Abstract
Under anaerobic conditions, the o-diphenol 4-tert-butylcatechol (TBC) irreversibly inactivates met and deoxytyrosinase enzymatic forms of tyrosinase. However, the monophenol 4-tert-butylphenol (TBF) protects the enzyme from this inactivation. Under aerobic conditions, the enzyme suffers suicide inactivation when it acts on TBC. We suggest that TBF does not directly cause the suicide inactivation of the enzyme in the hydroxylase activity, but that the o-diphenol, which is necessary for the system to reach the steady state, is responsible for the process. Therefore, monophenols do not induce the suicide inactivation of tyrosinase in its hydroxylase activity, and there is a great difference between the monophenols that give rise to unstable o-quinones such as L-tyrosine, which rapidly accumulate L-dopa in the medium and those like TBF, after oxidation, give rise to a very stable o-quinone.
Introduction
Tyrosinase (EC 1.14.18.1) is a copper-containing mono-oxygenase widely distributed throughout the phylogenetic scale. Tyrosinase catalyses two types of reactions on phenolic substrates, in which molecular oxygen intervenes: (a) the hydroxylation of monophenols to o-diphenols (monophenolase activity) and (b) the oxidation of o-diphenols to o-quinones (diphenolase activity), which, in turn, are polymerized to brown, red or black pigmentsCitation1. Tyrosinase has two copper atoms in its active site, which may be in three coordination states or forms: (i) Cu2+–Cu2+, met-tyrosinase, (ii) Cu2+––Cu2+ with a peroxide group in its active site, oxy-tyrosinase or (iii) Cu1+–Cu1+, deoxy-tyrosinaseCitation2,Citation3.
Melanogenesis is the principal enzymatic pathway responsible for browning in fruits and vegetablesCitation4, and it also causes pigmentation of the hair, eyes and skin in mammals and other animalsCitation1,Citation5. This pathway can be divided into a proximal and distal phase as follows:
The proximal phase begins with the hydroxylation of a monophenol (L-tyrosine) to its corresponding o-diphenol (L-dopa) and the oxidation of this o-diphenol to its corresponding o-quinone (o-dopaquinone). These two reactions correspond to those described as monophenolase and diphenolase activities of tyrosinase, respectivelyCitation6. Then, through non-enzymatic reactions, the o-dopaquinone evolves towards dopachrome.
The distal phase begins with the chemical decarboxylation of dopachrome towards 5,6-dihydroxyindole (DHI) or with an enzymatic tautomerization of the dopachrome towards 5,6-dihydroxyindole-2-carboxylic acid (DHICA)Citation1,Citation6. These compounds then polymerize giving rise to melanins.
Due to the wide specificity of tyrosinase, several types of substrate can be considered according to the stability of the corresponding quinone.
SA: Substrates yielding stable o-quinone. The clearest example is 4-tert-butylcatechol (TBC), whose o-quinone (o-TBQ) is very stable. In this case, the o-quinone can be measured and can be calculated accuratelyCitation7. The diphenolase activity can be determined, but not the monophenolase activityCitation8.
SB: Substrates yielding a stable coupling product. Substrates which produce a very unstable o-quinone, but that evolve to a stable product through a first-order reaction. An example is 3,4-dihydroxymandelic acid (DOMA), whose o-quinone evolves to 3,4-dihydroxybenzaldehyde (DOBA)Citation9. Other examples of type SB substrate are L-dopa, dopamine, L-α-methylnoradrenaline, isoproterenol, epinine and L-dopa methyl ester, whose o-quinone evolves to aminochromeCitation10.
SC: Substrates yielding a stable nucleophile-adduct. The oxidation of this type of substrate gives rise to an unstable o-quinone which suffers from the attack of a potent nucleophilic reagent (N) and produces a chromophoric adduct (NQ) with a clear stoichiometry. Among the nucleophilic reagents used are L-proline (Pro)Citation10, MBTHCitation11–13 and L-cysteine (Cys)Citation10. The accumulation of the chromophoric product permits us to characterize the monophenolase and diphenolase activities (L-tyrosine, tyramine, 4-hydroxyphenylacetic acid, 4-hydroxyphenylpropionic acid, L-dopa, dopamine, 3,4-dihydroxyphenylacetic acid, 3,4-dihydroxyphenylpropionic acid)Citation10.
SD: Substrates measured by means of ascorbic acid. The substrates give rise to a very unstable o-quinone that evolves with no clear stoichiometry, even after MBTH attack. These substrates can only be characterized through a reduction by ascorbic acid (AH2), either directly or spectrophotometrically following the disappearance of ascorbic acid or using chronometric methods that measure the time necessary for the ascorbic acid to be consumed due to the reaction with o-quinoneCitation7. In this case, measurement by reference to the reduction of the o-quinone by ascorbic acid makes it possible to characterize the diphenolase but not the monophenolase activityCitation7,Citation8.
A large number of studies have been made of the action of tyrosinase on different types of monophenols such as highly unstable o-quinonesCitation10,Citation14 and slightly unstable o-quinonesCitation8,Citation10,Citation15.
As indicated above, tyrosinase is the main enzyme involved in melanization, but is also responsible for the loss of quality of fruit and vegetables during post-harvest handling and processingCitation4. In addition, tyrosinase may cause disorders in pigmentationCitation16. In insects, the enzyme is associated with three processes: cuticle schlerotization, defensive encapsulation and melanization of foreign organisms, and wound healingCitation17. Therefore, inhibitors of this enzyme, which can be isolated from natural sources or be synthesized chemically, have been sought due to the many possible applicationsCitation18–20. Moreover, the enzyme may be inactivated when it acts on a series of substrates, which act as suicide substrates; see the work by Muñoz-MuñozCitation21 for a revision. The study of reversible and irreversible inhibitors and of suicide substrates is an interesting option for controlling the activity of this enzyme.
Both 4-tert-butylphenol (TBF) and 4-tert-butylcatechol (TBC) have been described as depigmenting agents since they act as tyrosinase inhibitorsCitation22. The same compounds have been described as apoptosis inducers in human melanocytes even without the tyrosinase activity of human melanocytesCitation23,Citation24. These phenolic compounds can act as alternative tyrosinase substrates, following a pathway other than the melanin biosynthesis pathway. At the same time, the o-diphenol TBC can act as a suicide substrate for this enzymeCitation21,Citation25.
Tyrosinase undergoes an inactivation process when it reacts with its o-diphenolic substrates, a phenomenon that has long been known in the case of enzymes from a variety of natural sources, including fungi, plant and animalsCitation21. The study of enzymatic inactivation by suicide substrates or mechanism-based inhibitors is of growing importance because of possible pharmacological applicationsCitation26,Citation27. In addition, suicide substrates and mechanism-based enzyme inactivators may be useful for studying enzymatic mechanisms and designing new drugsCitation27. Given that tyrosinase participates in different physiological processes, such as fruit and vegetable browning and pigmentation in animalsCitation1, the suicide inactivation process of this enzyme is of even more interest.
Our group has made several kinetic studies of the suicide inactivation of tyrosinase using mushroom tyrosinaseCitation21,Citation25, frog epidermis tyrosinaseCitation28 and peroxidases from different sourcesCitation29,Citation30. We have also studied the suicide inactivation of an enzyme that can be measured from coupled reactionsCitation31 and have published an experimental study, which used several experimental designs to kinetically study suicide inactivationCitation32.
In the mechanism proposed by our research group, the bifurcation of the catalytic and suicide inactivation pathways is considered to occur through the formCitation21,Citation25,Citation33. Other groups have also proposed that the suicide inactivation occurs through the same form,
Citation34,Citation35.
The main aim of kinetic studies with suicide substrates is their kinetic characterization through the parameters: (maximum apparent inactivation constant),
(a partition ratio between the catalytic and the inactivation pathways),
(catalytic constant) and
(Michaelis constant for the substrate)Citation21,Citation25,Citation29–31.
The kinetic mechanism for tyrosinase acting on monophenols and o-diphenols has been the subject of several studies, and various structural mechanisms have been proposedCitation36–39, e.g. substrates which give highly unstable o-quinones, such as L-tyrosine, L-dopa or dopamineCitation28,Citation33,Citation40. These studies showed that o-diphenol accumulation in the medium through chemical reactions is responsible for suicide inactivation when the enzyme acts on monophenols.
Here, we study the action of tyrosinase in aerobic and anaerobic conditions on monophenols and o-diphenols which, after oxidation, give rise to very stable o-quinones, as in the case of TBF and TBC. In the case of TBC, the enzyme is inactivated both in aerobic (suicide inactivation) and anaerobic (irreversible inactivation) conditions. However, TBF protects the enzyme in both conditions because the o-quinone is stable and cannot regenerate o-diphenol in the medium to inactivate the tyrosinase. But when catalytic concentrations of TBC are added to the reaction medium to follow the monophenolase activity, suicide inactivation occurs due to the action of the enzyme on the added o-diphenol. We try to demonstrate experimentally and confirm by computer simulations that tyrosinase does not suffer suicide inactivation when it acts on monophenols, but does so when acting on the o-diphenol that accumulates in the medium and which is necessary for the steady state to be reached. The stability of o-quinones, then, plays a fundamental role in melanogenesis during the formation of melanins and the suicide inactivation of the enzyme.
Notation
For clarity and for the sake of brevity, we will use the following notations in the text.
Species and concentrations
, Tyrosinase;
, instantaneous concentration of
;
, initial concentration of
;
, active enzyme;
, instantaneous concentration of
;
, met-tyrosinase
, instantaneous concentration of met-tyrosinase;
, initial concentration of met-tyrosinase;
, Deoxy-tyrosinase relaxed form;
, instantaneous concentration of deoxy-tyrosinase relaxed form;
, initial concentration of deoxy-tyrosinase relaxed form;
, deoxy-tyrosinase tense form after the transition of
to
;
, instantaneous concentration of
;
, initial concentration of
;
, inactive enzyme;
, instantaneous concentration of
; TBC, 4-tert-butylcatechol; [TBC], instantaneous concentration of TBC; [TBC]0, initial concentration of TBC;
, o-diphenol (TBC);
, instantaneous concentration of
;
, initial concentration of
;
, o-quinone product of the enzymatic reaction;
, instantaneous concentration of
;
, o-quinone concentration produced at the end of the reaction,
; TBF, 4-tert-butylphenol;
, monophenol (TBF);
, instantaneous concentration of monophenol;
, initial concentration of monophenol;
, molecular oxygen;
, initial concentration of oxygen;
, instantaneous concentration of oxygen;
, oxygen-value at
;
, oxygen consumed at
, i.e.
.
Kinetic parameters
, initial rate of tyrosinase acting on D, measuring Q;
, initial rate of tyrosinase acting on D, measuring
;
, apparent constant of suicide inactivation of tyrosinase in the presence of monophenol;
, maximum value of
for saturating substrate;
, apparent constant of irreversible inhibition of
by
in the presence of the monophenol
;
, apparent constant of irreversible inhibition of
by
in the presence of the monophenol
;
, partition ratio for the diphenolase activity measuring
;
, partition ratio for the diphenolase activity measuring
;
, number of total turnovers (in both cycles, hydroxylase and oxidase) made by one molecule of enzyme acting on
before its suicide inactivation, obtained by measuring the oxygen consumed;
, number of turnovers in the oxidase cycle made by one molecule of enzyme acting on
before its suicide inactivation, measuring oxygen consumed;
, number of turnovers in the hydroxylase cycle made by one molecule of enzyme acting on
before its suicide inactivation, obtained by measuring the oxygen consumed;
, Michaelis constant of tyrosinase for
;
, catalytic constant of the monophenolase activity for
;
, dissociation constant of the complex
in the presence of the monophenol;
, dissociation constant of the complex
;
, dissociation constant of the complex
in the presence of the monophenol;
, dissociation constant of the complex
.
Material and methods
Reagents
Mushroom tyrosinase (o-diphenol: oxidoreductase, EC 1.14.18.1) (8300 units/mg) was supplied by Sigma (Madrid, Spain). The enzyme was purified as described by Rodriguez-Lopez et al.Citation36. Protein concentration was determined by the Lowry methodCitation41. The substrates TBC and TBF were purchased from Sigma (Madrid, Spain). All other chemicals were of analytical grade. Stock solutions of the diphenolic substrates were prepared in 0.15 mM of phosphoric acid to prevent auto-oxidation. Milli-Q system (Millipore Corp., Billerica, MA) ultrapure water was used throughout the experiment.
Spectrophotometric assays
Diphenolase activity
These assays were carried out with a Perkin-Elmer Lambda-35 spectrophotometer (Perkin-Elmer Corp., Waltham, MA), on line interfaced to a PC-computer, where the kinetic data were recorded, stored and later analyzed. The product of the enzyme reaction 4-tert-o-benzoquinone is stable at long assay times, for which reason, the reaction was followed by measuring the appearance of at 410 nmCitation7,Citation10, with
= 1200 M−1 cm−1. The inactivation kinetics was studied in 30 mM sodium phosphate buffer (pH 7.0).
Monophenolase activity
These assays were carried out with the same apparatus as in the diphenolase activity assays. The reaction was followed by measuring the accumulated in the reaction medium at
= 410 nm (
= 1200 M−1 cm−1)Citation7,Citation10. The inactivation kinetics was studied in 30 mM sodium phosphate buffer (pH 7.0), adding the amount of o-diphenol (TBC) necessary for the system to reach the steady state when
, that is an initial steady state.
Oxymetric assays
Measurements of dissolved oxygen concentration were made with a Hansatech (Kings Lynn, Cambs, UK) oxygraph unit controlled by a PC. The oxygraph used a Clark-type silver/platinum electrode with a 12.5 µm Teflon membrane. The sample was continuously stirred during the experiment and maintained at 25 °C. The zero oxygen level for the calibration and experiments was obtained by bubbling oxygen-free nitrogen through the sample for 10 minCitation42. TBF was studied by means of this method, adding the amount of TBC necessary to reach the steady state at .
Kinetic data analysis of monophenolase and diphenolase activities
The experimental recordings of oxygen consumption in the action of tyrosinase on monophenol follow Equation (1):
whose parameters can be obtained by a non-linear regressionCitation43. Thus, the experimental recordings obtained follow Equation (1), from which the corresponding inactivation parameters,
(oxygen remaining at the end of the reaction),
(oxygen consumed by the end of the reaction) and
(apparent inactivation constant), can be determined.
The experimental recordings of accumulation in the action of tyrosinase on monophenol fit Equation (2):
whose parameters can be obtained by a non-linear regressionCitation43. The experimental recordings obtained fit Equation (2), from which the corresponding inactivation parameters,
and
, can be determined.
In the case of the diphenolase activity, the experimental data of time-based assays for accumulation in the action of tyrosinase on o-diphenol fit Equation (2). From this equation,
and
can be determined.
Simulation assays
The simulation shows how the concentrations of the ligand and enzymatic species involved in the reaction mechanism here proposed for tyrosinase evolve. The respective systems of differential equations have been solved numerically for particular sets of values of the rate constants and of initial concentrations of the species of the reaction mechanism. The numerical integration is based on the Runge–Kuta–Fehlberg algorithm, implemented on a PC-compatible computer program (WES)Citation44.
Simulated data of time-based assays for the accumulation of were fitted to Equation (2) (see Supplementary material).
Generation of
,
,
and 
The enzymatic forms were generated and their inactivation was studied following Muñoz-Muñoz et al.Citation40.
Evaluation of enzymatic species
,
and
in an enzymatic preparation of tyrosinase
The evaluation of these enzymatic species in an enzymatic preparation of tyrosinase was carried out as described by Muñoz-Muñoz et al.Citation40.
13C-NMR assays
13C-NMR spectra of TBC and TBF were obtained as described by Muñoz-Muñoz et al.Citation40.
Results and discussion
In this work, we study the reaction of different enzymatic forms of tyrosinase in its catalytic cycle with TBF/TBC both aerobic () and anaerobic (
,
and
) conditions ( in its kinetic form and Scheme 1SM in its structural form).
Scheme 1. Kinetic mechanism proposed to explain the monophenolase together with diphenolase and suicide inactivation pathways of tyrosinase acting on TBF/TBC (see Scheme 1SM for further details).
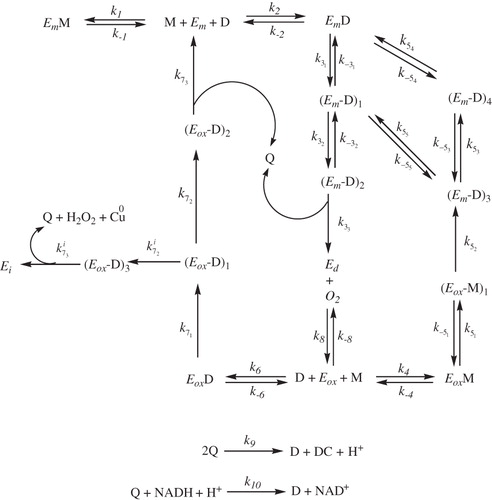
Calculation of the o-diphenol concentration necessary for the system to reach its steady state
First, to eliminate the lag period characteristics of the monophenolase activity when TYR acts on L-tyrosine, the value of the dimensionless parameter , which represents the ratio between
and
necessary for the system to reach the steady state, was calculatedCitation10. If the substrate varies very little in the assay, then
and
can be expressed as
[see the work by Garcia-Molina et al.Citation10]. If the concentration of o-diphenol added exceeds the value predicted by
, a burst of o-quinone accumulation results. However, when the o-quinone does not generate o-diphenol in the medium, as is the case of TBF,
was calculated in an opposite sense. That is, first, a burst was generated by adding a concentration of o-diphenol above the steady state and, then, the o-diphenol concentration added was gradually reduced until the burst was eliminated ()Citation8,Citation10.
Figure 1. Spectrophotometric recordings to calculate the constant [TBC]ss/[TBF]ss in the steady state, . The experimental conditions are sodium phosphate buffer (pH 7.0) 30 mM, [E]0 = 36 nM, [TBF]0 = 0.3 mM and the initial [TBC]0 (µM) concentrations are: (a) 35, (b) 30, (c) 28, (d) 25, (e) 20, (f) 18 and (g) 15.
![Figure 1. Spectrophotometric recordings to calculate the constant [TBC]ss/[TBF]ss in the steady state, . The experimental conditions are sodium phosphate buffer (pH 7.0) 30 mM, [E]0 = 36 nM, [TBF]0 = 0.3 mM and the initial [TBC]0 (µM) concentrations are: (a) 35, (b) 30, (c) 28, (d) 25, (e) 20, (f) 18 and (g) 15.](/cms/asset/94677e7e-901d-45ee-bce8-8177998b1420/ienz_a_782298_f0002_b.jpg)
shows the obtained by adding different concentrations of TBC while maintaining the initial concentration of TBF constant. As can be seen from curve (a), a burst appears in the accumulation of o-quinone as a consequence of the excessive concentration of TBC in the reaction medium. As this concentration diminishes [, curves (b–g)], the burst is eliminated and the steady state is reached at
[curve (g)]. The value obtained of
for the pair TBC/TBF is 0.05.
Inactivation of tyrosinase in aerobic conditions: suicide inactivation
The kinetic mechanism proposed in depicts the action of tyrosinase on monophenols and o-diphenols. It also depicts the suicide inactivation of the enzyme. The structural mechanism is depicted in Scheme 1SM (see Supplementary material).
In the mechanism proposed in , it can be seen how the enzyme is inactivated when its acts on the o-diphenol and, as the number of turnovers that inactivates one mole of enzyme is a constant for a given enzyme, an analytical expression can be established that relates the number of turnovers in the diphenolase cycle ( in the absence of M) with the number of inactivating turnovers in the mechanism of .
The diphenolase activity (action of tyrosinase on TBC) can be monitored spectrophotometrically by measuring the formation of o-quinone, since, in each turnover ( in the absence of M) two molecules of o-quinone are formed at , the quantity of o-quinone is
, and the number of turnovers is calculated by measuring the formation of o-quinone (
) is:
In the mechanism of , the number of turnovers made by the enzyme acting on M and measured by the consumption of oxygen is:
These turnovers are of two types, one for the hydroxylase cycle () and the other for the oxidase cycle (
). The abovementioned turnovers can produce inactivation, and, as two turnovers are produced in the hydroxylase cycle for every one in the oxidase cycle, the turnovers realized in the oxidase cycle as a function of the oxygen consumed will beCitation45:
The relation between Equations (3) and (5) is:
If the activity on monophenols is followed by measuring the o-quinone formation, which, in this case, is stable, bearing in mind the stoichiometry (in the oxidase cycle
and in the hydroxylase cycle
), Equation (6) can be expressed as:
Experimental demonstration of the validity of the analytical expressions shown in Equations (6) and (7) would confirm the hypothesis that tyrosinase is not inactivated in the hydroxylation of monophenols, as has been demonstrated in the case of L-tyrosine and L-dopa, compounds that generate very unstable o-quinones (o-dopaquinone) that lead to the accumulation of L-dopa in the mediumCitation40.
In the present case, the monophenol used (TBF) originates a very stable o-quinone and the only way to study the process is to add a quantity of o-diphenol (TBC) to the medium to prevent the burst while ensuring that the enzyme remains in the steady state (). Once [D]ss has been established, the ratio [D]ss / [M]ss = can be established and maintained in all the experiments.
Experimental approach
Since the substrate originates a stable quinone, a kinetic study of the suicide inactivation that occurs when tyrosinase acts on TBF can be carried out by measuring the consumption of oxygen or spectrophotometrically measuring the formation of o-quinone. depicts the oxygen consumption measured during the tyrosinase action on TBF, while the figure legend details the conditions. Analysis of the recordings depicted in , according to Equation (1), enables and
to be obtained. The inset in shows these parameters as a function of the initial enzyme concentration, and from the slope of
versus
the value of
can be obtained – one-third of these turnovers take place in the oxidase cycle. The inset also shows how the apparent inactivation constant (
) does not depend on the initial enzyme concentration.
Figure 2. Oxymetric recordings of the suicide inactivation of tyrosinase in its action on TBF, varying the initial enzyme concentration. The experimental conditions are sodium phosphate buffer (pH 7.0) 30 mM, [TBF]0 = 0.5 mM, [TBC]0 = 25 µM and the initial enzyme (nM) concentrations are: (a) 0.008, (b) 0.017, (c) 0.026, (d) 0.034, (e) 0.044, (f) 0.052 and (g) 0.06. Inset. Representation of (ˆ) and
(•) versus
.
![Figure 2. Oxymetric recordings of the suicide inactivation of tyrosinase in its action on TBF, varying the initial enzyme concentration. The experimental conditions are sodium phosphate buffer (pH 7.0) 30 mM, [TBF]0 = 0.5 mM, [TBC]0 = 25 µM and the initial enzyme (nM) concentrations are: (a) 0.008, (b) 0.017, (c) 0.026, (d) 0.034, (e) 0.044, (f) 0.052 and (g) 0.06. Inset. Representation of (ˆ) and (•) versus .](/cms/asset/369e7000-e0a3-4b07-ae02-9ce876780013/ienz_a_782298_f0003_b.jpg)
shows the spectrophotometric recordings of the accumulation of o-quinone in the action of tyrosinase on TBF. Analysis of the data by Equation (2) gives the values of and
. The inset in shows the values of
versus
which, from the slope of the straight line, give the value of
. Taking into account, half of
comes from the oxidase pathway and so the value of
is
. Note how
is independent of the enzyme concentrationCitation46,Citation47. The suicide inactivation of tyrosinase on TBC is described Muñoz-Muñoz et al.Citation25 and the values of the kinetic analysis are detailed in . The inset of shows the
versus
and from the slope the value of
can be obtained ().
Figure 3. Spectrophotometric recordings of the suicide inactivation of tyrosinase in its action on TBF, varying the initial enzyme concentration. The experimental conditions are sodium phosphate buffer (pH 7.0) 30 mM, [TBF]0 = 0.1 mM, [TBC]0 = 5 µM and the initial enzyme (nM) concentrations are: (a) 0.045, (b) 0.05, (c) 0.067 and (d) 0.075. The curves (e)–(h) represent the action of the enzyme on TBC. The experimental conditions are the same as curves (a)–(d), but [TBF]0 = 0 and [TBC]0 = 9 mM. The initial enzyme concentrations (nM) are: 0.30 (e), 0.32 (f), 0.34 (g) and 0.37 (h). Inset A. Representation of (ˆ) and
(•) versus
. Inset B. Representation of
(ˆ) and
(•) versus
.
![Figure 3. Spectrophotometric recordings of the suicide inactivation of tyrosinase in its action on TBF, varying the initial enzyme concentration. The experimental conditions are sodium phosphate buffer (pH 7.0) 30 mM, [TBF]0 = 0.1 mM, [TBC]0 = 5 µM and the initial enzyme (nM) concentrations are: (a) 0.045, (b) 0.05, (c) 0.067 and (d) 0.075. The curves (e)–(h) represent the action of the enzyme on TBC. The experimental conditions are the same as curves (a)–(d), but [TBF]0 = 0 and [TBC]0 = 9 mM. The initial enzyme concentrations (nM) are: 0.30 (e), 0.32 (f), 0.34 (g) and 0.37 (h). Inset A. Representation of (ˆ) and (•) versus . Inset B. Representation of (ˆ) and (•) versus .](/cms/asset/39016d90-441c-40da-94b3-7912ebd5d874/ienz_a_782298_f0004_b.jpg)
Table 1. Kinetic constants which characterize the suicide inactivation of tyrosinase by TBC and values of the chemical shifts of this compound obtained by Citation13C-NMR for the C-2 and C-1 carbons at pH 7.0*.
The experimental results shown in and their kinetic analysis according to Equations (1) and (2), give the values of and
, and the number of turnovers made by one mole of enzyme acting on TBF/TBC, measuring the consumption of oxygen or formation of o-quinone, respectively. From the data obtained for the inactivation of the enzyme acting on TBCCitation25, we obtain the value of
, which is equivalent to
. From these results and using Equations (6) and (7), we see that
and
.
These results are resumed in . Note that in the case of the pair TBF/TBC, the great stability of the o-quinones jeans that record the formation of o-quinone can be obtained both with the monophenol and o-diphenol, which cannot be done with the pair L-tyrosine/L-dopa due to the instability of the dopachromeCitation40.
Numerical integration of the mechanisms proposed
The numerical integration of the mechanisms proposed in in the presence and the absence ([M]0 = 0) of monophenol confirms the predictions indicated in Equation (7). Hence, in the “Supplementary material”, we show the set of differential equations that express the monophenolase and diphenolase mechanism of tyrosinase.
Figure 1SM shows the recordings obtained for the formation of o-quinone () in the diphenolase activity of the enzyme by varying the initial concentration. Analysis of the curves by means of Equation (2) gives the values of
and
, while their dependence on
is shown in Figure 1SM (inset). From this dependence of
versus
we obtain the value
.
Figure 2SM shows the recordings obtained for the formation of o-quinone () in the monophenolase and diphenolase activity according to . Analysis of the curves by means of Equation (2) gives the values of
and
, while their dependence on
is shown in Figure 2SM (inset). From the values of
we obtain the value of
. As expected, these values comply with Equation (7) ().
Inactivation of
,
and
under anaerobic conditions by TBC and its protection by TBF
shows the residual activity of tyrosinase when is preincubated with TBC. Substituting these data into Equation (8)
gives the value of
, and the analysis of these data according to Equation (9) (, Inset •), gives the values of
and
().
Figure 4. Residual activity, A/A0, corresponding to the irreversible inactivation of by TBC and its protection by TBF. The form
was obtained as described in the section “Material and methods” and immediately incubated with o-diphenol (TBC) in the presence of monophenol (TBF) or in its absence, taking aliquots at different times to measure the residual activity with [TBC]0 = 9 mM (
= 400 nm). The experimental conditions were: 30 mM sodium phosphate buffer (pH 6.0), 25 °C,
= 0.1 µM (see “Material and methods” section). The [TBC]0 concentrations were (µM): (a) 10 •, (b) 20 ˆ, (c) 35 ▪, (d) 50 □, (e) 60 ▴, (f) 80 ▵, (g) 100 ▾ and (h) 120 ▿. The experimental results follow Equation (8) and the apparent inactivation constants follow Equation (9). Inset. Representation of the values of
versus [TBC]0 in the absence (•) of TBF. In the presence of TBF (ˆ) ([TBF]0 = 40 µM), the experimental results follow Equation (10) to calculate
. These results were substituted into Equation (11) to obtain
and its analysis by Equation (12) gives
.
![Figure 4. Residual activity, A/A0, corresponding to the irreversible inactivation of by TBC and its protection by TBF. The form was obtained as described in the section “Material and methods” and immediately incubated with o-diphenol (TBC) in the presence of monophenol (TBF) or in its absence, taking aliquots at different times to measure the residual activity with [TBC]0 = 9 mM ( = 400 nm). The experimental conditions were: 30 mM sodium phosphate buffer (pH 6.0), 25 °C, = 0.1 µM (see “Material and methods” section). The [TBC]0 concentrations were (µM): (a) 10 •, (b) 20 ˆ, (c) 35 ▪, (d) 50 □, (e) 60 ▴, (f) 80 ▵, (g) 100 ▾ and (h) 120 ▿. The experimental results follow Equation (8) and the apparent inactivation constants follow Equation (9). Inset. Representation of the values of versus [TBC]0 in the absence (•) of TBF. In the presence of TBF (ˆ) ([TBF]0 = 40 µM), the experimental results follow Equation (10) to calculate . These results were substituted into Equation (11) to obtain and its analysis by Equation (12) gives .](/cms/asset/4b8626ae-5ab0-4d60-a129-e97afe7882c1/ienz_a_782298_f0005_b.jpg)
Table 3. Kinetic constants which characterize the inactivation of ,
and
by TBC and their protection by TBF under anaerobic conditions. The constants were obtained using the equations indicated.
In the presence of TBC and the monophenol (TBF) (, Inset ˆ), the residual activity data fit Equation (10) and the values of fit Equation (11), giving
and
. Analysis of these data by means of Equation (12) gives the values of
().
(inset) shows the values of (•) and
(ˆ) versus [TBC]0, which demonstrate how the monophenol protects the enzyme against inactivation by o-diphenol. Similar results are shown in and its inset for
. The results obtained are shown in . The protection presented by the monophenol against the irreversible inactivation caused by the o-diphenol is depicted in and . Possible molecular mechanisms of these inactivations are shown in the “Supplementary material”. Analysis of the residual activity data (for
and
) in the presence of monophenol (TBF) fits Equation (10) but the apparent inactivation constant is now given by Equation (11):
where
is expressed by Equation (12), which is used to calculate
.
Figure 5. Residual activity, A/A0, corresponding to the irreversible inactivation of by TBC and its protection by TBF. The form
was obtained as described in the section “Material and methods” and immediately incubated with o-diphenol (TBC) in the presence of monophenol (TBF) or in its absence, taking aliquots at different times to measure the residual activity with 9 mM TBC (
= 400 nm). The experimental conditions were: 30 mM sodium phosphate buffer (pH 6.0), 25 °C,
= 0.1 µM (see “Material and methods” section). The [TBC]0 concentrations were (µM): (a) 10 •, (b) 20 ˆ, (c) 50 ▪, (d) 100 □, (e) 150 ▴ and (f) 200 ▵. The experimental results follow Equation (8) and the apparent inactivation constants follow Equation (9). Inset. Representation of the values of
versus [TBC]0 in the absence (•) of TBF. In the presence of TBF (ˆ) ([TBF]0 = 40 μM), the experimental results were substituted into Equation (10) to calculate
. These results were again substituted into Equation (11) to obtain
and its analysis by Equation (12) gives
.
![Figure 5. Residual activity, A/A0, corresponding to the irreversible inactivation of by TBC and its protection by TBF. The form was obtained as described in the section “Material and methods” and immediately incubated with o-diphenol (TBC) in the presence of monophenol (TBF) or in its absence, taking aliquots at different times to measure the residual activity with 9 mM TBC ( = 400 nm). The experimental conditions were: 30 mM sodium phosphate buffer (pH 6.0), 25 °C, = 0.1 µM (see “Material and methods” section). The [TBC]0 concentrations were (µM): (a) 10 •, (b) 20 ˆ, (c) 50 ▪, (d) 100 □, (e) 150 ▴ and (f) 200 ▵. The experimental results follow Equation (8) and the apparent inactivation constants follow Equation (9). Inset. Representation of the values of versus [TBC]0 in the absence (•) of TBF. In the presence of TBF (ˆ) ([TBF]0 = 40 μM), the experimental results were substituted into Equation (10) to calculate . These results were again substituted into Equation (11) to obtain and its analysis by Equation (12) gives .](/cms/asset/5c46f988-5ec0-4a64-a4f9-ef42e8264fb9/ienz_a_782298_f0006_b.jpg)
Scheme 2. (A) Effect of monophenols on , inactivation by o-diphenol. (B) Effect of monophenols on
, inactivation by o-diphenol.
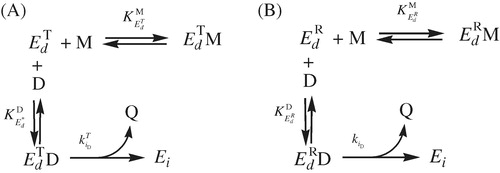
This analysis was also carried out for the form ().
Conclusions
In anaerobic conditions, monophenols protect the enzyme (,
and
forms) against inactivation by o-diphenol. In aerobic conditions, the monophenols do not inactivate the enzyme (
form) in the hydroxylase cycle directly, but, as this activity does not occur without the oxidase cycle acting on o-diphenol, it may be claimed that the monophenols inactivate tyrosinase indirectly. This implies that monophenols such as TBF, which, after oxidation, produce stable o-quinones that do not give rise to o-diphenol in the medium, do not bring about the suicide inactivation of the enzyme, but neither would the enzyme act on them due to the lack of o-diphenol in the medium.
Declaration of interest
The authors report no declarations of interest. This paper was partially supported by grants from: Ministerio de Educación y Ciencia (Madrid, Spain), project BIO2009-12956; Fundación Séneca (CARM, Murcia, Spain), projects 08856/PI/08 and 08595/PI/08, and Consejería de Educación (CARM, Murcia, Spain), BIO-BMC 06/01-0004. JLMM hold fellowships from Fundación Caja Murcia (Murcia, Spain).
References
- Prota G, d`Ischia M, Napolitano A. The chemistry of melanins and related metabolites. In: Nordlund JJ, Boissy RE, Hearing VJ, et al., eds. The pigmentary system, physiology and pathology. Oxford: Oxford University Press; 1998:307–32
- Solomon EI, Sundaran UM, Machonkin TE. Multicopper oxidases and oxygenases. Chem Rev 1996;96:2563–606
- Dietler C, Lerch K. Reaction inactivation of tyrosinase. In: King TE, Mason HS, Morrison M, eds. Oxidases and related redox systems. New York: Pergamon Press; 1982:305–17
- Martinez MV, Whitaker JR. The biochemistry and control of enzymatic browning. Trends Food Sci Technol 1995;6:195–200
- Kondo T, Hearing VJ. Update on the regulation of mammalian melanocyte function and skin pigmentation. Expert Rev Dermatol 2011;6:97–108
- Smith NPM, Pavel S, Riley PA. Mechanisms of control of the cytotoxicity of orthoquinone intermediates of melanogénesis. In: Creveling CR, ed. Role of catechol quinone species in cellular toxicity. Tenesee: F.P. Graham Publishing Co; 2000;191–246
- Muñoz JL, Garcia-Molina F, Varon R, et al. Calculating molar absortivities for quinones: application to the measurements of tyrosinase activity. Anal Biochem 2006;351:128–38
- Ros JR, Rodriguez-Lopez JN, Varon R, García-Cánovas F. Kinetic study of the oxidation of 4-tert-butylphenol by tyrosinase. Eur J Biochem 1994;222:449–52
- Rodríguez-Lopez JN, Serna Rodríguez P, Tudela J, et al. A continuous spectrophotometric method for the determination of diphenolase activity of tyrosinase using 3,4-dihydroxymandelic acid. Anal Biochem 1991;195:369–74
- Garcia-Molina F, Munoz JL, Varon R, et al. A review of spectrophotometric methods for measuring the monophenolase and diphenolase activities of tyrosinase. J Agric Food Chem 2007;55:9739–49
- Espín JC, Varón R, Tudela J, García-Cánovas F. Kinetic study of the oxidation of 4-hydroxyanisole catalyzed by tyrosinase. Biochem Mol Biol Int 1997;41:1265–76
- Espín JC, Varón R, Fenoll LG, et al. Kinetic characterization of the substrate specificity and mechanism of mushroom tyrosinase. Eur J Biochem 2000;267:1270–9
- Espín JC, García-Ruiz PA, Tudela J, García-Cánovas F. Study of the stereospecificity of pear and strawberry polyphenol oxidases. J Agric Food Chem 1998;46:2469–73
- Fenoll L, Rodriguez-Lopez JN, Garcia-Sevilla F, et al. Analysis and interpretation of the action mechanism of mushroom tyrosinase on monophenols and diphenols generating highly unstable o-quinones. Biochim Biophys Acta 2001;1548:1–22
- Fenoll LG, Rodriguez-Lopez JN, García-Sevilla F, et al. Oxidation by mushroom tyrosinase of monophenols generating slightly unstable o-quinones. Eur J Biochem 2000;267:5865–78
- Gupta AK, Gover MD, Nouri KN, Taylor SJ. The treatment of melasma: a review of clinical trials. J Am Acad Dermatol 2006;55:1048–65
- Ashida M, Brey P. Role of the integument in insect defense: pro-phenol oxidase cascade in the cuticular matrix. Proc Natl Acad Sci 1995;92:10698–702
- Sonmez F, Sevmezler S, Atahan A, et al. Evaluation of new chalcone derivatives as polyphenol oxidase inhibitors. Bioorg Med Chem Lett 2011;21:7479–82
- Demir D, Gencer N, Arslan O, et al. In vitro inhibition of polyphenol oxidase by some new diarylureas. J Enzyme Inhib Med Chem 2012;27:125–31
- Gencer N, Demir D, Sonmez F, Kucukislamoglu M. New saccharin derivatives as tyrosinase inhibitors. Bioorg Med Chem 2012;20:2811–21
- Muñoz-Muñoz JL, Garcia-Molina F, Varon R, et al. Suicide inactivation of the diphenolase and monophenolase activities of tyrosinase. IUBMB Life 2010;62:539–47
- Thorneby-Anderson K, Sterner O, Hansson C. Tyrosinase-mediated formation of a reactive quinone from the depigmenting agents, 4-tert-butylphenol and 4-tert-butylcatechol. Pigment Cell Res 2000;13:33–8
- Yang F, Boissi RE. Effects of 4-tertiary butylphenol on the tyrosinase activity in human melanocytes. Pigment Cell Res 1999;12:237–45
- Yang F, Sarangarajan R, Le Poole IC, et al. The cytotoxicity and apoptosis induced by 4-tertiary butylphenol in human melanocytes are independent of tyrosinase activity. Inves Dermatol 2000;114:157–64
- Muñoz-Muñoz JL, Garcia-Molina F, Garcia-Ruiz PA, et al. Phenolic substrates and suicide inactivation of tyrosinase: kinetics and mechanism. Biochem J 2008;416:413–40
- Silverman RB. Mechanism-based enzyme inactivators. Meth Enzymol 1995;249:240–83
- Zhong JY, Groutas WC. Recent developments in the design of mechanism-based and alternate substrate inhibitors of serine proteases. Curr Top Med Chem 2004;4:1203–16
- Tudela J, Garcia-Canovas F, Varon R, et al. Kinetic characterization of dopamine as a suicide substrate of tyrosinase. J Enzyme Inhibition 1987;2:47–56
- Arnao MB, Acosta M, del Rio JA, et al. A kinetic-study on the suicide inactivation of peroxidase by hydrogen-peroxide. Biochim Biophys Acta 1990;1041:43–7
- Hiner A, Hernandez-Ruiz J, Arnao MB, et al. A comparative study of the purity, enzyme activity, and inactivation by hydrogen peroxide of commercially available horseradish peroxidase isoenzymes A and C. Biotechnol Bioeng 1996;50:655–62
- Escribano J, Tudela J, Garcia-Carmona F, Garcia-Canovas F. A kinetic-study of the suicide inactivation of an enzyme measured through coupling reactions: application to the suicide inactivation of tyrosinase. Biochem J 1989;262:597–603
- García-Canovas F, Tudela J, Varon R, Vázquez AM. Experimental methods for kinetic study of suicide substrates. J Enzyme Inhibition 1989;3:81–90
- Muñoz-Muñoz JL, Acosta-Motos JR, Garcia-Molina F, et al. Tyrosinase inactivación in its action on L-dopa. Biochim Biophys Acta 2009;1804:1467–75
- Land E, Ramsden C, Riley PA. The mechanism of suicide-inactivation of tyrosinase: a substrate structure investigation. Tohoku J Exp Med 2007;212:341–8
- Ramsden C, Riley PA. Mechanistic studies of tyrosinase suicide inactivation. ARKIVOC 2010;i:260–74
- Rodriguez-Lopez JN, Fenoll LG, Garcia-Ruiz PA, et al. Stopped-flow and steady-state study of the diphenolase activity of mushroom tyrosinase. Biochemistry 2000;39:10497–506
- Koval IA, Gamez P, Belle C, et al. Synthetic models of the active site of catechol oxidase: mechanistic studies. Chem Soc Rev 2006;35:814–40
- Palavicini S, Granata A, Monzani E, Casella L. Hydroxylation of phenolics compounds by a peroxodicopper (II) complex: further insight into the mechanism of tyrosinse. J Am Chem Soc 2005;127:18031–6
- Rolff M, Schottenheim J, Decker H, Tuczek F. Copper-O2 reactivity of tyrosinase models towards external monophenolic substrates: molecular mechanism and comparison with the enzyme. Chem Soc Rev 2011;40:4077–98
- Muñoz-Muñoz JL, Garcia-Molina F, Acosta-Motos JR, et al. Indirect inactivation of tyrosinase in its action on L-tyrosine. Acta Biochim Pol 2011;58:477–88
- Lowry OH, Rosebrough NJ, Farr AL, Randall RJ. Protein measurement with the Folin phenol reagent. J Biol Chem 1951;193:265–75
- Rodríguez-Lopez JN, Ros-Martinez JR, Varon R, Garcia-Canovas F. Calibration of a Clark-type oxygen electrode by tyrosinase catalyzed-oxidation of 4-tert-butylcatechol. Anal Biochem 1992;202:356–60
- Jandel Scientific. Sigma Plot 9.0 for WindowsTM. Corte Madera: Jandel Scientific; 2006
- Garcia-Sevilla F, Garrido del Solo C, Duggleby RG, et al. Use of a window program for simulation of the progress curves of reactants and intermediates involved in enzyme-catalyzed reactions. Biosystems 2000;54:151–64
- Rodriguez-Lopez JN, Tudela J, Varon R, et al. Analysis of a kinetic model for melanin biosynthesis pathway. J Biol Chem 1992;267:3801–10
- Muñoz-Muñoz JL, Garcia-Molina F, Berna J, et al. Kinetic characterisation of o-aminophenols and aromatic o-diamines as suicide substrates of tyrosinase. Biochim Biophys Acta 2012;1824:647–55
- Munoz-Munoz JL, Berna J, Garcia-Molina F, et al. Unravelling the suicide inactivation of tyrosinase: a discrimination between mechanisms. J Mol Catal B-Enzymatic 2012;75:11–19