Abstract
Somatostatin receptor 2 (SSTR2), specifically over-expressed on many tumor cells, is a potential receipt for active targeting in cancer therapy. In the present study, octreotide (Oct), which had high affinity to SSTR2, was attached to N-(2-hydroxypropyl) methacrylamide (HPMA) polymeric system to enhance the antitumor efficiency of the anticancer drug doxorubicin (DOX). Two kinds of cell lines (HepG2 and A549), which overexpress SSTR2, were chosen as cell models. Compared with non-modified conjugates, Oct-modified conjugates exhibited superior cytotoxicity and intracellular uptake on both HepG2 and A549 cell lines. This might be due to the mechanism of receptor-mediated endocytosis. Subsequently, the in vivo biodistribution and antitumor activity evaluations showed that Oct modification significantly improved the tumor accumulation and antitumor efficacy of HPMA copolymer conjugates in SSTR2 over-expressed Kunming mice bearing H22 tumor xenografts. In summary, Oct-modified HPMA polymer-DOX conjugates might be a promising system for the treatment of SSTR2 over-expressed cancers.
Introduction
Cancer is one of the leading threats of human health in the world with an estimated 7 000 000 deaths per year. Low-molecular-weight anticancer drugs, such as doxorubicin, are employed to kill the cancer cells. However, they engaged several disadvantages including the low tumor accumulation and the high systemic toxicity (Cole & Deeley, Citation1998). To overcome these drawbacks, several strategies have been developed including pro-drugs and polymeric systems (van Brakel et al., Citation2008). Recently, N-(2-hydroxypropyl) methacrylamide (HPMA) copolymers attract much attention due to their unique characteristics such as passive tumor targeting and backbone modification. Drugs can be covalently conjugated to HPMA polymeric carriers, which provide specific accumulation at the tumor site based on the enhanced permeability and retention (EPR) effect (Rihova, Citation1998). Furthermore, in order to further improve the targeting efficiency, researchers combined HPMA polymeric systems with targeting moieties, which can specifically recognize receptors or antigens on cell surface so as to improve the drug accumulation not only in tumor tissue but also in intracellular level (Kopecek & Kopeckova, Citation2010). Most of these studies proved that the incorporation of targeting peptidic ligands is an effective and potential strategy to improve tumor-specific chemotherapy (Greish et al., Citation2011, Liu et al., Citation2009, Mitra et al., Citation2006, Yang et al., Citation2012), which encouraged a boom of new peptide discovery.
Somatostatin (SST), a neuropeptide endogenously produced by endocrine cell D, performs inhibitory action against several endocrine systems through blood circulation. Moreover, it elicits biological effects on tumors mediated by somatostatin receptors (SSTRs) (Watt et al., Citation2008). Fifty five kinds of human primary tumors were reported to express at least one SSTR subtype, and 46 of them were proved to overexpress SSTR2 with high affinity for the synthetic SST analogue octreotide (Oct) (Reubi et al., Citation1994). Thus, Oct is considered to be a valuable peptide both in clinical application and in cancer cell-specific targeting strategy. In clinical tumor scintiphotograph, Pentetreotide, a radio-diagnostic agent 111In-DIPA -octreotide, was widely used due to its high affinity to SSTR2 (Olsen et al., Citation1995). Moreover, Oct-modified anticancer agents, such as doxorubicin (DOX) loaded sterically stabilized liposomes (Oct-SSL-DOX), showed enhanced tumor accumulation and anticancer efficacy on SSTR2-overexpressed tumor models, suggesting that Oct might be a potential ligand in anticancer drug delivery (Engel et al., Citation2007, Shen et al., Citation2008, Zhang et al., Citation2010). However, Oct-modified HPMA copolymer for SSTR2-positive tumor cells has not been reported yet.
In the present study, a SSTR2-positive tumor cell-targeting drug delivery system, named HPMA copolymer-DOX-Oct conjugate, was synthesized and characterized. The in vitro cytotoxicity, intracellular uptake, in vivo biodistribution and antitumor activity in Kunming mice bearing H22 tumor xenografts were investigated.
Materials and methods
Materials
Octreotide was synthesized by KJ Biochem Co., Ltd. (Sichuan, China). Doxorubicin hydrochloride (DOX·HCl) was purchased from Huafeng United Technology Co., Ltd. (Beijing, China). 3-(4, 5-dimethyl- 2-tetrazolyl)-2, 5-diphenyl-2H tetrazolium bromide (MTT) and 4′, 6-diamidino-2-phenylindole (DAPI) were purchased from Sigma-Aldrich (St. Louis, MO). Fluorescein isothiocyanate (FITC) was obtained from Acros organics. L-Tyrosinamide hydrochloride (Tyr-NH2) was purchased from Bachem Americas Inc. (Torrance, CA).
Cell culture and animals
Human hepatocarcinoma cell line HepG2 was purchased from American Type Culture Collection (ATCC). Non-small cell lung cancer cell line A549 was purchased from China Center for Type Culture Collection, Chinese Academy of Sciences. H22 cell line was a kind gift from Department of Pharmacology, College of Basic and Forensic Medicine, Sichuan University. Cells were cultured in RPMI-1640, supplemented with 10% FBS and penicillin (100U/ml) / streptomycin (100U/ml) at 37 °C in a 5% CO2/95% air atmosphere.
Male Kunming mice (18 ± 2 g) were purchased from Animal Centre of the Institute of West China Medical Center. Animals were housed in accordance with approved guidelines by the animal welfare committee at Sichuan University and were provided with food and water.
Synthesis and characterization of HPMA copolymer-DOX-Oct conjugates
Synthesis of monomers
Monomers {N-(2-hydroxypropyl) methacrylamide (HPMA) (Duncan et al., Citation1987), N-methacryloyl-glycylglycyl-pnitrophenyl ester (MA-GG-ONp) (Rejmanová et al., Citation1977), N-methacryloyl-glycyl-phenylalanyl-leucyl-glycyldoxorubicin (MA-GFLG-DOX) (Ulbrich et al., Citation2000) and N-methacryloyl-tyrosinamide (MA-Tyr-NH2) (Lammers et al., Citation2007)} were synthesized according to previous reports. The 1H-NMR spectrums of these monomers were showed in appendices. Among these monomers, MA-GG-ONp and MA-Tyr-NH2 were used for targeting peptide attachment and 131I labeling, respectively.
Synthesis and characterization of HPMA polymers
The HPMA polymer precursors were synthesized by random radical precipitation copolymerization according to previous reports (Rathi et al., Citation1991). Briefly, HPMA and other necessary comonomers were dissolved in acetone with AIBN as the initiator. The solution was purged with nitrogen and stirred at 50 °C for 24 h. After the evaporation of the solvent, the solid residue was dissolved in methanol and fractionated on a Sephadex LH-20 column using methanol as eluent. The purified fraction was concentrated by vacuum evaporation and isolated by precipitation into diethyl ether. To determine the -ONp content, the copolymers were hydrolyzed with sodium hydroxide solution (0.1 M), and the released p-nitrophenol was measured at 400 nm by UV–Vis assay (Yang et al., Citation2012). The total Dox content was determined by UV spectrophotometry (ε = 11 500 L mol−1 cm−1, λ = 488 nm, water) (Subr et al., Citation1992). The fluorescence intensity of DOX (excitation at 488 nm and emission at 560 nm) and FITC (excitation at 494 nm and emission at 518 nm) were measured by fluorescence spectrophotometer. The molecular weight and polydispersity index (PDI) of the conjugates were estimated by size exclusion chromatography on a Superose 200 10/300GL analytical column (Amersham Biosciences, Piscataway, NJ) via calibration curve method.
Synthesis and characterization of HPMA copolymer-DOX-Oct conjugates
Oct was attached to HPMA copolymer-DOX conjugates (P-DOX) through substituting terminal -ONp group of the non-degradable dipeptide glycylglycine (GG). Briefly, P-DOX containing reactive -ONp groups and Oct (molar ratio of -ONp group and Oct is 1:1) were dissolved into water. The reaction mixture was stirred at room temperature for 24 h. The mixture was purified in deionized water using dialysis tube with 8000–14 000 molecular weight cut off. The Oct contents of the conjugates were determined by amino acid analysis (Commonwealth Biotech. Inc., Richmond, VA). Meanwhile, in order to remove highly cytotoxic -ONp groups, the P-DOX without Oct conjugation was purified in alkalescent solution using the same dialysis tube.
131I-radiolabeling of HPMA copolymers
The HPMA polymer precursors containing Tyr-NH2 was labeled with 131I by Iodogen method. Briefly, the PBS solution of copolymers (30 mg/ml, 100 μl) was added to Idogen tubes along with 187.5μCi 131I in 3μl saline. The mixture was agitated gently for 15 min at room temperature. Subsequently, 131I-labeled copolymers were purified by a Sephadex LH-20 column using saline as eluent.
In vitro biological characterization of HPMA copolymer-DOX conjugates
Quantitative PCR (QPCR) for SSTR2 mRNA
Total RNA was isolated from HepG2, A549 and H22 cells by extraction with Trizol Reagent (Applied Biosystems, Foster City, CA) following the manufacturer’s instructions and then reverse-transcribed into cDNA using random hexamer and SuperScript III reverse transcriptase (Invitrogen, Carlsbad, CA). Quantitative real-time PCR was performed by the Applied Biosystems 7500 Real-Time PCR System using Power SYBR Green PCR Master Mix (Applied Biosystems) in triplicate for each sample and each gene. The primers and probes were used as previously described (Singh et al., Citation2011).
Internalization assay by inverted fluorescence microscopy
The FITC-labeled non-modified (P-FITC) and Oct-modified (P-FITC-Oct) HPMA copolymer conjugates (equivalent of FITC) were incubated with HepG2 and A549 cells at 37 °C in the presence or absence of free Oct, respectively. At predetermined time points, cells were washed with PBS for three times. DAPI (10 µg/ml) was added to visualize the nuclei 10 min before imaging. Cell fluorescent imaging was performed using inverted fluorescence microscopy (XD-RFL, Sunny Optical Technology).
Quantitative study of uptake by flow cytometry
HepG2 and A549 cells were seeded on 12-well plates at a density of 1 × 105 cells per well. After incubation for 24 h, the cells were treated with P-DOX or P-DOX-Oct at 37 °C for 2 h and harvested. The samples were washed with PBS for three times followed by flow cytometric analysis immediately. The mean fluorescence intensity of 1 × 104 cells was recorded for each sample.
Cytotoxicity assay
The cytotoxicity of P-DOX and P-DOX-Oct against HepG2 and A549 cells was evaluated by MTT assay. The cells were seeded onto 96-well plates at a density of 7 × 103 cells per well and incubated for 48 h after the treatment with P-DOX and P-DOX-Oct for another 72 h at 37 °C. The cells were incubated with MTT (5 mg/ml, 20 µl per well) for 3 h. Then the supernatant was removed before adding dimethyl sulfoxide (150 µl per well) into the wells to dissolve the formazane of MTT. The absorption at 570 nm was recorded with an ELISA plate reader (Bio-Rad, Microplate Reader 550, Bio-Rad, Hercules, CA), and the growth inhibitory (GI) rate was calculated according to the following equation:
T is the absorption value of the treatment group; C is the absorption value of the control (untreated) group; B refers to the absorption value of the culture medium. IC50 values (µg/ml) were calculated by SPSS software, IBM, Armonk, NY.
Morphological analysis with DAPI staining
Apoptotic morphological changes of HepG2 and A549 cells were assessed by fluorescent microscopy with DAPI staining. After treatments of P-DOX or P-DOX-Oct for 24 h, the cells were washed with PBS, and stained with DAPI for 10 min at 37 °C. Then, cells were observed and photographed under inverted fluorescence microscopy (XD-RFL, Sunny Optical Technology, Yuyao, CHN).
In vivo study
Biodistribution study
The solid tumor model was obtained by subcutaneous (s.c.) injection of H22 cells (1 × 106) into the right flank of Kunming mouse. About 14 d after the inoculation, the animals were fed with sodium iodide solution (0.9%, w/v) for 2 d before the following experiments. Animals were randomized into two groups (12 mice/group), and intravenously (i.v.) administered with 131I-labeled non-modified HPMA copolymers (131I-P) or Oct-modified copolymers (131I-P-Oct) via tail vein (30 μCi per mouse).
Biodistribution studies were carried out at 24, 72 and 120 h post administration. At each time point, blood samples were collected from fossa orbitalis. Subsequently, the heart, lung, liver, spleen, kidney and tumor tissues were obtained, washed with saline and weighed. Then, all samples were gamma-counted (Perkin Elmer Wizard, 1470 Automatic Gamma Counter). The percentage-injected dose per gram tissue (%ID/g) was calculated.
In vivo antitumor activity
The antitumor activity of the conjugates was evaluated using Kunming mice bearing H22 xenografts. Tumors were inoculated and allowed to grow for 3 d before treatment. Tumor-bearing mice were randomized into four groups (14 mice per experimental group, 8 mice for control group) and intravenously administered with saline (control group), DOX·HCl, P-DOX and P-DOX-Oct (equivalent to 10 mg/kg DOX) in saline solutions via tail vein, respectively. Each mouse was injected on day 0, 2, 4 and 6. Observation lasted for 21 d. Tumor sizes were measured with calipers in two perpendicular diameters every 3 d and calculated as tumor volumes through the following equation:
“a” means the longer diameter, “b” means the shorter diameter.
The survival time was regularly scored. After the mice were sacrificed, the tumors were excised, weighed and photographed.
Statistical analysis
Data were presented as Mean ± SD. For statistical analysis of two groups, Student’s t test for independent means was used. The differences between the effects of different treatments for more than two groups were analyzed by one-way ANOVA using SPSS 17 software. Significant differences were defined as p < 0.05, and highly significant p < 0.01.
Results and discussion
Polymer synthesis and characterization
Non-modified HPMA copolymer conjugates (P-DOX, P-FITC and 131I-P) and Oct-modified HPMA copolymer conjugates (P-DOX-Oct, P-FITC-Oct and 131I-P-Oct) were successfully synthesized. The classical synthesis process was expressed as shown in . Dox was attached to the polymer backbone via a lysosomally degradable oligopeptide linker glycylphenylalanylleucylglycine (GFLG), which had been well studied in the field of drug release pharmacokinetics (Subr et al., Citation1992). Targeting peptide Oct was conjugated by the non-degradable dipeptide glycylglycine (GG). The feed composition and characteristics of these conjugates were shown in . The molecular weight of non-modified polymers was 16–28 kDa while slightly increased with the incorporation of Oct. The Dox contents of P-DOX and P-DOX-Oct were 5.18 wt% and 5.76 wt%, respectively. According to the result of amino acid detection, the content of Oct peptide in P-DOX-Oct, P-FITC-Oct and 131I-P-Oct were 3.9, 2.0 and 3.3 mol%, respectively.
Table 1. Characteristics of the synthesized HPMA copolymers.
QPCR for SSTR2 mRNA
It has been documented that the efficacy of HPMA copolymer-based nanomedicines could be enhanced by the incorporation of targeting moieties (peptides) via selectively binding ligands to antigens or receptors unique expressed or over-expressed on the target cells (Yuan et al., Citation2008). In this study, HPMA copolymers served as a linear scaffold, to which Oct was attached as a targeting moiety to SSTR2-expressed cells. In order to further demonstrate the role of the targeting drug delivery system in vitro and in vivo, three kinds of tumor cell lines with different expression levels of SSTR2 were selected by quantitative real-time PCR. showed that SSTR2 were expressed on all tested cells with the general level of SSTR2 expression as: HepG2 < A549, which correlated well with the report that A549 cell line showed a high protein expression of SSTR2 by immunohistochemistry (Shen et al., Citation2008). Moreover, H22 cell line, a widely used mouse hepatocellular carcinoma tumor model, showed significantly highest SSTR2 expression level. H22 cell line was cultivated in mouse ascites as suspension cell, which could be planted subcutaneously to create mouse hepatocellular xenograft. Therefore, both HepG2 and A549 cell lines were chosen to be the SSTR2 positive cell models in in vitro cell experiments and H22 was selected in the in vivo study.
Internalization assay
Generally, when multiple copies of targeting peptides are incorporated into HPMA copolymer, the binding affinity to their receptor may significantly increase, and the cellular uptake of copolymers might be enhanced due to the multivalent interactions (Mammen et al., Citation1998). The internalization of P-FITC and P-FITC-Oct in presence or absence of free Oct by HepG2 and A549 cell lines were observed under inverted fluorescence microscopy. In terms of HepG2 cells, more significant fluorescence signal of P-FITC-Oct () was observed compared to that of non-modified P-FITC () suggesting that a higher cellular uptake of copolymers could be achieved by the modification of Oct. To further verify the specific ligand-receptor mediated endocytosis by Oct, P-FITC and P-FITC-Oct were co-incubated and competed with free Oct. No remarkable changes of fluorescence were seen in non-modified P-FITC (), while the fluorescence intensity of P-FITC-Oct decreased significantly () possibly due to the competitive interaction between free Oct and conjugated Oct with the SSTR2 over-expressed on HepG2 cells. Similar results were also obtained in A549 cells (, , and ). All these findings indicated that the Oct covalently attached to the side chain of the polymer played an important role in enhancing the cell uptake of HPMA conjugates via ligand-receptor mediated endocytosis in SSTR2 over-expressed cells.
Figure 3. Internalization of P-FITC and P-FITC-Oct (equivalent to 50 mM of FITC) co-incubated with or without free Oct (0.04 mg/ml) in HepG2 (A–D) and A549 (E–H) cells by inverted fluorescence microscopy. The green signal indicated FITC-labeled conjugates while blue signal indicated nuclei. A: HepG2 cells incubated with P-FITC; B: HepG2 cells incubated with P-FITC and free Oct; C: HepG2 cells incubated with P-FITC-Oct; D: HepG2 cells incubated with P-FITC-Oct and free Oct; E: A549 cells incubated with P-FITC; F: A549 cells co-incubated with P-FITC and free Oct; G: A549 cells co-incubated with P-FITC-Oct; H: A549 cells incubated with P-FITC-Oct and free Oct.
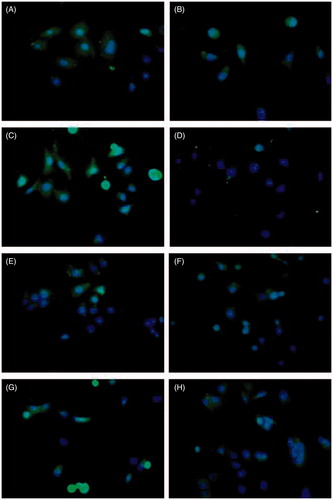
Figure 4. Quantitative study of uptake of P-DOX and P-DOX-Oct in HepG2 and A549 cells in presence or absence of free Oct. A: cells were incubated with various concentrations of conjugates for 2 h. B: cells were incubated with conjugates (0.6 mg polymer/ml) and 0.04 mg/ml of free Oct (#p < 0.05 for P-DOX-Oct versus P-DOX-Oct with free Oct).
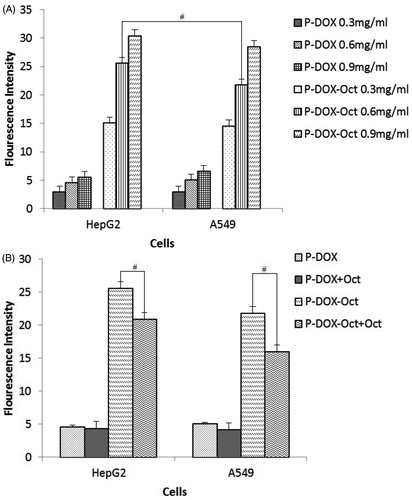
Figure 5. Morphological and nuclear changes of HepG2 (A-C) and A549 (D-F) cells treated with P-DOX and P-DOX-Oct for 24 h as determined by DAPI staining. A and D: control groups. B and E: P-DOX treated groups. C and F: P-DOX-Oct treated groups. The white arrows pointed to the apoptosis and necrosis.
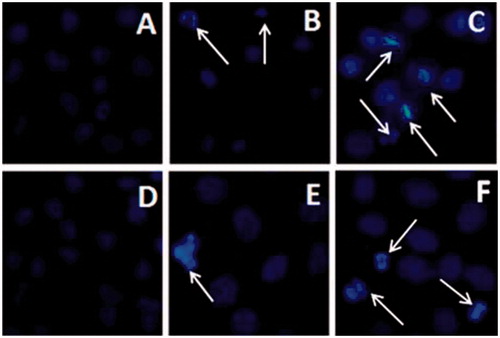
Quantitative study of uptake by flow cytometry
As shown in , for HepG2 and A549 cells, the quantitative uptake profiles of both P-DOX and P-DOX-Oct showed a concentration-dependent manners (from 0.3 mg/ml to 0.9 mg/ml). In accordance with results obtained in the Section “Internalization assay”, the Oct-modified P-DOX-Oct showed dramatically increased cell internalization compared to the non-modified P-DOX in all concentrations and in both cell lines. It is interesting although A549 had higher SSTR2 expression than HepG2, the cell uptake of P-DOX-Oct by HepG2 was unexpectedly higher than that of A549 at 0.6 mg/ml polymer but without significant difference among other two concentrations (0.3 mg/ml and 0.9 mg/ml polymers). The differences in ligands–receptor affinity and receptor-mediated endocytosis rate of different cell types could probably account for this observation, which suggested that cellular uptake of Oct-modified conjugates was not only affected by SSTR2 expression level but also depended on the different characteristics of different tumor cells. In order to further demonstrate the effect of targeting peptide on the uptake of copolymers, co-incubations of free Oct and copolymers were performed in both of the cell lines (). The addition of free Oct peptide also inhibited the internalization of P-DOX-Oct in both cell lines, which was attributed to the competitive inhibitory, whereas P-DOX was kept uninfluenced, again indicating the targeting ability of P-DOX-Oct towards SSTR2 over-expressed cells. Therefore, with an efficient drug intracellular concentration achieved by P-DOX-Oct, the superior cytotoxicity could be expected.
Cytotoxicity assay
The cytotoxicity of P-DOX and P-DOX-Oct against HepG2 and A549 cells was evaluated by MTT assay. The IC50 values of free DOX, P-DOX and P-DOX-Oct were listed in . The treatment of free DOX for 72 h showed most potent anticancer activity in both cell lines. The higher toxicity of free drug was caused by more rapid passive diffusion in vitro, while the macromolecular conjugates internalized into cell mainly through less efficient endocytosis. However, free DOX could not take advantage of EPR effect mediated by macromolecules such as P-DOX and P-DOX-Oct in vivo. When it comes to the comparison of P-DOX and P-DOX-Oct, the Oct-modified conjugates displayed significantly superior cytotoxicity with IC50 of 25.43 ± 1.73 μg/ml Dox equiv. for HepG2 cells and 37.20 ± 2.46 μg/ml Dox equiv. for A549 cells over P-DOX (IC50: 42.77 ± 2.95 μg/ml Dox equiv. for HepG2 and IC50: 59.5 ± 1.48 μg/ml Dox equiv. for A549, p < 0.05) to both SSTR2 expressed cell lines. In combination with the results obtained in the cell uptake assay, it could be assumed that the attachment of the targeting Oct could profoundly facilitate the cell internalization of the conjugates and result in enhanced intracellular drug concentration, which eventually led to the stronger cytotoxicity of P-DOX-Oct.
Table 2. The IC50 values of DOX, P-DOX and P-DOX-Oct against HepG2 and A549 cells (n = 3).
Morphological analysis with DAPI staining
Morphological and nuclear changes of HepG2 and A549 cells after treatment with conjugates were investigated by DAPI staining. No irregular bright blue fluorescence was observed in the control groups ( and ). In contrast, the cells treated with P-DOX and P-DOX-Oct for 24 h showed remarkable chromosomal condensation and morphological changes which occur in apoptotic and necrotic cells. More apoptosis and necrosis in HepG2 () and A549 () cells were induced by P-DOX-Oct than those of P-DOX treated group ( and ), which was in the line with MTT assay. Although A549 had higher expression of SSTR2, P-DOX-Oct showed stronger signal (green fluorescence) of apoptosis and necrosis in HepG2 cells (), indicating the stronger cytotoxicity of P-DOX-Oct against HepG2 cells. These results were agreed with MTT assays and the cellular uptake investigations.
In vivo biodistribution analysis
The biodistribution study of 131I-labeled non-modified (P) and Oct-modified (P-Oct) conjugates at 30 μCi per mouse dose was evaluated on Kunming mice bearing SSTR2 overexpressed H22 xenografts (). After cell recovery, H22 cell line was cultivated in peritoneal cavity of Kunming mice as suspension cell. For about 7 d, the suspension cell could be used to create subcutaneous mouse hepatocellular xenograft.
Figure 6. In vivo biodistribution of non-modified (A) and Oct-modified (B) HPMA copolymers at 24, 72 and 120 h.
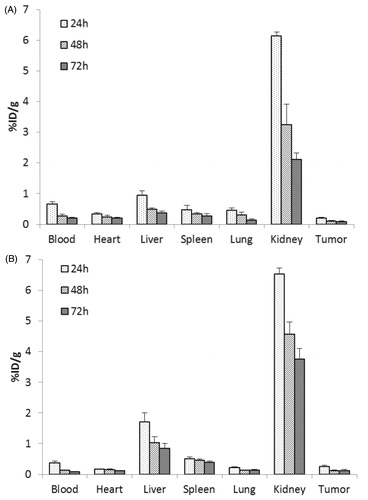
At 24, 72 and 120 h post-intravenous injection (p.i.), the accumulation of P-Oct in tumor was significantly higher than that of P (p < 0.05), but lower in heart, which indicated that Oct modification might not only achieve active tumor targeting, but also reduce the cardiac toxicity caused by doxorubicin. It should be noted that the higher accumulation of both conjugates was observed in kidney, which was in accordance with the report by Mark P. Borgman (Borgman et al., Citation2009). This was because of the renal elimination of HPMA polymer conjugates by kidney, and it showed a great potential of renal targeting strategy by using those conjugates. Furthermore, significantly higher liver accumulation of P-Oct was found compared with non-modified P, implying that Oct might play an important role in the biodistribution of copolymer. As we know, liver is an organ with large volume of blood flow and sort of SSTR2 expression, which might provide an opportunity for Oct conjugated HPMA copolymers to interact with SSTR2 in liver during their transportation by blood.
Besides, the blood clearance of P-Oct was approximately 2-fold faster than that of P. As shown in , the tumor-to-blood (T/B) ratio in P-Oct group was 2-fold higher than that in P group (p < 0.01) at 24 h and became much higher (3 folds) at 48 and 72 h. These results further demonstrated the enhanced localization in tumor and accelerated clearance from blood circulation after Oct modification.
Table 3. The tumor-to-blood ratios of the 131I-labeled copolymers (n = 4).
In vivo anti-tumor activity
In order to further study the tumor targeting and therapeutic efficiency, in vivo anti-tumor activity was investigated on Kunming mice bearing SSTR2 overexpressed H22 xenografts. As shown in , P-DOX-Oct displayed a more efficient and persistent inhibition of tumor growth than P-DOX during the 21 d of treatments. The final mean tumor volume of P-DOX-Oct group (496.7 ± 387.2 mm (Rihova, Citation1998) was much smaller than that of P-DOX (759.0 ± 340.8 mm (Rihova, Citation1998) and DOX·HCl (846.53 ± 451.0 mm (Rihova, Citation1998) groups, suggesting a better antitumor efficiency after Oct modification. Otherwise, P-DOX-Oct resulted in the highest inhibition ratio (67.90%) based on the mean weight of tumor, compared to P-DOX (42.42%) and DOX·HCl (40.62%) (). Although in vitro experiment has demonstrated that free DOX was significantly more cytotoxic than P-DOX-Oct and P-DOX probably due to its much faster cell internalization by passive diffusion, the anticancer activities of free DOX and P-DOX were comparable in vivo where macromolecules could exhibited their advantages of exploiting EPR effect. It was demonstrated that the half-life in blood was longer and the elimination rate from tumor was slower for the Dox conjugated to HPMA copolymers than for free DOX (Tomáš Etrych et al., Citation2008). Also, the EPR effect allows for the preferential accumulation of DOX-HPMA conjugates in tumor site, thus reducing the toxicity in other organs. Therefore the improved pharmacokinetics and biodistribution profiles of P-DOX based on EPR effect could make up for slower uptake by the tumor cells and resulted in almost equal anticancer efficacy compared with free DOX in the present study. Moreover, upon the EPR effect, the attachment of targeting moiety could provide an additional improvement of HPMA based DOX copolymer, which led to higher tumor accumulation observed in biodistribution study. Otherwise, the enhanced apoptosis-induction by P-DOX-Oct might also be the evidence of remarkable tumor growth inhibition.
Figure 7. Antitumor activity of DOX·HCl, P-DOX and P-DOX-Oct on Kunming mice bearing H22 xenografts. The controls were administrated with saline. Tumor volume were recorded (n = 5, #p < 0.05, versus DOX·HCl) and tumors were harvested at the end of experiments (21 d).
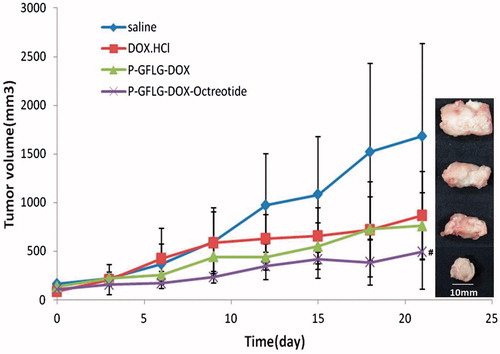
Table 4. The mean tumor weight after treatments (n = 5).
Conclusion
A novel Oct-modified HPMA copolymer-DOX conjugate was successfully synthesized and characterized. Various in vitro evaluations on two human carcinoma cell lines (HepG2 and A549) demonstrated that the active targeting conjugates displayed a significantly enhanced cellular uptake, apoptosis-induction and cytotoxicity, compared to the non-modified ones. The superior tumor accumulation and antitumor efficacy of P-DOX-Oct were further proved on a SSTR2 overexpressed H22 tumor-bearing mice model. The results demonstrated that Oct could be utilized as a targeting moiety to SSTR2 overexpressed tumor by attaching to the HPMA polymeric drug delivery system.
Declaration of interest
The research described above was supported by the National Natural Science Foundation (81072600).
References
- Borgman MP, Aras O, Geyser-Stoops S, et al. (2009). Biodistribution of HPMA copolymer-aminohexylgeldanamycin-RGDfK conjugates for prostate cancer drug delivery. Mol Pharm 6:1836–47
- Cole SP, Deeley RG. (1998). Multidrug resistance mediated by the ATP-binding cassette transporter protein MRP. Bioessays 20:931–40
- Duncan R, Kopecková-Rejmanová P, Strohalm J, et al. (1987). Anticancer agents coupled to N-(2-hydroxypropyl)methacrylamide copolymers. I. Evaluation of daunomycin and puromycin conjugates in vitro. Br J Cancer 55:165–74
- Engel JB, Schally AV, Dietl J, et al. (2007). Targeted therapy of breast and gynecological cancers with cytotoxic analogues of peptide hormones. Mol Pharm 4:652–8
- Greish K, Ray A, Bauer H, et al. (2011). Anticancer and antiangiogenic activity of HPMA copolymer-aminohexylgeldanamycin-RGDfK conjugates for prostate cancer therapy. J Contr Rel 151:263–70
- Kopecek J, Kopeckova P. (2010). HPMA copolymers: origins, early developments, present, and future. Adv Drug Deliv Rev 62:122–49
- Lammers T, Peschke P, Kuhnlein R, et al. (2007). Effect of radiotherapy and hyperthermia on the tumor accumulation of HPMA copolymer-based drug delivery systems. J Contr Rel 117:333–41
- Liu J, Kopeckova P, Buhler P, et al. (2009). Biorecognition and subcellular trafficking of HPMA copolymer-anti-PSMA antibody conjugates by prostate cancer cells. Mol Pharm 6:959–70
- Mammen M, Choi S-K, Whitesides GM. (1998). Polyvalent interactions in biological systems: implications for design and use of multivalent ligands and inhibitors. Angewandte Chemie Int Ed 37:2754–94
- Mitra A, Coleman T, Borgman M, et al. (2006). Polymeric conjugates of mono- and bi-cyclic alphaVbeta3 binding peptides for tumor targeting. J Contr Rel 114:175–83
- Olsen JO, Pozderac RV, George H, et al. (1995). Somatostatin receptor imaging of neuroendocrine tumors with indium-111 pentetreotide (Octreoscan). Semin Nucl Med 25:251–61
- Rathi RC, Kopečková P, Říhová B, Kopeček J. (1991). N-(2-hydroxypropyl) methacrylamide copolymers containing pendant saccharide moieties: synthesis and bioadhesive properties. J Polym Sci Part A: Polym Chem 29:1985–902
- Rejmanová P, Labský J, Kopeček J. (1977). Aminolyses of monomeric and polymeric p-nitrophenyl esters of methacryloylated amino acids. Die Makromolekulare Chemie 178:2159–68
- Reubi JC, Schaer JC, Waser B, Mengod G. (1994). Expression and localization of somatostatin receptor SSTR1, SSTR2, and SSTR3 messenger RNAs in primary human tumors using in situ hybridization. Cancer Res 54:3455–9
- Rihova B. (1998). Receptor-mediated targeted drug or toxin delivery. Adv Drug Deliv Rev 29:273–89
- Shen H, Hu D, Du J, et al. (2008). Paclitaxel-octreotide conjugates in tumor growth inhibition of A549 human non-small cell lung cancer xenografted into nude mice. Eur J Pharmacol 601:23–9
- Singh SP, Han L, Murali R, et al. (2011). SSTR2-based reporters for assessing gene transfer into non–small cell lung cancer: evaluation using an intrathoracic mouse model. Human Gene Ther 22:55–64
- Subr V, Strohalm J, Ulbrich K, et al. (1992). Polymers containing enzymatically degradable bonds, XII. Effect of spacer structure on the rate of release of daunomycin and adriamycin from poly [N-(2-hydroxypropyl)-methacrylamide] copolymer drag carriers in vitro and antitumour activity measured in vivo. J Contr Rel 18:123–32
- Tomáš Etrych, Tomáš Mrkvan, Petr Chytil, et al. (2008). N-(2-Hydroxypropyl)methacrylamide-based polymer conjugates with pH-controlled activation of doxorubicin. i. new synthesis, physicochemical characterization and preliminary biological evaluation. J Appl Polym Sci 109:3050–61
- Ulbrich K, Subr V, Strohalm J, et al. (2000). Polymeric drugs based on conjugates of synthetic and natural macromolecules. I. Synthesis and physico-chemical characterisation. J Contr Rel 64:63–79
- van Brakel R, Vulders RC, Bokdam RJ, et al. (2008). A doxorubicin prodrug activated by the staudinger reaction. Bioconjug Chem 19:714–18
- Watt HL, Kharmate G, Kumar U. (2008). Biology of somatostatin in breast cancer. Mol Cell Endocrinol 286:251–61
- Yang Y, Zhou Z, He S, et al. (2012). Treatment of prostate carcinoma with (galectin-3)-targeted HPMA copolymer-(G3-C12)-5-Fluorouracil conjugates. Biomaterials 33:2260–71
- Yuan F, Qin X, Zhou D, et al. (2008). In vitro cytotoxicity, in vivo biodistribution and antitumor activity of HPMA copolymer–5-fluorouracil conjugates. Eur J Pharm Biopharm 70:770–6
- Zhang J, Jin W, Wang X, et al. (2010). A novel octreotide modified lipid vesicle improved the anticancer efficacy of doxorubicin in somatostatin receptor 2 positive tumor models. Mol Pharm 7:1159–68
Appendices
HPMA monomer
MA-GG-ONp monomer
MA-GFLG-DOX monomer
MA-Tyr-NH2 monomer