Curcumin prevents inflammatory response, oxidative stress and insulin resistance in high fructose fed male Wistar rats: potential role of serine kinases. Chem Biol Interact. 2016;244:187–194. doi:10.1016/j.cbi.2015.12.012
- Reaven GM. Banting lecture role of insulin resistance in human disease. Diabetes. 1988;37(12):1595–1607. doi:10.2337/diab.37.12.1595
- Duque-Guimaraes DE, Ozanne SE. Nutritional programming of insulin resistance: causes and consequences. Trends Endocrinol Metab. 2013;24(10):525–535. doi:10.1016/j.tem.2013.05.006
- Hishinuma A, Majima M, Kurabayashi H. Insulin resistance in patients with stroke is related to visceral fat obesity and adipocytokines. J Stroke Cerebrovasc Dis. 2008;17(4):175–180. doi:10.1016/j.jstrokecerebrovasdis.2008.01.004
- Shimizu I, Yoshida Y, Minamino T. Pathological role of adipose tissue dysfunction in cardio-metabolic disorders. Int Heart J. 2015;56(3):255–259. doi:10.1536/ihj.14-401
- Sripetchwandee J, Chattipakorn N, Chattipakorn SC. Links between obesity-induced brain insulin resistance, brain mitochondrial dysfunction, and dementia. Front Endocrinol. 2018;9:496. doi:10.3389/fendo.2018.00496
- Hurrle S, Hsu WH. The etiology of oxidative stress in insulin resistance. Biomed J. 2017;40(5):257–262. doi:10.1016/j.bj.2017.06.007
- Maciejczyk M, Żebrowska E, Chabowski A. Insulin resistance and oxidative stress in the brain: what’s new? Int J Mol Sci. 2019;20(4):4. doi:10.3390/ijms20040874
- Furukawa S, Fujita T, Shimabukuro M, et al. Increased oxidative stress in obesity and its impact on metabolic syndrome. J Clin Invest. 2004;114(12):1752–1761. doi:10.1172/JCI21625
- Keaney JF Jr, Larson MG, Vasan RS, et al., Framingham Study. Obesity and systemic oxidative stress: clinical correlates of oxidative stress in the Framingham study. Arterioscler Thromb Vasc Biol. 2003;23(3):434–439. doi:10.1161/01.ATV.0000058402.34138.11
- Tangvarasittichai S. Oxidative stress, insulin resistance, dyslipidemia and type 2 diabetes mellitus. World J Diabetes. 2015;6(3):456–480. doi:10.4239/wjd.v6.i3.456
- Ropelle ER, Flores MB, Cintra DE, et al. IL-6 and IL10 anti-inflammatory activity links exercise to hypothalamic insulin and leptin sensitivity through IKKb and ER stress inhibition. PLoS Biol. 2010;8(8):1812. doi:10.1371/journal.pbio.1000465
- Davis JE, Braucher DR, Walker-Daniels J, Spurlock ME. Absence of Tlr2 protects against high-fat diet-induced inflammation and results in greater insulin-stimulated glucose transport in cultured adipocytes. J Nutr Biochem. 2011;22(2):136–141. doi:10.1016/j.jnutbio.2009.12.008
- Lee Y, Fluckey JD, Chakraborty S, Muthuchamy M. Hyperglycemia and hyperinsulinemia induced insulin resistance causes alterations in cellular bioenergetics and activation of inflammatory signaling in lymphatic muscle. FASEB J. 2017;31(7):2744–2759. doi:10.1096/fj.201600887R
- Asrih M, Jornayvaz FR. Inflammation as a potential link between nonalcoholic fatty liver disease and insulin resistance. J Endocrinol. 2013;218(3):25–36. doi:10.1530/JOE-13-0201
- Peng Y, Rideout D, Rakita S, Lee J, Murr M. Diet induced obesity associated with steatosis, oxidative stress, and inflammation in liver. Surg Obes Relat Dis. 2012;8:73–81. doi:10.1016/j.soard.2011.07.019
- Feillet-Coudray C, Fouret G, Vigor C, et al. Long-term measures of dyslipidemia, inflammation, and oxidative stress in rats fed a high-fat/high-fructose diet. Lipids. 2019;54:81–97. doi:10.1002/lipd.12128
- Basaranoglu M, Basaranoglu G, Sabuncu T, Sentürk H. Fructose as a key player in the development of fatty liver disease. World J Gastroenterol. 2013;19(8):1166–1172. doi:10.3748/wjg.v19.i8.1166
- Aguilera-Mendez A, Hernández-Equihua MG, Rueda-Rocha AC, et al. Protective effect of supplementation with biotin against high-fructose-induced metabolic syndrome in rats. Nutr Res. 2018;57:86–96. doi:10.1016/j.nutres.2018.06.007
- Bastard JP, Maachi M, Lagathu C, et al. Recent advances in the relationship between obesity, inflammation, and insulin resistance. Eur Cytokine Netw. 2006;17(1):4–12.
- Hariri N, Thibault L. High-fat diet-induced obesity in animal models. Nutr Res Rev. 2010;23(2):270–299. doi:10.1017/S0954422410000168