Abstract
We performed kinetic studies to determine whether oxamate analogues are selective inhibitors of LDH-C4, owing to their potential usefulness in fertility control and treatment of some cancers. These substances were shown to be competitive inhibitors of LDH isozymes and are able to discriminate among subtle differences that differentiate the active sites of LDH-A4, LDH-B4 and LDH-C4. N-Ethyl oxamate was the most potent inhibitor showing the highest affinity for LDH-C4. However, N-propyl oxamate was the most selective inhibitor showing a high degree of selectivity towards LDH-C4. Non-polar four carbon atoms chains, linear or branched, dramatically diminished the affinity and selectivity towards LDH-C4. N-Propyl oxamate significantly reduced ATP levels, capacitation and mouse sperm motility, in line with results shown by others, suggesting that LDH-C4 plays an essential role in mouse fertility.
Introduction
Mammalian LDHs (l-lactate:NAD+ oxidoreductase, EC 1.1.1.27) are tetrameric NAD+-specific dehydrogenases that catalyse the interconversion of lactate and pyruvate and participate in both glucose catabolism and gluconeogenesis from lactate.Citation1
LDH consists of A and B subunits that assemble into homo- or heterotetramers that are distributed in the body in combinations reflecting the metabolic requirements of different tissues and consistent with the catalytic properties of the isozymes. For example, LDH-A4 is most abundant in skeletal muscle where the oxygen deficiency from exercise requires glycolysis to satisfy metabolic needs, whereas LDH-B4 is expressed abundantly in cardiac muscle that is dependent upon aerobic metabolic pathways. Furthermore, the overall isozyme composition of a tissue changes with its developmental state.Citation2,Citation3
In addition to the two major A and B somatic isozymes, a third type of LDH polypeptide, denoted C, is found in testes. Synthesis of the C subunit occurs in the spermatocyte and coincides with gamete maturation and the repression of A and B isozymes synthesis.Citation4 Consequently, only the C4 homotetramer is observed in primary spermatocytes.
The tissue specificity of LDH-C4 is reflected in its remarkable thermal stability and its unique catalytic properties.Citation5–7 LDH-C4 is more sensitive to pyruvate inhibition and less sensitive to lactate inhibition and, consequently, is believed to perform as a lactate oxidase. It has been proposed that the physiological function of LDH-C4 is to generate the reducing equivalents necessary for sperm motility from the high concentrations of lactate found in the female reproductive tract. However, the discovery of LDH-C4 in association with sperm mitochondria, along with its broad specificity for alpha-hydroxy acids, has suggested that the enzyme participates in a transmitochondrial oxidation–reduction shuttle.Citation2,Citation8–10 Therefore, it appears that LDH-C4 is related to metabolic processes that provide energy for motility and survival of spermatozoa.
According to the above-mentioned functions of LDH-C4, it is evident that the development of selective inhibitors of LDH-C4 can be useful as biochemical tools for further characterization of the metabolic and fertility implications of LDH-C4 and would represent new lead compounds for the development of male antifertility drugs. Recently, it was reported that LDHc gene is expressed in a broad spectrum of tumours.Citation11,Citation12 Hence, the main objective of this study is to develop new selective inhibitors of LDH-C4 ().
Materials and methods
Materials
NAD+, NADP+, NADH, NADPH, d-glucose-6-phosphate, pyruvate, oxamate, l-malate and l-glutamate, all as sodium salts, and succinic acid were obtained from Sigma Chemical Co. (St. Louis, MO). Other chemicals used were of the highest purity available.
LDH-C4 from adult Swiss albino mouse testes was purified by a published method.Citation6 LDH-A4 from mouse skeletal muscle and LDH-B4 from mouse heart were purified by a procedure already described.Citation13 All the isozyme preparations showed a single band on polyacrylamide gel electrophoresis.
Mouse sperm was collected from vas deferens according to a published procedure.Citation14 Sperm were allowed to disperse for 10 min at 37°C in a Tyrode’s medium of pH 7.6 (120 mM NaCl, 2.8 mM KCl, 11.9 mM NaHCO3, 0.36 mM NaH2PO4, 0.49 mM MgCl2, 0.25 mM sodium pyruvate, 20 mM sodium lactate). The spermatozoa concentration was 8–10 × 106 spermatozoa per millilitre. Parallel incubations with and without inhibitor were started by the additions of the pre-warmed (37°C) medium.
General procedure for the preparation of the N-alkyl oxamates
A solution of 0.1 mol of the corresponding amine (ethylamine, propylamine, butylamine, isobutylamine and sec-butylamine) in ethyl ether (50 mL) was added dropwise to an ice-cold solution of diethyl oxalate (0.1 mol) in ethyl ether (100 mL). After being stirred 2 h, the reaction mixture was allowed to warm to room temperature and left overnight. A crystalline product separated, which was filtered off with suction, and the filtrate evaporated under slightly reduced pressure. Then, the residue was fractionated in vacuum. All the ethyl N-alkyl oxamates distilled as colourless oily products. Afterwards, 0.05 mol of the ethyl N-alkyl oxamates were shaken with 50 mL of 1 N NaOH for 30 min and extracted with ethyl ether. The aqueous phases were separated and acidified with 2 N HCl. Ether extraction and evaporation gave crude products which, on mixing with light petroleum, readily crystallized. The crystals were purified by recrystallization from chloroform.
N-Ethyl oxamic acid
The ethyl N-ethyl oxamate distilled at 70°C at 2 mm and it was used without further characterization. After recrystallization from chloroform, the free acid yield was 80%, mp 101–102°C. 1H-NMR (DMSO-d6) δ 1.03 (t, J = 7.2 Hz, 3H), 3.14 (quintuplet, J = 7.2 Hz, 2H), 8.70 (bs, 1H), IR (KBr) 3317, 2366, 1767, 1683, 1550, 1376 cm−1. Anal. Calcd. for C4H7NO3: C, 48.04; H, 6.03; N, 11.96. Found: C, 48.38; H, 6.21; N, 11.75.
N-Propyl oxamic acid
The ethyl N-propyl oxamate distilled at 80°C at 2 mm and it was used without further characterization. After recrystallization from chloroform, the free acid yield was 82%, mp 105–106°C. 1H-NMR (CDCl3) δ 0.96 (t, J = 7.0 Hz, 3H), 1.60 (sextuplet, J = 7.0 Hz, 2H), 3.34 (q, J = 7.0 Hz, 2H), 7.25 (bs, 1H), IR (KBr) 3293, 2981, 1770, 1674, 1556, 1370 cm−1. Anal. Calcd. for C5H9NO3: C, 45.80; H, 6.92; N, 10.68. Found: C, 45.84; H, 6.94; N, 10.87.
N-Butyl oxamic acid
The ethyl N-butyl oxamate distilled at 85°C at 2 mm and it was used without further characterization. After recrystallization from chloroform, the free acid yield was 82%, mp 106–107°C. 1H-NMR (CDCl3) δ 0.95 (t, J = 7.2 Hz, 3H), 1.38 (sextuplet, J = 7.2 Hz, 2H), 1.58 (quintuplet, J = 7.2 Hz, 2H), 3.38 (q, J = 7.2 Hz, 2H), 7.47 (bs, 1H), 9.16 (bs, 1H). IR (KBr) 3307, 2962, 1788, 1673, 1550, 1307 cm−1. Anal. Calcd. for C6H11NO3: C, 49.65; H, 7.64; N, 9.65. Found: C, 49.78; H, 7.35; N, 9.88.
N-Isobutyl oxamic acid
The ethyl N-isobutyl oxamate distilled at 93°C at 3 mm and it was used without further characterization. After recrystallization from chloroform, the free acid yield was 82%, mp 107–108°C. 1H-NMR (CDCl3) δ 0.95 (d, J = 6.67 Hz, 6H), 1.85 (m, J = 6.67 Hz, 1H), 3.18 (t, J = 6.67 Hz, 2H), 7.4 (bs, 1H), 7.78 (bs, 1H); IR (KBr) 3280, 2950, 1755, 1670, 1550, 1350 cm−1. Anal. Calcd. for C6H11NO3: C, 49.65; H, 7.64; N, 9.65. Found: C, 49.58; H, 7.48; N, 9.83.
N-sec-Butyl oxamic acid
The ethyl N-sec-butyl oxamate distilled at 92°C at 3 mm and it was used without further characterization. After recrystallization from chloroform, the free acid yield was 82%, mp 106–107°C. 1H-NMR (CDCl3) δ 0.91 (t, J = 7.4 Hz, 3H), 1.20 (d, J = 6.67 Hz, 3H), 1.54 (q, J = 7.0 Hz, 2H), 3.88 (m, J = 6.67 Hz, 1H), 7.21 (bs, 1H), 8.77 (bs, 1H); IR (KBr) 3300, 2950, 1765, 1675, 1550, 1355 cm−1. Anal. Calcd. for C6H11NO3: C, 49.65; H, 7.64; N, 9.65. Found: C, 49.48; H, 7.76; N, 9.73.
Determination of enzymatic activities
Lactate dehydrogenase activity was determined by recording the absorbance change at 340 nm produced by the oxidation of NADH. Assays were performed at 37°C. The reagent mixture contained 0.115 mM NADH, 50 mM sodium phosphate buffer, pH 7.4, sodium pyruvate (0.31 mM for LDH-C4 and LDH-B4 and 1.25 mM for LDH-A4) and the enzyme preparation, diluted with phosphate buffer, pH 7.4, to provide a ΔE340 of 0.06–0.07 per minute when the activity was assayed in a 1-cm light path. For determination of dissociation constants, the isozymes, the inhibitors (oxamate or oxamate derivatives) and the coenzyme were incubated with the buffer used in the assay for 10 min at 37°C before adding the substrate. Dissociation constants were determined from those of Km and V obtained with and without oxamate or oxamate derivatives added to the assay mixture using various concentrations of substrate at a constant inhibitor concentration, and plotting the slope (Km/V) against inhibitor concentrations.Citation15 Malate dehydrogenase (EC 1.1.1.37) l-malate:NAD+ oxidoreductase, glutamate dehydrogenase (EC 1.4.1.4) l-glutamate:NADP+ oxidoreductase (deaminating), isocitrate dehydrogenase (EC 1.1.1.42) isocitrate:NADP+ oxidoreductase (decarboxylating) and succinate dehydrogenase (EC 1.3.99.1) succinate:(acceptor) oxidoreductase were assayed in the crude extracts of mice organs. All enzymes were assayed by methods described by Wong et al.Citation13
Sperm motility evaluation
Sperm motility was quantified by the wave amplitude described by the flagellum.Citation16 The sperm suspensions were observed under phase contrast microscope and sperm movement was registered with a video camera that was connected to the microscope. The movements of mice sperm were recorded at 10 frames per second and were visualized on a videomonitor. We analysed a minimum of 20 sperm per sample. Only sperms that exhibited undulatory movement of the flagellum visible on the two-dimensional plane of the screen were analysed.
Sperm capacitation in vitro
The sperm cells were maintained at 37°C in a modified Tyrode’s medium (120 mM NaCl, 2.8 mM KCl, 11.9 mM NaHCO3, 0.36 mM NaH2PO4, 0.49 mM MgCl2, 0.25 mM sodium pyruvate, 20 mM sodium lactate, 3 mg/mL BSA, 1 mg/mL glucose, 1.7 mM CaCl2). To achieve the capacitation, the spermatozoa were incubated for 2 h with and without the inhibitor. At the end of this time, the cells were stained with chlortetracycline (CTC) according to the method described by Pietrobon et al.Citation17 The assay was performed by counting 100 cells per sample in high magnification at 400×. The samples were counted immediately, and sperm status characterized by the fluorescence pattern as described by Ward and Storey.Citation18
Sperm ATP levels
After incubation in modified Tyrode’s medium, with and without inhibitor, aliquots of 25 µL sperm suspension were transferred to a tube containing 450 µL of boiling extraction buffer (4 mM EDTA, 0.1 M Tris–HCl, pH 7.8), and incubated at 100°C for 5 min. The supernatant was collected after centrifugation at 20,000 g for 5 min. ATP was measured in duplicate 50 µL aliquots of the supernatant by using a luciferase bioluminescence assay according to the manufacturer’s protocol (ATP Bioluminescent Somatic Cell Assay Kit FL-ASC; Sigma Chemical Co.).
This investigation was performed following principles in the Declaration of Helsinki.
Results
In a first approximation, we evaluated the potencies of the mouse LDH isozyme inhibitors using the concentration–response plot for comparing the extent of inhibition. shows the inhibitory effect of low concentration N-propyl oxamate on the activity of LDH isozymes. Under the experimental conditions employed, only LDH-C4 was inhibited at 0.1 mM concentration of N-propyl oxamate; LDH-C4 was inhibited up to 70% without affecting the enzymatic activity of the other two isozymes.
Figure 2. Effect of N-propyl oxamate on mouse LDH isozymes. Velocities were calculated taking the maximum activity without the inhibitor as 100%. (◯) LDH-A4, (▾) LDH-B4 and (•) LDH-C4. We used a constant concentration of 0.31 mM pyruvate for LDH-C4 and LDH-B4 and 1.25 mM for LDH-A4.
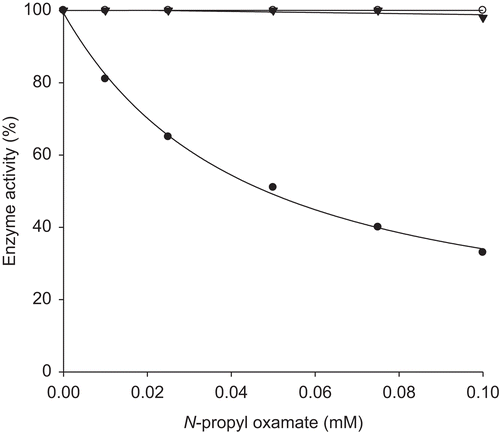
To identify true potencies of these compounds as LDH isozyme inhibitors, we determined the type of inhibitor and dissociation constants because these variables are more appropriate comparators by which the effectiveness of a compound is judged. The results for oxamate and oxamate derivatives are summarized in . Inhibition of mouse LDH-A4, LDH-B4 and LDH-C4 by oxamate was non-selective. Dissociation constants were 0.08, 0.06 and 0.03 mM for LDH-A4, LDH-B4 and LDH-C4, respectively. In all cases, inhibition was competitive to the binding of pyruvate, as shown in , for the inhibition of LDH-C4 by N-propyl oxamate. The lack of selectivity by oxamate and the pattern of competitive inhibition of the binding of pyruvate are consistent with previous studies of the three isozymes forms of LDH.Citation19,Citation20 Inhibition of LDH-A4, LDH-B4 and LDH-C4 by derivatives of oxamate reflects some enhancement in selectivity compared with oxamate (). As with oxamate, inhibition was competitive to the binding of pyruvate for the three LDHs (). In general, dissociation constants for oxamate derivatives increased as chain grew for all three LDH isozymes and increased the selectivity towards LDH-C4 isozyme in comparison with LDH-A4 and LDH-B4 (). The range of potency and selectivity for inhibition of the three LDHs by oxamate derivatives was broad. Inhibition by N-ethyl oxamate was, at least, six times more potent than any other oxamate derivative against all three isozymes, but it was not the most selective. N-Ethyl oxamate was 1000 times more potent than N-isobutyl derivative as inhibitor of LDH-C4 and 89 and 414 times more potent than N-sec-butyl derivative as inhibitor of LDH-A4 and LDH-B4, respectively. N-Propyl oxamate was a less potent inhibitor of all three LDHs than N-ethyl oxamate but it was more selective. N-Propyl oxamate was 25, 12.5 and 6 times less potent than N-ethyl oxamate as inhibitor of LDH-A4, LDH-B4 and LDH-C4, respectively. N-Propyl oxamate was 146 and 74 times more selective for LDH-C4 than LDH-A4 and LDH-B4, respectively. N-Ethyl oxamate was 70 and 17.5 times more selective for LDH-C4 than LDH-A4 and LDH-B4, respectively. The oxamates substituted with butyl and isobutyl groups were non-selective and were not potent inhibitors of the three LDHs. Their dissociation constants were at least 25-fold higher than those of oxamate with all three isozymes. The sec-butyl oxamate showed 30-fold increased selectivity for LDH-C4 over LDH-A4 and LDH-B4; however, it showed low potency. It was 156, 242 and 13 times weaker inhibitor than oxamate for LDH-A4, LDH-B4 and LDH-C4, respectively.
Table 1. Inhibition of mouse LDH isozymes by oxamate and oxamate derivatives.
Figure 3. Effect of pyruvate on the inhibitory activity of N-propyl oxamate on mouse LDH-C4. (A) Reciprocal values of V were calculated taking the maximum activity without inhibitor as 100%. (•) Assays without inhibitor, (◯) with 0.01 mM, (▾) with 0.075 mM of the N-propyl oxamate. (B) Determination of dissociation constant from re-plot of slope values against inhibitor concentrations.
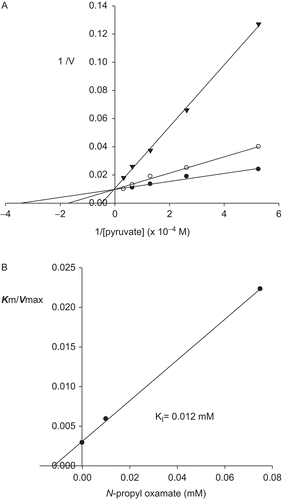
The effects of oxamate derivatives on the activity of LDH isozymes and other dehydrogenases were also compared (). Initial velocities were determined in the presence and absence of the oxamate derivatives. Concentrations of the substrates as well as those of the coenzymes and inhibitors are indicated in . Maximum enzymatic activity was attained at the concentrations of substrates and coenzymes indicated for each dehydrogenase. A strong inhibition of LDH-C4 was observed with a concentration of 0.1 mM of the ethyl and propyl oxamates, and there was no inhibition by butyl, isobutyl and sec-butyl derivatives. At this concentration, LDH-A4 and LDH-B4 were inhibited by N-ethyl oxamate and barely inhibited by N-propyl oxamate. At higher concentration of these oxamate derivatives (100 times higher, 10 mM), LDH-A4 and LDH-B4 were also inhibited. At this concentration, LDH-A4 and LDH-B4 were inhibited by N-butyl, N-isobutyl and N-sec-butyl derivatives, whereas glutamate, malate, succinate and isocitrate mouse dehydrogenases were only slightly inhibited by the oxamate derivatives (); however, there were detectable differences between inhibitors. These dehydrogenases were 3–4% more inhibited by N-ethyl oxamate than N-propyl oxamate; all four dehydrogenases were not affected by N-butyl oxamate. N-Isobutyl and N-sec-butyl oxamate derivatives inhibited succinate dehydrogenase activity without affecting malate, glutamate and isocitrate dehydrogenases. These results also indicated that N-substituted oxamates up to three carbon atom chains are selective inhibitors of mouse LDH-C4.
Table 2. Effect of N-substituted oxamates on the activity of mouse dehydrogenases.
We used N-propyl oxamate, due to its highest selectivity towards LDH-C4, to test its effect on other biological activities in mouse sperm. shows the effects of N-propyl oxamate on ATP levels, capacitation and sperm motility. All the measured parameters that are part of the fertilizing capacity of sperm decreased. The process of capacitation diminished 90%, and the ATP levels and sperm motility were reduced by 50%.
Table 3. Effect of N-propyl oxamate on biological activities related to fertilizing capacity of mouse sperm.
Discussion
Comparative studies of catalytic properties have revealed differences between LDH-C4 and the other lactate dehydrogenase isozymes.Citation7,Citation8,Citation10,Citation21 All LDH isozymes catalyse the reaction from pyruvate to lactate and from lactate to pyruvate. However, the LDH-C4 shows activity towards α-keto and α-hydroxy acid, of longer carbon chain than those of pyruvate and lactate ().
The C isozyme total chain, comprising 332 amino acids, presents four domains, homologous to those previously described for isozymes A and B: the N-terminal arm, the coenzyme-binding region, the loop region and the substrate-binding region.Citation10,Citation22 There are fewer differences between the same isozymes in different species, than between different types of isozymes within a given species. On the other hand, there are more homologies between A and B polypeptides than between either of them and C subunits. Key LDH catalytic residues are present in all three mammalian LDH subunits including the active site proton acceptor (His193) as well as coenzyme (Arg99, Asn138)- and substrate (Arg106, Arg169, Thr248)-binding residues.Citation23 X-ray crystallography of the LDH tertiary structures from a range of species are well-represented in the Protein Data Bank. A high level of structural similarity is apparent despite significant differences in primary structure between each of these enzymes and conformational change occurs only during catalysis.Citation24 In all LDH isozymes, the binding of substrate to LDH is obligatorily preceded by the formation of the LDH/NADH binary complex. Once the substrate reaches positioning close enough to the enzyme’s active site, which is buried into the enzyme, the loop region closes over the active site entrance, water leaves the binding pocket, the enzyme tightens around the bound substrate and key residues are brought in close proximity to the substrate.Citation24,Citation25
The mobile loop region of LDH-C4 (residues 94–115) from mouse is markedly different from that of somatic lactate dehydrogenase isozymes.Citation10,Citation26 The increased hydrophobicity of the amino acids in this region and the induced fit process that allows the enzyme to discriminate between keto acid with different side chains are most likely the cause of the unusual catalytic properties by the sperm-specific isozyme.
It is not generally considered that the dinucleotide-binding sites of dehydrogenases are attractive drug targets because the structures of the cofactor-binding sites of many dehydrogenases, as determined by crystallography, are similar. The classic crystallography studies of Rossmann et al.,Citation27 which identified the structural homology that defines the dinucleotide fold (Rossmann-fold), included LDH. For example, gossypol, gossypol derivatives and substituted dihydroxy naphthoic acids are competitive inhibitors of LDH isozymes and other numerous dehydrogenases by competing with the nucleotide cofactor.Citation28–30
To obtain possible specific inhibitors of mouse LDH-C4, we selected oxamate, an isosteric and isoelectronic analogue of pyruvate that is a competitive inhibitor of substrate-binding site on LDH isozymes,Citation31 that allowed us to increase the length of the inhibitor by the attachment of the non-polar, 1–4, linear or branched, carbon atom chain, trying to create an additional binding point that could increase the affinity and selectivity of these inhibitors for LDH-C4. In a previous study, we demonstrated that N-isopropyl oxamate was a competitive and selective inhibitor of mouse LDH-C4.Citation13 Therefore, we assumed that other oxamate derivatives would fulfil the structural and steric requirements to be competitive and selective inhibitors of mouse LDH-C4. The oxamate moiety will direct the N-alkyl oxamate molecule against the active site of LDH isozymes, and the attachment of the non-polar chain to the nitrogen will give N-alkyl oxamates more affinity and selectivity for mouse LDH-C4 isozyme, due to hydrophobic interactions and rearrangement of the protein structure induced by inhibitor binding, both associated with the non-polar mobile loop of this isozyme.
The kinetic studies showed that these substances were really inhibitors of LDH isozymes. The inhibitions were shown to be competitive with respect to the substrate pyruvate. LDH-C4 was more sensitive than LDH-A4 and LDH-B4 to inhibition by oxamate derivatives. The introduction of non-polar ethyl group in the nitrogen of the oxamate molecule increased the affinity for LDH-C4 15-fold compared with oxamate inhibition and the affinity towards N-propyl oxamate was 6-fold smaller than ethyl derivative although 2.5-fold better than oxamate. On the other hand, N-propyl oxamate was more selective than N-ethyl oxamate for LDH-C4. Apparently, linear or branched chains longer than three carbon atoms attached to the nitrogen of oxamate provide steric hindrance in LDH-C4 active site because butyl, isobutyl and sec-butyl oxamates had very high dissociation constants; their affinities to LDH-C4 were 62.5-, 167- and 33-fold, respectively, smaller than N-propyl oxamate.
The reduced inhibitory effects of the N-substituted derivatives with a chain longer than three carbon atoms on LDH-A4 and LDH-B4 cannot be attributed to the lack of space in the substrate-binding pocket of these isozymes because it was demonstrated that space is available in their substrate-binding sites for bulky inhibitors such as N-phenyl oxamate and salicylate derivatives.Citation31
It is clear that the mobile loop region must play a significant role in the differential binding of all the oxamate derivatives in LDH isozymes. On one hand, the increased hydrophobicity of the amino acids in this region on LDH-C4 allowed them to reach a position close enough to the enzyme’s active site; on the other hand, the loop closure over the active site that induces conformational changes in the isozymes could allow additional protein–inhibitor interactions that could be responsible for the selective inhibition. Studies of protein dynamics in binary (LDH/NADH) and ternary complexes (LDH/NADH/oxamate) and temperature-jump relaxation spectroscopy in human LDH-A4 and pig LDH-B4 have suggested that LDH/NADH complex exists in multiple conformations, some open and other close structures due to changes in internal hydrogen bonding and/or increases in the solvation of the protein structure. These studies also demonstrated that multiple open conformations yield multiple-binding pathways.Citation24,Citation25,Citation32 Also, a recent study, using quantum mechanics/molecular mechanics methods to capture the influence of the whole enzymatic environment on the reaction occurring in the enzyme active site, showed the important influence of the LDH quaternary structure on the structure of the active site.Citation33
All these conformational changes, to which mobile loop is of substantial importance and that occurs only during catalysis, might explain the selectivity of the inhibitors towards LDH-C4.
We examined the possibility that oxamate derivatives would inhibit other dehydrogenases different from LDH isozymes. At very high concentrations of oxamate derivatives (10 mM), glutamate, malate, succinate and isocitrate dehydrogenases were only slightly inhibited. These results indicated that oxamate derivatives containing a chain of no more than three carbon atoms were selective inhibitors of mouse LDH-C4, compared with other dehydrogenases.
We also found that N-propyl oxamate significantly reduces ATP levels, capacitation and mouse sperm motility. These results are consistent with those obtained by other investigators,Citation34–36 who used both, LDH inhibitors and generation of Ldhc null mouse to demonstrate that LDH-C4 plays an essential role in maintenance of the processes of glycolysis and ATP production in the flagellum that are required for progressive motility, sperm capacitation and mouse fertility.
In conclusion, the kinetic studies showed that these substituted oxamic acids are competitive inhibitors of LDH isozymes and are able to discriminate among subtle differences that differentiate the active sites of LDH-A4, LDH-B4 and LDH-C4. N-Ethyl oxamate was the most potent inhibitor showing the highest affinity for LDH-C4. However, N-propyl oxamate was the most selective inhibitor showing a high degree of selectivity towards LDH-C4. Moreover, N-propyl oxamate and other LDH competitive inhibitors in this study may have potential therapeutic use in effective contraception and in the treatment in a variety of cancers, where Ldhc gene was shown to express with high frequencyCitation11,Citation12 or in the treatment of lactic acidaemia associated with acute myocardial infarction.Citation37
Declaration of interest
This work was partially supported by research grants from the Secretaría de Investigación y Posgrado del Instituto Politécnico Nacional (SIP-IPN), México. C.W. and L.R.P. are fellows of the COFAA-IPN and of the SNI-CONACYT. M.A.Ch.T., A.C.H. and J.C.M. were fellows of the CONACYT and PIFI-IPN.
References
- Everse J, Kaplan NO. Lactate dehydrogenases: structure and function. Adv Enzymol Relat Areas Mol Biol 1973;37:61–133.
- Goldberg E, Eddy EM, Duan C, Odet F. LDHC: the ultimate testis-specific gene. J Androl 2010;31:86–94.
- Markert CL, Shaklee JB, Whitt GS. Evolution of a gene. Multiple genes for LDH isozymes provide a model of the evolution of gene structure, function and regulation. Science 1975;189:102–114.
- Li SS. Lactate dehydrogenase isoenzymes A (muscle), B (heart) and C (testis) of mammals and the genes coding for these enzymes. Biochem Soc Trans 1989;17:304–307.
- Hawtrey CO, Goldberg E. Some kinetic aspects of sperm specific lactate dehydrogenase in mice. J Exp Zool 1970;174:451–461.
- Wong C, Yañez R, Brown DM, Dickey A, Parks ME, McKee RW. Isolation and properties of lactate dehydrogenase isozyme X from Swiss mice. Arch Biochem Biophys 1971;146:454–460.
- Coronel CE, Burgos C, Gerez de Burgos NM, Rovai LE, Blanco A. Catalytic properties of the sperm-specific lactate dehydrogenase (LDH X or C4) from different species. J Exp Zool 1983;225:379–385.
- Blanco A, Burgos C, Gerez de Burgos NM, Montamat EE. Properties of the testicular lactate dehydrogenase isoenzyme. Biochem J 1976;153:165–172.
- Burgos C, Maldonado C, Gerez de Burgos NM, Aoki A, Blanco A. Intracellular localization of the testicular and sperm-specific lactate dehydrogenase isozyme C4 in mice. Biol Reprod 1995;53:84–92.
- Gupta GS. LDH-C4: a unique target of mammalian spermatozoa. Crit Rev Biochem Mol Biol 1999;34:361–385.
- Koslowski M, Türeci O, Bell C, Krause P, Lehr HA, Brunner J et al. Multiple splice variants of lactate dehydrogenase C selectively expressed in human cancer. Cancer Res 2002;62:6750–6755.
- Tang H, Goldberg E. Homo sapiens lactate dehydrogenase c (Ldhc) gene expression in cancer cells is regulated by transcription factor Sp1, CREB, and CpG island methylation. J Androl 2009;30:157–167.
- Wong C, Rodríguez-Páez L, Nogueda B, Pérez A, Baeza I. Selective inhibition of the sperm-specific lactate dehydrogenase isozyme-C4 by N-isopropyl oxamate. Biochim Biophys Acta 1997;1343:16–22.
- Mújica A, Moreno-Rodríguez R, Naciff J, Neri L, Tash JS. Glucose regulation of guinea-pig sperm motility. J Reprod Fertil 1991;92:75–87.
- Dixon M, Webb EC. Enzymes, 3rd ed. London: Longmans Group Ltd., 1979:349.
- Vera O, Muñoz MG, Jaffe K. Wave parameters of the sperm flagellum as predictors of human spermatozoa motility. Andrologia 1998;30:153–157.
- Pietrobon EO, Domínguez LA, Vincenti AE, Burgos MH, Fornés MW. Detection of the mouse acrosome reaction by acid phosphatase. Comparison with chlortetracycline and electron microscopy. J Androl 2001;22:96–103.
- Ward CR, Storey BT. Determination of the time course of capacitation in mouse spermatozoa using a chlortetracycline fluorescence assay. Dev Biol 1984;104:287–296.
- Battellino LJ, Jaime FR, Blanco A. Kinetic properties of rabbit testicular lactate dehydrogenase isozyme. J Biol Chem 1968;243:5185–5192.
- Holmes LD, Schiller MR, Boeker EA. Kinetic analysis of lactate dehydrogenase using integrated rate equations. Experientia 1993;49:893–901.
- Burgos C, Gerez de Burgos NM, Coronel CE, Blanco A. Substrate specificity of the lactate dehydrogenase isoenzyme C4 from human spermatozoa and a possible selective assay. J Reprod Fertil 1979;55:101–106.
- Li SS, Fitch WM, Pan YC, Sharief FS. Evolutionary relationships of vertebrate lactate dehydrogenase isozymes A4 (muscle), B4 (heart), and C4 (testis). J Biol Chem 1983;258:7029–7032.
- Holmes RS, Goldberg E. Computational analyses of mammalian lactate dehydrogenases: human, mouse, opossum and platypus LDHs. Comput Biol Chem 2009;33:379–385.
- Pineda JR, Callender R, Schwartz SD. Ligand binding and protein dynamics in lactate dehydrogenase. Biophys j 2007;93:1474–1483.
- Qiu L, Gulotta M, Callender R. Lactate dehydrogenase undergoes a substantial structural change to bind its substrate. Biophys J 2007;93:1677–1686.
- Chang SM, Lee CY, Li SS. Structural relatedness of mouse lactate dehydrogenase isozymes, A4 (muscle), B4 (heart), and C4 (testis). Biochem Genet 1979;17:715–729.
- Rossmann MG, Liljas A, Branden CI, Banaszak LJ. Evolutionary and structural relationships among dehydrogenases. In: Boyer PD, ed. The Enzymes, Vol. XI. New York: Academic Press, 1975:61–102.
- Gupta GS, Kapur S, Kinsky RG. Inhibition kinetics of lactate dehydrogenase isoenzymes by gossypol acetic acid. Biochem Int 1988;17:25–34.
- Vander Jagt DL, Deck LM, Royer RE. Gossypol: prototype of inhibitors targeted to dinucleotide folds. Curr Med Chem 2000;7:479–498.
- Yu Y, Deck JA, Hunsaker LA, Deck LM, Royer RE, Goldberg E et al. Selective active site inhibitors of human lactate dehydrogenases A4, B4, and C4. Biochem Pharmacol 2001;62:81–89.
- Baker BR. Design of Active-Site-Directed Irreversible Enzyme Inhibitors. New York: John Wiley & Sons, 1967:156–191.
- Zhadin N, Gulotta M, Callender R. Probing the role of dynamics in hydride transfer catalyzed by lactate dehydrogenase. Biophys J 2008;95:1974–1984.
- Swiderek K, Paneth P. Importance of the lactate dehydrogenase quaternary structure in theoretical calculations. J Phys Chem b 2010;114:3393–3397.
- Odet F, Duan C, Willis WD, Goulding EH, Kung A, Eddy EM et al. Expression of the gene for mouse lactate dehydrogenase C (Ldhc) is required for male fertility. Biol Reprod 2008;79:26–34.
- O’Flaherty CM, Beorlegui NB, Beconi MT. Lactate dehydrogenase-C4 is involved in heparin- and NADH-dependent bovine sperm capacitation. Andrologia 2002;34:91–97.
- Duan C, Goldberg E. Inhibition of lactate dehydrogenase C4 (LDH-C4) blocks capacitation of mouse sperm in vitro. Cytogenet Genome Res 2003;103:352–359.
- Kotlyar AB, Randazzo A, Honbo N, Jin ZQ, Karliner JS, Cecchini G. Cardioprotective activity of a novel and potent competitive inhibitor of lactate dehydrogenase. FEBS Lett 2010;584:159–165.