Abstract
The field of multivalent inhibition of enzymes is growing exponentially from the first reported multivalent effect on a glycosidase enzyme. However, the investigations have generally remained restricted to carbohydrate-processing enzymes. Carbonic anhydrases are ubiquitous metallo-enzymes involved in many key biological processes, that catalyze the reversible hydration/dehydration of . This study reports the first synthesis of multimeric xanthates addressing the selectivity and potency of CA multivalent inhibition. Six multivalent compounds containing three, four, and six xanthate moieties were prepared and assayed against four relevant CA isoforms together with their monovalent analogues. Some of the multimers were stronger inhibitors than the monomeric species. For hCA I, the two best molecules 18 and 20 showed an improvement of the ligand affinity of 4.8 and 2.3 per xanthate units (valence-corrected values), respectively, which corresponds to a clear multivalent effect. Moreover, the biochemical assays demonstrated that the multimeric presentation of xanthates, also affected the selectivity of the relative inhibition among the four CAs assayed.
Introduction
Carbonic anhydrases (CAs, EC 4.2.1.1), which are ubiquitous Zn-based metallo-enzymes catalyzing the reversible hydration/dehydration of carbon dioxide/bicarbonate, play an essential role in numerous physiological processes. In animals, they are critical in vital processes such as respiration, pH regulation, biosynthesis and calcification. Fifteen different human CA (hCA) isoforms and CA-related proteins (CARPs), belonging to the α-class and differing mainly in their location within the cell and tissues, have been reported to dateCitation1. CA inhibitors (CAIs) are already employed as diuretics and anti-glaucoma drugs. Furthermore, they are promising as anticonvulsant, anti-obesity, anticancer, and anti-virulent agents, among othersCitation2. Some CAIs, i.e. coumarinsCitation3 and lacosamideCitation4, inhibit the enzyme activity by occluding the entrance of the catalytic site and ortho-substituted benzoic acid derivatives can inhibit CA without entering the active site at allCitation5. However, most CAIs act by binding either to the Zn(II) cation, inside of the active site and crucial for the catalytic activity, or to the water molecule coordinated to itCitation6. Namely, the classical sulfonamides and their isosteres, phenols, thiophenols, polyamines, and metal complexing anions. Belonging to this last group of anionic compounds, several families of CAIs displaying the zinc-binding group (ZBG) have been recently reportedCitation7–10. By crystallography, it has been proven that this ZBG coordinates the Zn(II) from the CA active site through one of the sulphur atoms; the same S forms a hydrogen bond with the OH of amino acid residue Thr199 and other S interacts also through hydrogen bond with the NH group of the same residueCitation7. This binding mode explains the good inhibition rates of these compounds for various CA tested to dateCitation8. Three representative families containing this ZBG, i.e. dithiocarbamates, trithiocarbonates, and xanthates () have been intensively investigated.
Figure 1. Metal complexing anions containing the ZBG : dithiocarbamates, trithiocarbonates and xanthates.
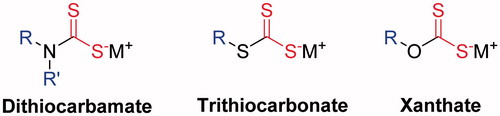
They display a wide range of inhibitory powers depending on the organic moiety present in the molecule and its orientation within the active site cavity, which promote distinct interactions with the surrounding amino acidsCitation7. Therefore, as the active site of all the CA isoforms is highly conservedCitation11, these kind of recently discovered CAIs offer the possibility of designing more selective and potent agentsCitation8. Herein we targeted the synthesis and study of multivalent alkali-metal xanthates as CAIs from distinct polyols.
Multivalency has gained much importance in the last years, particularly in the fields of biomedicine and bioorganic synthesis. The critical role of multivalency in essential biological phenomena is only starting to be unravelled nowCitation12. Many natural processes, particularly at the membranes interface, benefit from the multivalent display of biological entities to boost the efficacy of binding events. Especially, carbohydrate–protein interactions are notably weak at the monomer level, but can be tremendously enhanced if the ligand is displayed in a multivalent wayCitation13. Scientists have extensively studied the biophysical interactions among natural systems and created a plethora of artificial multivalent systems in order to understand the underlying mechanisms of multivalencyCitation14–22. The most scrutinized systems are likely lectin–carbohydrate dyads. Lectins are non-processing but only carbohydrate-binding proteins often expressing multiple binding sites. Since some biologically relevant lectins are involved in pathological processes, their inhibition can be of therapeutic interestCitation20–24. For instance, the adhesion of pathogenic bacteria to cells can be inhibited to prevent the bacterial infectionsCitation25–32. Nevertheless, the field of multivalent enzyme inhibition (MEI) has been barely explored until 2009, when a clear multivalent effect (and an increase in selectivity) was determined in α-mannosidase inhibition by iminosugar clustersCitation33. The term “multivalent effect”, generally only employed with carbohydrate-binding proteins, could then be applied to the field of carbohydrate-processing enzymes. After this groundbreaking result, considerable amount of research has been reported, although almost exclusively with carbohydrate-processing enzymes such as glycosidasesCitation34–42 and glycosyltranferasesCitation43.
In a recent work, we reported the inhibitory study of coumarin-derivatized fullerene hexakis-adducts toward a set of biologically relevant hCA isoformsCitation44. These multimeric compounds did not show strong multivalent effects, however they were always more potent than the respective monovalent analogues. Furthermore, the selectivity of the multimeric species varied with respect to the monovalent ones. MEI seems to be highly depending on the structure of the multivalent inhibitor: the valency, the topology, the structure of the central core and the length and rigidity of the spacer to which each inhibitor is grafted to the scaffold are parameters that must be fine-tuned to optimize a multivalent inhibition. Herein, we decided to explore both different multivalent scaffolds and CAI. A small collection of xanthate-derivatized compounds were prepared from multivalent polyol-scaffolds (). The multivalent xanthates contained either three, four or six functionalities. In order to asses a possible multivalent effect, the monovalent analogues for each of the linkers used were also prepared. All the compounds were assayed in vitro for inhibition against the cytosolic hCA I and II as well as the transmembrane, tumor-associated hCA IX and XII. Interestingly, hCA IX and XII could be targeted by multivalency since crystallographic studies have demonstrated that they both present a dimeric extracellular domainCitation45,Citation46. Therefore, inhibition of these two isoforms could be enhanced by multivalent mechanisms such as chelation if an appropriate design of the inhibitor is achieved. Other few previous works have explored the multivalent inhibition of CAs. For instance, Whitesides and collaborators studied the dependence of avidity on spacer length in bivalent model systemsCitation47. Also, very recently, Winum and co-workers analyzed the inhibition of multivalent CAIs-decorated silica nanoparticlesCitation48. Nevertheless, as far as we know, this study reports the first synthesis of multimeric xanthates as CAIs, designed to address the selectivity and the potency of CA multivalent inhibition.
Materials and methods
Chemistry
All the synthetic procedures are described in the Experimental Supporting Information (ESI). NMR spectra of all the non-previously reported compounds are also shown in the ESI.
CA inhibition
An SX.18MV-R Applied Photophysics stopped-flow instrument was used for assaying the CA-catalyzed CO2 hydration activity by using the method of KhalifahCitation49. Inhibitor and enzyme were pre-incubated for 15 min prior to assay. IC50 values were obtained from dose response curves working at seven different concentrations of test compound (from 0.1 nM to 50 µM), by fitting the curves using PRISM (www.graphpad.com) and non-linear least squares methods, values representing the mean of at least three different determinations, as described earlier by usCitation50,Citation51. The inhibition constants (KI) were then derived by using the Cheng–Prusoff equation, as follows: Ki = IC50/(1 + [S]/Km) where [S] represents the CO2 concentration at which the measurement was carried out, and Km the concentration of substrate at which the enzyme activity is at half maximal. All enzymes used were recombinant, produced in E. coli as reported earlier. The concentrations of enzymes used in the assay were: hCA I, 10.1 nM; hCA II, 8.4 nM; hCA IX, 7.9 nM and hCA XII, 12.5 nMCitation52,Citation53.
Results
Chemistry
The preparation of the monovalent analogues, as shown in Scheme 1, started from commercially available 3-chloropropan-1-ol and 2-(2-chloroethoxy)ethan-1-ol and followed parallel pathways. Both molecules were azidated by substitution of the chloride leaving group to provide 1 and 5, respectively, which were engaged in the synthesis of two distinct types of molecules. On the one hand, 1 and 5 were reacted with KOH and CS2 in Et2O, as reported in the literature for simple hydroxyl groupsCitation8, giving the desired monovalent xanthates 2 and 6 as yellowish precipitates. In addition, 1 and 5 were clicked to 3-methoxyprop-1-yne to afford 3 and 7, which were transformed in the monovalent xanthates analogues 4 and 8, respectively, through reaction with CS2 and KOH in DMSO/H2O 10:1. This last procedure was optimized since the use of the same conditions as for the monovalent analogues 2 and 6, did not afford the desired analogues in good yields. Compounds 1–8 were assayed against the hCAs studied to determine the importance of the presence of a triazole moiety and to assess the existence of a possible multivalent effect when compared to multimers.
Scheme 1. Synthesis of the monovalent analogues. Reagents and conditions: (a) NaN3, H2O, 18 h, 80 °C (1: from 3-chloropropan-1-ol, 95%; 5: from 2-(2-chloroethoxy)ethan-1-ol, 99%); (b) CS2, KOH, Et2O, 3 h, r.t. (2: from 1, 82%; 6: from 5, 83%); (c) 3-methoxyprop-1-yne, CuSO4/NaAsc, THF/H2O, o/n, r.t. (3: from 2, 99%; 7: from 6, 99%); (d) CS2, KOH, DMSO/H2O 10:1, 2 h, r.t. (4: from 3, 84%; 8: from 7, 77%).
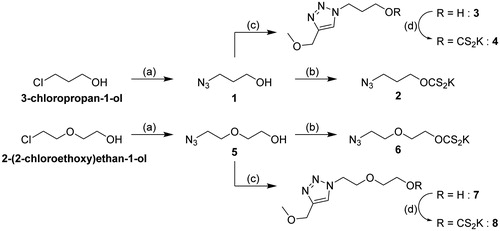
The synthesis of the multivalent xanthates started with the preparation of the multivalent alkyne-derivatized scaffolds that would be later functionalized to finally provide the desired xanthates clusters. As shown in Scheme 2, the three scaffolds were obtained in one step from commercially available starting materials in good to very good yields in comparison to previously reported reactions in bibliographyCitation54–56.
The synthesis of the multivalent xanthates from the formerly produced scaffolds was achieved in two steps, as depicted in Scheme 3, since the direct click of the azidated xanthates 2 and 6 was not feasible. Indeed, xanthates strongly coordinates Cu, thus a high excess of Cu complex must be added to catalyze the click reaction but it cannot be fully removed afterwards. Therefore, the first step was straightforward and involved the grafting of the two different hydroxyl azidated-linkers 1 and 5 through CuAAC reaction. The click reaction was either realized overnight at r.t. or under microwave irradiation at 80 °C for 2 h with similar outcomes. After purification of the products by simple co-precipitation and removal of the residual Cu salts by scavenging (QuadrasilMP®, Sigma Aldrich, St. Louis, MO), the xanthates were prepared with the optimized conditions in very good to excellent yields, considering the number of reactions per molecule.
Scheme 3. Synthesis of the multivalent xanthates. Reagents and conditions: (a) 1 or 5, CuSO4/NaAsc, 1,4-dioxane/H2O, o/n, r.t. or 2 h at 80 °C (9: from 1, 70%; 10: from 5, 67%; 11: from 1, 96%; 12: from 5, 79%; 13: from 1, 90%; 14: from 5, 90%); (b) CS2, KOH, DMSO/H2O, 2 h, r.t. (15: from 9, 90%; 16: from 10, 89%; 17: from 11, 97%; 18: from 12, 71%; 19: from 14, 74%; 20: from 14, 83%).
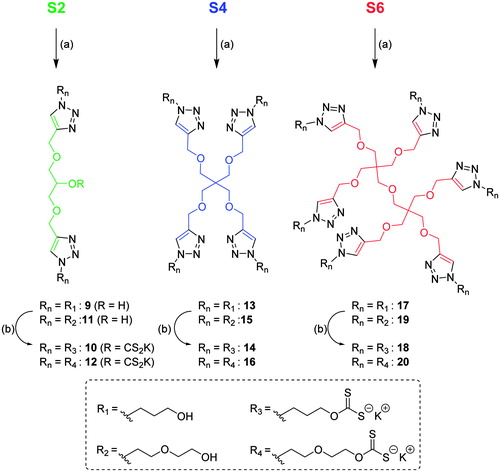
In order to develop an efficient methodology for the preparation, isolation and characterization of the desired multivalent xanthates, an intensive optimization of the procedure was required. It is worth to note that, as commented above, xanthates are not very stable molecules; they are known to be sensitive to acidic pH, high temperature, humidity and timeCitation57. The degradation process occurs through complex radical reactions giving the starting free hydroxyl and CS2 as products, among others. Contrarily to the synthesis of the monovalent analogues, herein it was not intended that the xanthate products precipitated during the course of the reaction. Precipitation would provide a mixture of partially functionalized polyols. Therefore, after screening of solvents, DMSO was eventually selected as the preferred solvent because it can solubilize the final compounds, thus avoiding premature precipitation of partially functionalized polyols.
The completion of the reaction could be followed by 1H NMR in DMSO-d6 thanks to the chemical shifts of the peak of the CH2 next to the hydroxyl groups (δ ≈ 3.60 ppm) which was converted into -OCS2K (δ ≈ 4.30 ppm). For the first tests, 1 eq/-OH of KOH and CS2 were added, but the reactions were very slow and not complete after several days. When an excess of both KOH and CS2 was used, the reactions were complete in 2 h, but some side-products were always produced and were found impossible to be separated by selective precipitation. Based on these observations, we eventually developed a satisfactory procedure which included an excess of CS2, since it can be removed by evaporation, and one equivalent of KOH per hydroxyl groups. Importantly, the KOH was finely crushed before the reaction and a few drops of water were added to the reactant mixture at the beginning of the reaction. After 2 h at r.t., the reactions were complete and the products able to be selectively precipitated with THF. The precipitates were washed three times with THF. To remove the last impurities and the traces of DMSO, the precipitates were dissolved in MeOH and rapidly precipitated again with Et2O. The products were extensively dried under high vacuum to remove any traces of solvent which could lead to degradation. The characterization of all the molecules was carried out unambiguously by 1H NMR, 13C NMR and HRMS.
CA Inhibition assays
The monovalent compounds 1–8 and the multivalent compounds 9–20 were assayed in vitro for inhibition against the four physiologically relevant CA isoforms, the cytosolic hCA I and II as well as the transmembrane, tumor-associated hCA IX and XII. The results for the compounds which presented some inhibition are shown in . Acetazolamide (5-acetamido-1,3,4-thiadiazole-2-sulfonamide) was used as a standard in the assayCitation49.
Table 1. Inhibition constants (KIs) by a stopped flow CO2 hydrase assay, with acetazolamide AAZ as standard drugCitation48.
Among the monovalent compounds, the alcohols 1, 3, 5 and 7 did not show any inhibitory effects against the four CA isoforms, as expected. The xanthates 2, 4, 6 and 8 on the other hand showed inhibitory effects (). The isoform more sensitive to these compounds was hCA I, for which the monovalent xanthates showed KIs ranging between 7.5 and 51.7 µM. The organic scaffold did not show much influence in the case of xanthates 2, 6 and 8, which had very similar inhibition constants whereas 4, which incorporates the substituted triazole moiety (present also in 8) but a shorter linker between the ring and the ZBG (compared to 8), was an order a magnitude a weaker CAI compared to the other monovalent xanthates prepared here. hCA II was not at all inhibited by these xanthates (and alcohols) up to 100 µM concentrations in the assay system, and the same situation was observed for the inhibition of hCA IX, except for 8, which was a low micromolar inhibitor (KI of 9.9 µM). Against hCA XII xanthates 2 and 6 showed some degree of inhibition (KIs of 9.4 – 93.9 µM).
For the multivalent compounds 9–20 reported here, the inhibition data were again more interesting against the slow cytosolic isoform hCA I. Molecules 9, 10, 12, 13, 15, 17 and 19 did not show any inhibitory effect. Against isoform hCA I, the alcohol 11 and the xanthate 14 were weak micromolar inhibitors (KIs of 70.8 – 82.9 µM). On the other hand, the polyxanthates derived from S6, 18 and 20, showed low-micromolar or submicromolar inhibitory action (KIs of 0.53 – 1.80 µM). Thus, in this case a very interesting multivalent effect is observed for the inhibition of hCA I, since the corresponding monomeric compounds were much less effective enzyme inhibitors compared to the multivalent xanthates. Indeed, the relative potency values of 18 and 20 were found to be 29 and 14, respectively (). Taking into account the valency of these hexameric species, the enhancement of the affinity per xanthate unit corresponds to 4.8 and 2.3, which is already significant for multivalent enzyme inhibitors. The same two xanthates, 18 and 20, were also inhibiting hCA II, but orders of magnitude less efficiently compared to hCA I (KIs of 66.1–68.9 µM). None of the other multivalent derivatives possessed inhibitory activity against this isoform. For hCA IX, three multivalent xanthates (16, 18 and 20) showed inhibition, with KIs ranging between 8.1 and 39.0 µM. Interestingly, hCA XII was not inhibited by the multivalent xanthates, and only the alcohol 11 showed a weak inhibitory action.
Discussion and conclusions
We designed monovalent alcohols/xanthates 1–8 and three series of multivalent derivatives 9–20 in order to investigate multivalent inhibition of CA isoforms involved in crucial physiologic and pathologic processes. An optimized procedure for the synthesis of multivalent xanthates was developed providing all the desired molecules in very good to excellent yields. Starting from readily available commercial materials, all the molecules, even the complex multimeric xanthates, were produced in three or less steps. The characterization was performed unambiguously by 1H NMR, 13C NMR and HRMS.
Some of the multivalent compounds, containing three, four, and six xanthate moieties showed interesting CA inhibitory activity, mainly against hCA I, an isoform whose physiologic function is still poorly understood. Together with their monovalent analogues, some of the multimers were significantly stronger inhibitors than the monomeric species although no strong “multivalent effect” was found in general (as compared to carbohydrate-lectin interactions). All four CA isoforms on which we tested the xanthates have been crystallized alone or in adduct with various classes of inhibitors. The two cytosolic isoforms hCA I and II are monomers whereas the two transmembrane ones are dimers with two accessible active sites. However, as shown from the data in the , both monomeric as well as dimeric isoforms were inhibited in a rather similar manner by the multivalent derivatives reported in the paper. The best hCA I inhibitors in the reported series had inhibition constants of 0.53 – 1.80 µM, and they were two orders of magnitude less efficient against the physiologically dominant cytosolic isoform hCA II. Thus, an interesting class of hCA I-selective inhibitors was detected in this work. Nonetheless, the biological assays demonstrated that the multimeric presentation of xanthates, indeed affected the selectivity of the relative inhibition among the four CAs assayed, and may open new directions in the research of isoform selective CAIs binding to the metal ion from the enzyme active site.
Supplementary material available online
Supplementary Information
IENZ_1072177_Supp.pdf
Download PDF (2.8 MB)Acknowledgements
We are grateful to Dr. Abdellatif Tikad for technical support.
Declaration of interest
The authors report no conflict of interest. We thank the People Programme (Marie Curie Actions) of the European Union’s Seventh Framework Programme “DyNano” FP7/2007–2013/under REA grant agreement no. 289033 for the financial support.
References
- Pastorekova S, Parkkila S, Pastorek J, Supuran CT. Carbonic anhydrases: current state of the art, therapeutic applications and future prospects. J Enzyme Inhib Med Chem 2004;19:199–229
- Alterio V, Di Fiore A, D'Ambrosio K, et al. Multiple binding modes of inhibitors to carbonic anhydrases: how to design specific drugs targeting 15 different isoforms? Chem Rev 2012;112:4421–68
- Vu H, Pham NB, Quinn RJ. Direct screening of natural product extracts using mass spectrometry. J Biomol Screen 2008;13:265–75
- Temperini C, Innocenti A, Scozzafava A, et al. The coumarin-binding site in carbonic anhydrase accommodates structurally diverse inhibitors: the antiepileptic lacosamide as an example and lead molecule for novel classes of carbonic anhydrase inhibitors. J Med Chem 2010;53:850–4
- D'Ambrosio K, Carradori S, Monti SM, et al. Out of the active site binding pocket for carbonic anhydrase inhibitors. Chem Commun 2015;51:302–5
- Maresca A, Temperini C, Pochet L, et al. Deciphering the mechanism of carbonic anhydrase inhibition with coumarins and thiocoumarins. J Med Chem 2010;53:335–44
- Carta F, Aggarwal M, Maresca A, et al. Dithiocarbamates: a new class of carbonic anhydrase inhibitors. Crystallographic and kinetic investigations Chem Commun 2012;48:1868–70
- Carta F, Akdemir A, Scozzafava A, et al. Xanthates and trithiocarbonates strongly inhibit carbonic anhydrases and show antiglaucoma effects in vivo. J Med Chem 2013;56:4691–700
- Innocenti A, Scozzafava A, Supuran CT. Carbonic anhydrase inhibitors. Inhibition of cytosolic isoforms I, II, III, VII and XIII with less investigated inorganic anions. Bioorg Med Chem Lett 2009;19:1855–7
- Innocenti A, Scozzafava A, Supuran CT. Carbonic anhydrase inhibitors. Inhibition of transmembrane isoforms IX, XII, and XIV with less investigated anions including trithiocarbonate and dithiocarbamate. Bioorg Med Chem Lett 2010;20:1548–50
- Supuran CT, Scozzafava A. Carbonic anhydrase inhibitors and their therapeutic potential. Expert Opin Ther Pat 2000;10:575–600
- Varki A, Cummings RD, Esko JD, et al. Essentials of glycobiology. 2nd ed. New York: Cold Spring Harbor; 2009
- Fasting C, Schalley CA, Weber M, et al. Multivalency as a chemical organization and action principle. Angew Chem Int Ed Engl 2012;51:10472–98
- Pieters RJ. Maximising multivalency effects in protein-carbohydrate interactions. Org Biomol Chem 2009;7:2013–25
- Lahmann M. Architectures of multivalent glycomimetics for probing carbohydrate–lectin interactions. Top Curr Chem 2009;288:17–65
- Chabre YM, Roy R. Design and creativity in synthesis of multivalent neoglycoconjugates. Adv Carbohydr Chem Biochem 2010;63:165–393
- Sanchez-Navarro M, Munoz A, Illescas BM, et al. [60]Fullerene as multivalent scaffold: efficient molecular recognition of globular glycofullerenes by concanavalin A. Chem Eur J 2011;17:766–9
- Reymond J-L, Darbre T. Peptide and glycopeptide dendrimer apple trees as enzyme models and for biomedical applications. Org Biomol Chem 2012;10:1483–92
- Zeng X, Andrade CA, Oliveira MD, Sun XL. Carbohydrate-protein interactions and their biosensing applications. Anal Bioanal Chem 2012;402:3161–76
- Bernardi A, Jiménez-Barbero J, Casnati A, et al. Multivalent glycoconjugates as anti-pathogenic agents. Chem Soc Rev 2013;42:4709–27
- Adak AK, Lin H-J, Lin C-C. Multivalent glycosylated nanoparticles for studying carbohydrate-protein interactions. Org Biomol Chem 2014;12:5563–73
- Cecioni S, Imberty A, Vidal S. Glycomimetics versus multivalent glycoconjugates for the design of high affinity lectin ligands. Chem Rev 2015;115:525–61
- Deniaud D, Julienne K, Gouin SG. Insights in the rational design of synthetic multivalent glycoconjugates as lectin ligands. Org Biomol Chem 2011;9:966–79
- Renaudet O, Roy R. Multivalent scaffolds in glycoscience: an overview. Chem Soc Rev 2013;42:4515–17
- Imberty A, Chabre YM, Roy R. Glycomimetics and glycodendrimers as high affinity microbial anti-adhesins. Chem Eur J 2008;14:7490–9
- Durka M, Buffet K, Iehl J, et al. The functional valency of dodecamannosylated fullerenes with Escherichia coli FimH--towards novel bacterial antiadhesives. Chem Commun 2011;47:1321–3
- Hartmann M, Lindhorst TK. The bacterial lectin fimh, a target for drug discovery – carbohydrate inhibitors of type 1 fimbriae-mediated bacterial adhesion. Eur J Org Chem 2011;2011:3583–609
- Hartmann M, Papavlassopoulos H, Chandrasekaran V, et al. Inhibition of bacterial adhesion to live human cells: activity and cytotoxicity of synthetic mannosides. FEBS Lett 2012;586:1459–65
- Nierengarten I, Buffet K, Holler M, et al. A mannosylated pillar[5]arene derivative: chiral information transfer and antiadhesive properties against uropathogenic bacteria. Tetrahedron Lett 2013;54:2398–402
- Kouki A, Pieters RJ, Nilsson UJ, et al. Bacterial adhesion of streptococcus suis to host cells and its inhibition by carbohydrate ligands. Biology 2013;2:918–35
- Luczkowiak J, Munoz A, Sanchez-Navarro M, et al. Glycofullerenes inhibit viral infection. Biomacromolecules 2013;14:431–7
- Boukerb AM, Rousset A, Galanos N, et al. Antiadhesive properties of glycoclusters against Pseudomonas aeruginosa lung infection. J Med Chem 2014;57:10275–89
- Diot J, Garcia-Moreno MI, Gouin SG, et al. Multivalent iminosugars to modulate affinity and selectivity for glycosidases. Org Biomol Chem 2009;7:357–63
- Compain P, Decroocq C, Iehl J, et al. Glycosidase inhibition with fullerene iminosugar balls: a dramatic multivalent effect. Angew Chem Int Ed Engl 2010;49:5753–6
- Cagnoni AJ, Varela O, Gouin SG, et al. Synthesis of multivalent glycoclusters from 1-thio-beta-d-galactose and their inhibitory activity against the beta-galactosidase from E. coli. J Org Chem 2011;76:3064–77
- Decroocq C, Rodríguez-Lucena D, Ikeda K, et al. Cyclodextrin-based iminosugar click clusters: the first examples of multivalent pharmacological chaperones for the treatment of lysosomal storage disorders. ChemBioChem 2012;13:661–4
- Almant M, Mastouri A, Gallego-Yerga L, et al. Probing the nature of the cluster effect observed with synthetic multivalent galactosides and peanut agglutinin lectin. Chem Eur J 2013;19:729–38
- Risquez-Cuadro R, Garcia Fernandez JM, Nierengarten JF, Ortiz Mellet C. Fullerene-sp2-iminosugar balls as multimodal ligands for lectins and glycosidases: a mechanistic hypothesis for the inhibitory multivalent effect. Chem Eur J 2013;19:16791–803
- Brissonnet Y, Ortiz Mellet C, Morandat S, et al. Topological effects and binding modes operating with multivalent iminosugar-based glycoclusters and mannosidases. J Am Chem Soc 2013;135:18427–35
- Decroocq C, Joosten A, Sergent R, et al. The multivalent effect in glycosidase inhibition: probing the influence of valency, peripheral ligand structure, and topology with cyclodextrin-based iminosugar click clusters. ChemBioChem 2013;14:2038–49
- Bonduelle C, Huang J, Mena-Barragan T, et al. Iminosugar-based glycopolypeptides: glycosidase inhibition with bioinspired glycoprotein analogue micellar self-assemblies. Chem Commun 2014;50:3350–2
- Compain P, Bodlenner A. The multivalent effect in glycosidase inhibition: a new, rapidly emerging topic in glycoscience. ChemBioChem 2014;15:1239–51
- Durka M, Buffet K, Iehl J, et al. The inhibition of liposaccharide heptosyltransferase WaaC with multivalent glycosylated fullerenes: a new mode of glycosyltransferase inhibition. Chem Eur J 2012;18:641–51
- Abellán-Flos M, Tanc M, Supuran CT, Vincent SP. Exploring carbonic anhydrase inhibition with multimeric coumarins displayed on a fullerene scaffold. Org Biomol Chem 2015;13:7445–51
- Whittington DA, Waheed A, Ulmasov B, et al. Crystal structure of the dimeric extracellular domain of human carbonic anhydrase XII, a bitopic membrane protein overexpressed in certain cancer tumor cells. PNAS 2001;98:9545–50
- Alterio V, Hilvo M, Di Fiore A, et al. Crystal structure of the catalytic domain of the tumor-associated human carbonic anhydrase IX. PNAS 2009;106:16233–8
- Mack ET, Snyder PW, Perez-Castillejos R, et al. Dependence of avidity on linker length for a bivalent ligand-bivalent receptor model system. J Am Chem Soc 2012;134:333–45
- Touisni N, Kanfar N, Ulrich S, et al. Fluorescent silica nanoparticles with multivalent inhibitory effects towards carbonic anhydrases. Chem Eur J 2015;21:10306–9
- Khalifah RG. The carbon dioxide hydration activity of carbonic anhydrase. I. Stop-flow kinetic studies on the native human isoenzymes B and C. J Biol Chem 1971;246:2561–73
- Maresca A, Scozzafava A, Vullo D, Supuran CT. Dihalogenated sulfanilamides and benzolamides are effective inhibitors of the three β-class carbonic anhydrases from Mycobacterium tuberculosis. J Enzyme Inhib Med Chem 2013;28:384–7
- Maresca A, Vullo D, Scozzafava A, et al. Inhibition of the β-class carbonic anhydrases from Mycobacterium tuberculosis with carboxylic acids. J Enzyme Inhib Med Chem 2013;28:392–6
- Scozzafava A, Menabuoni L, Mincione F, et al. Carbonic anhydrase inhibitors: synthesis of sulfonamides incorporating dtpa tails and of their zinc complexes with powerful topical antiglaucoma properties. Bioorg Med Chem Lett 2001;11:575–82
- Vomasta D, Innocenti A, König B, Supuran CT. Carbonic anhydrase inhibitors: Two-prong versus mono-prong inhibitors of isoforms I, II, IX, and XII exemplified by photochromic cis-1,2-α-dithienylethene derivatives. Bioorg Med Chem Lett 2009;19:1283–6
- Veliev MG, Agaev NM, Shatirova MI, et al. Synthesis diacetylenic ethers with terminal acetylenic bonds and studying them as inhibitors of steel corrosion in hydrochloric acid. Russ J Appl Chem 2010;83:1957–61
- Yao F, Xu L, Fu G-D, Lin B. Sliding-graft interpenetrating polymer networks from simultaneous “click chemistry” and atom transfer radical polymerization. Macromolecules 2010;43:9761–70
- Rajaram H, Palanivelu MK, Arumugam TV, et al. “Click” assembly of glycoclusters and discovery of a trehalose analogue that retards Abeta40 aggregation and inhibits Abeta40-induced neurotoxicity. Bioorg Med Chem Lett 2014;24:4523–8
- Mustafa S, Hamid A, Naeem A, Sultana Q. Effect of pH, temperature and time on the stability of potassium ethyl xanthate. J Chem Soc Pak 2004;26:363–5