Abstract
Two different nano- and micro-collagen fiber production methods are introduced and discussed. First one is the electrospinning method, that is very common technique to produce nanofibers from different polymeric solutions and recently collagen solutions are employed to produce nanofibers for different biomedical applications. This technique is extremely versatile method to produce nanofibers in a relatively short time, easy to control the fiber diameter and orientation with small pore sizes and a high surface area. The second method is self-assembly of collagen micro-fibers by co-extrusion method. The collagen fibers are obtained without any cross-linker, by using mainly ionic interactions. We demonstrated that self-assembled collagen fibers have well preserved their native structure (0.90 PP-II fraction), when compared with electrospun collagen fibers (0.38 PP-II fraction). However, it was only possible to produce collagen fibers with nanodimensions by using electrospinning method.
Introduction
Collagen represents the main structural protein in human body that is accounting for approximately 30% of all vertebra body protein. More than 90% of the extracellular protein in the tendon and bone and more than 50% in the skin consist of collagenCitation1. Connective tissue derives prominent features such as mechanical strength and activation of the blood clotting cascade from collagen and its architectural arrangementCitation2,Citation3.
The collagenous spectrum ranges from Achilles tendons to the cornea. Hence, different collagen types are necessary to confer distinct biological features to the various types of connective tissues in the body. Thus, collagen comprises a family of genetically distinct molecules which have a unique triple-helix configuration of three polypeptide subunits known as α-chains in common. Currently, at least 13 types have been isolated which vary in the length of the helix and nature and size of the non-helical portionsCitation4,Citation5. Collagen type I is predominant in higher order animals especially in the skin, tendon, and bone where extreme forces are transmitted. It is a compound of three chains, two of which are identical, termed α1(I), and α2(1) chain with different amino acid composition or it can rarely represent a trimer built of three α1(I) chainsCitation5.
The combined use of two techniques namely electrospray and spinning is made use in a highly versatile technique called electrospinning. Biomedical field is one of the important application areas among others utilizing the technique of electrospinning in tissue engineering and drug-delivery applicationsCitation6. A high electric field is applied to the droplet of a fluid which may be a melt or solution coming out from the tip of a die, which acts as one of the electrodes. This leads to the droplet deformation and finally to the ejection of a charged jet from the tip of the cone accelerating towards the counter electrode leading to the formation of continuous fibers. The important advantages of electrospinning technique are the production of very thin fibers to the order of few nanometers with large surface areas, ease of functionalization for various purposes, superior mechanical properties and ease of process as suggested by many experts in this fieldCitation7–14. The possibility of large scale productions combined with the simplicity of the process makes this technique very attractive for many different applications.
An alternative collagen fiber production method is self-assembly of collagen fibers. The process involves co-extrusion of collagen solution with a fiber formation buffer (FFB) into a bath that contains distilled water. These fibers are reported to have a D period characteristic of collagen in tissuesCitation15,Citation16.
In our previous work, we demonstrated that collagen was completely unfolded in fluorinated solvents and after the spinning process we observed refolding to a certain degree, but PP-II fraction did not exceed 42% in solvent system discussed in the articleCitation17. Moreover, electrospun nanofibers are not ordered similarly to the ones encountered in the Extracellular Matrix (ECM) of living cellsCitation18. Nanofibers are extremely sensitive to the temperature in solution and this could be also one of the important factors that cause discrepancy in experimental results in the literature. It was possible to increase the PP-II fraction of collagen by almost 1.5-fold, just decreasing the preparation temperatureCitation19 for CD experiments by 100. In this article, we would like to highlight the importance of electrospinning and self-assembly methods in drug release applications. We characterized and compared both production methods and products, using CD spectropolarimetry and scanning electron microscopy (SEM) images. Preserving of the native structure was discussed, based on the comparison of PP-II fractions, obtained from CD spectra.
Experimental section
Materials
Collagen type I was the gift from the Kensey Nash Corporation. Potassium chloride (KCl, ≥99.5%), acetic acid (HAc, 99%), ethanol (≥99.8%), sodium chloride (NaCl, ≥99.5%, impurities were insoluble matter), sodium phosphate dibasic (Na2HPO4 ≥99%), and potassium phosphate monobasic (KH2PO4, 99.99%) were purchased from Merck KGaA (Darmstadt, Germany). 2,2,2-trifluoroethanol (TFE, ≥99%) was purchased from Sigma-Aldrich (St. Louis, MO). All chemicals were used as received.
Preparation of collagen solutions
The collagen used in these experiments was a water-insoluble lyophilized foam powder consisting of tropocollagen extracted from bovine dermis (generously donated by the Kensey Nash Corporation, Exton, PA) and was used without further purification. 10% w/v collagen solution was prepared by dissolving collagen in TFE (electrical conductivity: 250 μS/cm, at 25 °C).
Electrospinning procedure
The prepared electrospinning solutions were loaded into a 2 mL syringe (Omnifix, B. Braun, Melsungen, Germany) with a blunt end nozzle, controlled by a syringe pump (Harvard Apparatus Pump 33, Holliston, MA). The solution was pushed through a capillary blunt steel needle (21 gauge, 0.7 mm i.d. × 50 mm length) at a constant speed (0.5 μL/min). The steel needle was connected to a high voltage source (Spellman Bertan Series 205B High Voltage Electronics, NY) by tungsten electrodes. The electric potential is needed to start the spinning process and thus form a jet. The applied DC voltage was held at 18–20 kV. A Cu collector was placed 15 cm from the needle tip to collect the electrospun collagen nanofibers. The nanofiber meshes were collected on cover-glasses placed onto the collector.
Production of self-assembled collagen fibers
Collagen fibers were self-assembled from a collagen solution, using a co-extrusion process. The system consists of two separate syringes. One 5-mL syringe contained a 1% w/v solution of collagen and the other syringe contained a FFB (135 mM NaCl, 30 mM TrizmaBase (Tris), and 5 mM sodium phosphate dibasic, pH 7.4). With the aid of a syringe pump (Harvard Apparatus Pump 33, Holliston, MA), the two solutions were simultaneously extruded into a bath of distilled water through a tubing system.
Characterization
Circular dichroism (CD) spectropolarimetry was employed to characterize the structure of collagen fibers and the samples were illuminated by SEM.
Scanning electron microscopy (SEM)
The morphology of the produced electrospun nanofibers and microfibers were evaluated using QuantaTM 250 FEG Environmental Scanning Electron Microscope (FEI CORPORATE, OR) after Au/Pd sputtering.
Circular dichroism (CD)
CD spectra were recorded using a J-815 spectropolarimeter (Jasco Co., Tokyo, Japan). The instrument was routinely calibrated with a 0.06% (w/v) aqueous solution of ammonium D-10-(+)-camphor sulfonate at 290.5 nm. The spectra were scanned between 260 and 190 at 0.1 nm intervals. Three repeat scans at a scan rate of 10 nm min−1, 8 s response time, and 1 nm bandwidth were averaged for each sample and its respective blank. The collagen nanofiber solutions after electrospinning were prepared by dissolving the weighed-in material in 0.05-M acetic acid. The final concentrations of all CD samples were adjusted to 0.1 mg/mL collagen by diluting the freshly prepared stock solution with an appropriate aliquot of the corresponding solvent and subsequent vortexing. The collagen concentration in the solutions was verified via the absorption of the respective samples measured at 192 nm to ensure the same concentration in all samples. CD spectra were collected using rectangular quartz glass cuvettes with 0.1-cm optical path length. Before testing collagen solution, the baseline spectra of pure 0.05 M acetic acid was used as a blank control for the corresponding native collagen samples. The averaged blank spectrum was subtracted from the averaged sample spectrum to get the corrected line shape. All spectra were recorded at 15 °C, using a rectangular cell holder, connected to a water thermostat. The heating rate was fixed to 0.5 K/min, and at each selected temperature a 3 min delay was implemented to ensure thermal equilibrium in the cell. CD spectra were smoothened by the adaptive smoothing method, which is part of the Jasco Spectra Analysis software.
Results and discussion
The secondary structure of collagen fibers were analyzed by CD spectropolarimetry and fibers were illuminated by SEM.
SEM results
demonstrates the Nicon Camera images of self-assembled collagen fibers. The fiber diameters are controlled by the inner diameter of tubing system that was used in co-extrusion process. The fibers are randomly oriented, having spaghetti-like appearance, and in white color after vacuum drying process. They have a porous structure. When it gets wet, it behaves as a gel and swells.
Figure 1. Images of self-assembled collagen fibers (a) after vacuum dry and (b) in wet conditions: swelled and porous structures could be seen easily.
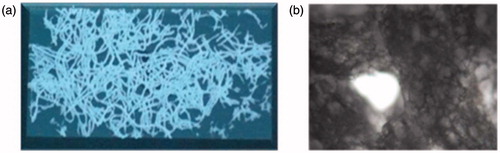
shows (a) the SEM images of electrospun collagen nanofiber network, (b) the fiber dimensions in the network and (c) SEM images of self-assembled collagen fibers. While electrospun collagen nanofibers have smooth surface and cylindrical morphology, self-assembled collagen fibers consist of many fibrils, wrapped together. The nanofiber dimensions in electrospinning process mainly defined by the solution concentration and solvent type. The collagen fiber dimensions in self-assembly process defined by the inner diameter of the tubing system. Electrospun collagen nanofibers with 150–200 nm diameter were obtained from 10% collagen solution by employing TFE as the solvent (). Self-assembled collagen fibers with 10 μm diameter were obtained by using PTFE tubing with 10 μm inner diameter.
Circular dichroism results
CD utilizes the differential absorption of left- and right- handed circular polarized light in an asymmetric environment to assess secondary structure. The CD spectra of self-assembled collagen fibers, electrospun collagen nanofibers from TFE and native collagen in 0.05 M HAc are presented in ), respectively.
Figure 3. CD spectra of (a) electrospun collagen nanofibers, (b) self-assembled collagen fibers, and (c) native collagen. They all obtained by solubilizing 0.1 mg/mL material in 0.05 M HAc.
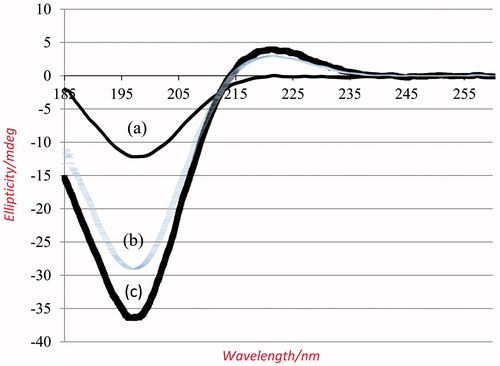
We employed Ackerman et al. methodCitation20 to extract the fraction of triple helix, mainly relying on the equilibrium state of collagen spectra. The fraction of folded collagen (θ) was defined as
(1)
where θobs, θt and θu represented the ellipticities of observed, triple helix and unordered state, respectively, and measured at 15 °C and 90 °C, respectively, in 0.05 M HAc. The PP-II peak at 221.5 nm exhibited positive values at 15 °C and transformed to a negative ellipticity at 90 °C, which indicated that collagen completely unfolded at that temperature. Obviously, ellipticities at temperatures between 15 °C and 90 °C represented a combination of triple helix and random coil structuresCitation17. shows the spectra of (a) the electrospun collagen nanofibers from TFE, (b) self-assembled collagen fibers, and (c) native collagen in HAc at 15 °C. Native collagen solution at 15 °C displayed the highest amount of PP-II fraction, whereas collagen nanofiber solution from TFE displayed the lowest amount of PP-II. The self-assembled collagen solution scored the very high PP-II fraction, close to the native collagen. The θ fraction is 0.38 for electrospun nanofibers obtained from TFE and 0.90 for self-assembled collagen fibers.
Conclusions
Collagen is a biodegradable material and easily absorbed in the body. Since collagen is a part of the body, it is non-antigenic, a non- toxic biopolymer, hemostatic in nature and encourage blood coagulation and shows better biocompatibilityCitation21. Collagen can be regenerated in a number of different forms, such as foams, films and nanofibers and compatible with synthetic polymers. Nanofibers with high surface area and porosity have enormous scope for applications in different bioengineering fields that require mechanically stable and biologically functional tissue scaffoldsCitation21. The material properties can be suitably tailored by varying the fiber orientation and solution concentrationCitation22. These electrospun collagen fibrils closely resembled the structural and biological properties of natural polymer tissues. Nanofiber mats due to their high functional characteristics find application as drug-carriers for the drug delivery systemCitation23.
In this article, we investigated the structure of collagen fibers that were obtained by using different regeneration methods, namely electrospinning and self-assembly. Two different characterization methods were employed; SEM and CD Spectropolarimetry. The experimental results were compared with the structure of native collagen. As we demonstrated in our previous publications, after the spinning process, the PP-II fraction did not exceed 42%Citation17,Citation18 and when we paid special attention to humidity and solubilizing temperatures, 63% PP-II fraction was obtained from HAcCitation19. In this publication, we were able to increase PP-II fraction up to 90% by using self-assembly of collagen fibers.
Controlled delivery of drugs at a defined rate over a defined period is possible with biocompatible matrices of either electrospun collagen nanofibers or self-assembled collagen fibers. It is also shown that the release of tetracycline from the electrospun mats was found much greater than from the cast filmsCitation23. However, there is still a need to crosslink electrospun nanofibers in order to have more control on drug release rate. Biodegradation rate of the electrospun collagen fibers will be depend on the crosslinking density.
Acknowledgements
The authors would like to thank Dr Jochen Bürck and Bianca Posselt for the CD measurements (IBG2, Karlsruhe Institute of Technology, Germany).
Declaration of interest
This study is supported by TUBITAK’s Co-Funded Brain Circulation Program (2236, Project Number: 112C025). The authors declare that there are no competing interests.
References
- Piez KA. Collagen. In: Kroschwitz JI, ed. Encyclopedia of polymer science and engineering. New York: Wiley; 1985:699–727
- Barnes MJ. The collagen–platelet interaction. In: Weiss JB, Jayson MIV, eds. Collagen in health and disease. Edinburgh: Churchill Livingstone; 1982:179–97
- Nimni ME, Harkness RD. Molecular structures and functions of collagen. In: Nimni ME, ed. Collagen Vol. I – biochemistry. Boca Raton (FL): CRC Press; 1988:1–79
- Kucharz EJ. The collagens: biochemistry and pathophysiology. Berlin: Springer–Verlag; 1992:7–29
- Miller EJ. Collagen types: structure, distribution and functions. In: Nimni ME, ed. Collagen Vol. I – biochemistry. Boca Raton (FL): CRC Press; 1988:139–57
- Vasita R, Katti DS. Nanofibers and their applications in tissue engineering. Int J Nanomed 2006;1:15–30
- Greiner A, Wendorff JH. Electrospinning: a fascinating method for the preparation of ultrathin fibers. Angewandte Chem 2007;46:5670–703
- Boudriot U, Dersch R, Greiner A, Wendorff JH. Electrospinning approaches toward scaffold engineering–a brief overview. Artif Organs 2006;30:785–92
- Teo WE, Ramakrishan S. A review on electrospinning design and nanofibre assemblies. Nanotechnology 2006;17:R89–106
- Dersch R, Graeser M, Greiner A, Wendorff JH. Electrospinning of nanofibres: towards new techniques, functions, and applications. Aust J Chem 2007;60:719–28
- Greiner A, Wendorff JH, Yarin AL, Zussman E. Biohybrid nanosystems with polymer nanofibers and nanotubes. Appl Microbiol Biotechnol 2006;71:387–93
- Ko FK. Nanomaterials handbook. Boca Raton (FL): CRC Press LLC; 2006:553–64
- Tan S, Huang S, Wu B. Some fascinating phenomena in electrospinning processes and applications of electrospun nanofibers. Polym Int 2007;56:1330–9
- Burger C, Hsiao BS, Chu B. Nanofibrous materials and their applications. Annu Rev Mater Res 2006;36:333–68
- Landis WJ, Silver FH, Freeman JW. Collagen as a scaffold for biomimetic mineralization of vertebrate tissues. J Mater Chem 2006;16;1495–1503
- Pins GD, Huang EK, Christiansen DL, Silver FH. Effects of static axial strain on the tensile properties and failure mechanisms of self-assembled collagen fibers. J Appl Polym Sci 1997;63:1429–40
- Bürck J, Heissler S, Geckle U, et al. Recemblance of electrospun collagen nanofibers to their native structure. Langmuir 2013;29:1562–72
- Diesner M-O, Welle A, Kazanci M, et al. In vitro observation of dynamic ordering processes in the extracellular matrix of living, adherent cells. Biointerphases 2011;6:171–9
- Kazanci M. Solvent and temperature effects on folding of electrospun collagen nanofibers. Mater Lett 2014;130:223–6
- Ackerman MS, Bhate M, Shenoy N, et al. Sequence dependence of the folding of collagen -like peptides- Single amino acids affect the rate of triple-helix nucleation. Biol Chem 1999;274:7668–73
- Li W, Laurencin CT, Caterson, EJ, et al. Electrospun nanofibrous structure: a novel scaffold for tissue engineering. J Biomed Mater Res 2002;60:613–21
- Boland ED, Wnek GE, Simpson DG, et al. Tailoring tissue engineering scaffolds using electrostatic processing techniques. J Macromol Sci-Pure Appl Chem 2001;A38:1231–43
- Kenawy E-R, Bowlin GL, Mansfield K, et al. Release of tetracycline hydrochloride from electrospun poly(ethylene-co-vinylacetate), poly(lactic acid), and a blend. J Control Rel 2002;17:57–64