Abstract
Melatonin (MLT) is a strong free-radical scavenger, which protects the body from the effects of oxidants. In recent years, MLT have been described resulting in much attention in the development of synthetic compounds possessing. As a part of our ongoing study a series of indole-based MLT analogue hydrazide/hydrazone derivatives were synthesized, characterized and in vitro antioxidant activity was investigated by evaluating their reducing effect against oxidation of a redox sensitive fluorescent probe. Membrane stabilizing effect of all compounds was also investigated by lactate dehydrogenase leakage assay. Furthermore voltammetric methods have been applied to the synthesized compounds to characterize oxidation potentials to get insight into their metabolism owing to the oxidation mechanisms taking place at the electrode and in the body share similar principles.
Introduction
Oxidants and antioxidants have a significant function in maintaining a balance between free radicals and the antioxidant system of the bodyCitation1. Reactive oxygen species (ROS) and reactive nitrogen species are well known as both harmful and beneficial species. Overproduction of ROS results in oxidative stress that can cause fatal damage to cell structures, including lipids and membranes, proteins, and DNACitation2. It is known that antioxidants could be suitable for preventive in several diseases related with oxidative stress such as atherosclerosis, inflammatory injury, cancer, cardiovascular diseases, and neurodegenerative disordersCitation3,Citation4.
It is experimentally and clinically well established that melatonin (MLT) and its metabolites can function as endogenous free-radical scavengers and broad-spectrum antioxidantsCitation5. Studies also showed the role of MLT and its derivatives in many physiological processes and therapeutic functions, such as the regulation of circadian rhythm and immune functionsCitation6. In recent years, many physiological properties of MLT have been described resulting in much attention in the development of synthetic compounds possessing the indole ring. Synthetic indole derivatives such as indole-3-propionic acidCitation7, indole amine-triazolesCitation8, and stobadineCitation9 have shown significant antioxidant activity. Our group identified the antioxidant activity of MLT analogue indole derivatives such as 2-phenylindolesCitation10, indole-3-propionamidesCitation11, 5-bromoindole hydrazide/hydrazones derivativesCitation12, and N-methylindole hydrazide/hydrazonesCitation13. In these studies most of the compounds showed significant antioxidant activity at concentrations comparable with or much higher than that of MLT.
Hydrazone derivatives possess a range of pharmacological activities including antitumoral, anti-microbial, anti-malarial, anti-convulsant, and anti-inflammatory activityCitation13. Recently hydrazones were found as potential antioxidantsCitation14–17. It was shown that oxidative stress induces lipid peroxidation of cellular membranes resulting in the generation of reactive carbonyl compounds that involves in the “carbonyl stress”Citation18,Citation19. Hydrazine derivatives also exhibit carbonyl scavenger activity by the reaction of ketones/aldehydesCitation20.
The use of electrochemical techniques for the determination of compounds of pharmaceutical interest is continually gaining in importanceCitation21. The obtained results from the redox properties of drugs and biomolecules might have profound effects on our understanding of their in vivo redox behavior or pharmaceutical activityCitation22–24. Voltammetric techniques are most suitable for investigating the redox properties of new drug candidates to obtain information for its metabolic fateCitation25. Electrode mechanisms might mimic enzyme reactions; therefore, electrochemistry may be of value in the study of enzyme reactions in biological systemsCitation24. Due to the existence similarity between electrochemical and biological reactions it can be assumed that the oxidation mechanisms taking place at the electrode and in the body share similar principles. We have previously reported the electroanalytical evaluation and determination of indole-3-propionic acid derivativesCitation26, indolylthiohydantoin derivativesCitation27, 2-phenylindole derivativesCitation28, and indole-3-carboxaldehyde izonicotinoyl hydrazonesCitation29 by voltammetric studies in order to enlighten the possible relevance to in vitro metabolism of these compounds.
In new drug development studies, combination of different pharmacophores in the same structure may lead to new compounds having higher biological activity. It was thought the combination of indole-and hydrazone-type compounds might provide new effective drugs against free radicals. As a part of our ongoing study 21 new indole-based MLT analogue hydrazide/hydrazone derivatives were synthesized and their antioxidant activity was investigated in vitro by evaluating their reducing effect against oxidation of a redox sensitive fluorescent probe, 2′,7′-dichlorofluorescin (DCFH)-diacetate (DA), in the presence or absence of H2O2. The results were compared with a well-known antioxidant ascorbic acid and MLT, as their parent compound. Membrane stabilizing effect of all compounds was also investigated via lactate dehydrogenase (LDH) leakage assay in Chinese Hamster Ovary (CHO) cells. All the analogue compounds were characterized on the basis of 1H- and 13C-NMR, mass, Fourier transform infrared spectroscopy (FT-IR) spectra and elemental analysis. Furthermore voltammetric studies were established for the electrochemical oxidation and determination of synthesized MLT analogues using a glassy carbon electrode and investigated the possible oxido-reduction mechanisms that gave ideas about in vitro metabolism of these compounds.
Material and methods
With this study based on MLT, series of new indole imines were developed. Three parts of the MLT molecule were modified in order to find out the antioxidant behavior and structure activity relationship of the new indole analogue compounds. The main modifications of MLT molecule were shown in . The target imines derived from 1-methylindole-2-carboxaldehyde and appropriate hydrazine or hydrazide derivatives using simple reaction strategies. For the synthesis of compounds 1a-p a similar methodology has been adopted from Kidwai et al. Citation30. Phenyl hydrazine derivatives and 1-methylindole-2-carboxaldehyde were heated in the presence of ethanol. The hydrazones 1t, 1u were prepared from the reaction of equimolar amounts of hydrazide with 1-methylindole-2-carboxaldehyde in the presence of ethanol. Finally compound 1v was synthesized using equimolar amounts of hydrazine hydrate with 1-methylindole-2-carboxaldehyde and compound 1y was synthesized using one equimolar of hydrazine hydrate with two equimolars 1-methylindole-2-carboxaldehyde in the presence of ethanol (). All the compounds were characterized on the basis of spectral data.
Figure 1. Parts of the MLT molecule modified to develop new indole-based MLT analogue compounds. MLT, melatonin.
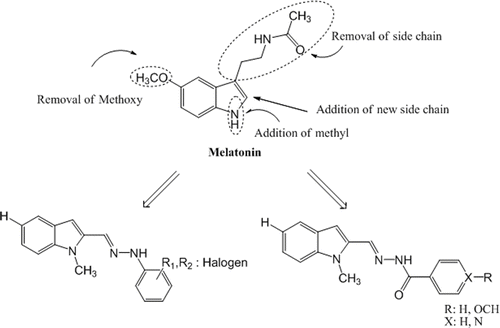
The chemical reagents used in synthesis were purchased from Sigma (Taufkirchen, Germany) and Aldrich (St. Louis, MO, USA). Uncorrected melting points were determined with a Buchi SMP-20 apparatus. The 1H- and 13C-NMR spectra were measured with a Varian 400MHz using TMS internal standard and DMSO-d6 as solvent. ESI Mass spectra were determined on a Waters micromass ZQ.FT-IR spectra were recorded on Jasco 420Fourier. Elemental analyses were performed using CHNS-932 (LECO). All spectral analysis was performed at Ankara University, Faculty of Pharmacy, Central Laboratory. Voltammetric measurements were performed using a Bioanalytical Systems (BAS 100 W, USA) electrochemical analyzer.
General procedure for the synthesisof compounds 1a-p.
1-Methylindole-2-carboxaldehyde (0.1 mmol) was reacted with phenyl hydrazine or its derivatives (0.13 mmol) in 10 ml of ethanol in the presence of 0.5 g CH3COONa for 30 min on the hot water bath. On cooling, the precipitate was collected washed with cold ethanol to give 1a-p with 22–91% yield.
1-Methylindole-2-carboxaldehyde phenylhydrazone (1a) Yield 41.1%; m.p. 185–186°C; 1H-NMR: 4.06(3H, s), 6.70(1H, s), 6.76(1H, m), 7.04(3H, m), 7.21(3H, m), 7.50(2H, dd), 8.03(1H, s, azomethine CH), 1038(1H, s, hydrazine NH); 13C-NMR: 32.55, 104.41, 110.31, 112.56, 119.50, 120.32, 120.94, 122.87, 127.91, 129.93, 131.03, 135.79, 139.58, 145.82 (azomethine CH); ESI MS m/z 250 (M + 1, %100), 251 (M + 2); Anal. Calcd. For C16H15N3: C, 77.07%; H, 6.07%; N, 16.86%. Found: C, 76.11%; H, 6.35%; N, 16.28%. FT-IR (KBr) cm−1 1603 (C=N azomethine stretch), 3302 (N–H stretch).
1-Methylindole-2-carboxaldehyde (2- fluorophenyl)hydrazone (1b) Yield 54.3%; m.p. 157–158°C; 1H-NMR: 4.06(3H,s), 6.728(1H, s), 6.78(1H, m), 7.12(4H, m), 7.49(3H, m), 8.32(1H, s, azomethine), 10.29(1H, s, hydrazine); 13C-NMR: 32.53, 104.94, 110.39, 114.33, 115.66, 115.84, 119.32, 120.36, 121.09, 123.09, 125.80, 127.82, 133.88, 134.04, 135.47, 139.63, 148.61 (azomethine C), 150.98; ESI MS m/z 268 (M + 1, %100), 269 (M + 2); Anal. Calcd. For C16H14N3F: C, 71.88%; H, 5.28%; N, 15.73%. Found: C, 71.22%; H, 5.60%; N, 15.29%. FT-IR (KBr) cm−1 1623 (C=N azomethine stretch), 3320 (N–H stretch).
1-Methylindole-2-carboxaldehyde (3- fluorophenyl)hydrazone (1c) Yield 48.9%; m.p. 153–154°C; 1H-NMR: 4.07(3H, s), 6.79(3H, m), 7.05(1H, t), 7.23(2H, m), 7.51(2H, dd), 8.05(1H, s, azomethine CH), 10.61(1H, s, hydrazine NH); 13C-NMR: 31.75, 98.14, 98.41, 104.28, 104.65, 104.87, 107.98, 109.58, 119.57, 120.28, 122.30, 127.01, 130.73, 131.49, 134.59, 138.85, 147.01(azomethine C), 161.99, 162.09, 164.48; ESI MS m/z 268 (M + 1, %100), 269 (M + 2); Anal. Calcd. For C16H14N3F: C, 71.88%; H, 5.28%; N, 15.73%. Found: C, 70.99%; H, 5.46%; N, 15.12%. FT-IR (KBr) cm−1 1609 (C=N azomethine stretch), 3305 (N–H stretch).
1-Methylindole-2-carboxaldehyde (4- fluorophenyl)hydrazone (1d) Yield 51.1%; m.p. 174–175°C; 1H-NMR: 4.05(3H, s), 6.70(1H, s), 7.11(6H, m), 7.50(2H, dd), 8.02(1H, s, azomethine CH), 10.39(1H, s, hydrazine NH); 13C-NMR: 32.51, 104.41, 110.29, 113.51, 116.30, 116.53, 120.29, 120.92, 122.85, 127.86, 131.08, 135.70, 139.54, 142.51 (azomethine C), 155.39, 157.72; ESI MS m/z 268 (M + 1, %100), 269 (M + 2); Anal. Calcd. For C16H14N3F: C, 71.88%; H, 5.28%; N, 15.73%. Found: C, 71.22%; H, 5.40%; N, 15.22%. FT-IR (KBr) cm−1 1609 (C=N azomethine stretch), 3298 (N–H stretch).
1-Methylindole-2-carboxaldehyde (2,4-difluorophenyl)hydrazone (1e) Yield 27.4%; m.p. 159–160°C; 1H-NMR: 4.05(3H, s), 6.73(1H, s), 7.04(2H, m), 7.23(2H, m), 7.47(3H, m), 8.30(1H, s, azomethine CH), 10.26(1H, s, hydrazine CH); ESI MS m/z 286 (M + 1, %100), 287 (M + 2); Anal. Calcd. For C16H13N3F2: C, 67.34%; H, 4.60%; N, 14.73%. Found: C, 66.62%; H, 4.74%; N, 14.40%. FT-IR (KBr) cm−1 1602 (C=N azomethine stretch), 3317 (N–H stretch).
1-Methylindole-2-carboxaldehyde (2,5-difluorophenyl)hydrazone (1f) Yield 61.9%; m.p. 168–169°C; 1H-NMR: 4.05(3H, s), 6.55(1H, m), 6.78(1H, s), 7.14(4H, m), 7.53(2H, dd), 8.35(1H, s, azomethine CH), 10.35(1H, s, hydrazine NH); ESI MS m/z 286 (M + 1, %100), 287 (M + 2); Anal. Calcd. For C16H13N3F2: C, 67.34%; H, 4.60%; N, 14.73%. Found: C, 67.10%; H, 4.58%; N, 14.30%. FT-IR (KBr) cm−1 1631 (C=N azomethine stretch), 3325 (N–H stretch).
1-Methylindole-2-carboxaldehyde (3,5-difluorophenyl)hydrazone (1g) Yield 59.9%; m.p. 183–184°C; 1H-NMR: 4.02(3H, s), 6.54(1H, m), 6.61(2H, dd), 6.77(1H, s), 7.03(1H,t), 7.18(1H, m), 7.49(2H, dd), 8.05(1H, s, azomethine CH), 10.78(1H, s, hydrazine NH); ESI MS m/z 286 (M + 1, %100), 287 (M + 2); Anal. Calcd. For C16H13N3F2: C, 67.34%; H, 4.60%; N, 14.73%. Found: C, 67.03%; H, 4.88%; N, 14.30%. FT-IR (KBr) cm−1 1631 (C=N azomethine stretch), 3305 (N–H stretch).
1-Methylindole-2-carboxaldehyde (2-chlorophenyl)hydrazone (1h) Yield 56.3%, m.p. 136.5-137.5°C; 1H-NMR: 4.03(3H, s), 6.77(2H, m), 7.03(1H,t), 7.25(3H, m), 7.47(3H, d. dd), 8.47(1H, s, azomethine-CH), 9.23(1H, s, hydrazine-NH); 13C-NMR: 32.49, 105.04, 110.43, 114.44, 116.82, 120.29, 120.39, 121.14, 123.17, 127.78, 128.86, 130.09, 134.86, 135.37, 139.60, 141.91 (azomethine C); ESI MS m/z 284.7 (M + 1, %100), 286.7 (M + 3); Anal. Calcd. For C16H14N3Cl: C, 67.71%; H, 4.98%; N, 14.81%. Found: C, 67.37%; H, 5.16%; N, 14.64. FT-IR (KBr) cm−1 1591 (C=N azomethine stretch), 3316 (N–H stretch).
1-Methylindole-2-carboxaldehyde (3-chlorophenyl)hydrazone (1i) Yield 28.1%, m.p. 172–173°C; 1H-NMR: 4.05(3H, s), 6.78(2H,m), 6.96(1H,m), 7.05(2H,m), 7.22(2H, m), 7.51(2H, dd), 8.05(1H, s, azomethine-CH), 10.59(1H, s, hydrazine-NH). 13C-NMR: 32.49, 105.05, 110.37, 111.29, 111.82, 118.83, 120.36, 121.07, 123.09, 127.78, 131.54, 132.45, 134.56, 135.33, 139.62, 147.25 (azomethine C); ESI MS m/z 284.41(M + 1, %100), 286.56 (M + 3); Anal. Calcd. For C16H14N3Cl: C, 67.71%; H, 4.98%; N, 14.81%. Found: C, 67.24%; H, 5.27%; N, 14.77%. FT-IR (KBr) cm−1 1599 (C=N azomethine stretch), 3297 (N–H stretch).
1-Methylindole-2-carboxaldehyde (4-chlorophenyl)hydrazone (1j) Yield 91.5%, m.p. 198–199°C; 1H-NMR: 4.05(3H, s), 6.73(1H, s), 7.04(3H, m), 7.23(3H, m), 7.51(2H, dd), 8.04(1H, s, azomethine-CH), 10.53(1H,s, hydrazine-NH); 13C-NMR: 32.52, 104.80, 110.34, 113.99, 120.33, 121.00, 122.65, 127.82, 129.69, 131.86, 135.49, 139.60, 144.73 (azomethine C); ESI MS m/z 284.7(M + 1, %100), 286.8 (M + 3); Anal. Calcd. For C16H14N3Cl: C, 67.71%; H, 4.98%; N, 14.81%. Found: C, 67.51%; H, 5.26%; N, 14.37%. FT-IR (KBr) cm−1 1599 (C=N azomethine stretch), 3304 (N–H stretch).
1-Methylindole-2-carboxaldehyde (2,4-dichlorophenyl)hydrazone (1k) Yield 24.7%, m.p. 143–144°C; 1H-NMR: 4.04(3H, s), 6.78(1H, s), 7.06(1H, m), 7.21(1H, m), 7.34(1H, dd), 7.25(4H, m), 8.51(1H, s, azomethine), 10.09(1H, s, hydrazine); 13C-NMR: 32.49, 105.39, 110.48, 115.43, 117.28, 120.42, 121.22, 122.81, 123.30, 127.74, 128.87, 129.32, 135.15, 135.59, 139.64, 141.11 (azomethine C); ESI MS m/z 318(M + ,%100), 320 (M + 2); Anal. Calcd. For C16H13N3Cl2: C, 60.37%; H, 4.12%; N, 13.21%. Found: C, 60.20%; H, 4.16%; N, 13.00%. FT-IR (KBr) cm−1 1593 (C=N azomethine stretch), 3309 (N–H stretch).
1-Methylindole-2-carboxaldehyde (3,4-dichlorophenyl)hydrazone (1l) Yield 40.9%; m.p. 220–221°C; 1H-NMR: 4.04(3H, s), 6.79(1H, s), 7.02(2H, m), 7.21(2H, m), 7.50(3H, m), 8.06(1H, S, azomethine), 10.68(1H, s, hydrazine); 13C-NMR: 31.70, 104.56, 109.64, 112.12, 112.64, 119.31, 119.61, 120.35, 122.42, 126.96, 130.94, 131.52, 132.34, 134.34, 138.88, 145.06 (azomethine); ESI MS m/z 318(M +, %100), 320 (M + 2); Anal. Calcd. For C16H13N3Cl2: C, 60.37%; H, 4.12%; N, 13.21%. Found: C, 60.05%; H, 4.19%; N, 12.84%. FT-IR (KBr) cm−1 1589 (C=N azomethine stretch), 3300 (N–H stretch).
1-Methylindole-2-carboxaldehyde (3,5-dichlorophenyl)hydrazone (1m) Yield 22.0 %; m.p. 173.5–174.5°C; 1H-NMR: 4.04(3H, s), 6.82(1H, s), 6.87(1H, t), 6.98(2H, d), 7.06(1H,t), 7.21(1H, m), 7.52(2H, dd), 8.07(1H, s, azomethine), 10.76(1H, s, hydrazine); 13C-NMR: 32.46, 105.67, 110.45, 110.75, 118.02, 120.43, 121.22, 123.34, 127.71, 133.83, 134.93, 139.70, 147.97(azomethine); ESI MS m/z 318(M+, %100), 320 (M + 2); Anal. Calcd. For C16H13N3Cl2: C, 60.37%; H, 4.12%; N, 13.21%. Found: C, 60.34%; H, 4.38%; N, 13.01%. FT-IR (KBr) cm−1 1580 (C=N azomethine stretch), 3306 (N–H stretch).
1-Methylindole-2-carboxaldehyde (2-bromophenyl)hydrazone (1n) Yield 34.8%; m.p. 134–135°C; 1H-NMR: 4.05(3H, s), 6.75(2H, m), 7.05(1H, t), 7.21(1H, m), 7.32(1H, m), 7.52(4H, m), 8.53(1H, s, azomethine CH), 9.72(1H, s, hydrazine NH); 13C-NMR: 32.46, 105.00, 106.68, 110.44, 114.91, 120.39, 121.00, 121.16, 123.17, 127.78, 129.40, 133.32, 135.04, 135.38, 139.60, 142.96(azomethine C); ESI MS m/z 328 (M+, %100), 287 (M + 2), 330 (M + 2), 331 (M + 3); Anal. Calcd. For C16H14N3Br: C, 58.53%; H, 4.30%; N, 12.80%. Found: C, 58.36%; H, 4.51%, N, 12.69%. FT-IR (KBr) cm−1 1602 (C=N azomethine stretch), 3305 (N–H stretch).
1-Methylindole-2-carboxaldehyde (3-bromophenyl)hydrazone (1o) Yield 44.5%; m.p. 177–178°C; 1H-NMR: 4.05(3H, s), 6.76(1H, s), 6.91(1H, t), 7.03(2H, m), 7.19(3H, m), 7.51(2H, dd), 8.05(1H, s, azomethine CH), 10.56(1H, s, hydrazine NH); 13C-NMR: 32.48, 105.04, 110.38, 111.65, 114.72, 120.36, 121.73, 123.10, 123.15, 127.79, 131.85, 132.48, 135.33, 139.63, 147.37 (azomethine C); ESI MS m/z 328 (M+, %100), 287 (M + 2), 330 (M + 2), 331 (M + 3); Anal. Calcd. For C16H14N3Br: C, 58.53%; H, 4.30%; N, 12.80%. Found: C, 57.88%; H, 4.49%; N, 12.33%. FT-IR (KBr) cm−1 1590 (C=N azomethine stretch), 3296 (N–H stretch).
1-Methylindole-2-carboxaldehyde (4-bromophenyl)hydrazone (1p) Yield 87.2%; m.p. 207–208°C; 1H-NMR: 4.05(3H, s), 6.73(1H, s), 6.91(1H, t), 7.02(2H, m), 7.19(3H, m), 7.46(4H, m), 8.04(1H, s, azomethine CH), 10.53(1H, s, hydrazine NH); 13C-NMR: 32.52, 104.83, 110.22, 110.34, 114.51, 120.34, 121.01, 123.01, 127.82, 131.95, 132.51, 135.48, 139.61, 145.10 (azomethine C); ESI MS m/z 328 (M + ,%100), 287 (M + 2), 330 (M + 2); Anal. Calcd. For C16H14N3Br: C, 58.53%; H, 4.30%; N, 12.80%. Found: C, 58.49%; H, 4.51%; N, 12.62%. FT-IR (KBr) cm−1 1590 (C=N azomethine stretch), 3306 (N–H stretch).
1-Methylindole-2-carboxaldehyde (2,3-dimethylphenyl)hydrazone (1r) Yield 73.9%, m.p. 151–152°C; 1H-NMR: 2.14(3H, s), 2.24(3H, s), 4.06(3H, s), 6.67(2H, t), 7.03(2H, m), 7.50(2H,dd), 8.34(1H, s, azomethine-CH), 9.59(1H, s, hydrazine-NH); 13C-NMR: 12.62, 20.11, 31.68, 103.36, 109.50, 110.17, 119.04, 119.50, 120.12, 120.85, 122.02, 125.93, 127.13, 131.58, 135.174, 136.28, 138.73, 142.92 (azomethine-C); ESI MS m/z 278 (M + 1, %100), 279 (M + 2); Anal. Calcd. For C18H19N3:C, 77.39%; H, 6.91%; N, 15.16%. Found: C, 76.83%; H, 6.78%; N, 13.98. FT-IR (KBr) cm−1 1586 (C=N azomethine stretch), 3338 (N–H stretch).
1-Methylindole-2-carboxaldehyde (2,4- dimethylphenyl)hydrazone (1s) Yield 50.7%, m.p. 146–147°C; 1H-NMR: 2.20(3H, s), 3.35(3H, s), 4.05(3H, s), 6.69(1H,s), 6.92(2H,t), 7.04(1H, m), 7.20(2H, m), 7.50(2H, dd), 8.30(1H, s, azomethine-CH), 9.52(1H, s, hydrazine-NH); 13C-NMR: 18.12, 20.87, 32.43, 103.96, 110.26, 112.62, 120.27, 120.87, 121.32, 122.74, 127.91, 128.00, 131.67, 131.92, 135.99, 139.48, 141.46 (azomethine-C); ESI MS m/z 278 (M + 1, %100), 279 (M + 2); Anal. Calcd. For C18H19N3:C, 77.39%; H, 6.91%; N, 15.16%. Found: C, 77.50%; H, 7.13%; N, 14.59%. FT-IR (KBr) cm−1 1615 (C=N azomethine stretch), 3307 (N–H stretch).
General procedure for the synthesis of compounds 1t,1u
A solution of 1-methylindole-2-carboxaldehyde (0.05 mmol) and izonicotinic acid hydrazide (for 1t) or anisic acid hydrazide (for 1u) (0.05 mmol) in 50 mL of ethanol was heated for 2.5 h on the hot water bath. On cooling, the precipitate was collected washed with cold ethanol to give 1t and 1u with 12–16% yield.
1-Methylindole-2-carboxaldehyde izonicotynoilhydrazone (1t) Yield 16.5%; m.p. 190–191°C; 1H-NMR: 4.08(3H, s), 6.95(1H, s), 7.07(1H, t), 7.25(1H, t), 7.55(2H, m), 7.82(2H, d), 8.59(1H, s, azomethine CH), 8.75(2H, d), 12.06(1H, s, hydrazine NH); ESI MS m/z 279 (M + 1, %100), 280 (M + 2); Anal. Calcd. For C16H14N4O: C, 69.04%; H, 5.07%; N, 20.14%. Found: C, 66.80%; H, 5.65%; N, 17.84%. FT-IR (KBr) cm−1 1649 (C=N azomethine stretch), 3320 (N–H stretch).
N-(4-methoxybenzoyl)-N′-(1-methylindolyl-2-methylene)-hydrazine (1u) Yield 12.4%; m.p. 199–200°C; 1H-NMR: 3.82(3H, s), 4.07(3H, s), 6.88(1H, s), 7.06(3H, m), 7.23(1H, t), 7.53(2H, dd), 7.91(2H, d), 8.57(1H, s, azomethine CH), 11.71(1H, s, hydrazine NH); ESI MS m/z 308 (M + 1, %100), 309 (M + 2); Anal. Calcd. For C18H17N3O2: C, 70.33%; H, 5.58%; N, 13.68%. Found: C, 68.40%; H, 6.42%, N, 12.42%. FT-IR (KBr) cm−1 1647 (C=N azomethine stretch), 3327 (N–H stretch).
General procedure for the synthesis of compounds 1v,1y
1-Methylindole-2-carboxaldehyde (0.1 mmol) and hydrazine hydrate (0.1 mmol for 1v, 0.2 mmol for 1y) in 25 mL of ethanol was heated for 4 h on the hot water bath. On cooling, the precipitate was collected washed with cold ethanol to give 1v and 1y with 56–57% yield.
N,N′-bis-(1-methylindole-2-ylmethylene)hydrazine (1v) Yield 56.0%; m.p. 297–298°C; 1H-NMR: 4.16(6H, s), 7.13(4H, m), 7.31(2H, t), 7.62(2H, dd), 8.90(2H, s, azomethine CH); ESI MS m/z 315 (M + 1, %100), 316 (M + 2), 317 (M + 3); Anal. Calcd. For C20H18N4: C, 76.4%; H, 5.58%; N, 13.68%. Found: C, 68.40%; H, 6.42%; 12.42%. FT-IR (KBr) cm−1 1627 (C=N azomethine stretch).
1-Methylindole-2-carboxaldehyde hydrazone (1y) Yield 57.1%; m.p. 124–125°C; 1H-NMR: 4.69(3H, s), 7.30(1H, s), 7.66(2H, s, hydrazine NH), 7.90(1H, t), 7.92(1H, s), 8.19(1H, d), 8.27(1H, d), 8.67(1H, s, azomethine CH); 13C-NMR: 32.80, 103.11, 110.95, 120.91,121.49,123.10, 128.71, 133.43, 137.31, 139.89(azomethine C); ESI MS m/z 174 (M + 1, %100); Anal. Calcd. For C10H11N3: C, 69.33%; H, 6.40%; N, 24.27%. Found: C, 69.34%; H, 6.79%; N, 23.34%. FT-IR (KBr) cm−1 1463 (C=N azomethine stretch), 3355 (N–H stretch).
Biological activity studies
Antioxidant activity on ROS-induced DCFH-DA oxidation
For estimation of ROS inside cells DCFH-DA was used as a fluorescent probe. In cellular systems non fluorescent DCFH-DA readily crosses the cell membrane and undergoes hydrolysis by intracellular esterases to nonfluorescent DCFH. DCFH is then rapidly oxidized in the presence of reactive oxygen species to highly fluorescent DCFHCitation31. In the present study, human erythrocytes were used to evaluate the potential antioxidant effect of test compounds. The assay is performed as detailed in Shirinzadeh et al.Citation13. Effects of the compounds (100 μM) on DCFH oxidation were investigated after incubation of the cells with test compounds alone or after co-incubation of the cells with test compounds and H2O2 (10 μM) as a known reactive oxygen species. Antioxidant effects of the compounds were compared to the effect of ascorbic acid (100 μM) as a well-known antioxidant and melatonin (MLT) (100 μM) as their parent compound.
Membrane stabilizing effect; LDH leakage
CHO-K1 cells were cultured in FC-1: Dulbecco’s Modified Eagles Medium (1:1), supplemented with 10% foetal bovine serum, 1% of a 100 U/ml penicillin-streptomycin solution, 2 mM (final concentration) l-glutamine and 1 mM (final concentration) sodium pyruvate at 37°C, 5% CO2. This medium was used in all cell incubations. Cytotoxic effects of the compounds were evaluated via LDH activity assay according to the method of Hassoun et al.Citation32 with a minor modification. The activity of LDH in 100 μL of media was determined by direct calculation based on the decrease in absorbanceCitation33.
Electrochemical measurements
The cyclic, linear sweep, differential pulse voltammetric (DPV) and square wave voltammetric (SWV) measurements at a glassy carbon electrode were performed using a BAS 100W (Bioanalytical System, Los Angeles, California, USA) electrochemical analyzer. The three electrode system a glassy carbon working electrode (BAS; Ø: 3 mm diameter), with a platinum wire counter electrode and Ag/AgCl reference electrode (KCl 3M, BAS) and a standard one-compartment three-electrode cell of 10 mL capacity. For analytical application, the following parameters were employed: SWV amplitude, 25 mV; frequency, 15 Hz; scan increment, 4 mV. DPV parameters were: pulse amplitude, 50 mV; pulse width 50 ms; scan rate, 20 mV s−1. The pH values were measured using a pH meter Model 538 (WTW, Wien, Austria) using a combined electrode (glass electrode reference electrode) with an accuracy of ±0.05 pH. A stock solution of the selected synthesized indoles was prepared daily by direct dissolution in methanol. The working solutions were prepared by the dilution of the stock solution with selected supporting electrolytes contained different percent of methanol. Four different supporting electrolytes, namely sulfuric acid (0.1 M), phosphate buffer (0.2 M, pH 2.0–8.0), acetate buffer (0.2 M, pH 3.7–5.7), and Britton–Robinson buffer (0.04 M, pH 2–12) were prepared in double distilled water. All solutions were protected from light and were used within 24 h to avoid decomposition.
Results and discussion
Antioxidant effects on ROS-induced DCFH-DA oxidation
The protective effect of newly synthesized MLT analogues against DCFH-DA oxidation was determined in human erythrocytes that were preloaded with the fluorescent probe. Oxidation of the probe was screened at 10 min intervals up to 60 min. DCFH oxidation at 60 min incubation is given for cells exposed to the test compound alone () and for cells co-exposed to H2O2 and the test compounds (). The aim of the second model () is to evaluate the antioxidant capacity of the synthesized MLT analogs in a ROS challenge situation. Almost all compounds were found to exhibit higher antioxidant activity when they are challenged with H2O2. Comparing to the results from our previous studyCitation13, N-methylation of the indole ring decreased the antioxidant capacity of all MLT derivatives. Similar to our previous resultsCitation13 among mono-halogenated derivatives p-halogenation was found to decrease antioxidant activity compared to o- and m-substitution of the same halogen atom. 1t, 1u, and 1y had the weakest antioxidant activity in both cases (with or without H2O2). This can be explained by having no aromatic halogenations or a carbonyl group on the side chain.
Figure 3. Oxidation of DCFH via reactive oxygen species in erythrocytes after incubation with ascorbic acid, MLT and newly synthesized analogs for 60 min. Control group represents cells incubated with PBS without any compound addition. Values are mean ± SD of three individual experiments. *p < 0.05, compared to control group. **p < 0.005, compared to control group. #p < 0.0005, compared to control group. MLT, melatonin.
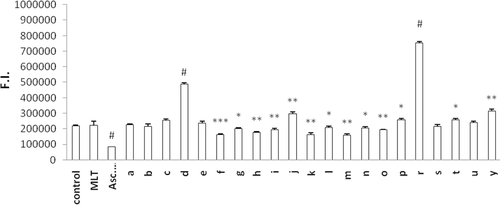
Figure 4. Antioxidant effect of indole-analogs on H2O2-induced oxidation of DCFH in erythrocytes. Control group represents cells incubated with PBS without any compound addition. Values are mean ± SD of three individual experiments. §p < 0.005, compared with control. *p < 0.05, compared with H2O2 group. **p < 0.005, compared with H2O2 group. #p < 0.0005, compared with H2O2 group.
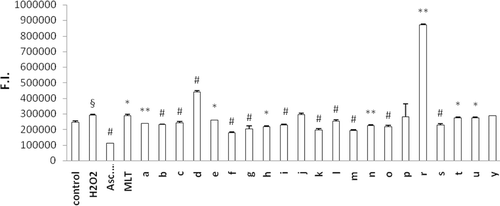
Membrane stabilizing effect; LDH leakage
Lipid peroxidation is known to change membrane fragility, motility and permeabilityCitation34. LDH is a cytoplasmic enzyme and its leakage from injured cells into the culture medium has been shown to be useful as an indicator of cellular membrane damage. In the present study, we determined LDH activity in the culture medium in order to assess the membrane stabilizing effect of MLT analogues. No increases in LDH activity were observed with any of the tested compounds. Even several compounds (1b, 1g, and 1t) were found to decrease LDH leakage indicating membrane stabilizing effect. This assay is also an indicator of a possible cytotoxic effect of the test compounds by means of inducing membrane damage. From this point of view, as possible drug candidates, we can conclude that none of the synthesized MLT analogs are found to have cytotoxic effect. In accordance with the antioxidant assay ( and ) 1d and 1r were found to have the highest, but statistically not significant, LDH activity in media (). This finding can be explained by the increased ROS formation with 1d and 1r analogs resulted in membrane damage and leakage of the LDH enzyme into the media.
Figure 5. Effect of MLT analogs on LDH leakage from CHO cells into the culture media. Control group represents cells incubated in regular media without any compound addition. % 2.5 DMSO is used to cause membrane damage where ascorbic acid is used as an antioxidant. *p < 0.05, **p < 0.005 compared with control.
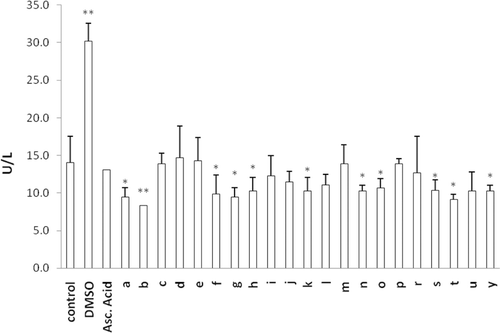
Electrochemical measurements
In this study the measurements from the selected indole derivatives are two-dimensional, with the potential being related to qualitative properties and current related properties. In order to characterize the electrochemical process that occurs on the glassy carbon electrode, both pH, nature of the buffer and scan rate studies were carried out in the potential range from −0.25 to 1.6 V. Cyclic voltammetry (CV) experiments were achieved over a range from acidic (0.1M H2SO4) to alkaline (pH 12.00) in acidic solutions and different buffer aqueous media. For the quantitative determination, DPV and SWV methods were applied to the selected synthesized derivatives [Citation35–37]. For the synthesized compounds, the peak currents and peak potentials were determined in different supporting electrolytes such as H2SO4, phosphate, acetate, and Britton-Robison buffers containing 40, 50, 20, and 30% methanol (v/v) to maintain solubility for 1f, 1g, 1y, and 1u, respectively. The compounds were selected as two of the most active and structurally similar compounds 1f, 1g, and structurally different compounds 1y and 1u. Therefore, several electrochemical measurements using cyclic, linear sweep, differential pulse, and square wave voltammetric methods were performed with various supporting electrolytes and buffers in order to obtain such information.
As first step, compounds 1f, 1g, 1y, and 1u were subjected to a cyclic and linear sweep voltammetric studies with the aim to characterize of their electrochemical oxidation behavior in details on the glassy carbon electrode over a wide pH range (1.5–12.0). Voltammograms exhibited one distinct and well defined main anodic peak (Ox1) and/or one ill-defined anodic wave at different potential values depending on pH values and supporting electrolyte composition ( and ). In addition to this first oxidation step, the second oxidation response was obtained when working on compound 1u depending on the methoxy benzene oxidation (Ox2). CV measurements showed an irreversible nature of the oxidation process. In acidic media, the anodic oxidation did not occur until about +0.50 V for all selected compounds on glassy carbon electrode (). By reversing at +1.80 V no reduction signal corresponding to the anodic response was observed on the cathodic branch in all pH values (). The anodic oxidation peaks of the selected indolic compounds decreased to the second or higher cycles ( and ). This phenomenon may be partly attributed to the consumption of adsorbed indolic compounds on the electrode surface.
Figure 6. CV voltammograms of 20 µg/mL 1f and 10 µg/mL 1g, 1y, and 1u in 0.1 M H2SO4. (A,B,C, and D): (A) 20 µg/mL 1f, 40% MeOH (B) 10 µg/mL 1g, 50% MeOH(C) 10 µg/mL 1y 20% MeOH (D) 10 µg/mL 1u 30% MeOH.
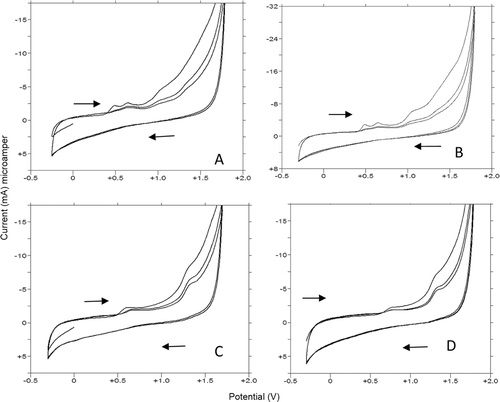
Figure 7. CV voltammograms of 1f, 1g, 1y, and 1u. (A,B,C, and D): (A) 20 µg/mL 1f, pH 6 FT (B) 10 µg/mL 1g, pH 4.7 AT (C) 10 µg/mL 1y, pH 7.0 BRT (D) 10 µg/mL 1u, pH 10 BRT.
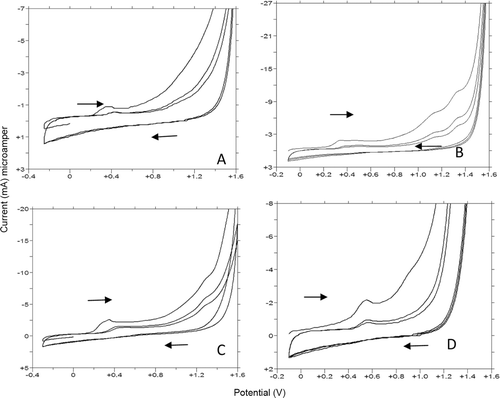
The effect of the potential scan rate varied between 5 and 1000 mV s−1 on the peak current and potential of the selected indolic compounds were evaluated. The different amounts shifts in the peak potentials confirmed the irreversibility of the oxidation processes of these compounds (). Scan rate studies were carried out to assess whether the processes at the glassy carbon electrode was under diffusion or adsorption control for the first and/or second peaks. When the scan rate was varied between 5 and 1000 mV s−1 in 20 µg/mL solution of compound 1f, and 10 µg/mL solution of compounds 1g, 1y, and 1u a linear dependence of the peak intensity ip (µA) upon the square root of the scan rate ν1/2 (mV s−1) was found in all studied supporting electrolytes, demonstrating a diffusional behavior. The equation is noted in for all compounds and all studied media. All the equations gave a straight line with a slope values between 0.36 and 0.66, very close to the theoretical value of 0.5, which is expressed for an ideal reaction the diffusion controlled electrode processCitation38. Nonetheless, the peak potentials shifted to the more positive potentials to the anodic direction when the scan rate was increased () in all supporting electrolyte.
Table 1. The results of the scan rates experiments on 1f, 1g, 1y, and 1u.
The effect of pH on the peak potential and intensity were investigated between pH 2.0 and 12.0 for all selected indole compounds using CV, DPV, and SWV techniques. All obtained results from CV, DPV, and SWV were found similar. For this reason, only DPV results for the obtained oxidation steps were given as below for Ep–pH equations:
Ep (mV) = 664.25 − 55.67 pH; r = 0.998, n = 11 (for 1f, between pH 2 and 12)
Ep (mV) = 497.02 − 50.78 pH; r = 0.997, n = 10 (for 1g, between pH 2 and 12)
Ep (mV) = 632.68 − 42.40 pH; r = 0.999, n = 6 (for 1y, between pH 1 and 12)
Ep (mV) = 915.82 − 45.03 pH; r = 0.981, n = 8 (for 1u, Ox1, between pH 2 and 12)
Ep (mV) = 1293.90 − 18.63 pH; r = 0.994, n = 5 (for 1u, Ox2, between pH 1 and 6)
The peak potential of the anodic process moved to less positive potential values and an ill-defined oxidation wave disappeared by increasing pH. In all instances and techniques, the indole compounds undergoes one main irreversible oxidation process and/or one additional ill-defined wave, which shifted towards less positive potentials as the pH was increased. When working on compound 1u, one additional oxidation step was obtained depending on the apart from indole oxidation, which is occurred by the methoxy benzene oxidation (Ox2).
According to the slopes of the above equations are found between 42.40 and 55.67 mV/pH for the main oxidation process (Ox1) of all studied compounds. These slopes are found close to the expected theoretical value of 59 mV/pH. For the second oxidation step of compound 1u, the slope value was obtained as 18.63 mV/pH. These slopes are found close to the expected theoretical value of 30 mV/pH. According to the obtained slope values of these equations, same amount of electrons and protons are involved in the rate-determining steps. The experimental results showed that shapes of the curves and maximum peak current were obtained in different supporting electrolytes and pH values with constant amount of methanol, which indicated in . The main peak that appeared with less positive potential was the best developed and became sharper and occurred as without shoulder in these supporting electrolytes. For this reason, the indicated supporting electrolytes in were chosen with respect to have sharp response and better peak shape for the calibration equation.
Table 2. Calibration parameters of 1f, 1g, 1y, and 1u.
Although the exact oxidation mechanism was not determined, some conclusions about the potentially electroactive centers under working conditions could be reached. From the CV curves, the main voltammetric behavior of indole and methoxy benzene derivatives, which are structurally related to the mechanism of oxidation of synthesized indole compounds, may be postulated by the oxidation of these groupsCitation26–29,Citation35–41. Our results on model compounds show similar behavior that the electroactive center corresponding to the main anodic response was the nitrogen atom on the indole ring. In addition to, the methoxy benzene anodic response in compound 1u was obtained as the second oxidation step. Indole and its derivatives are extensively metabolized in vivoCitation26–29,Citation36,37. To support the working hypothesis that indole moiety (similar oxidation pathway with indol-3-substituted derivative) and methoxy benzene moiety (similar to phenolic group oxidation step) of compounds 1f, 1g, 1y, and 1u that undergoes oxidation, behavior of Step I (related to indole moiety) and Step II (related to methoxy benzene moiety) of the compounds were compared with similar model compounds. Comparative study on indole, synthesized indole compounds, indole-3-acetic acid, etodolac, fluvastatine, and ziprasidon were realized by CV at a function of pH in order to identify the oxidation process of the synthesized compounds. It was assumed that oxidation occurred firstly on the nitrogen atom of indole ring, which is electroactive in both acidic and basic media, leading finally to hydroxylation of the benzene ringCitation36–39,Citation42. The electrochemical oxidation of indole derivatives with a substituent presented similar features to the indole electroactive centre. It is a well-established fact that the benzene ring of indoles is less reactive than the pyrrole ring. Since all indole derivatives are substituted, the oxidation reaction taking place at the main peak (Ox1) corresponds to the oxidation at nitrogen on the pyrrole ringCitation43,Citation44. The cyclic voltammograms of the indole library and model compounds demonstrated that the oxidation potential peak values were close to those of the nonsubstituted indole nucleus, but higher than those described for the antioxidant melatonin. In addition, no reversibility was observed in the obtained voltammograms. The lack of reversibility is an advantage, meaning that once oxidized these species do not tend to receive electrons.
In addition to this step for 1u, second oxidation step was obtained because of the methoxy group on the benzene moiety of the molecule. Nearly in all pH values, both indole and methoxy benzene rings responses of 1u was clearly and separately obtained. The study on verapamil, tamsulosin, and other synthesized indole compounds was realized by CV at GC electrode as a function of pH in order to identify the responsible atom for the second oxidation process of 1u. Our results revealed a good agreement with the redox mechanism postulated for similar compounds such as 4-methoxyphenol, tamsulosin and verapamil suggested that tamsulosin can be determined electrochemically by oxidation of methoxybenzene groupsCitation40,Citation41,Citation45,Citation46.
Analytical applications and methods validation
In order to develop a voltammetric methodology for determining compound 1f, g, y, and u, DPV and SWV modes were selected. In this study SWV were used as an alternative technique. Various electrolytes such as sulphuric acid, Britton–Robinson, phosphate, and acetate buffer were examined as a supporting electrolyte. The best condition for analytical applications proved to be indifferent supporting electrolytes, which are indicated in . These supporting electrolytes with a constant amount of methanol were chosen for the subsequent experiments. The effects of methanol amount on peak current and potential were also studied. As expected, the peak current decreased with changing ionic strength and viscosity of the medium. Good correlations were obtained in different concentration ranges using both techniques, which were reported in . Above the linearity high limit concentration, a loss of linearity was probably due to the adsorption of the compounds on the electrode surface. The characteristics of the calibration plots are summarized in . The detection limit and quantification limit of the procedures () were calculated according to the 3 s/m and 10 s/m criterions, respectively, where s is the standard deviation of the peak currents (five runs) and m is the slope of related calibration graphCitation45–47. The low values of SE of slope and intercept and greater than 0.999 correlation coefficient nearly in all media established the precision of the proposed method. The developed methods were validated according to the standard procedures and the results obtained are shown in . Accuracy, precision and reproducibility of the proposed method were assessed by performing replicate analysis of the standard solutions in supporting electrolytes within the calibration curves. The within day and between day precision, accuracy and reproducibility were determined as the RSD% (relative standard deviation) and the results are shown in Citation31,Citation47,Citation48.
Conclusions
The present work aimed to synthesize, characterize investigate the potential antioxidant effects, and electrochemical evaluation of indole-based MLT analogue hydrazide/hydrazone derivatives. In general all the synthesized indole derivatives were found to have potent antioxidant activity, even higher than MLT itself, according to the results of in vitro antioxidant experiments revealing differences in their relative potencies probably related to electronic distribution. Having halogenated aromatic side chain increase the antioxidant activity. Antioxidant effect of indole-analogs on H2O2-induced oxidation of DCFH in erythrocytes showed that all analogues except 1d and 1r were effective.
We considered several possible interactions during the fluorescence measurements and performed several negative controls. We examined the fluorescence of untreated loaded cells. The healthy cells exhibited lower levels of fluorescence that was relatively stable during the experiment (with a slight gradual increase possibly due to auto oxidation) to make sure that the cells are not progressing to death or another oxidative event is not taking place. The fluorescence intensity of the compounds in the same test environment without DCFH was analyzed to assure that the added compounds are not giving any fluorescence at the same excitation and emission wavelengths (which can cause false positive results). None of the compounds were found to have any. Finally in order to make sure that added compounds do not have a quenching effect we examined the absorbance spectrum of the compounds and determined that the absorbance peaks were not overlapping with either the excitation and emission peak of the oxidized dye.
Although we have thought about the possible esterase inhibition because of the following reasons such an interaction for the present compounds is not possible. First of all two known esterases were identified in RBC cytosol; acetylcholinesterase and arylesterase. The esterases are reported to exhibit specificities for certain substrates and inhibitors but a drug is often hydrolysed by more than one esterase at different sites. Among the most common acetylcholinesterase inhibitors are phosphorus-based compounds, which are designed to bind to the active site of the enzyme. The structural requirements are a phosphorus atom bearing two lipophilic groups, a leaving group (such as a halide or thiocyanate), and a terminal oxygen50,51. As a conclusion none of the compounds that we tested are structurally thought to be an inhibitor of the esterases as well as even one of the isozyme or the type of the esterase in RBC cytosol is inhibited by any of our compound we think that there should still be enough activity to hydrolyse DCFH-DA.
Structural investigation of the rest of the active compounds showed that having o- and m-halogenated aromatic side chain increase the antioxidant activity (such as compounds 1f, 1k, and 1m). These are the most promising compounds that should be kept in mind for designing new MLT-based indole derivatives for our ongoing study.
Lack of a methoxy group in the five positions did not affect the antioxidant capacity of the new indole derivatives. In fact, the in vitro assays showed that lack of a methoxy group, introduction of a methyl group at the nitrogen in the indole ring and a halogenated aromatic side chain resulted in much more active compounds than MLT itself. This may be due to increased stability of the indole ring and delocalization of the electrons to help to scavenge free radicals by forming stable indolyl cation radicals.
The use of electrochemical techniques to study mechanisms directly with the electrodes and the determination of compounds of pharmaceutical interest is becoming a mature fieldCitation23.
Electrochemical mechanisms are important to all redox chemistry including biological systems usually concerning electron transport chains. Such reactions are most often studied with electrode techniques such as CVCitation35. In this study electrochemical behavior of selective MLT analogues through mechanistic studies was investigated. It was observed that oxidation occurred firstly on the nitrogen atom of indole ring leading finally to hydroxylation of the benzene ringCitation36–39,Citation42. This investigation gives some suggestions about the possible in vivo metabolism of newly synthesized compounds.
This study presents supportive data and will contribute for the design of indole-related drugs with improved antioxidant activity.
Declaration of interest
This work was supported by The Scientific and Technological Research Council of Turkey (TÜBİTAK) Research and Development Grant 109S099. Cell culture laboratory where the antioxidant activity studies performed was established with the TÜBİTAK Research and Development Grant 108S202.
The authors report no conflicts of interest.
References
- Singh RP, Sharad S, Kapur SJ. Free radicals and oxidative stress in neuro degenerative diseases: Relevance of dietary antioxidants. Ind Acad Clin Med 2004;4:218–225.
- Valko M, Leibfritz D, Moncol J, Cronin MT, Mazur M, Telser J. Free radicals and antioxidants in normal physiological functions and human disease. Int J Biochem Cell Biol 2007;39:44–84.
- Fresco P, Borges F, Diniz C, Marques MP. New insights on the anticancer properties of dietary polyphenols. Med Res Rev 2006;26:747–766.
- Suzen S. (2007). Topics in Heterocyclic Chemistry, Bioactive Heterocycles V. In: Khan MTH, ed. Antioxidant activities of synthetic indole derivatives and possible activity mechanisms. Berlin, Heidelberg: Spinger-Verlag,; 11, 145–178.
- Reiter RJ, Tan DX, Cabrera J, D’Arpa D, Sainz RM, Mayo JC et al. The oxidant/antioxidant network: role of melatonin. Biol Signals Recept 1999;8:56–63.
- Korkmaz A, Reiter RJ, Tan DX, Manchester LC. Melatonin; from pineal gland to healthy food. Spatula 2011;1:33–36.
- Cano A, Alcaraz O, Arnao MB. Free radical-scavenging activity of indolic compounds in aqueous and ethanolic media. Anal Bioanal Chem 2003;376:33–37.
- Andreadou I, Tsantili-Kakoulidou A, Spyropoulou E, Siatra T. Reactions of indole derivatives with cardioprotective activity with reactive oxygen species. Comparison with melatonin. Chem Pharm Bull 2003;51:1128–1131.
- Misik V, Ondrias K, Stasko A. EPR spectroscopy of free radical intermediates of antiarrhythmic-antihypoxic drug stobadine, a pyridoindole derivative. Life Sci 1999;65:1879–1881.
- Suzen S, Bozkaya P, Coban T, Nebiogu D. Investigation of the in vitro antioxidant behaviour of some 2-phenylindole derivatives: discussion on possible antioxidant mechanisms and comparison with melatonin. J Enzyme Inhib Med Chem 2006;21:405–411.
- Ates-Alagoz Z, Coban T, Suzen S. A Comparative study: evaluation of antioxidant activity of melatonin and some indole derivatives. Med Chem Res 2005;14:169–179.
- Gürkök G, Coban T, Suzen S. Melatonin analogue new indole hydrazide/hydrazone derivatives with antioxidant behavior: synthesis and structure-activity relationships. J Enzyme Inhib Med Chem 2009;24:506–515.
- Shirinzadeh H, Eren B, Gurer-Orhan H, Suzen S, Ozden S. Novel indole-based analogs of melatonin: synthesis and in vitro antioxidant activity studies. Molecules 2010;15:2187–2202.
- Rajitha G, Saideepa N, Praneetha P. Synthesis and evaluation of N-(α-benzamidocinnamoyl) aryl hydrazone derivatives for anti-inflammatory and antioxidant activities. Ind J Chem 2011;50B:729–733.
- Prathapa KJ, Himajaa M, Malib SV, Ranjithaa A, Karigarc A, Sikarwar M. Synthesis and antioxidant activity of novel ketone hydrazones bearing 5-(Pyridine-3-yl)-1,3,4-thiadiazole. J Pharm Res 2010;3:2460–2462.
- Kumar PP, Rani BL. Synthesis and characterization of new Schiff bases containing pyridine moiety and their derivatives as antioxidant agents. Int J Chem Tech Research 2011;3:155–160.
- Thangadurai TD, Gowri M, Natarajan K. Synthesis and characterisation of ruthenium(III) complexes containing monobasic bidentate Schiff bases and their biological activities. Synth React Inorg Met-org Chem 2002;32:329–343.
- Belkheiri N, Bouguerne B, Bedos-Belval F, Duran H, Bernis C, Salvayre R et al. Synthesis and antioxidant activity evaluation of a syringic hydrazones family. Eur J Med Chem 2010;45:3019–3026.
- Negre-Salvayre A, Coatrieux C, Ingueneau C, Salvayre R. Advanced lipid peroxidation end products in oxidative damage to proteins. Potential role in diseases and therapeutic prospects for the inhibitors. Br J Pharmacol 2008;153:6–20.
- Yılmaz AD, Coban T, Suzen S. JSynthesis and antioxidant activity evaluations of melatonin-based analogue indole-hydrazide/hydrazone derivatives. J Enzyme Inhib Med Chem 2012;27:428–436.
- Suzen S, Karaaslan C. Electrochemical behavior of biologically important indole derivatives. J Int Electrochem 2011;2011:1–10.
- Kissinger PT, Heineman WR, eds. (1996). Laboratory techniques in electroanalytical chemistry. 2nd ed. New York: Marcel Dekker.
- Wang J, ed. (1988). Electroanalytical techniques in clinical chemistry and laboratory medicine. New York: VCH Publisher Inc.
- Hart HP. (1990). Electroanalysis of biologycally important compounds. England: Ellis Horwood Ltd.
- Vire JC, Kauffmann JM. Curr. Top. Trends in electrochemistry in drug analysis. Electrochem 1994;3:493–515.
- Süzen S, Ates-Alagöz Z, Demircigil BT, Ozkan SA. Synthesis and analytical evaluation by voltammetric studies of some new indole-3-propionamide derivatives. Farmaco 2001;56:835–840.
- Suzen S, Demircigil T, Buyukbingol E, Ozkan SA. Electroanalitical evaluation and determination of 5-(3′-indolyl)-2-thiohydantoin derivatives by voltammetric studies: possible relevance to in vitro metabolism. New J Chem 2003;27:1007–1011.
- Bozkaya P, Dogan B, Suzen S, Nebioglu D, Ozkan SA. Determination and investigation of electrochemical behaviour of 2-phenylindole derivatives: discussion on possible mechanistic pathways. Can J Anal Sci Spec 2006;51:125–139.
- Shirinzadeh H, Yılmaz AD, Gumustas M, Suzen S, Ozden S, Ozkan SA. Electrochemical behavior of indole-3-carboxaldehyde izonicotinoyl hydrazones: discussion on possible biological behavior. Chem High T Synt 2010;13:619–627.
- Kidwai M, Negi N, Gupta SD. Synthesis and antifertility activity of 1,5-diaryl-3-(3’-indolyl)formazans. Chem Pharm Bull 1994;42:2363–2364.
- Lautraite S, Bigot-Lasserre D, Bars R, Carmichael N. Optimisation of cell-based assays for medium throughput screening of oxidative stress. Toxicol In Vitro 2003;17:207–220.
- Hassoun EA, Roche VF, Stohs JS. Release of enzymes by ricin from macrophages and Chinese hamster ovary cells in culture. Toulouse Med 1993;3:119–129.
- Moss DW, Henderson AR, Kochmar JR. (1986). Enzymes. In: Tietz NW, ed. Textbook of clinical chemistry. Philadelphia: WB Saunders, 619–663.
- Barros SB, Simizu K, Junqueira VB. Liver lipid peroxidation-related parameters after short-term administration of hexachlorocyclohexane isomers to rats. Toxicol Lett 1991;56:137–144.
- Suzen S, Ozkan SA. (2011). Medicinal and pharmaceutical applications and prospects of electrochemistry. In: Ozkan SA, ed. Electroanalytical methods in pharmaceutical analysis and their validation. NHB Publishing, ISBN: 978-0-9664286-7-4.
- Kul D, Gumustas M, Uslu B, Ozkan SA. Electroanalytical characteristics of antipsychotic drug ziprasidone and its determination in pharmaceuticals and serum samples on solid electrodes. Talanta 2010;82:286–295.
- Altun Y, Dogan-Topal B, Uslu B, Ozkan SA. Anodic behavior of sertindole and its voltammetric determination in pharmaceuticals and human serum using glassy carbon and boron-doped diamond electrodes. Electrochim Acta 2009;54:1893–1903.
- Laviron E, Roullier C, Degrand J. A multilayer model for the study of space distributed redox modified electrodes: Part II. Theory and application of linear potential sweep voltammetry for a simple reaction. Electroanal Chem 1980;112:11–23.
- Goyal RN, Kumar RN, Signhal NK. Oxidation chemistry and biochemistry of indole and effect of its oxidation product in albino mice. Bioelectrochem Bioenerg 1998;45:47–53.
- Ozkan SA, Uslu B, Aboul-Enein HY. Voltammetric investigation of tamsulosin. Talanta 2003;61:147–156.
- Demircan S, Ozkan SA, Kır S. Electroanalytical characterization of verapamil and its voltammetric determination in pharmaceuticals and human serum. Anal Lett 2007;40:1177–1195.
- Jennings P, Jones AC, Mount AR, Thomson AD. Electrooxidation of 5-substituted indoles. J Chem Soc Faraday Trans 1997;93:3791–3797.
- Enache TA, Oliveira-Brett AM. Pathways of electrochemical oxidation of indolic compounds. Electroanalysis 2011;23:1337–1344.
- Somers KRF, Kryachko ES, Ceulemans A. Theoretical study of indole: protonation, indolyl radical, tautomers of indole, and its interaction with water. Ceulemans Chem Phys 2004;301:61–79.
- Grimshow J, ed. (2000). Electrochemical reactions and mechanisms in organic chemistry. 1st ed. Amsterdam: Elsevier.
- Lund H, Hammerich O. (2001). Organic electrochemistry. 4th ed. New York: Marcel Dekker.
- Dekker M. (1997). Analytical Method Development and Validation. In: Swartz ME, Krull IS, eds. New York: Marcel Dekker, Inc.
- Ermer J, Miller JHMcB. (2005) Method validation in pharmaceutical analysis. 1st ed. Weinheim, Germany: Wiley-VCH.
- Cossum PA. Role of the red blood cell in drug metabolism. Biopharm Drug Dispos 1988;9:321–336.