Abstract
Objectives
The aim of this paper was to investigate the efficacy and activity variation associated with phosphoinositide 3-kinase (PI3K) signal transduction when combining erlotinib with radiation, using different administration schedules.
Materials and methods
Erlotinib was delivered to A973 cancer cells in the following three ways: (1) irradiation after administration, (2) irradiation upon administration, and, (3) irradiation before administration. The cell-survival rates were detected using colony-forming assays, while cell apoptosis was detected with flow cytometry. The expression levels of C-MET, p-C-MET, AKT, and p-AKT were determined via Western blotting analysis, under 6 Gy irradiation with/ without erlotinib.
Results
The sensitizer enhancement ratios (SERs) of erlotinib irradiation after administration, irradiation upon administration, and irradiation before administration groups were 2.19, 1.53, and 1.38, respectively. A higher apoptosis rate was observed when irradiation was delivered after erlotinib. In addition, changes in cell apoptosis were found to be related to concurrent changes in C-MET, p-C-MET, AKT, and p-AKT expression. Protein expression increased in the combination groups, with trends showing a negative relationship with cell apoptosis.
Conclusion
The radiosensitive effect of erlotinib varied because of the different administration schedules; this variation may be related to PI3K signal transduction and its associated regulating effect.
Introduction
Epidermal growth factor receptor (EGFR) is an important target in tumor therapy,Citation1–Citation7 and EGFR tyrosine kinase inhibitors (TKIs) have shown antitumor activities in non–small cell lung cancer patients.Citation8,Citation9 When EGFR TKIs are used concurrently with radiotherapy, their potential effects may increase. However, the optimal administration schedule for combining TKIs with radiotherapy is unclear, and the mechanism of TKIs also warrants further research. This study investigated the different radiosensitization effects of TKIs and their relationship to C-MET-phosphoinositide 3-kinase (PI3K)-AKT signal transduction expression at different drug administration times, in A973 cells. The findings of this study provide support for the potential improvement in the therapeutic effect when TKI is combined with radiotherapy.
Material and methods
Reagents
Roswell Park Memorial Institute (RPMI) 1640 cell culture medium (Gibco, Carlsbad, CA, USA) was provided by Hangzhou Sijiqing Biological Engineering Materials Co, Ltd (Hangzhou, People’s Republic of China). Primary antibodies against C-MET and phosphorylated C-MET (p-C-MET), as well as against AKT and phosphorylated AKT (p-AKT), were obtained from Santa Cruz Biotechnology Inc (Dallas, TX, USA). Secondary antibody, horse radish peroxidase (HRP)-labeled goat anti-mouse IgG, was obtained from Jackson Laboratories, Inc. (West Grove, PA, USA). Erlotinib (a TKI) was generously provided by F Hoffman-La Roche Ltd (Basel, Switzerland); Annexin V and propidium iodide (PI) were purchased from Cell Signaling Technology, Inc (Beverly, MA, USA).
Cell culture and ionizing radiation treatment
The A973 lung cancer cell line (Peking University, Beijing, People’s Republic of China)Citation10,Citation11 was maintained in 10% heat-inactivated fetal bovine serum (Amresco LLC, Solon, OH, USA) and RPMI 1640 medium supplemented with 4 mM glutamine, 100 mg/mL streptomycin, 100 IU/mL penicillin, and 20 mM hydroxyethyl piperazineethanesulfonic acid (HEPES) (Amresco LLC), in a humid atmosphere consisting of 5% CO2 and 95% air, at 37°C. In a tissue-culture flask (75 cm2) (Nest Biotechnology Co., Ltd, People’s Republic of China), exponentially growing A973 cells were irradiated by linear accelerator at a dose rate of 2 Gy/min, with 60Co as the radioactive source.
Clonogenic survival
The ability of cells to maintain clonogenic capacity was defined as clonogenic survival. Briefly, samples with or without erlotinib were exposed to radiation doses of 0, 1, 2, 4, 6, 8, and 10 Gy. The cells were fixed with methanol and stained with Giemsa after a 14-day incubation period and then, the colonies were counted (ie, those containing more than 50 cells). The experiments were repeated three times, and the data were represented as means ± standard deviation. A dose-survival curve was then generated. Survival parameters, such as sublethal damage repair (quasi-threshold dose [Dq]), extrapolation number (N),Citation12–Citation14 and the cellular radiosensitivity (mean lethal dose [D0]), were calculated using a multitarget, single-hit model.Citation12–Citation14 The cell-survival curves were plotted using Origin® 7.5 (OriginLab Corp, Northampton, MA, USA) software, while the sensitizer enhancement ratios (SERs) were calculated as D0 values.Citation12–Citation14
Cell apoptosis analysis by flow cytometry
Six groups of cells were used in the experiments: the control (non-treated) group and five experimental groups treated with erlotinib-only, irradiation-only, irradiation after erlotinib, irradiation before erlotinib, or erlotinib concurrent with irradiation. In the groups that received both erlotinib and irradiation, erlotinib was administered 24 hours before irradiation, concurrent with irradiation, and 24 hours after irradiation, respectively. Erlotinib was removed, in all groups, after 12 hours of incubation. Each test was performed three times. The concentration of erlotinib used in the experiments was 50 nM. All combination-treatment groups, as well as the radiation-only group, were exposed to 6 Gy X-rays. After treatment, all the cells were harvested. For the detection of apoptotic cells, the cells were trypsinized and washed with cold phosphate-buffered saline, and then, they were stained with PI and annexin V for 15 minutes in the dark. The cells were then analyzed using fluorescence-activated cell sorting (FACS) on a FACSCalibur machine (BD Biosciences, San Jose, CA, USA).
Western blot analysis
After irradiation and/or erlotinib treatment, the C-MET-, p-C-MET-, AKT-, and p-AKT-expression levels in the A973 cells were determined by Western blot. The Western blot treatment groups were the same as those used for the FACS. Electrophoresis was performed with polyacrylamide gel under reducing conditions, and then, the proteins were transferred to a nitrocellulose membrane (Bio-Rad Laboratories, Hercules, CA, USA). The blots were incubated with the first antibody at room temperature for 4 hours, followed by incubation with the secondary antibody (horseradish peroxidase labeling goat anti mouse IgG) for 1 hour. The film was then developed and fixed.Citation15,Citation16 Semiquantification analysis was performed with Image Tool 3.0 grayscale scanning software (UTHSCSA ImageTool 3.0, Department of Dental Diagnostic Science at The University of Texas Health Science Center, San Antonio, TX, USA).
Statistical analysis
The data were plotted as the means ± standard deviation. Analysis of variance (ANOVA) tables were used for comparisons between multiple groups using SPSS version 17 software (SPSS, Chicago, IL, USA).
Results
Survival curve after different dose irradiation with or without erlotinib
The survival curves of the A973 cells under different treatment regimens are shown in . The radiobiological parameters of the A973 cells were as follows: D0 = 1.12, Dq = 0.11, and N = 1.25, in the groups receiving irradiation after administration; D0 = 1.60, Dq = 0.16, and N = 1.26, in the groups receiving simultaneous irradiation; D0 = 1.78, Dq = 0.34, and N = 1.55, in the groups receiving irradiation before administration; and D0 = 2.45, Dq = 1.17, and N = 3.01, in the groups receiving only radiation. The SERs of the groups receiving erlotinib irradiation after administration, simultaneous irradiation and administration, and irradiation before administration were 2.19, 1.53, and 1.38, respectively. The enhanced SERs in the erlotinib-treated cells revealed that the biological effect of irradiation improved significantly when the cells were treated with erlotinib, while the best radiosensitive effect was observed in the group receiving irradiation after erlotinib (P < 0.05) compared with the other groups.
Figure 1 Dose-survival curves of the A973 cells after irradiation with or without erlotinib, based on different administration schedules. (A) Radiation groups; (B) groups receiving irradiation before erlotinib administration; (C) groups receiving simultaneous irradiation and erlotinib administration; and (D) groups receiving irradiation after erlotinib administration.
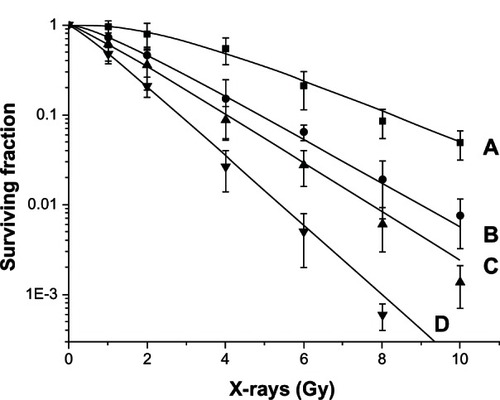
Cell apoptosis
Compared with single-treatment groups, the A973 cells had higher apoptosis rates in all the combination-treatment groups. This effect was the most significant in the group receiving irradiation after administration (P < 0.05) compared with every other group. The percentage of apoptosis indicated that apoptosis might be an important mechanism associated with the radiosensitization effect (). The apoptosis rates were as follows: 0.5% ± 0.4% for the control groups; 21.3% ± 3.5%, for the radiation-only groups; 13.2% ± 2.7%, for the erlotinib-only groups; and 54.7% ± 6.3%, for the groups receiving radiation after erlotinib (P < 0.05) (group receiving irradiation after erlotinib administration compared with the other groups, based on the ANOVA analysis); were 37.6% ± 4.2%, for the groups receiving radiation and erlotinib simultaneously; and were 36.8% ± 3.9%, for the groups receiving radiation before erlotinib.
Figure 2 The apoptosis rates for the A973 cells after different treatment regimens (Q1–Q4 represent different quadrants, with Q4 showing the apoptotic cells). (A) Control groups; (B) Radiation-only groups; (C) Erlotinib-only groups; (D) Irradiation after erlotinib administration groups; (E) Simultaneous irradiation and erlotinib administration groups; and (F) Irradiation before erlotinib administration groups.
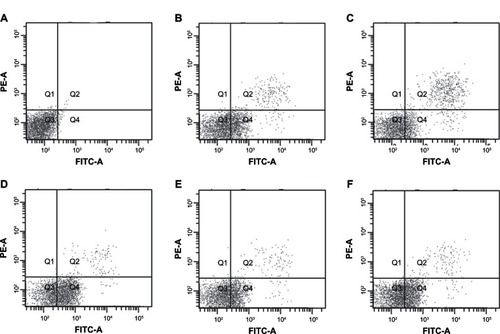
Expression of C-MET, p-C-MET, AKT and p-AKT in the A973 cells after irradiation and/or erlotinib treatment
After treatment with 6 Gy radiation with or without erlotinib, the protein expression of C-MET, p-C-MET, AKT, and p-AKT increased in all of the groups. In all combination groups, the expression of these proteins was the highest in groups receiving irradiation before erlotinib administration and the lowest in groups receiving irradiation after administration (). The C-MET and AKT levels were correlated with those of p-C-MET and p-AKT (). Furthermore, trends were related to the apoptotic rate in the A973 cells (P < 0.05) (based on the correlation test). In grey scanning analysis, the expression of C-MET and p-C-MET was semiquantitated using the C-MET/β-actin ratio, whereas the expression of AKT and p-AKT was semiquantitated using the AKT/β-actin ratio. The relative p-C-MET-expression levels of the control groups and the groups receiving radiation-only, erlotinib-only, irradiation after erlotinib administration, simultaneous irradiation and erlotinib administration, and irradiation before erlotinib administration were 17.2% ± 8.3%, 28.8% ± 6.5%, 20.0% ± 5.1%, 40.5% ± 6.7%, 57.8% ± 9.6%, and 61.8% ± 11.8%, respectively. The relative p-AKT-expression levels of the control groups and the groups receiving radiation-only, erlotinib-only, irradiation after erlotinib administration groups, simultaneous irradiation and erlotinib administration, and irradiation before erlotinib administration were 19.2% ± 4.1%, 26.2% ± 3.8%, 22.6% ± 4.3%, 39.5% ± 7.1%, 54.9% ± 4.3%, and 60.7% ± 7.1%, respectively. The expression levels of C-MET, p-C-MET, AKT and p-AKT in the radiation-only groups and combination groups were significantly higher than in the control groups (P < 0.05). The expression levels of C-MET, p-C-MET, AKT, and p-AKT in the groups receiving irradiation before erlotinib administration, simultaneous irradiation concurrent administration, and erlotinib-only administration were higher than the expression levels in the group receiving irradiation after erlotinib administration (P < 0.05).
Figure 3 Western blot analysis of C-MET, p-C-MET, AKT, and p-AKT in the A973 cells subjected to 6 Gy irradiation and/or erlotinib-treatment. Left: expressions of C-MET, p-C-MET, AKT, and p-AKT in the A973 cells. Upper right: histogram of C-MET and p-C-MET analysis by grey scanning. Lower right: histogram of AKT and p-AKT analysis by grey scanning. (A) Control groups; (B) Radiation groups; (C) Erlotinib groups; (D) Irradiation after erlotinib administration groups; (E) Simultaneous irradiation and erlotinib administration groups; and (F) Irradiation before erlotinib administration groups.
Abbreviations: p-AKT, phosphorylated AKT; p-C-MET, phosphorylated C-MET.
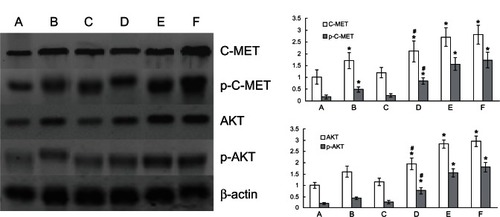
Discussion
Previous clinical studies assessing the combined treatment of radiation and the erlotinib demonstrated tolerance to toxicity and enhanced efficacy.Citation17–Citation20 However, basic research in this area is lacking, and the optimal administration schedule for this combined treatment remains undetermined. Moreover, the mechanisms involved in optimizing the effects of such treatment need further research. The results of this study show that the radiosensitizing effects of erlotinib differed when the drug was administered according to different administration schedules, suggesting that these effects might be related to PI3K signal transduction.
Compared with the radiation-only group and the erlotinib-only group, the clonogenic assay showed more desirable results in every combination group. Further, the lethal effect in the group receiving radiation after erlotinib administration was higher than the effects observed in the other combination groups. Our results also showed that radiation after erlotinib administration enhanced the apoptosis rate of the A973 cells more significantly than did the other treatment groups. This result suggests that apoptosis plays an important role when erlotinib is combined with radiation. Increased cell apoptosis was further stimulated by changes in the expression levels of the proteins; these changes were related to PI3K signal transduction. The levels of C-MET, p-C-MET, AKT, and p-AKT proteins were higher in all of the treatment groups and were maximized in those groups receiving irradiation before administration; the lowest levels were observed in the groups receiving irradiation after administration. The trends exhibited by the C-MET and AKT levels mirrored those of the p-C-MET and phosphor-AKT levels, respectively (). As such, the expression levels and variation trends of these genes provided the experimental basis for different radiosensitization effects at different administration times.
The reason for the different radiosensitization effects associated with erlotinib may be related to the activation of PI3K signal transduction. More specifically, the cross talk of mitogen-activated protein kinase (MAPK) and PI3K may provide an answer to this question. Both EGFR-RAS-MEK-extracellular signal-regulated kinase (ERK) signal transduction and C-MET-PI3K-AKT signal transduction have roles in the prevention of apoptosis, the regulation of cell cycle progression, and cell proliferation. Further, there are multiple levels of cross talk between the PI3K/AKT and Ras/MAPK signaling pathways.Citation21–Citation24 When C-MET-PI3K-AKT signal transduction was activated by radiation,Citation25,Citation26 the high levels of C-MET, p-C-MET, AKT, and p-AKT promoted cell survival and prevented cell death, thereby decreasing the killing effect of the TKIs administered simultaneously with radiation.
In agreement with the results from previous studiesCitation27–Citation29 that assessed the combination of erlotinib and irradiation in tumor treatment, our study also found that erlotinib exerted a radiosensitive effect when it was combined with irradiation. However, we explored the different radiosensitive effects observed when erlotinib was combined with radiation, using different schedules, as well as the relationship between such treatment and PI3K signal transduction. The results of this study indicate that PI3K signal transduction may play an important role in the effect exerted by the combination of erlotinib and irradiation. This study also provides useful data regarding the regulating effect of TKIs administered simultaneously with radiation, via the PI3K signal transduction inhibitor.
In summary, our study explored optimal administration schedules (irradiation after administration) for the combination of erlotinib and radiation, as well as the relationship between such treatments and PI3K signal transduction. Although this study was preliminary, the radiotherapy effects and gene expression results may be correlated. Nevertheless, many issues remain to be addressed, and we believe that the application of irradiation in combination with EGFR inhibitors has great potential that requires further fundamental research.
Acknowledgments
This research was funded by the Tianjin Medical University Science and Technology Development Fund (Grant No 2010KY31) and the Tianjin Health Bureau Science and Technology Development Fund (Grant No 2012KZ066).
Disclosure
The authors report no conflicts of interest in this work.
References
- GschwindAFischerOMUllrichAThe discovery of receptor tyrosine kinases: targets for cancer therapyNat Rev Cancer2004436137015122207
- BaselgaBEpidermal growth factor receptor pathway inhibitorsUpdate Cancer Ther20061299310
- CiarielloFTortoraGEGFR Antagonists in Cancer TreatmentN Engl J Med2008358111160117418337605
- CiarielloFTortoraGA novel approach in the treatment of cancer: targeting the epidermal growth factor receptorClin Cancer Res20017102958297011595683
- ZhuZTargeted cancer therapies based on antibodies directed against epidermal growth factor receptor: status and perspectivesActa Pharmacol Sin20072891476149317723181
- HarariPMEpidermal growth factor receptor inhibition strategies in oncologyEndocr Relat Cancer200411468970815613446
- DassonvilleOBozecAFischelJLMilanoGEGFR targeting therapies: monoclonal antibodies versus tyrosine kinase inhibitors. Similarities and differencesCrit Rev Oncol Hematol2007621536117324578
- KrisMGNataleRBHerbstRSEfficacy of gefitinib, an inhibitor of the epidermal growth factor receptor tyrosine kinase, in symptomatic patients with non-small cell lung cancer: a randomized trialJAMA2003290162149215814570950
- FukuokaMYanoSGiacconeGMulti-institutional randomized phase II trial of gefitinib for previously treated patients with advanced non-small-cell lung cancer(The IDEAL 1 Trial) [corrected]J Clin Oncol200321122237224612748244
- LiuFLiYYuYFuSLiPCloning of novel tumor metastasis-related genes from the highly metastatic human lung adenocarcinoma cell line Anip973J Genet Genomics200734318919517498616
- LiWMuDSongLMolecular mechanism of silymarin-induced apoptosis in a highly metastatic lung cancer cell line anip973Cancer Biother Radiopharm201126331732421711112
- ChuPMChiouSHSuTLEnhancement of radiosensitivity in human glioblastoma cells by the DNA N-mustard alkylating agent BO-1051 through augmented and sustained DNA damage responseRadiat Oncol20116721244709
- CariveauMJStackhouseMCuiXLClofarabine acts as radiosensitizer in vitro and in vivo by interfering with DNA damage responseInt J Radiat Oncol Biol Phys200870121322018037589
- QuanzMBerthaultNRoulinCSmall-molecule drugs mimicking DNA damage: a new strategy for sensitizing tumors to radiotherapyClin Cancer Res20091541308131619190126
- KimIAShinJHKimIHHistone deacetylase inhibitormediated radiosensitization of human cancer cells: class differences and the potential influence of p53Clin Cancer Res2006123 Pt 194094916467109
- MaPCJagadeeswaranRJagadeeshSFunctional expression and mutations of c-Met and its therapeutic inhibition with SU11274 and small interfering RNA in non-small cell lung cancerCancer Res20056541479148815735036
- LindJSLagerwaardFJSmitEFSenanSPhase I study of concurrent whole brain radiotherapy and erlotinib for multiple brain metastases from non-small-cell lung cancerInt J Radiat Oncol Biol Phys20097451391139619289264
- StinchcombeTEBogartJANovel approaches of chemoradiotherapy in unresectable stage IIIA and stage IIIB non-small cell lung cancerOncologist201217568269322531360
- MerimskyOChengCKAuJSvon PawelJReckMEfficacy and safety of first-line erlotinib in elderly patients with advanced non-small cell lung cancerOncol Rep201228272172722614912
- MehtaVKRadiotherapy and erlotinib combined: review of the preclinical and clinical evidenceFront Oncol201223122645717
- MainesMDBiliverdin reductase: PKC interaction at the cross-talk of MAPK and PI3K signaling pathwaysAntioxid Redox Signal20079122187219517919068
- AksamitieneEKiyatkinAKholodenkoBNCross-talk between mitogenic Ras/MAPK and survival PI3K/Akt pathways: a fine balanceBiochem Soc Trans201240113914622260680
- MengesCWMcCanceDJConstitutive activation of the Raf-MAPK pathway causes negative feedback inhibition of Ras-PI3K-AKT and cellular arrest through the EphA2 receptorOncogene200827202934294018059341
- JungKHParkBHHongSSProgress in cancer therapy targeting c-Met signaling pathwayArch Pharm Res201235459560422553051
- De BaccoFLuraghiPMedicoEInduction of MET by ionizing radiation and its role in radioresistance and invasive growth of cancerJ Natl Cancer Inst2011103864566121464397
- LiuXYaoWNewtonRCScherlePATargeting the c-MET signaling pathway for cancer therapyExpert Opin Investig Drugs20081779971011
- SteaBFalseyRKislinKTime and dose-dependent radiosensitization of the glioblastoma multiforme U251 cells by the EGF receptor tyrosine kinase inhibitor ZD1839 (‘Iressa’)Cancer Lett20032021435114643025
- AnderssonUJohanssonDBehnam-MotlaghPJohanssonMMalmerBTreatment schedule is of importance when gefitinib is combined with irradiation of glioma and endothelial cells in vitroActa Oncol200746795196017917826
- NandaADias-SantagataDCStubbsHUnusual tumor response and toxicity from radiation and concurrent erlotinib for non-small-cell lung cancerClin Lung Cancer20089528528718824451