Abstract
One of the significant problems in vaccination projects is the lack of an effective vaccine against hepatitis C virus (HCV). The goal of the current study is to evaluate and compare two DNA constructs encoding HCV core and coreE1E2 genes alone or complexed with MPG peptide as a delivery system for stimulation of antibody responses and IFN-γ secretion in Balb/c mice model. Indeed, MPG cell penetrating peptide was used to improve DNA immunization in mice. Our results demonstrated that MPG forms stable non-covalent nanoparticles with pcDNA-core and pcDNA-coreE1E2 at an N/P ratio of 10:1. The in vitro transfection efficiency of core or coreE1E2 DNA using MPG and TurboFect delivery systems was confirmed by western blot analysis. The results indicated the expression of the full-length core (∼21 kDa), and coreE1E2 (∼83 kDa) proteins using an anti-His monoclonal antibody. In addition, the expression of HCV core and coreE1E2 proteins was performed in bacteria and the purified recombinant proteins were injected to mice with Montanide 720 adjuvant. Our data showed that the immunized mice with HCV core and coreE1E2 proteins generated the mixture of sera IgG1 and IgG2a isotypes considerably higher than other groups. Furthermore, DNA constructs encoding core and coreE1E2 complexed with MPG could significantly induce IFN-γ secretion in lower concentrations than the naked core and coreE1E2 DNAs. Taken together, the DNA formulations as well as protein regimens used in this study triggered high-level IFN-γ production in mice, an important feature for the development of Th1 immune responses.
Introduction
More than 170 million people are chronically infected with hepatitis C virus (HCV), and there are 3–4 million new HCV infections annually worldwide, but no vaccine is available (Torresi et al., Citation2011; Swadling et al., Citation2013; Gummow et al., Citation2015). The HCV infection may lead to immune system dysfunction, especially for the development of Th1 immune responses that are required to clear virus-infected cells (Ma et al., Citation2002). The HCV polyprotein is post-translationally cleaved into three structural (i.e. Core, E1 and E2) and seven non-structural (i.e. NS2, NS3, NS4A, NS4B, NS5A, NS5B and P7) proteins and these proteins play important roles in virus entry, replication, assembly and pathogenesis (Yousaf et al., Citation2011; Chandler et al., Citation2012). Core protein contains first 191 amino acids and can be divided into three domains: Domain 1 (amino acids 1–117), Domain 2 (amino acids 118–174) and Domain 3 (amino acids 175–191). Domains 2 and 3 are hydrophobic (Ma et al., Citation2002). The nucleocapsid (Core) protein is a multifunctional protein and highly conserved among various HCV genotypes, which is involved in many viral and cellular processes. It is an attractive target for HCV vaccine (Alekseeva et al., Citation2009). Two envelop proteins (E1 and E2) are highly glycosylated and play an important role in cell entry (Sharma, Citation2010; Keyvani et al., Citation2012). The envelope glycoproteins are present as large disulphide linked oligomers on the surfaces of HCV particles (Sharma, Citation2010; Verstrepen et al., Citation2015). Envelope proteins are considered important in the HCV vaccine design due to their role in virus–host recognition, immune selection and viral neutralization (Ma et al., Citation2002). Among different vaccination strategies, DNA- and protein-based vaccinations have attracted a special interest for prevention or treatment of HCV infections (Ma et al., Citation2002). The enhanced T-cell immune responses are necessary for effective clearance of the virus and DNA vaccines result in a cell-mediated bias (Dueñas-Carrera, Citation2004; Guan et al., Citation2015; Gummow et al., Citation2015). DNA vaccination can induce both humoral and cellular immune responses, but the cellular uptake of naked DNA injected into muscle is not very efficient, thus the DNA is degraded by interstitial nucleases before the nucleus entry for transcription (Jiao et al., Citation2003). For this reason, several delivery systems were designed to develop targeted delivery of DNA vaccines to antigen presenting cells (APCs) (Karpenko et al., Citation2004; Liu et al., Citation2013). In recent years, cell penetrating peptides (CPPs) have been suggested as one of the most efficient delivery systems to protect and nuclear entry of nucleic acids. These peptides contain less than 30 amino acids and possess cationic and hydrophobic residues that facilitate interaction of DNA with the cell surface (Laufer & Restle, Citation2008; Morris et al., Citation2008; Bolhassani, Citation2011). Among them, MPG peptide was known as a potent delivery system (Morris et al., Citation1997, Citation1999). In addition, the use of biotechnology for generation of the recombinant proteins especially structural proteins could be considered as an important strategy in stimulation of immune responses. Many studies have produced HCV core or HCV core-E1E2 proteins in different expression systems, such as bacteria (Linbai et al., Citation1996; Hemmat et al., Citation2011), insects (Baumert et al., Citation1998; Choi et al., Citation2004; Yazdani-Neyshabouri et al., Citation2012), and yeast (Donato et al., Citation2006; Fazlalipour et al., Citation2014). Usually, the truncated fragments of core, E1 or E2 were produced in bacterial systems (Aghasadeghi et al., Citation2006). However, development of adjuvants along with better HCV immunogens will be needed for a successful HCV vaccine.
In this study, we developed the production of the full length HCV core and also core-E1E2 protein using bacterial expression system and reverse staining method. Montanide adjuvant was used to enhance the potency of core or coreE1E2 protein regimens in mice immunization. In addition, in vitro delivery of plasmid DNA encoding HCV core and coreE1E2 genes was performed using MPG peptide to determine the core and coreE1E2 expression. Then, the data obtained after mice immunization with naked or formulated plasmid DNA encoding HCV structural proteins were evaluated.
Materials and methods
Preparation of DNA constructs
The sequences of HCV core and core-E1E2 genes were obtained from GenBank (Accession number: AB047640). For the generation of core or coreE1E2-expressing plasmids (pcDNA-core or pcDNA-coreE1E2), the core or coreE1E2 genes were individually subcloned from pET-core and pQE-coreE1E2 (previously provided in Department of Hepatitis and AIDS, Pasteur Institute of Iran) into the BamHI/HindIII cloning sites of pcDNA 3.1 (−) expression vector (Invitrogen, Carlsbad, CA). It should be noted that core and coreE1E2 cloned in pET 28a and pQE30 consisted of His-tag in C-terminal regions for evaluating in vitro expression. DNA constructs containing core and coreE1E2 were purified in large-scale using Endotoxin-free Giga-kit (Qiagen, Germany). The presence of the inserted genes was confirmed by PCR and restriction enzyme digestion as detected on gel electrophoresis. Finally, DNA concentration was determined by the absorbance measured at 260 nm. The accuracy of these constructs was confirmed by DNA sequencing.
Expression of core and coreE1E2 proteins in Escherichia coli
For generation of the recombinant core and coreE1E2 proteins, the bacterial cultures containing pET-core [BL21 (DE3) strain], and pQE-coreE1E2 [M15 strain] were grown to an optical density of 0.7–0.8 at 600 nm and protein expression was induced with 1 mM IPTG for 3 h at 37 °C. Protein samples were analyzed by SDS-PAGE in 12.5% (W/V) polyacrylamide gel. The recombinant core and coreE1E2 proteins were purified by an imidazole-SDS-Zn reverse staining method (Mirnurollahi et al., Citation2015). Protein concentration was measured using BCA assay kit (Pierce, Rockford, IL) and spectrophotometer, and kept at −70 °C prior to use.
Formation of MPG/pcDNA-core and MPG/pcDNA-coreE1E2 nanoparticles
The MPG peptide (GALFLGFLGAAGSTMGAWSQPKKKRKV: Morris et al., Citation1997) was synthesized by Biomatik Corporation (Cambridge, Ontario, Canada). The synthetic MPG peptide was dissolved in water at 2 mg/ml as a stock solution. The plasmid DNAs [pcDNA-core or pcDNA-coreE1E2] were mixed with MPG at N/P ratios of 1:1, 2:1, 5:1, 10:1 and 15:1 in PBS (pH 7.4), and incubated for 1 h at room temperature. The formation of peptide-DNA complex was assessed by agarose gel retardation assay. The size and zeta-potential of the MPG/DNA complexes were assessed by a Zetasizer Nano ZS instrument. Moreover, the size and morphology of MPG/DNA nanoparticles were analyzed at an N/P ratio of 10:1 with an atomic force microscopy (AFM, Kev80).
Stability and cytotoxicity of MPG/DNA nanoparticles
To determine the stability of MPG/DNA complexes against DNA nucleases, DNase I was added to the complexes at N/P ratio of 10:1 with a final concentration of 1.37 U enzyme per 1 μg DNA and the mixtures were incubated at 37 °C for 1 h followed by the addition of stop solution (200 mM sodium chloride, 20 mM EDTA and 1% SDS). In addition, to evaluate the serum stability, the nanoparticles at an N/P ratio of 10:1 were exposed to 10% serum and incubated for 5 h at 37 °C. Then, DNA plasmids were released from peptide by adding 10% SDS solution for 2 h and analyzed with electrophoresis on agarose gel. Finally, the cytotoxicity of MPG/pcDNA-core and MPG/pcDNA-coreE1E2 complexes was assessed at N/P ratio of 10:1 in HEK 293 T cell line using the MTT assay (Sadeghian et al., Citation2012).
In vitro expression of core and coreE1E2 proteins in HEK-293 cells
The pcDNA harboring the core or fused coreE1E2 genes [pcDNA-core and pcDNA-coreE1E2] were prepared in large scale with high purity. Human HEK-293 cells were maintained in complete RPMI (Sigma, Germany) medium supplemented with 10% fetal calf serum (FCS, Gibco, Germany) at 37 °C and 5% CO2 atmosphere. Then, the cells were seeded at a density of 2 × 105 cells/well into a 12-well plate and transfected using TurboFect cationic polymer (2 μg DNA: 4 μl TurboFect, Fermentas, Germany), and MPG (N/P ratio = 10:1) as in vitro transfection reagents. HEK-293 cells were used as a negative control. The medium was replaced after 6 h incubation at 37 °C with RPMI with 10% FCS. The cells were harvested 48 h post-transfection, washed and resuspended in PBS. The quality of protein expression was detected by western blotting. In summary, the proteins were separated on a 12% separating gel and transferred to nitrocellulose membrane electrophoretically. The recombinant proteins were identified by using anti-His monoclonal antibody (ab18184, 1:10 000, Abcam) as primary antibody and anti-mouse IgG-HRP (A9044, 1:10 000, Sigma) as secondary antibody. The immunoreactive protein bands were visualized using peroxidase substrate named 3,3′-diaminobenzidine (DAB, Sigma).
Mice immunization
Inbred Balb/c female mice, 6–8-week old were obtained from the breeding stocks maintained at the Pasteur Institute of Iran. All animal procedures were performed according to approved protocols and in accordance with the recommendations for the proper use and care of laboratory animals. Ten groups of six mice were selected. For priming, test groups were immunized subcutaneously (s.c.) at the footpad with DNA constructs expressing core or coreE1E2 as the naked (100 μg, G1 and G3) or formulated by MPG peptide (N/P ratio of 10:1, 10 μg, G2 and G4) and also the core or coreE1E2 recombinant proteins emulsified by Montanide ISA720 (M720) (10 μg, Oil:aqueous phase ratio of 7:3, G5 and G6). The control groups (G7-G10) were injected with MPG, pcDNA3.1, Montanide and PBS, respectively. For boosting, all groups received the regimens similar to priming. shows the regimens of each group.
Table 1. Mice immunization using core or coreE1E2 constructs in different formulations.
Monitoring humoral immune response
Mice sera were collected from all groups 3 weeks after the last injection. Pooled sera for each group were stored at −20 °C. IgG1 and IgG2a isotypes (1:1000, Southern Biotechnology Association, Birmingham, AL) were measured using indirect ELISA against the recombinant core (10 μg/ml), coreE1E2 (10 μg/ml) proteins as coating antigens. The pooled mice sera were diluted 1:50 in 1% BSA/PBS-Tween (Bolhassani et al., Citation2008).
Cytokine assay
To further characterize the immune responses to different immunization strategies, the levels of IFN-γ and IL-4 secretion in mice splenocytes were determined three weeks after final boosting. Four mice from each group were sacrificed three weeks after immunization and spleens were homogenized. After lysis using ACK buffer, splenocytes were washed and re-suspended in complete RPMI medium. Cells were then seeded at a density of 2 × 106 cells/ml in the presence of 10 μg/ml core and coreE1E2 proteins as a recall antigen and medium alone (RPMI with 10% FCS). Concanavalin A (ConA: 5 μg/ml) was also used as the positive control. Plates were incubated for 3 days at 37 °C in 5% CO2 humidified atmosphere. Culture supernatants from stimulated splenocytes under the same conditions were collected in triplicate and assayed for IFN-γ and IL-4 by a sandwich ELISA (R & D, Minneapolis, MN), according to the manufacturer’s instructions. The lower detection limits of IFN-γ and IL-4 were 2 and 7 pg/ml, respectively.
Statistical analysis
Statistical analysis was performed using Prism 5.0 software (GraphPad, San Diego, CA). One-way ANOVA and Student’s t-test were performed to analyze immune responses. For all analyses, p < 0.05 was considered statistically significant. Data are presented as mean ± standard deviation (SD).
Results
Recombinant HCV core or coreE1E2 protein expression and purification
The core and coreE1E2 proteins used in immunization were produced in E. coli. The expression of poly-histidine tagged core and coreE1E2 was induced by IPTG and purified by reverse staining method. A ∼21 kDa band for core protein and ∼83 kDa for core-E1E2 protein were revealed in SDS-PAGE (data not shown). The purified poly-histidine tagged core and coreE1E2 proteins were dialyzed into phosphate-buffered saline before it was used in mouse immunization.
Confirmation of MPG/core DNA or MPG/coreE1E2 DNA nanoparticles
The MPG/DNA nanoparticles were generated using interaction of the negatively charged pcDNA-core/coreE1E2 with the cationic MPG peptide. The increasing amounts of MPG showed a dose-dependent change in the electrophoretic mobility of the plasmids. As indicated in DNA retardation assay (), the DNA molecules did not migrate into the agarose gel at N/P ratio of 10:1, indicating the formation of the MPG/core or coreE1E2 DNA complexes. Indeed, MPG peptide condensed pcDNA-core and pcDNA-coreE1E2 into small nanoparticles in similar N/P ratio (10:1). Furthermore, the nanoparticle size and positive charge were obtained at an N/P ratio of 10:1 (∼180–200 nm for core and 300–400 nm for coreE1E2) and could be selected for in vitro and in vivo delivery of pcDNA-core and pcDNA-coreE1E2. AFM analysis of nanoparticles at an N/P ratio of 10:1 showed a spherical shape with a narrow size distribution (). The stability of MPG-based nanoparticles was determined in presence of DNase and serum. After DNase I treatment, the naked DNA was degraded, while the MPG/DNA complexes at the N/P ratio of 10:1 protected the pcDNA-core and pcDNA-coreE1E2 (). Moreover, our data showed that unprotected plasmid DNA was degraded in the presence of serum after 5 h incubation with FCS. In contrast, recovered DNA from nanoparticles remained intact in agarose gel electrophoresis (). MTT results indicated that MPG peptide in a concentration of 10 μg and MPG/pcDNA-core or MPG/pcDNA-coreE1E2 nanoparticles at the N/P ratio of 10:1 are not cytotoxic for in vitro gene delivery and in vivo immunization (data not shown).
Figure 1. Physicochemical properties of the MPG/DNAs complexes: (A) Representative gel retardation assay of MPG peptide complexed with pcDNA-core and pcDNA-coreE1E2 at different N/P ratios; Lane 1: naked plasmid DNA as a control (pcDNA-core), Lane 2: N/P = 1:1, Lane 3: N/P = 2:1, Lane 4: N/P = 5:1, Lane 5: N/P = 10:1, Lane 6: N/P = 15:1 for pcDNA-core, Lane 7: naked plasmid DNA as a control (pcDNA-coreE1E2), Lane 8: N/P = 1:1, Lane 9: N/P = 2:1, Lane 10: N/P = 5:1, Lane 11: N/P = 10:1, Lane 12: N/P = 15:1 for pcDNA-coreE1E2. The DNA complexed with MPG that was not able to migrate into the gels was observed at an N/P ratio of 10:1; (B) The AFM micrograph of the spherical nanoparticles formed at N/P = 10:1 for pcDNA-core and pcDNA-coreE1E2: the nanoparticle was shown in circle; (C) Stability analysis of MPG-based core or coreE1E2 nanoparticles against DNase I. Lane 1: naked plasmid DNA with DNase, Lane 2: N/P = 10:1; (D) Stability analysis of MPG-based core or coreE1E2 nanoparticles against serum: Lane 1: naked plasmid DNAs as a control, Lane 2: N/P = 10:1.
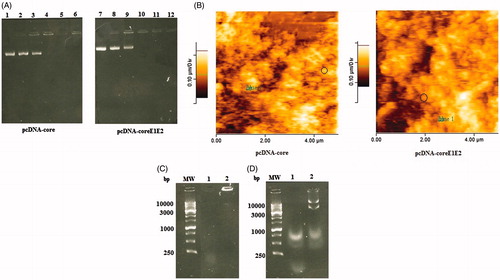
In vitro assay of cell transfection using nanoparticles
The efficiency of core and coreE1E2 gene expression was assessed in HEK 293 T cell line using turbofect, and MPG peptide delivery systems. Western blot analysis showed a dominant band of 21 kDa in transfected cells expressing core and also a clear band of 83 kDa in transfected cells expressing coreE1E2 that they were not detected in the untransfected cells ().
Figure 2. Western blot analysis using an anti-His monoclonal antibody showed the expression of the full-length core (Lane 1 and 2, ∼21 kDa), and coreE1E2 (Lane 1 and 2, ∼83 kDa) proteins using TurboFect and MPG, respectively. Any detectable band was not observed in untransfected cells as a negative control (Lane 3).
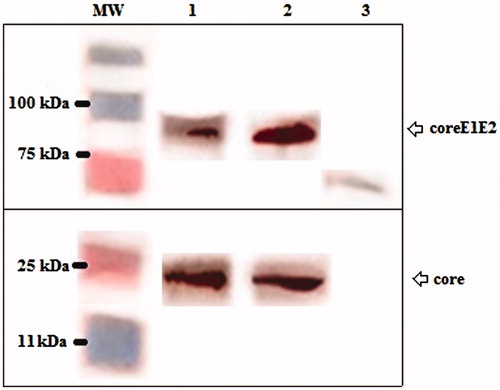
Antibody responses to different HCV core and coreE1E2 modalities in mice
The core/coreE1E2-specific IgG1 and IgG2a responses were evaluated from the mice sera by ELISA, three weeks after the last immunization. As shown in , the DNA constructs expressing HCV core or coreE1E2 proteins elicited the mixture of IgG1 and IgG2a responses significantly higher than the control groups (p < 0.05). These responses were further increased by mice immunization with the recombinant core or coreE1E2 proteins (G5 and G6) against DNAs-based immunizations (G1–G4, p < 0.05), indicating a mixture of Th1 and Th2-biased responses. Indeed, mice immunization with the naked DNAs and the DNAs formulated with MPG induced lower antibody levels than core or coreE1E2 proteins-based immunization (p < 0.05). It seems that formulation of the recombinant core and coreE1E2 proteins with Montanide affects the elevated IgG1 and IgG2a levels. In addition, the same immune responses were observed between the naked DNA-based immunizations (G1 and G2) and the MPG/DNA nanoparticles (G3 and G4), however, MPG could stimulate IgG1 and IgG2a levels in lower DNA concentrations compared to high concentrations of the naked core or coreE1E2 DNA constructs.
Figure 3. Analysis of IgG2a (A) and IgG1 (B) antibody levels with respect to the coated antigens (the recombinant core and coreE1E2 proteins) using ELISA at 3 weeks after third immunization. Data show antibody responses of pooled mice sera with independent experiments, and error bars indicate mean ± SD; (*p < 0.05).
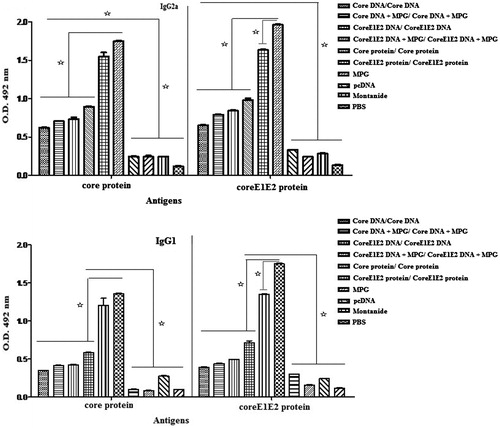
Immunization of Balb/c mice with DNA constructs formulated with MPG-induced strong HCV-specific cellular immune responses compared to the naked DNA constructs
Mice immunization with the recombinant core or coreE1E2 proteins as well as the DNA constructs expressing core and coreE1E2 (G1–G6) effectively enhanced the IFN-γ levels compared to the control groups (p < 0.05, ). Moreover, the levels of IFN-γ with respect to core and coreE1E2 antigens were significantly higher in groups injected with DNA/MPG (G2 and G4) compared to the naked DNAs (G1 and G3; p < 0.05). On the other hand, significant immune responses in the form of core- and coreE1E2-specific IFN-γ secretions were detected after a HCV coreE1E2 protein administration as compared to HCV core protein immunization. The results confirmed a significant high level of IFN-γ between groups with respect to both core and coreE1E2 antigens as following:
Figure 4. Analysis of IFN-γ secretion in mice immunized by core and coreE1E2 in different formulations at 3 weeks after booster: all assays were performed in triplicate. Error bars indicate mean ± SD detected in pg/ml. Mice immunization with protein-based immunizations and then the DNAs formulated by MPG-induced high HCV-specific cellular immune responses; (*p < 0.05).
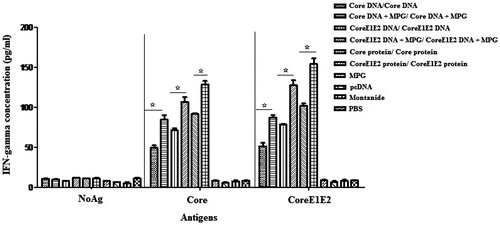
There was no significant difference for IL-4 production in different groups (p > 0.05, data not shown).
Discussion
One strategy for vaccine development is the use of DNA technology, which has the potential to generate strong humoral and cellular immune responses (Guan et al., Citation2015; Gummow et al., Citation2015). Hepatitis C core protein is a highly immunogenic natural infection, but it seems to have low immunogenicity in experimental studies (Alekseeva et al., Citation2009). A study showed that the plasmids (e.g. pCMVcore, pCMVcoreIRES and pCMVcoreKozak) differ in core-expression capacity and consequently in stimulation of immune responses. Herein, mice immunization with highly expressing pCMVcoreKozak induced specific IFN-γ, but poor antibody response. The best strategy was the heterologous pCMVcoreKozak prime/core protein boost regimen that generated the mixed Th1/Th2-cellular response with core-specific antibodies. In fact, administration of highly expressed HCV core gene may inhibit core-specific immune response; while a heterologous DNA prime/protein boost regimen averted the negative effects of intracellular core expression (Alekseeva et al., Citation2009). In the current study, to describe if immune responses could be influenced by core and also coreE1E2 expression levels, Balb/c mice were immunized two times with the pcDNA plasmids expressing HCV core and also coreE1E2 as the naked DNA and also DNA complexed with MPG. The induction of IFN-γ responses against core- and coreE1E2 antigens was stronger in mice immunized with coreE1E2/MPG and then core/MPG nanoparticles as compared to coreE1E2 and core DNA constructs. This cell-mediated immune response (Th1-biased response) was higher in groups immunized with the recombinant coreE1E2 and core proteins than other groups. The highest anti-core or anti-coreE1E2 IgG1 and IgG2a responses were raised in mice immunized with the recombinant coreE1E2 and core proteins as compared to DNA-immunized mice. The DNA constructs could significantly generate a mixture of IgG1 and IgG2a responses against control groups. However, low concentrations of DNA complexed with MPG could considerably elicit IFN-gamma responses compared to high concentrations of the naked core or coreE1E2 DNA constructs. Indeed, core or coreE1E2-specific antibody responses could be enhanced by CpG motif of the plasmid backbone. A study indicated that intramuscular delivery of HCV E2-expressing DNA vaccine to mice-induced robust antibody and CTL responses if the vector is designed, so that antigen is secreted or membrane-bound rather than it remains intracellular. Furthermore, the use of DNA vectors optimized for their CpG content could further augment the humoral responses (Ma et al., Citation2002). On the other hand, core or coreE1E2-specific IL-4 secretions were not detected in this experiment. It should be noted that the DNA immunogens used (core or coreE1E2) encode not only a structural component of the virus, but also a pathogenic factor, which can interact with a broad range of cellular proteins and influence various host cell functions. Indeed, the immune response in DNA immunization is determined by the dose of gene and by the gene capacity to direct an efficient antigen expression (Alekseeva et al., Citation2009). Up to now, several DNA constructs have been designed against HCV infections. For instance, the first DNA vaccine was evaluated in Cuba in Phase I trial. It is the combination of plasmid expressing HCV core/E1/E2 with the recombinant core protein. On the other hand, some recombinant proteins are enough to induce immune response but they require adjuvant therapy. For example, the first preventive vaccine candidate containing the envelope E1/E2 glycoproteins in an oil water adjuvant MF59 along with a CpG oligonucleotide developed neutralizing antibodies and T-cell lymphocyte proliferation. Furthermore, the first candidate therapeutic vaccine was a recombinant HCV E1 protein in alum adjuvant, which was administered to humans and induced HCV-specific antibody and T-cell responses in patients (Halliday et al., Citation2011; Keyvani et al., Citation2012; Gorzin et al., Citation2014). In summary, the ability to induce strong Th1-type immune responses was obtained using two different strategies: DNA- and protein-based immunizations. Our results indicated that core and coreE1E2 protein immunizations three times in mice, can stimulate high IgG isotypes and IFN-γ as compared to the DNA constructs. In addition, MPG could increase IFN-γ secretion in DNA immunization (two times, 10 μg) significantly higher than the naked DNA (100 μg). Indeed, we could improve the DNA constructs expressing core and coreE1E2 using an efficient delivery system (MPG), a suitable vector with strong promoter and CpG motif as well as optimization of injection dose and number. On the other hand, the recombinant core and coreE1E2 proteins could elicit robust humoral and cellular immune responses using Montanide adjuvant. In this study, for the first time, we simultaneously evaluated the potency of MPG cell penetrating peptide and Montanide 720 for improving DNA and protein immunizations, respectively. In addition, we compared the efficiency of DNA and protein-based immunizations. However, the MPG-mediated gene delivery as well as the recombinant protein emulsified in adjuvant is a feasible HCV vaccine strategy that should be further tested after challenge in the small and large animal models. This study may allow the development of a prophylactic or therapeutic HCV vaccine directed to Th1 immune response.
Declaration of interest
The authors report no conflicts of interest.
References
- Aghasadeghi MR, Sadat SM, Budkowska A, et al. (2006). Evaluation of a native preparation of HCV core protein (2-122) for potential applications in immunization, diagnosis and mAb production. Iranian J Publ Health 35:1–10
- Alekseeva E, Sominskaya I, Skrastina D, et al. (2009). Enhancement of the expression of HCV core gene does not enhance core-specific immune response in DNA immunization: advantages of the heterologous DNA prime, protein boost immunization regimen. Genet Vaccines Ther 7:1–7
- Baumert TF, Ito S, Wong DT, Liang TJ. (1998). Hepatitis C virus structural proteins assemble into virus like particles in insect cells. Virol 72:3827–36
- Bolhassani A. (2011). Potential efficacy of cell-penetrating peptides for nucleic acid and drug delivery in cancer. Biochim Biophys Acta Rev Cancer 1816:232–46
- Bolhassani A, Zahedifard F, Taghikhani M, Rafati S. (2008). Enhanced immunogenicity of HPV16E7 accompanied by Gp96 as an adjuvant in two vaccination strategies. Vaccine 26:3362–70
- Chandler DE, Penin F, Schulten K, Chipot C. (2012). The p7 protein of Hepatitis C virus forms structurally plastic, minimalist ion channels. PLoS Comput Biol 8:e1002702. doi:10.1371/journal.pcbi.1002702
- Choi SH, Kim SY, Park KJ, et al. (2004). Hepatitis C virus core protein is efficiently released into the culture medium in insect cells. J Biochem Molec Biol 37:735–40
- Donato GM, Rivero NA, Grillo JM, et al. (2006). Expression and processing of hepatitis C virus structural proteins in Pichia pastoris yeast. Biochem Biophys Res Commun 342:625–31
- Dueñas-Carrera S. (2004). DNA vaccination against hepatitis C. Curr Opin Mol Ther 6:146–50
- Fazlalipour M, Keyvani H, Monavari SHR, Mollaie HR. (2014). Recombinant core-E1E2 protein expressed in Pichia pastoris yeast, a candidate vaccine for hepatitis C virus. J Antivir Antiretrovir 6:139–47
- Gorzin Z, Gorzin AA, Tabarraei A, et al. (2014). Immunogenicity evaluation of a DNA vaccine expressing the Hepatitis C virus non-structural protein 2 gene in C57BL/6 mice. Iran Biomed J 18:1–7
- Guan J, Deng Y, Chen H, et al. (2015). Priming with two DNA vaccines expressing hepatitis C virus NS3 protein targeting dendritic cells elicits superior heterologous protective potential in mice. Arch Virol 160:2517–24
- Gummow J, Li Y, Yu W, et al. (2015). A multi-antigenic DNA vaccine that induces broad HCV-specific T-cell responses in mice. J Virol 89:1–8
- Halliday J, Klenerman P, Barnes E. (2011). Vaccination for Hepatitis C virus: closing in on an evasive target. Expert Rev Vaccines 10:659–72
- Hemmat J, Yakhchali B, Khajeh KH, et al. (2011). Over-expression of full-length core protein of hepatitis C virus by Escherichia coli cultivated in stirred tank fermentor. Iran J Biotechnol 9:245–52
- Jiao X, Wang RYH, Feng Z, et al. (2003). Modulation of cellular immune response against hepatitis C virus nonstructural protein 3 by cationic liposome encapsulated DNA immunization. Hepatology 37:452–60
- Karpenko LI, Nekrasova NA, Ilyichev AA, et al. (2004). Comparative analysis using a mouse model of the immunogenicity of artificial VLP and attenuated Salmonella strain carrying a DNA-vaccine encoding HIV-1 polyepitope CTL-immunogen. Vaccine 22:1692–9
- Keyvani H, Fazlalipour M, Monavari SHR, Mollaie HR. (2012). Hepatitis C virus-proteins, diagnosis, treatment and new approaches for vaccine development. Asian Pacific J Cancer Prev 13:5917–35
- Laufer S, Restle T. (2008). Peptide-mediated cellular delivery of oligonucleotide-based therapeutics in vitro: quantitative evaluation of overall efficacy employing easy to handle reporter systems. Curr Pharm Des 14:3637–55
- Linbai Y, Jinrong G, Xiaolin M, et al. (1996). Expression of E1 gene of a hepatitis C virus in E. coli and protein purification. Wuhan Univ J Natural Sci 1:279–82
- Liu Y, Li F, Qi Z, et al. (2013). The effects of HIV Tat DNA on regulating the immune response of HIV DNA vaccine in mice. Virol J 10:1–11
- Ma X, Forns X, Gutierrez R, et al. (2002). DNA-based vaccination against hepatitis C virus (HCV): effect of expressing different forms of HCV E2 protein and use of CpG-optimized vectors in mice. Vaccine 20:3263–71
- Mirnurollahi SM, Bolhassani A, Irani S, Davoudi N. (2015). Expression and purification of HCV core and core-E1E2 proteins in different bacterial strains. Iran J Biotech 13:e1249
- Morris MC, Deshayes S, Heitz F, Divita G. (2008). Cell-penetrating peptides: from molecular mechanisms to therapeutics. Biol Cell 100:201–17
- Morris M, Vidal P, Chaloin L, et al. (1997). A new peptide vector for efficient delivery of oligonucleotides into mammalian cells. Nucleic Acids Res 25:2730–6
- Morris MC, Chaloin L, Méry J, et al. (1999). A novel potent strategy for gene delivery using a single peptide vector as a carrier. Nucleic Acids Res 27:3510–17
- Sadeghian F, Hosseinkhani S, Alizadeh A, Hatefi A. (2012). Design, engineering and preparation of a multi-domain fusion vector for gene delivery. Int J Pharm 427:393–9
- Sharma SD. (2010). Hepatitis C virus: molecular biology & current therapeutic options. Indian J Med Res 131:17–34
- Swadling L, Klenerman P, Barnes E. (2013). Ever closer to a prophylactic vaccine for HCV. Expert Opin Biol Ther 13:1109–24
- Torresi J, Johnson D, Wedemeyer H. (2011). Progress in the development of preventive and therapeutic vaccines for hepatitis C virus. J Hepatol 54:1273–85
- Verstrepen BE, Boonstra A, Koopman G. (2015). Immune mechanisms of vaccine induced protection against chronic hepatitis C virus infection in chimpanzees. World J Hepatol 7:53–69
- Yazdani-Neyshabouri SH, Aghasadeghi MR, Jahanian Najafabadi A, et al. (2012). Expression of recombinant hepatitis C virus (HCV) Core, E1 and E2 proteins by the baculovirus expression vector system. African J Microbiol Res 6:4152–7
- Yousaf MZ, Idrees M, Saleem Z, et al. (2011). Expression of core antigen of HCV genotype 3a and its evaluation as screening agent for HCV infection in Pakistan. Virology 8:364. doi:10.1186/1743-422X-8-364