Abstract
Complexes with a composition of VCl2-n(bzac)2(HL1,2)n (I – IV) (where bzac = 1-phenyl-1,3-butanedionate anion (C6H5COCHCOCH3−); HL1=C6H5OCH2C(O)NHO−, HL2 = C6H5CH=CHC(O)NHO−, n = 1 and 2) have been synthesised from the reactions of (VCl2(bzac)2) with equi- and bi-molar amounts of potassium phenoxyacetohydroxamate and cinnamohydroxamate (KHL1,2) in THF + MeOH solvent medium. The complexes have been characterised by elemental analyses, molar conductivity, magnetic measurements, IR, electronic and mass spectral studies. The physicochemical and spectral studies suggest a distorted octahedral geometry around vanadium in the complexes. The antibacterial activities of the newly synthesised complexes, vanadium precursor and ligands have been screened in vitro against six bacterial species. The complexes have shown higher antibacterial effect than the free ligands.
Introduction
Over the years, an immense research interest has been directed towards coordination chemistry of vanadium, owing to the promising insulin-mimetic, appetite-suppressing and antihypertensive effects exhibited by vanadium complexes [Citation1]. The literature contains numerous reports on the chemistry of the vanadyl ion, VO2+, however, of late the chemistry of easily synthesisable dichloro vanadium (IV) chelate complexes from VO2+, constituted appropriate precursors for alkylation, reduction and substitution reactions and have been reported to be functionalised with a variety of ligands. Several octacoordinated non-oxovanadium (IV) complexes with multidentate N, O donor ligands [Citation2] and biphenylphenols have been reported [Citation3]. Of the numerous ligands known to form vanadium complexes, hydroxamic acids (naturally occurring or synthetic) constitute an important family of organic bioligands [Citation4]. The naturally occurring hydroxamic acids (siderophores) are involved in the microbial transport of iron [Citation5]. Hydroxamic acids, with the NHOH moiety are constituents of antibiotics, antifungal agents, food additives, tumour inhibitors and growth factors [Citation6–8], because of their low toxicities they have a wide spectrum of activities in biological systems [Citation9–11]. The powerful biological activity of structurally heterogeneous hydroxamic acids is related to their ability to form stable chelates with a variety of metal ions [Citation12,Citation13]. Metal complexes of hydroxamic acids have also attracted considerable attention because of their tautomerisation [Citation14] exhibiting hydroxamic or hydroximic acid forms. The theoretical and experimental studies have suggested that the hydroxamic form is prevalent in free acids [Citation15] or metal hydroxamates [Citation16]. Fewer studies have described vanadium (IV) and (V) hydroxamate complexes as compared to many other transition metal hydroxamates [Citation17–21] whereas vanadyl sulphate, ammonium vanadate and vanadyl acetylacetonate have been exploited as precursors [Citation22]. We have been interested in exploring the use of dichlorovanadium (IV) β-diketonates as precursors for the synthesis of new complexes. There has been much research interest in coordination compounds of biologically active ligands, so in continuation of our earlier work [Citation23] and as a result of the biological importance of vanadium and hydroxamate ligands, we report the synthesis and structural characterisation of mixed ligand non-oxovanadium (IV) complexes containing 1-phenyl-1, 3-butanedionate and hydroxamate ligands from the reactions of VCl2(bzac)2 with potassium phenoxyacetohydroxamate and cinnamohydroxamate ( and ). The newly synthesised complexes have been structurally characterised and assayed for their antibacterial activity, as the development of effective antibacterial drugs is a matter of great concern and the results are described.
Material and methods
Reagent-grade solvents were dried and distilled prior to use. All other chemicals were reagent grade. VCl2(bzac)2 was prepared from VO(bzac)2 using published methods [Citation24, Citation25] under nitrogen atmosphere and its formation and purity was checked by C, H, Cl and V microanalysis and IR spectral data. The potassium phenoxyacetohydroxamate and cinnamohydroxamate were synthesised using published methods [Citation26]. The vanadium content in the complexes was determined as V2O5 while chlorine was determined by Volhard’s method [42]. The carbon, hydrogen and nitrogen analysis were obtained on Eager 300 NCH System Elemental Analyser (Chandigarh). The molar conductances (10−3 M solutions in methanol) were obtained at 25 ± 0.1°C on Elico Conductivity Bridge Type CM-82T (Shimla). The magnetic susceptibilities were measured at room temperature by Guoy’s method using Hg[Co(NCS)4] as calibrant [43]. IR spectra of complexes were recorded as KBr pellets on a Nicolet-5700 FTIR spectrophotometer (Shimla). The pellets were prepared in a dry box to avoid the action of moisture. The electronic spectra of complexes were recorded on a Varian Cary-100 Bio UV-VIS spectrophotometer (Shimla) using methanol as solvent. The FAB-mass spectra were recorded on a Jeol SX 102/DA-6000 mass spectrometer/data system (Lucknow) using Argon/Xenon (6 KV, 10 mA). The accelerating voltage was 10 KV and m-nitrobenzylalcohol (NBA) was used as the matrix. All bacteria used were clinical isolates taken from the Post-Graduate Institute of Medical Education and Research, Chandigarh, India.
Synthesis
Preparation of VCl2-n(bzac)2(HL1,2)n
In a typical reaction to a solution of potassium phenoxyacetohydroxamate (0.46 g, 2 mmol /0.92 g, 4 mmol) /potassium cinnamohydroxamate (0.45 g, 2 mmol /0.90 g, 4 mmol) in methanol (20 mL), a solution of VCl2(bzac)2 (1 g, 2 mmol) in THF (20 mL) was added in separate experiments. The reaction mixture was stirred for 1 h and was then refluxed for 4–5 h. The white residue obtained during the course of the reaction was filtered and identified as KCl. The filtrate was distilled off to remove excess of solvent. The concentrate was then dried under vacuum by repeatedly treating with petroleum ether whereupon light-green and violet-blue coloured solids were obtained. These were recrystallised from dichloromethane.
Antibacterial activity test
The precursor VCl2(bzac)2, potassium phenoxyacetohydroxamate (KHL1), potassium cinnamohydroxamate (KHL2) and vanadium (IV) complexes derived from these ligands of composition VCl2-n(bzac)2(HL1,2)n (where bzac = 1-phenyl-1,3-butanedionate anion (C6H5COCHCOCH3–); HL1=C6H5OCH2C(O)NHO–, HL2=C6H5CH=CHC(O)NHO–, n=1 and 2) were screened in vitro for their antibacterial activity on selected bacteria Escherichia coli, Staphylococcus aureus, Proteus mirabilis, Pseudomonas aeruginosa, Staphylococcus epidermidis and Shigella flexneri using the minimum inhibitory concentration (MIC) method. All the samples were tested in triplicate.
MIC determination by two-fold serial dilution
The MIC assay [Citation27] was performed in a 96-well microtitre plate. For MIC assay of each test drug; a stock solution of 1mg/ mL of each drug was prepared in DMSO and a row of twelve wells was used out of which the last two wells were taken as control where no drug was added. To each of the ten wells, 100 μL of the Muller-Hinton broth was added, except the first well which received 200 μL of broth containing 500 μg/mL concentration of the test drug in DMSO. From the first well (containing test drug), 100 μL broth was withdrawn using a sterile tip, then added to the 100 μL of the broth in the second well and the contents mixed four times. Next, 100 μL was withdrawn from the second well and added to the third well and so on. In this way, a range of two-fold serial dilutions were prepared giving concentrations ranging from 500 μg/mL to 0.98 μg/mL. The broth in each of the wells was inoculated with 2 μL of the bacterial culture and the contents were mixed by ten clockwise and ten anti-clockwise rotations on a flat surface. The plate was then incubated at 35°C and the observations for growth of bacteria were recorded after 24 h.
Results and Discussion
Chemistry
The interaction of VCl2(bzac)2 with equi- and bimolar amounts of potassium phenoxyacetohydroxamate and potassium cinnamohydroxamate in THF + methanol solvent medium led to the formation of complexes VCl2-n(bzac)2(HL1,2)n in quantitative yields according to
Scheme 1. Schematic view of synthesis of bis(1-phenyl-1,3-butanedionato)non-oxovanadium(IV) hydroxamates (where n = 1,2).
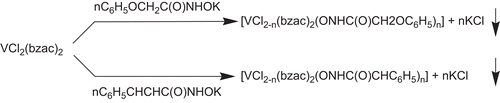
The complexes were light green and bluish-violet in colour, microcrystalline and soluble in common organic solvents such as methanol, chloroform, dichloromethane and acetonitrile, etc. The molar conductance values of the complexes (10−3M solutions) () in methanol in the 4.24 to 5.12 Scm2 mole−1 range suggested their non-electrolytic nature. The magnetic moment values (measured at room temperature) of the complexes were in the 1.71–1.75 BM range which suggested they were of a paramagnetic nature.
Table 1. Analytical Data of non-oxovanadium(IV) complexes.
IR spectra
A comparison of the IR spectra of VCl2-n(bzac)2(HL1,2)n with those of VCl2(bzac)2 and potassium phenoxyacetohydroxamate and cinnamohydroxamate has been useful in providing information regarding the formation of complexes. The absorption bands occurring in the 1680–1597 cm−1 and 1650–1560 cm−1 regions in potassium phenoxyacetohydroxamate and cinnamohydroxamate respectively have been attributed to υ(C=O) mode. The newly synthesised vanadium (IV) complexes exhibited bands due to υ(C=O) mode in 1663–1592 cm−1 and 1660–1552 cm−1 range respectively. No change in absorption band was observed due to υ(C–N) mode (occurring at ~1370 cm−1 in free ligands upon complexation). The bands occurring at 3297 cm−1 and 3229 cm−1 assigned to υ(N–H) mode in KHL1 and KHL2 respectively were observed to appear at ~3292−1 and ~3232 cm−1 in the respective complexes. This observation is indicative of the fact that –NH group is retained and does not participate in bonding. The bands due to υ(N-O) mode occurring at 938 cm−1 and 958 cm−1 in free ligands have been found to move to higher wave numbers and appeared at the ~975 cm−1 and ~971 cm−1 bands in respective series of complexes, suggesting that both the phenoxyacetohydroxamate and cinnamohydroxamate ions coordinated via the oxygen atom of the -NHO group only [Citation19]. The monodentate nature of the ligands thus inferred is in consonance with the observation that hydroxamate ligands which behave as bidentate ligands in binary complexes act as monodentate ligands in the presence of secondary ligands. This observation is contrary to previous reports describing O, O; N, N and N, O coordination in various hydroxamic acids [Citation28]. The two to three absorption bands occurring in the 560–465 cm−1 region have been assigned to υ(V–O) mode [Citation29] in complexes which is obvious for the mixed-ligand complexes. In the far-IR spectra of the VCl(bzac)2(HL1,2) complexes, the absorption bands appearing at ~365 cm−1 may be attributed to the υ(V–Cl) mode [Citation30]. The absorption bands due to υas(C=O) and υs(C=O) modes in VCl2(bzac)2 occur in 1590–1490 cm−1 and 1380–1310 cm−1 regions respectively. It may be mentioned here that we were unable to make unambiguous assignments for the absorption bands due to the oylacetonate ion in newly synthesised complexes due to the occurrence of some common absorption bands of hydroxamate and benzoylacetonate ions in the same region.
Electronic spectra
The electronic absorption spectra of potassium phenoxyacetohydroxamate and cinnamohydroxamate had sharp bands at 226, 257 nm and 221, 250 nm respectively in MeOH. These bands may be attributed to ligand π→ π* transitions. The light-green and bluish-violet solutions of the mixed-ligand complexes of composition VCl(bzac)2(HL1)] and V(bzac)2(HL1)2 displayed bands in 700–800 nm, 500–600 nm range and at 348 nm were assigned to LMCT benzoylacetonate(π*)/hydroxamate ligand (π*) → vanadium (dπ) charge transfer spectra, 2Eg ← 2T2g transition in octahedral geometry and MLCT vanadium (dπ ) → benzoylacetonate(π*) /hydroxamate ligand (π*) transitions respectively. In analogy to previous reports on non-oxovanadium(IV) complexes [Citation2,Citation31–33] the electronic spectra of bluish-violet solutions of VCl(bzac)2(HL2) and V(bzac)2(HL2)2 exhibited bands at 500–600 nm, 400–500 nm and ~315 nm assigned to 2Eg ← 2T2g transition in octahedral geometry, benzoylacetonate/cinnamohydroxamate ligand (π*) → vanadium (dπ) and MLCT vanadium (dπ) → benzoylacetonate(π*) /hydroxamate ligand (π*) transitions respectively.
Mass spectra
The major FAB-MS peaks observed for the precursor VCl2(bzac)2 and its derivatives with hydroxamate ligands are given in . Although the mass spectra of VCl2(bzac)2 did not display any molecular ion peak, the fragment ions observed at m/e 472, 411, 245, 205 and 534 corresponding to [VCl2(bzac)2 + CO]+, [VCl(bzac)2 + 2H]+, [VCl(bzac) – 2H]+, [VCl2(bzac) – C6H5]+ and [V(bzac)3]+ respectively clearly showed the formation of precursor [Citation34,Citation35]. The most intense peak at m/e 154 seemed to be that of the matrix.
Table 2. Mass-spectral data of non-oxovanadium(IV) complexes.
The FAB-MS spectra of VCl(bzac)2(HL1) (I), V(bzac)2(HL1)2] (II), [VCl(bzac)2(HL2) (III) and V(bzac)2(HL2)2 (IV) showed base peaks at 379, 154, 375 and 375 corresponding to [V(bzac)(HL1) + H]+, matrix, [V(bzac)(HL2) + H]+ and [V(HL2)2]+ ions in respective complexes. The fragment ions occurring at m/e 401, 423 and 461 may be attributed to [VO(HL1)2 + 2H]+, [VO(HL1)2 + Na + H]+, [VCl(HL1)2 + CH3CO]+ in the case of complex (I). The fragment ions at 678 and 701 corresponding to [V(bzac)2(HL1)2 – CO + H]+ and [V(bzac)2(HL1)2 – 4H]+ appeared in complex (II).
The FAB-MS peaks observed at m/e 467, 439 and 374 corresponded to (VCl(bzac)2 + 2NHO – 3H)+, [VCl(bzac)(HL2) + 2CH3]+ and [V(HL2)(bzac)]+ and the fragment ions at 730, 683 and 566 to [V(bzac)2(HL2)2 + NHO + 2H]+, [V(bzac)2(HL2) 2– CH3 + H]+, [V(bzac)2(HL2) + NHO]+, showed the formation of (III) and (IV) complexes respectively.
Based upon physicochemical and FTIR, UV-VIS and mass spectral data coupled with magnetic moment measurements, a distorted-octahedral geometry around vanadium may tentatively be proposed ().
Anti bacterial activity
The literature contains numerous reports concerned with the antimicrobial activity of ligands and their complexes against pathogenic bacteria and plant pathogenic fungi [Citation36, Citation37]. Hence, in the present work, the precursor VCl2(bzac)2, ligands and newly synthesised complexes were tested in vitro for their antibacterial activity against E. coli, S. aureus, P. mirabilis, P. aeruginosa, S. epidermidis and S. flexneri. From the MIC values given in it is quite apparent that both the ligands have similar antibacterial activity (62.5 – 250 μg/ mL) for all bacterial species except that HL2 is more effective against P. aeruginosa than that of HL1. The VCl2(bzac)2 has been observed to inhibit the bacterial growth in a concentration range of 125–250 μg/mL.
Table 3. Antibacterial activity of ligands and vanadium (IV) complexes by MIC method in μg/mL.
The newly synthesised complexes have been found to be more active (15.6 – 62.5 μg/mL) than free ligands HL1 and HL2. The non-chloro vanadium(IV) complexes (II) and (IV) have shown pronounced activity at a MIC of 15.6 μg/mL against S. epidermidis and S. flexneri respectively which is higher than that of the commercial antibiotic streptomycin used as a standard for the comparison of results. These complexes are also more effective against P. mirabilis compared to complexes (I) and (III). On the other hand, complexes (I) and (III) have shown higher antibacterial activity at MIC 31.2 μg/mL against the bacteria S. flexneri and P. aeruginosa respectively relative to complexes (II) and (IV). It is worth pointing out here that although all the complexes have shown similar antibacterial activity against E. coli and S. aureus, this observed trend is not the same for all these bacteria. The explanation to this observation may be ascribed to different liposolubility of complexes for different bacteria [Citation38,Citation39]. The enhancement in activity may be attributed to the coordination of hydroxamate ligands to the metal ion and an efficient diffusion of the metal complexes into bacterial cells [Citation40,Citation41]. The antibacterial activity of these complexes was compared with commercial antibiotic (streptomycin) and promising bioactivity was shown by the complexes studied.
Conclusion
The VCl2(bzac)2 has been exploited as precursor for the synthesis of mixed-ligand non-oxovanadium(IV) complexes derived from phenoxyacetohydroxamate and cinnamohydroxamate ligands. IR spectral studies depicted the unidentate nature of hydroxamate ligands in regard to coordination through the hydroxylamine oxygen (-NHO) only is displayed. The complexes have shown promising antibacterial activity against all the bacteria tested.
Acknowledgements
The authors would like to thank IIT Roorkee for magnetic susceptibility measurements and Department of Biotechnology, Himachal Pradesh University, Shimla for providing laboratory facilities.
Declaration of interest
Meena Kumari would like to thank the University Grant Commission, New Delhi for providing financial support. The authors would like to thank the Department of Science & Technology (DST), Government of India, New Delhi for providing financial assistance for use of the FT-IR and UV-Vis. spectrophotometer facility to the department. The authors would also like to thank Sophisticated Analytical Instrument Facility of CDRI, Lucknow and Punjab University, Chandigarh for recording mass spectra and elemental analysis.
References
- Butler A, Carrano CJ. Coordination chemistry of vanadium in biological system. Coord Chem Rev 1991; 109:61–105.
- Sutradhar M, Mukherjee G, Drew MGB, Ghosh S. Simple general method of generating non-oxo, non-amavadine model octacoordinated vanadium(IV) complexes of some tetradentate ONNO chelating ligands from various oxovanadium(IV/V) compounds and structural characterization of one of them. Inorg Chem 2007;46:5069–5075.
- Sharma N, Meena Thakur, M, Kumar V, Chaudhry SC. Synthesis and characterization of non-oxovanadium(IV) complexes of biphenylphenols. Ind Chem 2008;47A:685–689.
- Kehl H, ed. Chemistry and Biology of Hydroxamic Acids, NY, Karger S, 1982:6
- Köster B. ABC transporter-mediated uptake of iron, siderophores, heme and vitamin B12. Res Microbiol 2001;152:291–301.
- Arnold M, Brown DA, Degg O, Errington W, Hasse W, Herlihy K, Kemp TJ, Nimir H, Werner R. Hydroxamate-bridged dinuclear nickel complexes as models for urease inhibition. Inorg Chem 1998;37:2920–2925.
- Botos I, Scapozza L, Zhang D, Liotta LA, Meyer EF. Batimastat, a protein matrix metalloproteinase inhibitor, exhibits an unexpected mode of binding. Proc Nat Acad Sci USA 1996;93:2749–2754.
- Miller MJ. Synthesis and therapeutic potential of hydroxamic acid based siderophores and analogs. Chem Rev 1989;89:1563–1579.
- Dixon NE, Hinds JA, Fihelly AK, Gazzola C, Winzor DJ, Blakeley RL, Zerner B. The molecular size and the mechanism of inhibition by hydroxamic acids. Spectrophotometric titrations of enzymes with reversible inhibitors. Canad J Biochem 1980;58:1323–1334.
- Wilker SH, Prescott JM. Stereospecificity of amino acid hydroxamate inhibition of aminopeptidases. J Biol Chem 1983;258:13517–13521.
- Baker JO, Wilkes SH, Bayliss ME, Prescott JM. Hydroxamates and aliphatic boronic acids: marker inhibitors for aminopeptidase. Biochemistry 1983;22:2098–2103.
- Kurzak B, Kozlowski H, Farkas E. Hydroxamic and aminohydroxamic acids and their complexes with metal ions. Coord Chem Rev 1992;114:169–200.
- Boukhris S, Souizi A, Robert A. One-pot synthesis of α-chlorohydroxamic acids using gem dicyano epoxides. Tetrahed Lett 1996;37:179–182.
- Schrami J, Tkallecová M, Pataridis S, Soukupová L, Blchta V, Roithová J, Exner O. Ring-substituted benzohydroxamic acids: 1H, 13C and 15N NMR spectra and NHOH proton exchange. Magn Reso Chem 2005;43:535–542.
- Salmon L, Prost E, Merienne C, Hardré R, Morgant G. A convenient preparation of aldonohydroxamic acids in water and crystal structure of l-erythronohydroxamic acid. Carbohydr Res 2001;335:195–204.
- Santana MD, Garcia G, Peréz J, Molinis E, López G. Mononuclear hydroxamate five-coordinate nickel(II) complexes: structural and spectroscopic characterization. Inorg Chem 2001;40:5701–5703.
- Marmion CJ, Griffith D, Nolan KB. Hydroxamic acids–an intriguing family of enzyme inhibitors and biomedical ligands. Eur J Inorg Chem 2004;3003–3016.
- Banerjee TK, Brahma SK, Bag SP. Synthesis and spectroscopic studies of vanadium(IV) hydroxamates and their mixed ligated derivatives. Ind J Chem 1993;32A:776–780.
- Banerjee TK, Brahma SK, Bag SP. Studies on some simple and mixed ligated oxovanadium(V) complexes of hydroxamic acids. Ind J Chem 1992;31A:202–204.
- Fisher DC, Barclay-Peet SJ, Balfe CA, Raymond KN. Synthesis and characterization of vanadium (V) and -(IV) hydroxamate complexes. X-ray crystal structures of oxochlorobis(benzohydroxamato)vanadium(V) and oxoisopropoxo(N,N’-dihydroxy-N,N’-diisopropylheptanediamido)vanadium(V). Inorg Chem 1989;28:4399–4403.
- Shechter Y, Fridkin M, Gold Washer I, Gershonov E. Vanadium compounds in the treatment of elevated blood sugar levels US Patent 5888993, 2002.
- Maurya MR. Development of the coordination chemistry of vanadium through bis(acetylacetonato)oxovanadium(IV): synthesis, reactivity and structural aspects. Coord Chem Rev 2003;237:163–181.
- Sharma N, Kumari M, Kumar V, Chaudhry SC, Kanwar SS. Synthesis, characterization and antibacterial activity of vanadium (IV) complexes of hydroxamic acids. J Coord Chem 2010;63:176–184.
- Rowe RA, Jones MM. Vanadium(IV)oxy(acetylacetonate); [Bis(2,4-pentandiono)oxovanadium(IV). Inorg Synth 1957;5:113–116.
- Hambley TW, Hawkins CJ, Kabanos TA. Synthetic, structural, and physical studies of tris(2,4pentanedionato)vanadium(IV) hexachloroantimonate(V) and tris(1-phenyl-1,3-butanedionato)vanadium (IV) hexachloroantimonate(V). Inorg Chem 1987;26:3740–3745.
- Hauser CR, Renfrow WB. Benzohydroxamic acid. J Org Synth 1953;2:67.
- Mackie TJ, McCartney, Practical Medical Microbiology, Melbourne, Churchill Livingstone Press, 1989:696.
- Farkas E, Brown DA, Cittaro R, Glass WK. Metal complexes of glutamic acid-γ-hydroxamic acid (Glu- γ -ha) (N-hydroxyglutamine) in aqueous solution. J Chem Soc Dalton Trans 1993;2803–2807.
- Liu SX, Gao S. Synthesis, crystal structure and spectral properties of VO(acetylacetone)(benzoylhydrazone)(8-quinolinol). Polyhedron 1998;17:81–84.
- Von Dreele RB, Fay RC. Octahedral vanadium(IV) complexes. Synthesis and stereochemistry of vanadium(IV) beta-diketonates. J Am Chem Soc 1972;94:7935–7936.
- Paine TK, Weyhermuller T, Slep LD, Neese F, Bill E, Bothe E, Wieghardt K, Chaudhuri P. Nonoxovanadium(IV) and oxovanadium(V) complexes with mixed O, X, O-donor ligands (X = S, Se, P, or PO). Inorg Chem 2004;43:7324–7338.
- Cooper SR, Koh YB, Raymond KN. Synthetic, structural, and physical studies of bis(triethylammonium) tris(catecholato)vanadate(IV), potassium bis(catecholato)oxovanadate(IV), and potassium tris(catecholato)vanadate (III). J Am Chem Soc 1982;104:5092–5102.
- Auerbach U, DellsVedova BSPC, Wieghardt K, Nuber B, Weiss J. Synthesis and characterization of stable pseudo-octahedral tris-phenolato complexes of vanadium-(III), -(IV), and -(V). J Chem Commun 1990;1004.
- CJ Hawkins, Kabanos TA. Synthesis and characterization of (catecholato)bis(.beta.-diketonato)vanadium(IV) complexes. Inorg Chem 1989;28:1084–1087.
- Henderson W, Mcindoe JS. Mass Spectrometry of Inorganic and Organometallic Compounds. Chichester: John Wiley and Sons, 2005:147.
- Guilherme LR, Massabni AC, Cuin A, Oliveir LAA, Castellano EE, Heinrich TA, Costa-Neto CM. Synthesis, characterization, crystal structure, and biological studies of vanadium complexes with glycolic acid. J Coord Chem 2009;62:1561–1571.
- Colak AT, Colak F, Yesilel OZ, Buyukgungor O. Synthesis, characterization, crystal structure and biological activities of supramolecular compounds of Mn(II) and Zn(II) with dipicolinic acid and 8-hydroxyquinoline. J Coord Chem 2009;62:1650–1660.
- Yu LC, Tang ZL, Yi PG, Liu SL. Hydrothermal synthesis, crystal structures and antibacterial activities of two transition metal complexes with ciprofloxacin. J Coord Chem 2009;62:894–902.
- Saha DK, Padhye S, Anson CE, Powell AK. Hydrothermal synthesis, crystal structure, spectroscopy, electrochemistry and antimycobacterial evaluation of the copper (II) ciprofloxacin complex: [Cu(cf)2(BF4)2]·6H2O. Inorg Chem Commun 2002;5:1022–1027.
- Chohan ZH, Supuran CT, Scozzafava A. Metalloantibiotics: synthesis and antibacterial activity of cobalt(II), copper(II), nickel(II) and zinc(II) complexes of kefzol. J Enz Inhib Med Chem 2004;19:79–84.
- Chohan ZH, Hassan MUI, Khan KM, Supuran CT. In-vitro antibacterial, antifungal and cytotoxic properties of sulfonamide-derived Schiff’s bases and their metal complexes. J Enz Inhib Med Chem 2005;20:183–188.
- Vogel AI, A text book of quantitative inorganic analysis including elementary instrumental analysis. Longman, London, 1975:537.
- Figgis BN, Lewis J, , “The magnetochemistry of complex compounds” in Modern coordination chemistry, principles and methods. Lewis J, Wilkins RG, New York, 1964:415.