Abstract
Brain cancer is a kind of tough carcinoma because the blood–brain barrier (BBB) remains a formidable obstacle in medicine. Curcumin (Cur) was determined to have anticancer potency on several kinds of carcinoma. However, its medical application was limited because of its poor bioavailability, unsatisfying dispersity and rapid metabolism in vivo. In this study, Cur was delivered by nanostructured lipid carriers (NLCs) for brain cancer treatment. The physiochemical characters of NLC-Cur were detected by using high-performance liquid chromatography, Transmission electron microscopy and photon correlation spectrum analyses. The cellular uptake and the anticancer efficiency were determined both in vitro and in vivo by flow cytometry detection, MTT assay, AO/EB, Annexin-V/PI, DCFDA and tumor-bearing mice. NLC-Cur was synthesized by using sol-gel method and with the size around 100 nm. After loaded in NLC, the IC50 of NLC-Cur was 20 µg/mL, only one-fourth of the plain drug. The plasmid concentration of Cur was highly increased (6.4-folds) in mice via intraperitoneally after loaded with NLC. Furthermore, NLC-Cur enhanced the targeting effect of Cur to brain and tumor, which finally increased the inhibition efficiency of Cur from 19.5% to 82.3%. The FITC analysis confirmed that the inhibition effect mostly came from apoptosis, but not necrosis. The time depending cellular uptake, reactive oxygen species production, markedly increased bio-availability and tumor targeting effect played an important role in the efficacy of NLC-Cur. Our work indicated the medical application of NLC-Cur on brain cancer treatment, and also provided a novel method for new anticancer agents’ development.
Introduction
Brain cancer is a very tough carcinoma because of the blood–brain barrier (BBB) (Van Tellingen et al., Citation2015). Chemical treatment for brain tumors is still subject to many constraints. Only lipid-soluble chemotherapy drugs can pass through intimal cells, and then enter the tumor cells to produce effect (Sarin, Citation2010). Such patterns affect the speed and efficiency of drug action. The current commonly used chemotherapy drug is a nitrosourea alkylating agents 1,3-bis(2-chloroethyl)-1-nitrosourea (BCNU) and 1-(2-chloroethyl)-3-cyclohexyl-1-nitrosourea (CCNU), or with PVC regimen (vincristine, procarbazine++CCNU), which have a certain effect, but will induce a delayed and cumulative myelosuppression and pulmonary toxicity (Cairncross et al., Citation2014; Venur al., Citation2015). So, the demand for safe and effective novel agent for brain cancer treatment is urgent.
Curcumin (cur) is a major yellow pigment-derived turmeric. It has been used for thousands of years as a food addictive in India. Due to its pharmacological effects, recently many researches have focused on its antioxidant, anti-inflammatory and anti-tumorigenic properties (Bandyopadhyay, Citation2014; Vecchi Brumatti et al., Citation2014; El-Bahr, Citation2015; Shanmugam et al., Citation2015). Numerous works prove that cur exerts strong anticancer effects against a far ranging of human cancer cells, including breast cancer, lung cancer and HCC (Jia et al., Citation2014; Chen et al., Citation2015; Guo et al., Citation2015). The mechanisms of the anticancer efficiency of cur are also revealed as the remarkable inhibitive effect on nuclear factor (NF-κB) activity and the downregulation of NF-κB regulated gene products, including prostaglandin E2, cyclooxygenase (COX)-2 and IL-8 (Su et al., Citation2006(a); Wang et al., Citation2006; Chiang et al., Citation2014). Besides, as a food additive, it is considered as a safe agent, which is sure to win out over many chemotherapeutic drugs. Despite the promising therapeutic functions of cur in cancer treatment, its poor oral bioavailability and extremely rapid systemic elimination have restricted its clinical applications (Park et al., Citation2013). In order to overcome these drawbacks, various kinds of carriers have been developed for drug delivery including liposomes, phospholipids, vesicles, lipid-based nanoparticle, biodegradable microsphere and so on (Gong et al., Citation2013; Shelma & Sharma, Citation2013; Wang et al., Citation2013). To conquer the disadvantages of Cur and achieve brain targeting, the lipid-based nanoparticles are perfect choices as a carrier for brain cancer treatment. Nanostructured lipid carrier (NLC) is one kind of the lipid-based nanoparticles, which has the advantages of non-toxic, biodegradable, brain targeting and diversified administration (Liu et al., Citation2015). The brain targeting effect of NLC and the anticancer effect of Cur suggest that NLC-Cur might have a remarkable anticancer efficacy for brain cancer treatment.
In this study, the encapsulation of Cur by NLC for the treatment of brain cancer is firstly explored. NLC-Cur is prepared and characterized, the bioavailability and brain targeting effect of NLC is evaluated, and anticancer effect and related mechanisms on brain cancer A172 is explored both in vitro and in vivo.
Materials and methods
Materials
Tripalmitin, oleic acid, polysorbate 80, and Cur and BCA protein assay kit were obtained from Aladdin Regent Co., Ltd. (Shanghai, China). DMEM, fetal calf serum, penicillin–streptomycin and trypsinase are obtained from Gibco (BRL, Grand Island, NY). Dimethyl sulfoxide (DMSO), FITC, AO/EB, DCFDA and Annexin V-APC/7-AAD Kit are purchased from Sigma Chemical Co. (St. Louis, MO). Female BALB-C nude mice about 5–6 weeks were purchased from Shanghai Laboratory Animal Co. (SLAC, Shanhai, China), Ltd. and boarded in stainless steel cages in a specific pathogen free animal room.
Preparation of NLC and NLC-Cur
The hot high pressure homogenization (HPH) technique was used to prepare NCL and NLC-Cur. Briefly, 100 mg of the lipid phase (tripalmitin–oleic acid, 50:50 weight ratio) melted at 70 °C was pre-emulsified in 30 mL of a 3% (w/v) polysorbate 80 solution using a homogenizer (IKA® RCT, Staufen, Germany) at 10 000 rpm for 10 min. The pre-emulsion was further subjected to hot HPH (GEA Niro Soavi NS1001L, Homogenizer Systems, Italy) at 800 bar for five cycles. The dispersion thus obtained was cooled at 4 °C with gentle stirring to form the NLC.
For drug encapsulation, 80 mg of Cur was added to the melted lipid phase, to produce NLC loaded with drug (NLC-Cur). And at the end of the protocol, the resulting suspension was washed twice with distilled water by centrifuging at 40 000 g for 4 h removing the supernatant. Thus, the free Cur was removed. The pellet was resuspended in distilled water, and stored at −20 °C for 2 h, followed by freeze-drying that converted it to powder form.
Characterization of NLC and NLC-Cur
The morphage and size distribution of NLC and NLC-Cur was observed and evaluated by transmission electron microscopy (TEM) and photon correlation spectrum (PCS). Briefly, the morphage and inner structure of NLC-Cur and blank NLC was determined by TEM measurements, drops of diluted solution of NLC-Cur and blank NLC were placed in carbon-coated copper TEM grids (150 mesh, Ted Pella Inc., Rodding, CA). The samples were visualized at 120 kV using a Philips 201 transmission electron microscope (Philips/FEI Inc., Barcliff, Manor, NY). The TEM photograph was taken with the NIH imaged software (Bethesda, MD). Particle size and the polydispersity index were determined at 25 °C by photon correlation spectroscopy (Zetasizer Nano ZS, Malvern Instruments, Malvern, UK).
Loading capacity detection
Cur was analyzed by high-performance liquid chromatography (HPLC) using Agilent 1100 series (Agilent) on a C18 column (25 cm × 4.6 mm, 5 μm). The mobile phase consists of a gradient between buffer A (acetonitrile) and buffer B (5% acetonitrile, 1%T FA). The detection wavelength was set at 420 nm and the retention time was 5.3 min. Acetonitrile (3 mL) was used to demulsified a certain amount of the well-prepared NLC-Cur (N1). The concentration of Cur was assayed by HPLC, and the amount of Cur (N2) in NLC-Cur was then calculated. The loading capacity (LC%) was obtained from Equation (Equation1(1) ). The free Cur remained in the supernatant was detected and calculated as Nf, and the total Cur was Nt, so the encapsulation ratio (ER%) was obtained from Equation (Equation2
(2) )
(1)
(2)
Cell culture, cell viability and apoptosis analysis
Human brain cancer cell (A172) was grown in media as suggested by ATTC (Manassas, VA). The cell viability/growth response to Cur or NLC-Cur was measured using the 3-(4,5-dimethylthiazol-2-yl)-2, 5-diphenyl tetrazolium bromide (MTT) assay kit. To measure apoptosis and necrosis, A172 cells were treated with 20 µg/mL Cur or NLC-Cur for 24 h, harvested by trypsinization, stained with APC-conjugated Annexin V and Annexin V and 7-AAD (PI) as per the manufacturer's protocol and subjected to flow cytometry analysis. The ratios of apoptosis and necrosis were calculated by the software WinMDI 2.9 (Joseph Trotter). At meantime, cells treated with 20 µg/mL Cur or NLC-Cur for 24 h were stained with AO/EB following the protocol of the manufacturer as described previously (Mohankumar et al., Citation2014).
Intracellular reactive oxygen species
To test the reactive oxygen species (ROS) level, A172 cells were seeded in six-well plates and then treated with 20 µg/mL NLC-Cur or Cur in complete medium for 1 h. After treatment with the test drugs, cells were harvested and resuspended in PBS containing 10 μM DCFDA for 30 min at 37 °C and the fluorescence intensity was analyzed by FACS Caliber (BD Bioscience, Franklin Lakes, NJ).
Cellular uptake detection
Confocal microscopy and flow cytometry were used together to determine the cellular uptake of NCL-Cur. Cells were seeded at a seeding density of 1.5 × 105 cells/well and incubated at 37 °C for attachment. Then, the cells were treated with NCL-Cur (20 μg/mL) for 15 min, 1, 2 and 4 h in a cell culture incubator. After that, incubated cells were washed twice with PBS and cells were viewed and imaged under a confocal laser scanning microscope (Leica TCS SP5, Leica Microsystems GmbH, Solms, Germany). Fluorescence images were obtained at an excitation wavelength of 488 nm (cur).
Animal studies
Treatment of mice bearing human lung cancer xenografts
Five to six weeks old female nude mice (Taconics, Ncr nude) were maintained in a maximum isolation environment, according to an institutionally approved animal protocol. After 1 week’s acclimation, 5 × 106 A172 cells suspended in 100 µL sterile Dulbecco’s phosphate-buffered saline mixed with matrigel (2:1) were implanted subcutaneously into the flank of each mouse.
Nude mice bearing A172 xenografts were treated daily, 5 days/week, with PBS (control) intraperitoneally (i.p.), or NLC-Cur 150 mg/kg (i.p.) or Cur 150 mg/kg (i.p.) starting when the average tumor volume was about 180 mm3. Tumor volumes were measured every 3–4 days after drug injection.
Plasma concentration and tissue distribution
Five to six weeks old female nude mice bearing A172 xenografts were given NLC-Cur 150 mg/kg or Cur 150 mg/kg by intraperitoneal injection. The whole blood was get from heart of euthanized mice at different time points after the injection. At 1 h time point, each organs from euthanized mice, including heart, spleen, liver, intestine, lung, brain and tumor, were cut into small pieces and homogenized to get organ solutions. The protein concentrations of the homogenized organs were detected by a BCA protein assay kit. The Cur in each solution (100 µL) was extracted by acetonitrile (200 µL) and the concentration of Cur was detected by HPLC analysis. The concentrations of Cur in each organ was calculated according to the protein concentration.
Statistical analysis
Data are expressed as the mean ± SEM. Statistical analysis was performed by one-way ANOVA followed by Newman–Keuls test with GraphPad Prism 4.0 (Lajolla, CA). The level of statistical significance was defined as p < 0.05.
Results
Morphage and size distribution of NLC and NLC-Cur
The morphage and size distribution are detected by using TEM and PCS. As shown in , the mean size of NLC is 194.2 nm, and that of NLC-Cur is 214.0 nm. Both are round in shape, as shown in , with an average size of around 100 nm, which is much smaller than the size detected by PCS. The different results of size come from the reason that the size detected by PCS is the hydrodynamic size of the nanoparticles. And if two or more particles aggregate together, they would be considered and calculated as one, which lead to the doubled size as TEM detection. NLC-Cur is not as spherical as NLC, which might come from the drug loading of CUR. With the results of HPLC analysis, the encapsulation ratio is calculated as 88.6%, and the loading capacity is calculated as 27.4%.
The cytotoxicity of CUR and NCL-CUR on A172 cells
MTT assay is used here to evaluate the inhibitory effect of CUR and NCL-CUR on A172 cells. As shown in , the inhibitory effect of CUR is increased with the increase of drug concentration, and the IC50 is 80 µg/mL. The inhibition effect is significantly enhanced by the carrier of NLC, and the IC50 is less than 20 µg/mL. The inhibition ratio can reach as high as 90% when the concentration of NLC-Cur is 80 µg/mL. NLC itself do not have significant anticancer efficacy on A172 cells.
The apoptosis induction of Cur and NCL-Cur on A172 cells
The apoptosis induction effect is determined by the methods of AO/EB and Annexin V-APC/7-AAD. In AO/EB detection, cells with green fluorescence are living cells and cells with orange red fluorescence are apoptotic cells. As shown in , after the treatment, control cells turn on green fluorescence, which indicates that cells are in good condition, so do the cells treated with CUR(1) and NLC. Meanwhile, cells treated with CUR(2) and NLC-Cur(1) begin to turn on orange red fluorescence. In addition, in the plate of NLC-Cur(2) treatment, more than half cells turn on an orange red fluorescence, and the fluorescence is more bright and shows a circular uniform or a pyknosis and crumb structure.
Figure 3. Microscope photos of A172 cells treated with Cur, NCL-Cur and NLC stained with AO/EB: (1) means lower concentration of 10 µg/mL, (2) means higher concentration of 20 µg/mL, and doses are based on the amount of CUR.
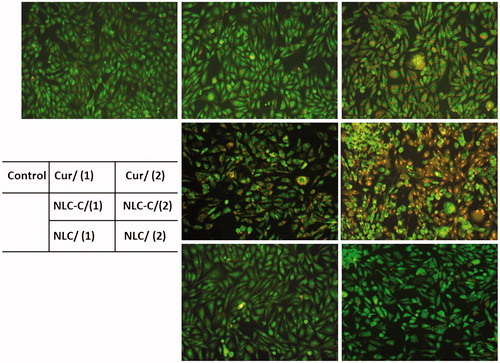
The Annexin V-APC/7-AAD further proves and qualified the result of AO/EB staining. As shown in , Q2 region presents the cells of late apoptosis and Q3 presents the cells of early apoptosis. The total ratio of early and late apoptotic cells is 24.7% when A172 cells is treated with NLC/Cur(2), which is nearly as triple as that of Cur(2).
The ROS level of A172 cells treated with Cur and NCL-Cur
We next explore the efficiency of Cur/NCL-Cur on cellular ROS levels in A172 cells by the analysis of flow cytometry using the redox-sensitive fluorescent probe 2′,7′-dichlorofluorescein diacetate (DCFDA). Cells are treated with different concentrations of Cur or NCL-Cur for 6 h before detection. Both Cur and NCL-Cur can cause a marked increase in ROS levels at 20 µg/mL, and only NCL-Cur enhanced ROS level at 10 µg/mL. NLC-Cur enhanced ROS level to 2.6-folds as control. NLC itself do not have function on the ROS level of A172 cells ().
The time-dependent cellular uptake
The uptake time is an essential factor in the efficiency of the cellular uptake of nanoparticles, which not only increase cellular uptake but also promote the intracellular lysosome proton sponge effect over time (Durazo & Kompella, Citation2012; Heller et al., Citation2012) Generally, when the nanoparticles first touch the cell membrane, the cell membrane proceeds with the production of surface molecules to package the nanoparticles, thereby enabling the nanoparticles to enter the cells. With the increase of exposure time between the nanoparticles and cellular membrane, the cellular uptake of nanoparticles also increases accordingly. As shown in , NLC-Cur enters A172 cells at the beginning 15 min. The increasing fluorescence intensity in 60 min, 2 h and 4 h indicates the increasing cellular uptake. From the cellular morphology and fluorescence distribution, we can even surmise that NCL-Cur enter into and accumulate in the mitochondria and nuclei of A172 cells.
Pharmacokinetics – biodistribution
To further explore the advances of NLC-Cur, we undertake a detailed assessment of the pharmacokinetics of both Cur and NLC-Cur administered i.p. to wt BALB/c mice. As shown in , compared to Cur, NLC-Cur consistently generated higher blood levels of Cur whether administered i.p., and the half-time is prolonged from 3.1 to 5.7 h. According to AUC values, administration of Cur in NLC increased the blood levels of Cur by 6.4-fold. Then, the bio-distributions of Cur in heart, spleen, intestine, lung, liver, brain and tumor are examined in nude mice bearing A172 xenografts, as shown in . In agreement with differences in the profiles of blood levels, NLC-Cur generated much higher levels than Cur in the xenografts and all organs examined.
NLC-Cur inhibits the growth of human brain cancer xenografts
Nude mice bearing A172 xenografts are treated with Cur or NLC-Cur every day for 19 days, started when the average tumor volume is about 180 mm3. As shown in , NLC-Cur significantly inhibits the growth of these xenografts, regressing them during the first 13 days and retarding their growth thereafter. By day 19, compared to controls, NLC-Cur reduced tumor volume by 82.3% (p < 0.005). In contrast, Cur’s effect on xenograft growth is not significant.
Discussion
Our work establishes the incorporation of Cur into NLC to achieve higher bioavailability and better anticancer efficiency. The enhanced efficiency of NLC-Cur both in vitro and in vivo comes from a series of effects: (1) The increased ROS level in vitro that can induce the apoptosis of A172 cells; (2) The delivery of Cur into cells in a time-dependent way; (3) Significantly improved AUC level in plasma and prolonged half-time of Cur after the incorporation; (4) Brain tumor targeting effect of NLC to deliver much more drug to carcinoma.
NLC-Cur responsiveness to A172 cells is much higher than Cur, because NLC can enhance the apoptosis ratio and ROS level in cells and lead to the accumulation of NLC-Cur in cells and even cellular nuclei. Compared to Cur, NLC-Cur could efficiently increase the ROS level in cells at only 10 µg/mL. ROS is very essential in the anticancer treatment, and many drugs have function through ROS-mediated pathways (Choi et al. Citation2014; Lin et al., Citation2015; Stander et al., Citation2015). It is reported that Cur-induced apoptosis of human colon cancer colo 205 cells through the production of ROS (Su et al., Citation2006a,Citationb). In our work, NLC strengthens the effect of Cur on the production of ROS and enhanced the cytotoxicity of Cur on A172 cells. This kind of enhancement by NLC for anticancer agents is also revealed by other carcinoma researches, such as human prostate cancer (Bondì et al., Citation2007), lung cancer (Shao et al., Citation2015), breast cancer (Ng et al., Citation2015) and so on.
Cellular uptake is a key point for anticancer agents to kill carcinoma. Many agents fail to kill cancer because the pump out function of cancer cell lines. It is reported that NLC could diminish the pump out function and help agents achieve time depending cellular uptake (Zhang et al., Citation2014), which make NLC as a good carrier for many chemical agents. From confocal observation we can see the time depending cellular uptake of NLC-Cur, and we can see that the fluorescence intensity getting stronger and stronger, which consequently increased the induction of apoptosis and cytotoxicity of Cur on A172 cells.
The extremely low bio-availability and fast metabolism of Cur restricts its application in medicine. Many methods have been taken to work out this problem, among which nanocarrier has raised more and more attention. There are many nanodelivery systems reported in recent years, including PLGA (Mayol et al., Citation2015), polymer (Alizadeh et al., Citation2015), SLNs (Wang et al., Citation2013) and NLCs (Madane & Mahajan, Citation2014). Both SLNs and NLCs are reported as an ideal nanocarrier for helping passing through the BBB, and NLC appears to be superior to SLN with smaller particle size and higher drug loading (Gokce et al., Citation2012). In our work, we find that NLCs could largely enhanced the brain and tumor targeting effect for Cur, prolong the half-time, and increase the blood levels of Cur by 6.4-fold. The brain targeting effect of NLC is come from its lipid base, which is also determined in the delivery for CNS acting drugs, where 2.5-times better permeation is exhibited (Alam et al., Citation2013). The ameliorated pharmacokinetic after loading in NLCs ensures the enhanced efficiency of Cur in mice bearing with A172 xenografts with an inhibition ratio of 82.3% on tumor growth.
Conclusion
Our findings suggest that NLC is an effective drug delivery system for Cur, accounting for the enhanced therapeutic efficacy of Cur against human brain cancer both in vitro and in vivo. The NLC formulation not only improved the apoptotic induction effect of Cur, but was also brain and tumor targeting, which triggered carcinoma digression. Given the efficacy of NLC-Cur, we speculate that Cur, when appropriately formulated, is a promising agent for the treatment of brain cancer.
Declaration of interest
The authors report no declarations of interest. The work was supported by “‘Fundamental Research Funds for Central Universities” (No. 1508219065)
References
- Alam MI, Baboota S, Ahuja A, Ali M, et al. (2013). Intranasal infusion of nanostructured lipid carriers (NLC) containing CNS acting drug and estimation in brain and blood. Drug Deliv 20:247–51
- Alizadeh AM, Sadeghizadeh M, Najafi F, et al. (2015). Encapsulation of curcumin in diblock copolymer micelles for cancer therapy. Biomed Res Int 2015:824746
- Bandyopadhyay D. (2014). Farmer to pharmacist: curcumin as an anti-invasive and antimetastatic agent for the treatment of cancer. Front Chem 2:113. doi: http://dx.doi.org/10.3389/fchem.2014.00113
- Bondì ML, Craparo EF, Giammona G, et al. (2007). Nanostructured lipid carriers-containing anticancer compounds: preparation, characterization, and cytotoxicity studies. Drug Deliv 14:61–7
- Cairncross JG, Wang M, Jenkins RB, et al. (2014). Benefit from procarbazine, lomustine, and vincristine in oligodendroglial tumors is associated with mutation of IDH. J Clin Oncol 32:783–90
- Chen QY, Jiao DM, Wang LF, et al. (2015). Curcumin inhibits proliferation-migration of NSCLC by steering crosstalk between a Wnt signaling pathway and an adherens junction via EGR-1. Mol Biosyst 11:859–68
- Chiang IT, Liu YC, Hsu FT, et al. (2014). Curcumin synergistically enhances the radiosensitivity of human oral squamous cell carcinoma via suppression of radiation-induced NF-κB activity. Oncol Rep 31:1729–37
- Choi JY, Cho HJ, Hwang SG, et al. (2014). Podophyllotoxin acetate enhances γ-ionizing radiation-induced apoptotic cell death by stimulating the ROS/p38/caspase pathway. Biomed Pharmacother 70:111–18
- Durazo SA, Kompella UB. (2012). Functionalized nanosystems for targeted mitochondrial delivery. Mitochondrion 12:190–201
- El-Bahr SM. (2015). Effect of curcumin on hepatic antioxidant enzymes activities and gene expressions in rats intoxicated with aflatoxin B1. Phytother Res 29:134–40
- Gokce EH, Korkmaz E, Dellera E, et al. (2012). Resveratrol-loaded solid lipid nanoparticles versus nanostructured lipid carriers: evaluation of antioxidant potential for dermal applications. Int J Nanomedicine 7:1841–50
- Gong C, Wu Q, Wang Y, et al. (2013). A biodegradable hydrogel system containing curcumin encapsulated in micelles for cutaneous wound healing. Biomaterials 34:6377–87
- Guo Y, Shu L, Zhang C, et al. (2015). Curcumin inhibits anchorage-independent growth of HT29 human colon cancer cells by targeting epigenetic restoration of the tumor suppressor gene DLEC1. Biochem Pharmacol 94:69–78
- Heller A, Brockhoff G, Goepferich A. (2012). Targeting drugs to mitochondria. Eur J Pharm Biopharm 82:1–18
- Jia T, Zhang L, Duan Y, et al. (2014). The differential susceptibilities of MCF-7 and MDA-MB-231 cells to the cytotoxic effects of curcumin are associated with the PI3K/Akt-SKP2-Cip/Kips pathway. Cancer Cell Int 14:126. doi:10.1186/s12935-014-0126-4
- Lin H, Gao X, Chen G, et al. (2015). Indole-3-carbinol as inhibitors of glucocorticoid-induced apoptosis in osteoblastic cells through blocking ROS-mediated Nrf2 pathway. Biochem Biophys Res Commun 460:422–7
- Liu Q, Li J, Pu G, Zhang F, et al. (2015). Co-delivery of baicalein and doxorubicin by hyaluronic acid decorated nanostructured lipid carriers for breast cancer therapy. Drug Deliv 15:1–5
- Madane RG, Mahajan HS. (2014). Curcumin-loaded nanostructured lipid carriers (NLCs) for nasal administration: design, characterization, and in vivo study. Drug Deliv 4:1–9
- Mayol L, Serri C, Menale C, et al. (2015). Curcumin loaded PLGA-poloxamer blend nanoparticles induce cell cycle arrest in mesothelioma cells. Eur J Pharm Biopharm 93:37–45
- Mohankumar K, Pajaniradje S, Sridharan S, et al. (2014). Mechanism of apoptotic induction in human breast cancer cell, MCF-7, by an analog of curcumin in comparison with curcumin – an in vitro and in silico approach. Chem Biol Interact 210:51–63
- Ng WK, Saiful Yazan L, Yap LH, et al. (2015). Thymoquinone-loaded nanostructured lipid carrier exhibited cytotoxicity towards breast cancer cell lines (MDA-MB-231 and MCF-7) and cervical cancer cell lines (HeLa and SiHa). Biomed Res Int 2015:263131
- Park W, Amin AR, Chen ZG, Shin DM. (2013). New perspectives of curcumin in cancer prevention. Cancer Prev Res (Phila) 6:387–400
- Sarin H. (2010). Overcoming the challenges in the effective delivery of chemotherapies to CNS solid tumors. Ther Deliv 1:289–305
- Shanmugam MK, Rane G, Kanchi MM, et al. (2015). The multifaceted role of curcumin in cancer prevention and treatment. Molecules 20:2728–69
- Shao Z, Shao J, Tan B, et al. (2015). Targeted lung cancer therapy: preparation and optimization of transferrin-decorated nanostructured lipid carriers as novel nanomedicine for co-delivery of anticancer drugs and DNA. Int J Nanomedicine 10:1223–33
- Shelma R, Sharma CP. (2013). In vitro and in vivo evaluation of curcumin loaded lauroyl sulphated chitosan for enhancing oral bioavailability. Carbohydr Polym 95:441–8
- Stander XX, Stander BA, Joubert AM. (2015). Synergistic anticancer potential of dichloroacetate and estradiol analogue exerting their effect via ROS-JNK-Bcl-2-mediated signalling pathways. Cell Physiol Biochem 35:1499–526
- Su CC, Chen GW, Lin JG, et al. (2006a). Curcumin inhibits cell migration of human colon cancer colo 205 cells through the inhibition of nuclear factor kappa B/p65 and down-regulates cyclooxygenase-2 and matrix metalloproteinase-2 expressions. Anticancer Res 26:1281–8
- Su CC, Lin JG, Li TM, et al. (2006b). Curcumin-induced apoptosis of human colon cancer colo 205 cells through the production of ROS, Ca2+ and the activation of caspase-3. Anticancer Res 26:4379–89
- Van Tellingen O, Yetkin-Arik B, de Gooijer MC, et al. (2015). Overcoming the blood-brain tumor barrier for effective glioblastoma treatment. Drug Resist Update 19:1–12
- Vecchi Brumatti L, Marcuzzi A, Tricarico PM, et al. (2014). Curcumin and inflammatory bowel disease: potential and limits of innovative treatments. Molecules 19:21127–53
- Venur VA, Peereboom DM, Ahluwalia MS. (2015). Current medical treatment of glioblastoma. Cancer Treat Res 163:103–15
- Wang X, Wang Q, Ives KL, Evers BM. (2006). Curcumin inhibits neurotensin-mediated interleukin-8 production and migration of HCT116 human colon cancer cells. Clin Cancer Res 12:5346–55
- Wang P, Zhang L, Peng H, et al. (2013). The formulation and delivery of curcumin with solid lipid nanoparticles for the treatment of on non-small cell lung cancer both in vitro and in vivo. Mater Sci Eng C Mater Biol Appl 33:4802–8
- Zhang W, Liu J, Zhang Q, et al. (2014). Enhanced cellular uptake and anti-proliferating effect of chitosan hydrochlorides modified genistein loaded NLC on human lens epithelial cells. Int J Pharm 471:118–26