Abstract
Carbonic anhydrase 9 (CA9) and carbonic anhydrase 12 (CA12) were proposed as potential targets for cancer therapy more than 20 years ago. However, to date, there are only very few antibodies that have been described to specifically target CA9 and CA12 and also block the enzymatic activity of their targets. One of the early stage bottlenecks in identifying CA9- and CA12-inhibiting antibodies has been the lack of a high-throughput screening system that would allow for rapid assessment of inhibition of the targeted carbon dioxide hydratase activity of carbonic anhydrases. In this study, we show that measuring the esterase activity of carbonic anhydrase offers a robust and inexpensive screening method for identifying antibody candidates that block both hydratase and esterase activities of carbonic anhydrase’s. To our knowledge, this is the first implementation of a facile surrogate-screening assay to identify potential therapeutic antibodies that block the clinically relevant hydratase activity of carbonic anhydrases.
Introduction
Carbonic anhydrases (CA’s) are zinc-containing metalloenzymes that can catalyze the reversible conversion of carbon dioxide and water into bicarbonate and proton, thereby playing a crucial role in pH regulationCitation1–7. Currently, there are 15 human CA isoforms known, which differ in their cellular localization and rate of enzymatic activityCitation2. Carbonic anhydrase 9 (CA9) and carbonic anhydrase 12 (CA12) are membrane-expressed isoforms whose over-expression has been associated with different types of cancersCitation8–10. In the past 20 years, since CA9 and CA12 were found to be associated with cancer progression, it has been difficult to develop inhibitors that specifically block these two CA isoforms and inhibit tumor progression. This lack of success has been mainly due to the high sequence and structural similarity that exists between all CA isoforms and their widespread expression by normal cells and tissuesCitation8,Citation10,Citation11. Progress has been made regarding the design of sulfonamide-derived small molecules with CA-blocking activity, since these compounds can be used in vitro and for imaging purposes. However, their therapeutic potential remains unclear. A second major area of interest within the past decade has been the generation of monoclonal antibodies (mAbs), specifically targeting either CA9 or CA12Citation12–15. One CA9-specific mAb (G250, Girentuximab) has entered late-stage clinical developmentCitation16. Unfortunately, the anti-tumor effect of Girentuximab in patients with renal cancer was limited, and no clinical activity was observed in a recently published large randomized trialCitation16–19. The lack of efficacy was partially ascribed to the inability of Girentuximab to block CA9 enzymatic activityCitation16.
So far, little success has been made in identifying carbonic anhydrase-binding antibodies that also block CA enzymatic activity. 6A10 was the first and remains the best-described antibody for the inhibition of CA12 hydratase enzyme activity, with a potential biological role in the reduction of tumor growthCitation15,Citation20,Citation21. However, only very few antibodies that can specifically bind and inhibit the hydratase activity of CA9 have appeared in the literature, such as the recently described MSC8 antibodyCitation22,Citation23. Lack of effective and efficacious screening methods, which are easy to perform and offer a rapid and high throughput system for identifying CA9 and CA12 blocking antibodies, is believed to be one of the main reasons for this failure.
Carbonic anhydrase enzyme activity can be measured by a variety of enzymatic assays including the quantification of CO2 consumption or detection of the release of protonsCitation24. All of those methods suffer from one or more disadvantages including interference of the assay buffer/substrate with the enzymatic activity, time-consuming process and expense or complexity of instrumentationCitation24. These disadvantages are critical and outweigh any advantages of the above-mentioned methods (e.g. improved sensitivity), especially for being a rapid and inexpensive screening method warranted for the identification of inhibitory antibodies. In contrast, the measurement of the esterase activity of carbonic anhydrase is the only read-out of the carbonic anhydrase, which has none of the above-mentioned disadvantages. Despite the relatively slow kinetics compared to the physiologically relevant hydratase activity, esterase activity offers the definite advantage of a facile colorimetric assay that can be implemented at high-throughputCitation25. The esterase activity of carbonic anhydrases was discovered in the early 1960sCitation26. Since then, several structural, functional and mutational studies have shown that the hydratase activity and the esterase activity of carbonic anhydrase share similar mechanism, and importantly, the same catalytic pocket, implicating that esterase activity is indicative of hydratase activityCitation27–32.
Using the recently established antibodies that can specifically bind to and inhibit the hydratase enzymatic activity of CA9 (MSC8) and CA12 (6A10), we demonstrate that esterase activity can serve as a rapid, robust and inexpensive surrogate marker for the identification of antibodies blocking carbonic anhydrase enzymatic activityCitation20,Citation22.
Materials and methods
Recombinant human CA9 and human CA12 enzymes were purchased from Sino Biological Inc., (Beijing, China). TMB peroxidase substrate from KPL, Inc. (Gaithersburg, MD). ImmunoTM Nunc Maxisorp 96-well flat-bottom plates were from Thermo Fisher Scientific (Roskilde, Denmark). All optical density/absorbance measurements were performed with Synergy H1 plate reader from BioTek (Luzern, Switzerland). Antibodies were purchased from various sources, human isotype antibodies from Southernbiotech (Birmingham, AL), rat isotype antibody from Biolegend (San Diego, CA) and anti-CA4-polyclonal antibody and anti-CA14-polyclonal antibody from Santa Cruz Biotechnology (Dallas, TX). Horse radish peroxidase (HRP) conjugated secondary antibodies, anti-human (1:20 000), anti-rat (1:10 000) and anti-rabbit (1:4000), acetazolamide (AZA), 4-nitrophenyl acetate (4-NPA or pNPA) and all other materials were purchased from Sigma-Aldrich (St. Louis, MO), unless otherwise specified.
Antibody production
mAb, 4AB9 (unpublished), was generated and produced, while the mAbs MSC8, MSC12 and 6A10 were re-cloned and produced at 4-Antibody AG (Basel, Switzerland). In brief, transient transfection of both heavy and light chain expression vectors into FS-CHO cells was performed for production of IgG. Transfected cells were grown in FreeStyle CHO-S/BD-Select CHO medium. Antibodies were purified by chromatography on protein-A columns.
Enzyme-linked immunosorbent assay
All the solutions/buffers were prepared in 1×PBS. Enzyme-linked immunosorbent assay (ELISA) plate wells were coated with 100 μl of 2 mg/ml antigen (CA9 or CA12) and incubated over night at 4 °C. Blocking was performed with 1% bovine gelatin (200 μl/well) at room temperature (RT) for 1 h; 5 mg/ml of primary antibodies (100 μl/well) were incubated for 1.5 h at 37 °C. HRP-conjugated secondary antibodies (100 μl/well) were incubated at RT for 1 h protected from light. Between all the above-mentioned steps, washing was done three times with 1×PBS (200 μl/well). After washing, freshly prepared peroxidase substrate (100 μl/well), pre-warmed to RT, was added and incubated at RT up to 30–40 min (protected from light) for the blue-color product formation. The peroxidase reaction was stopped by adding 50 μl of 2 N H2SO4, and the formation of a stable yellow color was measured at 450 nm. The non-specific binding of primary and secondary antibodies was always subtracted, i.e. the background absorbance of the wells coated with 1 × PBS.
Esterase activity assay
Final concentration of the reaction components: 5 μg/ml of CA9 (93 nM) or CA12 (114 nM), 1 mM of substrate, pNPA, in 100 μl of assay buffer (12.5 mM Tris, 75 mM NaCl and pH 7.5) CA9 and CA12 final concentrations used in the esterase assay were determined by titrating the enzymes at a fixed concentration (1mM) of pNPA substrate (Figure S3, Supplementary material). For inhibition studies the enzymes, CA9 or CA12 were pre-incubated with AZA or antibodies at 37 °C for one hour. In all cases, the reaction was initiated by adding the substrate, and the enzyme activity was measured by monitoring the production of colored product, 4-nitrophenol (4-NP/pNP), at 400 nm every 3 min for about 2 h at 37 °C. In all cases, before calculating the percentage of enzyme activity, absorbance of the spontaneous hydrolysis of the substrate alone and also substrate in presence of the antibodies or AZA was subtracted from esterase activity in absence and presence of antibodies or AZA. Slope of the initial velocity of enzyme activity was calculated by plotting absorbance (400 nm) on Y-axis and time (min) on X-axis. The slope of the initial rate of reaction was converted to the percentage of enzyme activity. Percentage of inhibition of antibodies or AZA was calculated by considering the enzyme activity in absence of any inhibitors or antibodies as 100%. The IC50 values were obtained by non-linear least-squares method using PRISM 6 (Graphpad Software Inc., La Jolla, CA).
Results
Inhibition of CA9 and CA12 esterase activity by AZA
CA9 and CA12 esterase activity was inhibited by the well-characterized carbonic anhydrase inhibitor AZA in a dose-dependent manner with an IC50 of 0.14 μM ± 0.1 and 0.27 μM ± 0.1, respectively (), in agreement with the published data on CA1 and CA2Citation33,Citation34 A detailed kinetic characterisation of CA9 and CA12 esterase activity with and without AZA is presented in supplementary material (Figure S1 and Table S2).
Specific binding of antibodies to CA9 and CA12
The antibodies MSC8, MSC12 and 4AB9 bound specifically to CA9 (ELISA) but not to CA12, while the 6A10 antibody bound only to CA12 but not to CA9 (). The human and rat isotype control antibodies and a CA4-specific polyclonal antibody serum did not bind to CA9 or CA12, confirming the specificity of the CA9 and CA12 antibodies.
Antibodies inhibiting CA9 and CA12 esterase activity
Initially, we screened both CA9 and CA12 binding as well as non-binding antibodies at a concentration of 20 μg/ml for their ability to inhibit esterase activity of CA9 and CA12. The non-binding antibodies (human and rat isotype control antibodies as well as the anti-CA4 pAb) and two CA9 binding antibodies (MSC12 and 4AB9) did not inhibit the esterase activity of either CA9 or CA12, whereas MSC8 and 6A10 antibodies showed a significant inhibition of the esterase activity of CA9 and CA12, respectively (). Since none of the non-binding antibodies (tested at 20 μg/ml) inhibited esterase activity, we considered only the binding antibodies for further evaluation at a higher antibody concentration of 40 μg/ml. Even at 40 μg/ml, only MSC8 and 6A10 reproducibly inhibited the CA9 and CA12 esterase activity, respectively, while the other CA9-binding antibodies (MSC12 and 4AB9) did not inhibit CA9 and CA12 esterase activity ().
Antibody dose-dependent inhibition of CA9 and CA12 esterase activity
We further investigated the dose-dependency of MSC8 and 6A10 with regard to inhibition of CA9 and CA12 esterase activity, respectively, which allowed us subsequently to compare and correlate the inhibitory properties of these antibodies as determined in our esterase assay system with the published hydratase activity. Only the MSC8 antibody (CA9-binding and hydratase-inhibiting antibody) exhibited a dose-dependent inhibition of CA9 esterase-activity with an IC50 of 15 μg/ml ± 4, while the antibodies MSC12 (CA9-binding antibody) and 6A10 (CA12-binding and hydratase-inhibiting antibody) did not show any inhibition of the CA9 esterase-activity even at the highest antibody concentration tested (60 μg/ml, ).
Figure 5. Dose-dependent inhibition of CA9 esterase activity with MSC8, MSC12 and 6A10. IC50 of MSC8 is 15 μg/ml ± 4. Data represent three independent experiments each in triplicates.
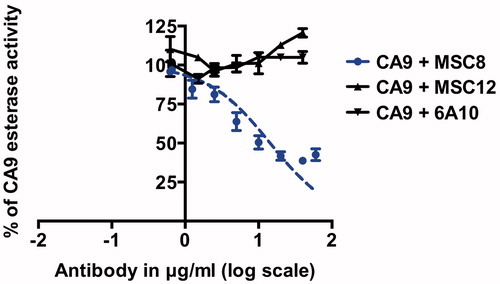
In contrast, dose-dependent inhibition of CA12 esterase-activity was observed only for 6A10 (CA12-binding and hydratase-inhibiting antibody) with an IC50 of 25 μg/ml ± 6, whereas MSC8 (CA9-binding and hydratase-inhibiting antibody) did not inhibit CA12 esterase-activity even at the highest antibody concentration tested (60 μg/ml, ).
Comparison of hydratase and esterase activities
We analyzed the published IC50 values of a series of small molecule compounds () by plotting the correlation between their hydratase and esterase IC50 values on CA1 () and on CA2 ()Citation33,Citation35. The IC50 values of these compounds () showed relatively low correlation between hydratase and esterase activities, R2 = 0.61 for CA1 isoform () and R2 = 0.65 for CA2 isoform (). The correlation plot shows several outliers, compounds that have the varying IC50 (decreasing or increasing) between hydratase and esterase activity on a given CA isoform, while the IC50 correlation pattern of these compounds also varies between the CA1 and CA2 isoforms. The esterase and hydratase IC50 values of MSC8 on CA9 and 6A10 on CA12 are in agreement with the CA1 and CA2 correlation plots or at least in the same order of magnitude. In general, it is noteworthy that the antibodies have much lower IC50 values on both esterase and hydratase activities of CA9 and CA12 compared to the IC50 values of most of the compounds on CA1 and CA2.
Figure 7. Correlation of esterase and hydratase IC50 values; 19 small molecule compounds on CA1 (A) and on CA2 (B). The correlation is indicated by the best-fitted linear curve with its corresponding R2 value calculated using Micro Soft Excel by taking the hydratase IC50 values on the X-axis and esterase IC50 values on the Y-axis. Both (A) and (B) shows the correlation of esterase and hydratase IC50 values of MSC8 on CA9 and 6A10 on CA12. IC50 values of antibodies and 19 small molecule compounds used in this figure are listed in .
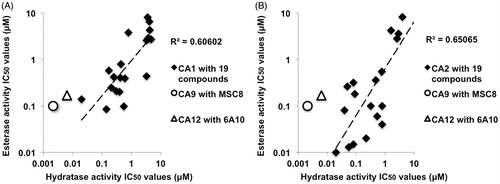
Table 1. IC50 values of 19 small molecule compounds on CA1 and on CA2 were collectively obtained from Ekinci et al.Citation35 and Kasimogullari et alCitation33.
Discussion
We could confirm that CA9 and CA12 have esterase activity, which can be inhibited by AZA in a dose-dependent manner with IC50 values similar to the ones published for CA1 and CA2Citation33,Citation34.
We were able to confirm that MSC8, MSC12 and 4AB9 antibodies specifically bound to CA9, while the antibody 6A10 bound only to CA12. The control antibodies (human isotype, rat isotype and anti-CA4) neither bound CA9 nor CA12. With the exception of MSC8 and 6A10, all the remaining antibodies tested did not inhibit CA9 or CA12 esterase activities, respectively. The finding that MSC8 inhibited the esterase activity of CA9 but not CA12 and that 6A10 inhibited the esterase activity of CA12 but not CA9 confirmed that MSC8 and 6A10 antibodies maintain their specificity for inhibiting the esterase activity similar to what has been published regarding their impact on hydratase activity.
MSC8 and 6A10 antibodies showed a dose-dependent inhibition of esterase activity with IC50 values of 15 μg/ml and 25 μg/ml for their respective targets, CA9 and CA12. However, the esterase IC50 values of MSC8 and 6A10 in this study are higher than the published hydratase IC50 values, 0.32 μg/ml and 0.96 μg/ml, respectivelyCitation20,Citation22. These differences between the esterase and hydratase IC50 values could be ascribed to the use of recombinant human CA9 and CA12 for the present esterase activity assays, whereas the published hydratase activity assays were performed with cell derived membrane fractions containing human CA9 and CA12. In addition, it has been shown that the esterase and hydratase IC50 values may not have the best correlation even with the same compound for a particular CA isoformCitation33,Citation35. Moreover, IC50 values are generally very dependent on several experimental conditions such as the enzyme and substrate concentrations. Note that variations in the IC50 values of MSC8 and 6A10 between hydratase and esterase activities need to be interpreted very carefully as we are comparing the IC50 values of esterase activity from the current data with the IC50 values of hydratase activity from two independent publicationsCitation20,Citation22.
Using the IC50 values of a series of small molecule compounds on CA1 and CA2 isoforms from literature, we demonstrated that the hydratase and esterase IC50 values have a correlation. However, the correlation is low having several outliers with varying IC50 values for the same compound on a given CA isoform, and in addition, the IC50 values of a compound also vary depending on the targeted CA isoformCitation33,Citation35. Such variations in IC50 values suggest that the IC50 values of a given compound do not necessarily have to be identical for both esterase and hydratase activity of a particular carbonic anhydrase isoform. The IC50 values of esterase and hydratase activities of CA9 (MSC8) and CA12 (6A10) also showed a correlation in a similar manner to that of the CA1 and CA2 with small molecule compounds, suggesting that the inhibition (either by small molecules or by antibodies) of esterase and hydratase activities do correlate with each other. The correlation between esterase and hydratase IC50 values suggests that the esterase assay can be employed as a surrogate high-throughput screening method for hydratase activity in order to screen carbonic anhydrase inhibitors or inhibitory antibodies in our case. However, the IC50 correlation being very low, underlines the necessity to confirm the inhibition of hydratase activity of the inhibiting antibodies identified through esterase assay by alternative methods.
Irrespective of the differences in IC50 values between the hydratase and esterase activity, our finding that the antibodies MSC8 and 6A10, which are known to inhibit hydratase activity, can also inhibit the esterase activity of their respective targets, suggests that the esterase surrogate assay has great potential for screening antibodies that block carbonic anhydrase enzyme activity. Inhibition with the MSC8 antibody reached a plateau and did not show complete inhibition of the esterase activity even at the highest concentrations tested; in contrast, the 6A10 antibody continued to provide further inhibition with increasing antibody concentration up to the highest concentration tested. A similar tendency was observed for inhibition of hydratase activityCitation15,Citation20,Citation22, further supporting the notion that these antibodies have similar mode of inhibition for both esterase and hydratase activities. Complete inhibition of CA12 enzyme activity by 6A10 suggests that the antibody might interact or fit much better with the catalytic pocket leading to the complete inhibition, whereas MSC8 might be lacking some of the crucial interactions required for complete inhibition of the CA9 enzymatic activity. It would be very interesting to investigate the structural interactions between the antibodies and CA9 and CA12. Such insights will be helpful for a better understanding of the mode of inhibition and also for the further development of efficient inhibitory antibodies. Both MSC8 and 6A10 antibodies inhibiting the esterase activity under non-physiological conditions follow the same pattern as the hydratase inhibition, suggesting that the inhibition of esterase activity reflects the hydratase inhibition. Even though MSC8 could not completely inhibit the CA9 enzyme activities (both esterase and hydratase), MSC8 showed lower IC50 values than IC50 values of 6A10 on CA12 enzyme activities despite 6A10 showing complete inhibition at higher concentrations. One should be aware that the IC50 values are not necessarily the only and best parameter to quantify the inhibitory capability of the antibodies. While the current manuscript demonstrates a rapid and high-throughput approach to identify inhibitory antibodies, these will need to be further characterized for more precise parameters such as inhibition constant (Ki) and binding affinity, etc.
So far, the esterase activity assay-method of carbonic anhydrases has been extensively explored only in the context of screening small molecule inhibitors against CAsCitation36–38. To our knowledge, we provide the first application of the esterase activity assay of CAs for exploring antibodies inhibiting carbonic anhydrase enzymatic activity. This method is simple and inexpensive and can be performed at high-throughput scale. Therefore, it has great potential for the identification of new CA9 and CA12 inhibiting antibodies, which may eventually lead to the development of new therapeutic antibodies for cancer therapy.
Conclusion
We demonstrate in this study that antibodies inhibiting the hydratase activity of carbonic anhydrases do also inhibit their esterase activity. We conclude that an esterase activity assay for carbonic anhydrases can be used as a facile and powerful screening tool for the identification of novel antibodies that specifically inhibit one or more of the carbonic anhydrase isoforms in a quick, robust and inexpensive manner.
Supplementary material available online.
Supplemental Material.pdf
Download PDF (230 KB)Acknowledgements
We are grateful to Norbert Markuly, Achim Klein and Alessandra Franchino for technical support. We thank the whole 4-Antibody team in Basel and in Jena for antibody production and characterization. Special thanks to Dr. Marc Creus for reviewing the manuscript internally.
Declaration of interest
The authors thank the Commission for Technology and Innovation (CTI), Switzerland, for their financial support, project number 14446.1 PFLS-LS. The co-authors Dr. Volker Seibert and Dr. Marc van Dijk are employees of 4-Antibody AG, Basel, Switzerland (wholly-owned subsidiary of Agenus Inc., USA).
References
- Lindskog S. Structure and mechanism of carbonic anhydrase. Pharmacol Therapeut 1997;74:1–20
- Breton S. The cellular physiology of carbonic anhydrases. JOP 2001;2:159–64
- Neri D, Supuran CT. Interfering with pH regulation in tumours as a therapeutic strategy. Nat Rev Drug Discov 2011;10:767–77
- Chiche J, Ilc K, Laferriere J, et al. Hypoxia-inducible carbonic anhydrase IX and XII promote tumor cell growth by counteracting acidosis through the regulation of the intracellular pH. Cancer Res 2009;69:358–68
- Swietach P, Wigfield S, Cobden P, et al. Tumor-associated carbonic anhydrase 9 spatially coordinates intracellular pH in three-dimensional multicellular growths. J Biol Chem 2008;283:20473–83
- Swietach P, Patiar S, Supuran C, et al. The role of carbonic anhydrase 9 in regulating extracellular and intracellular pH in three-dimensional tumor cell growths. J Biol Chem 2009;284:20299–310
- Svastova E, Hulikova A, Rafajova M, et al. Hypoxia activates the capacity of tumor-associated carbonic anhydrase IX to acidify extracellular pH. FEBS Lett 2004;577:439–45
- Pastorek J, Pastorekova S, Callebaut I, et al. Cloning and characterization of MN, a human tumor-associated protein with a domain homologous to carbonic anhydrase and a putative helix-loop-helix DNA binding segment. Oncogene 1994;9:2877–88
- Zavada J, Zavadova Z, Pastorekova S, et al. Expression of MaTu-MN protein in human tumor cultures and in clinical specimens. Int J Cancer 1993;54:268–74
- Tureci O, Sahin U, Vollmar E, et al. Human carbonic anhydrase XII: cDNA cloning, expression, and chromosomal localization of a carbonic anhydrase gene that is overexpressed in some renal cell cancers. Proc Natl Acad Sci USA 1998;95:7608–13
- Supuran CT. Carbonic anhydrases: novel therapeutic applications for inhibitors and activators. Nat Rev Drug Discov 2008;7:168–81
- Zavada J, Zavadova Z, Pastorek J, et al. Human tumour-associated cell adhesion protein MN/CA IX: identification of M75 epitope and of the region mediating cell adhesion. Br J Cancer 2000;82:1808–13
- Chrastina A, Zavada J, Parkkila S, et al. Biodistribution and pharmacokinetics of 125I-labeled monoclonal antibody M75 specific for carbonic anhydrase IX, an intrinsic marker of hypoxia, in nude mice xenografted with human colorectal carcinoma. International journal of cancer J Int du Cancer 2003;105:873–81
- Chrastina A, Pastorekova S, Pastorek J. Immunotargeting of human cervical carcinoma xenograft expressing CA IX tumor-associated antigen by 125I-labeled M75 monoclonal antibody. Neoplasma 2003;50:13–21
- Battke C, Kremmer E, Mysliwietz J, et al. Generation and characterization of the first inhibitory antibody targeting tumour-associated carbonic anhydrase XII. Cancer Immunol Immunother 2011;60:649–58
- Oosterwijk-Wakka JC, Boerman OC, Mulders PF, Oosterwijk E. Application of Monoclonal antibody g250 recognizing carbonic anhydrase ix in renal cell carcinoma. Int J Mol Sci 2013;14:11402–23
- Muselaers CH, Oosterwijk E, Bos DL, et al. Optimizing lutetium 177-anti-carbonic anhydrase IX radioimmunotherapy in an intraperitoneal clear cell renal cell carcinoma xenograft model. Mol Imaging 2014;13:1–7
- Muselaers CHJ, Stillebroer AB, Desar IME, et al. Tyrosine kinase inhibitor sorafenib decreases 111in-girentuximab uptake in patients with clear cell renal cell carcinoma. J Nucl Med 2014;55:242–7
- Arie S, Belldegrun KC, Kloepfer P, et al. A randomized double blind phase III study to evaluate adjuvant cG250 treatment versus placebo in patients with high-risk ccRCC—Results and implications for adjuvant clinical trials. J Clin Oncol 2013;31:4507
- Gondi G, Mysliwietz J, Hulikova A, et al. Antitumor efficacy of a monoclonal antibody that inhibits the activity of cancer-associated carbonic anhydrase XII. Cancer Res 2013;73:6494–503
- Dekaminaviciute D, Kairys V, Zilnyte M, et al. Monoclonal antibodies raised against 167–180 aa sequence of human carbonic anhydrase XII inhibit its enzymatic activity. J Enzyme Inhib Med Chem 2014;29:804–10
- Murri-Plesko M, Hulikova A, Oosterwijk E, et al. Antibody inhibiting enzymatic activity of tumour-associated carbonic anhydrase isoform IX. Eur J Pharmacol 2011;657:173–83
- Xu C, Lo A, Yammanuru A, et al. Unique biological properties of catalytic domain directed human anti-CAIX antibodies discovered through phage-display technology. PLoS One 2010;5:e9625
- Krishnamurthy VM, Kaufman GK, Urbach AR, et al. Carbonic anhydrase as a model for biophysical and physical-organic studies of proteins and protein-ligand binding. Chem Rev 2008;108:946–1051
- Verpoorte JA, Mehta S, Edsall JT. Esterase activities of human carbonic anhydrases B and C. J Biol Chem 1967;242:4221–9
- Tashian RE, Douglas DP, Yu Y-SL. Esterase and hydrase activity of carbonic anhydrase-I from primate erythrocytes. Biochem Biophys Res Commun 1964;14:256–61
- Gould SM, Tawfik DS. Directed evolution of the promiscuous esterase activity of carbonic anhydrase II. Biochemistry 2005;44:5444–52
- Fierke CA, Calderone TL, Krebs JF. Functional consequences of engineering the hydrophobic pocket of carbonic anhydrase II. Biochemistry. 1991;30:11054–63
- Elleby B, Sjöblom B, Lindskog S. Changing the efficiency and specificity of the esterase activity of human carbonic anhydrase II by site-specific mutagenesis. Eur J Biochem 1999;262:516–21
- Alexander RS, Nair SK, Christianson DW. Engineering the hydrophobic pocket of carbonic anhydrase II. Biochemistry 1991;30:11064–72
- Alexander RS, Kiefer LL, Fierke CA, Christianson DW. Engineering the zinc binding site of human carbonic anhydrase II: structure of the His-94→Cys apoenzyme in a new crystalline form. Biochemistry 1993;32:1510–8. PubMed PMID: 8431430
- Lopez M, Vu H, Wang CK, et al. Promiscuity of carbonic anhydrase II. Unexpected ester hydrolysis of carbohydrate-based sulfamate inhibitors. J Am Chem Soc 2011;133:18452–62
- Kasimogullari R, Bulbul M, Gunhan H, Guleryuz H. Effects of new 5-amino-1,3,4-thiadiazole-2-sulfonamide derivatives on human carbonic anhydrase isozymes. Bioorg Med Chem 2009;17:3295–301
- Iyer R, Barrese AA, IIIrd Parakh S, et al. Inhibition profiling of human carbonic anhydrase II by high-throughput screening of structurally diverse, biologically active compounds. J Biomol Screening 2006;11:782–91
- Ekinci D, Beydemir S, Alim Z. Some drugs inhibit in vitro hydratase and esterase activities of human carbonic anhydrase-I and II. Pharmacol Rep 2007;59:580–7
- Ekinci D, Karagoz L, Ekinci D, et al. Carbonic anhydrase inhibitors: in vitro inhibition of alpha isoforms (hCA I, hCA II, bCA III, hCA IV) by flavonoids. J Enzyme Inhib Med Chem 2013;28:283–8
- Akincioglu A, Akbaba Y, Gocer H, et al. Novel sulfamides as potential carbonic anhydrase isoenzymes inhibitors. Bioorg Med Chem 2013;21:1379–85
- Bayram E, Senturk M, Kufrevioglu OI, Supuran CT. In vitro inhibition of salicylic acid derivatives on human cytosolic carbonic anhydrase isozymes I and II. Bioorg Med Chem 2008;16:9101–5