Abstract
Context and objective: The present study is to elucidate the comparative inhibition of tetrameric carbonyl reductase (TCBR) activity by alkyl 4-pyridyl ketones, and to characterize its substrate-binding domain.
Materials and methods: The inhibitory effects of alkyl 4-pyridyl ketones on the stereoselective reduction of 4-benzoylpyridine (4-BP) catalyzed by TCBR were examined in the cytosolic fraction of pig heart.
Results: Of alkyl 4-pyridyl ketones, 4-hexanoylpyridine, which has a straight-chain alkyl group of five carbon atoms, inhibited most potently TCBR activity and was a competitive inhibitor. Furthermore, cyclohexyl pentyl ketone, which is substituted by cyclohexyl group instead of phenyl group of hexanophenone, had much lower ability to be reduced than hexanophenone.
Discussion and conclusion: These results suggest that in addition to a hydrophobic cleft corresponding to a straight-chain alkyl group of five carbon atoms, a hydrophobic pocket with affinity for an aromatic group is located in the substrate-binding domain of TCBR.
Introduction
Carbonyl reductase (EC 1.1.1.184) is an enzyme that catalyzes NADPH-dependent reduction of endogenous and xenobiotic carbonyl compoundsCitation1–4. The cDNA for carbonyl reductase was first cloned by Wermuth et al.Citation5, and the enzyme belongs to the short-chain dehydrogenase/reductase (SDR) superfamily. So far, four kinds of mammalian carbonyl reductases (CBR1, CBR2, CBR3 and CBR4) are characterizedCitation5–8. Carbonyl reductases have the ability to catalyze stereoselective reduction of ketone-containing drugs, such as acetohexamide and loxoprofen, to their alcohol metabolitesCitation9–12.
We have recently purified a new tetrameric carbonyl reductase (TCBR) from the cytosolic fraction of pig heart, using 4-benzoylpyridine (4-BP) as a substrateCitation13. The purified TCBR is now designated dehydrogenase/reductase (SDR family) member 4 (DHRS4) and the catalytic properties of mammalian DHRS4 including human enzyme have been reportedCitation2,Citation3,Citation13,Citation14. The purified TCBR (pig DHRS4), like human DHRS4, has the ability to reduce ketones, quinones and retinals efficientlyCitation13,Citation14. Interestingly, when the cytosolic fraction of pig heart was applied to a DEAE-Sephacel column, the activity of 4-BP-reducing enzyme appeared as a single peak, similar to the result obtained from the cytosolic fraction of rabbit heartCitation15. Furthermore, in the cytosolic fraction of pig heart, 4-BP was stereoselectively reduced to S(−)-α-phenyl-4-pyridylmethanol (S(−)-PPOL), as shown in Citation16. These findings suggest that in the cytosolic fraction of pig heart, 4-BP is mainly reduced to S(−)-PPOL by TCBRCitation17, and that the subcellular fraction is useful as the reaction system of TCBR when 4-BP is used as the substrate.
Our previous articleCitation18 has examined the substrate inhibition of TCBR activity by a variety of alkyl phenyl ketones, by using the cytosolic fraction of pig heart. Of alkyl phenyl ketones, hexanophenone having a straight-chain alkyl group of five carbon atoms inhibited TCBR activity most potently and was a competitive inhibitor. These results suggest that a hydrophobic cleft corresponding to straight-chain alkyl group of five carbon atoms in length is located in the substrate-binding domain of TCBRCitation18. As shown in , 4-BP used as the substrate has 4-pyridyl group and phenyl group across a ketone group in the chemical structure. The purpose of the present study is to elucidate the comparative inhibition of TCBR activity by alkyl 4-pyridyl ketones (), and to characterize its substrate-binding domain.
Materials and methods
Materials
4-BP was purchased from Wako Pure Chemicals (Osaka, Japan). S(−)-PPOL and R(+)-PPOL were synthesized from 4-BPCitation16. Hexanophenone and 4-acetylpyridine were obtained from Aldrich (Milwaukee, WI). All other chemicals were of reagent grade.
Synthesis of alkyl 4-pyridyl ketones
The synthesis of alkyl 4-pyridyl ketones, except 4-acetylpyridine, was carried out according to a slight modification of the method reported by usCitation19. A solution of pyridine-4-aldehyde (5.4 g, 50 mmol) in anhydrous tetrahydrofuran (30 ml) was added to an ice-cooled solution of the corresponding Grignard reagent (freshly prepared from Mg and alkyl bromide in anhydrous tetrahydrofuran (65 mmol)) under a nitrogen atmosphere. The mixture was stirred at room temperature for 12 h and then quenched with aqueous saturated NH4Cl solution (100 ml). The resulting mixture was extracted with CHCl3 (3 × 50 ml) and the CHCl3 layer was washed with brine. The extract was dried over anhydrous MgSO4. After evaporation of the solvent, the residue was chromatographed on silica gel to give alkyl 4-pyridyl alcohols (n-hexane-ethyl acetate, 5:1 (v/v)). To the mixture of the alkyl 4-pyridyl alcohols (15 mmol) in acetone (15 ml) and MgSO4 (3.9 g, 32 mmol) in water (70 ml) was added to KMnO4 (4.7 g, 30 mmol). The mixture was stirred at 60 °C for 1 h and then quenched with methanol (about 10 ml). The resulting mixture was filtered with celite and the filtrate was extracted with CHCl3 (3 × 50 ml). The organic layer was washed with brine, dried over MgSO4 and concentrated under reduced pressure. The residue was purified by chromatography on silica gel to give alkyl 4-pyridyl ketones (n-hexane-ethyl acetate, 4:1 (v/v)). The structures of alkyl 4-pyridyl ketones were confirmed by IR, 1H-NMR and mass spectrometry.
Synthesis of cyclohexyl pentyl ketone
A solution of cyclohexanecarboxaldehyde (1.0 g, 8.9 mmol) in anhydrous tetrahydrofuran (5 ml) was added to a solution of 2.0 M pentylmagnesium bromide in diethyl ether (4.5 ml, 9.0 mmol) at −15 °C under argon atmosphere. The reaction mixture was stirred at the same temperature for 1 h and then diluted with aqueous NH4Cl solution (50 ml). The resulting mixture was extracted with ethyl acetate (50 ml). The organic layer was washed with brine and dried over MgSO4. The organic layer was evaporated to give a residue, which was chromatographed on silica gel (n-hexane-ethyl acetate, 75:1) to afford alcohol (786.6 mg, 48%). The structure of alcohol was confirmed by IR, 1H-NMR and 13C-NMR spectroscopy. A mixture of alcohol (100 mg, 0.54 mmol) and Dess--Martin reagent (460 mg, 1.08 mmol) in CH2Cl2 (2 ml) was stirred for 30 min at room temperature. The reaction mixture was diluted with diethyl ether (30 ml). The organic layer was washed with 7% aqueous NaHCO3 (30 ml) and dried over MgSO4. Evaporation of organic solvent gave a crude residue, which was chromatographed on silica gel (n-hexane-ethyl acetate, 50:1) to afford cyclohexyl pentyl ketone (86.3 mg, 87%). The structure of cyclohexyl pentyl ketone was confirmed by IR, 1H-NMR and 13C-NMR spectroscopy.
Preparation of the cytosolic fraction from pig heart
Pig hearts were supplied from a slaughterhouse and stored at −80 °C. The tissues were homogenized in three volumes of 10 mM sodium potassium phosphate buffer containing 1.15% KCl (pH 6.0). The homogenates were centrifuged at 105 000 × g for 60 min to obtain the cytosolic fraction which was used for the determination of TCBR activity, as described below.
Assay of TCBR activity
TCBR activity was assayed by determining S(−)-PPOL formed from 4-BP in the cytosolic fraction of pig heart. The reaction mixture consisted of substrate (500 μM 4-BP), NADPH-generation system (50 μM NADP+, 1.25 mM glucose-6-phosphate, 50 mU glucose-6-phosphate dehydrogenase and 1.25 mM MgCl2), pig heart cytosol and 100 mM sodium potassium phosphate buffer (pH 6.0) in a final volume of 0.5 ml. The reaction mixture was incubated at 37 °C for 10 min and boiled for 2 min to stop the reaction. After centrifugation at 5000 rpm, the supernatant (20 μl) was subjected to high-performance liquid chromatography (HPLC) for determination of the reduction products (S(−)-PPOL and R(+)-PPOL) of 4-BP.
HPLC was carried out using a Tosoh DP-8020 HPLC apparatus (Tosoh, Tokyo, Japan) equipped with a Daicel Chiralpak AD-RH column (Daicel, Tokyo, Japan) and a Tosoh UV-8020 monitor (254 nm). A mixture of 20 mM borate buffer (pH 9.0)–acetonitrile (6:4, v/v) was used as a mobile phase at a flow rate of 0.5 ml/min. In the case of inhibition experiments, alkyl 4-pyridyl ketones were dissolved in methanol and added to the reaction mixture at a concentration of 500 μM. The final concentration of methanol did not exceed 2% (v/v), and this concentration did not affect the enzyme reaction.
Furthermore, the inhibition for the stereoselective reduction of 4-BP to S(−)-PPOL by 4-hexanoylpyridine was kinetically analyzed using Lineweaver-Burk plots. Protein concentration was determined by the method of Lowry et al.Citation20 with bovine serum albumin as the standard.
Determination of kinetic parameters for 4-hexanoylpyridine and hexanophenone
The reduction of 4-hexanoylpyridine and hexanophenone was measured spectrophotometrically by monitoring the decrease in the absorbance of NADPH at 340 nm (ε = 6220 M−1 cm−1). The reaction mixture consisted of substrate, 0.3 mM NADPH, pig heart cytosol and 100 mM sodium potassium phosphate buffer (pH 6.0) in a final volume of 0.5 ml. The enzyme reaction was initiated by the addition of 4-hexanoylpyridine or hexanophenone at various concentrations to the reaction mixture. The parameters (Km, Vmax and Vmax/Km) in the reduction of 4-hexanoylpyridine and hexanophenone were kinetically determined. One unit of enzyme activity was defined as the amount catalyzing the oxidation of 1 μmol of NADPH/min at 37 °C.
Reduction of cyclohexyl pentyl ketone and hexanophenone
The reduction of cyclohexyl pentyl ketone and hexanophenone was measured spectrophotometrically, as described above. The reaction mixture consisted of substrate, 0.3 mM NADPH, pig heart cytosol and 100 mM sodium potassium phosphate buffer (pH 6.0) in a final volume of 0.5 ml. The enzyme reaction was initiated by the addition of cyclohexyl pentyl ketone or hexanophenone at a concentration of 100 μM to the reaction mixture.
Results
Inhibition of TCBR activity by alkyl 4-pyridyl ketones
We synthesized alkyl 4-pyridyl ketones having a straight-chain alkyl groups () and examined the inhibitory potencies of these ketones for TCBR activity. TCBR activity was estimated as the ability to reduce 4-BP to S(−)-PPOL in the cytosolic fraction of pig heart, since the subcellular fraction is useful as the reaction system of TCBR when 4-BP is used as the substrate. As shown in , the inhibitory potencies of alkyl 4-pyridyl ketones for TCBR activity increased with an increase in the number up to five carbon atoms in the alkyl group, and 4-hexanoylpyridine was the most potent inhibitor of the enzyme activity. The inhibitory potencies of 4-heptanoylpyridine and 4-octanoylpyridine, which have respective six and seven carbon atoms in the alkyl groups, were lower than that of 4-hexanoylpyridine.
Figure 3. Relationship between the number of carbon atoms in alkyl 4-pyridyl ketones and their inhibitory potencies for TCBR activity in the cytosolic fraction of pig heart. 4-BP at a concentration of 500 μM was used as the substrate. The concentration of these ketones added as inhibitors was 500 μM. Each bar represents the mean ± SD of three or four experiments.
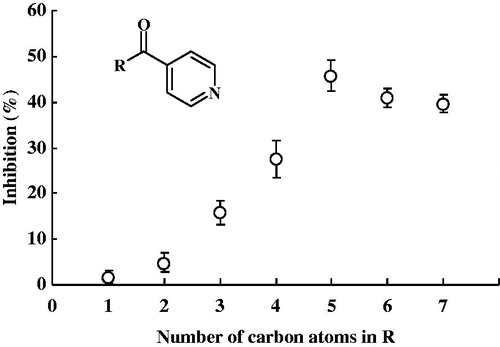
Kinetic mechanism for inhibition of TCBR activity by 4-hexanoylpyridine
The kinetic mechanism for inhibition of TCBR activity by 4-hexanoylpyridine was analyzed using Lineweaver-Burk plots. As expected, 4-hexanoylpyridine was confirmed to inhibit the enzyme activity competitively ().
Figure 4. Lineweaver-Burk plots for the reduction of 4-BP to S(−)-PPOL in the absence and in the presence of 4-hexanoylpyridine. Open circle (○), in the absence of 4-hexanoylpyridine; closed circle (•), in the presence of 4-hexanoylpyridine (500 μM). Each bar represents the mean ± SD of four experiments.
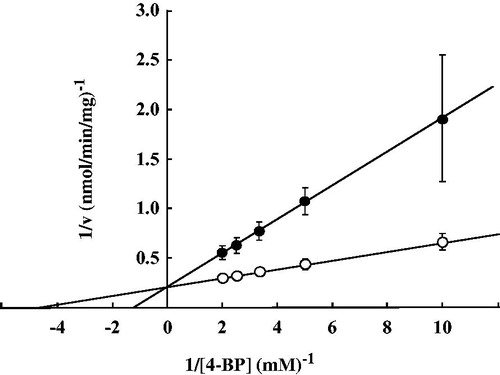
Reduction of cyclohexyl pentyl ketone and hexanophenone
The reduction of cyclohexyl pentyl ketone, which is substituted by cyclohexyl group (ring of six carbon atoms) instead of phenyl group (ring of six carbon atoms) of hexanophenone, was examined spectrophotometrically by monitoring the decrease in the absorbance of NADPH at 340 nm. As shown in , cyclohexyl pentyl ketone had much lower ability to be reduced in pig heart cytosol than hexanophenone.
Discussion
Our recent studyCitation18 has demonstrated that alkyl phenyl ketones having a straight-chain alkyl group can inhibit TCBR activity (the stereoselective reduction of 4-BP to S(−)-PPOL) in the cytosolic fraction of pig heart. Interestingly, the inhibitory potencies of alkyl phenyl ketones for TCBR activity were found to increase with an increase in the number up to five carbon atoms in the alkyl group. Of alkyl phenyl ketones examined, hexanophenone was the most potent inhibitor for the enzyme activity and a competitive inhibitor. On the basis of these results, we have proposed that an alkyl hydrophobic cleft, which fits most efficiently to a straight-chain alkyl group of five carbon atoms, is located in the substrate-binding domain of TCBRCitation18.
In the present study, the inhibitory potencies of alkyl 4-pyridyl ketones having a straight-chain alkyl group for TCBR activity were further examined in the cytosolic fraction of pig heart. Of alkyl 4-pyridyl ketones, 4-hexanoylpyridine having a straight-chain alkyl group of five carbon atoms inhibited the enzyme activity most potently and was a competitive inhibitor. These results provide further evidence that a hydrophobic cleft corresponding to a straight-chain alkyl group of five carbon atoms in length is located in the substrate-binding domain of TCBRCitation18. In addition to the alkyl hydrophobic cleft, it is likely that an aryl hydrophobic pocket is located in substrate-binding domain of TCBR. The pocket probably has affinity for an aromatic group. This is supported from the facts that cyclohexyl pentyl ketone, which is substituted by cyclohexyl group instead of phenyl group of hexanophenone, is little reduced in the cytosolic fraction of pig heart.
The inhibitory potencies of alkyl 4-pyridyl ketones for TCBR activity were confirmed to be lower than those of alkyl phenyl ketones having a straight-chain alkyl group of the corresponding carbon atoms, by comparing with the experimental data reported previouslyCitation18. Furthermore, the Vmax/Km value (186 ± 30 mU/mg/min, n = 4) for the reduction of 4-hexanoylpyridine was significantly smaller than that (410 ± 13 mU/mg/min, n = 4) of hexanophenone. These may be because 4-pyridyl group exhibits lower hydrophobicity than phenyl group. 4-BP used as the substrate in this study has 4-pyridyl group and phenyl group across a ketone group in the chemical structure (). Judging from the hydrophobicity, it is reasonable to assume that phenyl group rather than 4-pyridyl group in 4-BP binds predominantly to the aryl hydrophobic pocket located in the substrate-binding domain of TCBR. As a result, 4-BP may be reduced stereoselectively to S(−)-PPOL by TCBR.
In conclusion, the present study demonstrates that a hydrophobic cleft corresponding to a straight-chain alkyl group of five carbon atoms in length and a hydrophobic pocket with affinity for an aromatic group are located in the substrate-binding domain of TCBR. Further studies are in progress to elucidate the characteristics in substrate-binding domain of TCBR.
Declaration of interest
The authors report no declarations of interest.
References
- Forrest GL, Gonzalez G. Carbonyl reductase. Chem Biol Interact 2000;129:21–40
- Matsunaga T, Sintani S, Hara A. Multiplicity of mammalian reductases for xenobiotic carbonyl compounds. Drug Metab Pharmacokinet 2006;21:1–18
- Oppermann U. Carbonyl reductases: the complex relationship of mammalian carbonyl- and quinone-reducing enzymes and their role in physiology. Annu Rev Pharmacol Toxicol 2007;47:293–322
- Hoffmann F, Maser E. Carbonyl reductases and pluripotent hydroxysteroid dehydrogenases of the short-chain dehydrogenase/reductase superfamily. Drug Metab Rev 2007;39:87–144
- Wermuth B, Bohren KM, Heinemann G, et al. Human carbonyl reductase: nucleotide sequence analysis of a cDNA and amino acid sequence of the encoded protein. J Biol Chem 1988;263:16185–8
- Nakanishi M, Deyashiki Y, Ohshima K, Hara A. Cloning, expression and tissue distribution of mouse tetrameric carbonyl reductase. Identity with an adipocyte 27-kDa protein. Eur J Biochem 1995;228:381–7
- Miura T, Nishinaka T, Terada T. Different functions between human monomeric carbonyl reductase 3 and carbonyl reductase 1. Mol Cell Biochem 2008;315:113–21
- Endo S, Matsunaga T, Kitade Y, et al. Human carbonyl reductase 4 is a mitochondrial NADPH-dependent quinone reductase. Biochem Biophys Res Commun 2008;377:1326–30
- McMahon RE, Marshall FL, Culp HW. The nature of the metabolites of acetohexamide in the rat and in the human. J Pharmacol Exp Ther 1965;149:272–9
- Imamura Y, Kojima Y, Higuchi T, et al. Stereoselective reduction of acetohexamide in cytosol of rabbit liver. J Pharmacobiodyn 1989;12:731–5
- Takasaki W, Tanaka Y. Application of antibody-mediated extraction for the stereoselective determination of the active metabolite of loxoprofen in human and rat plasma. Chirality 1992;4:308–15
- Ohara H, Miyabe Y, Deyashiki Y, et al. Reduction of drug ketones by dihydrodiol dehydrogenases, carbonyl reductase and aldehyde reductase of human liver. Biochem Pharmacol 1995;50:221–7
- Usami N, Ishikura S, Abe H, et al. Cloning, expression and tissue distribution of a tetrameric form of pig carbonyl reductase. Chem Biol Interact 2003;143–144:353–61
- Matsunaga T, Endo S, Maeda S, et al. Characterization of human DHRS4: an inducible short-chain dehydrogenase/reductase enzyme with 3β-hydroxysteroid dehydrogenase activity. Arch Biochem Biophys 2008;477:339–47
- Imamura Y, Migita T, Otagiri M, et al. Purification and catalytic properties of a tetrameric carbonyl reductase from rabbit heart. J Biochem 1999;125:41–7
- Shimada H, Fujiki S, Oginuma M, et al. Stereoselective reduction of 4-benzoylpyridine by recombinant pig heart carbonyl reductase. J Mol Catal B Enzym 2003;23:29–35
- Shimada H, Oginuma M, Hara A, Imamura Y. 9,10-Phenanthrenequinone, a component of diesel exhaust particles, inhibits the reduction of 4-benzoylpyridine and all-trans retinal and mediates superoxide formation through its redox cycling in pig heart. Chem Res Toxicol 2004;17:1145–50
- Imamura Y, Narumi R, Shimada H. Inhibition of carbonyl reductase activity in pig heart by alkyl phenyl ketones. J Enzyme Inhib Med Chem 2007;22:105–9
- Imamura Y, Higuchi T, Nozaki Y, et al. Purification and properties of carbonyl reductase from rabbit kidney. Arch Biochem Biophys 1993;300:570–6
- Lowry OH, Rosebrough NJ, Farr AL, Randall RJ. Protein measurement with the Folin phenol reagent. J Biol Chem 1951;193:265–75