Abstract
A series of new 6-substituted-N-[3-{2-(substituted phenyl)-ethenyl} quinoxaline-2(1H)-ylidene]-1,3-benzothiazole-2-amine (4a–f) were designed and synthesized by condensing 2-amino-benzothiazole-6-sulfonic acid amide (1) with chalcones of quinoxaline-2-one (3a–f) in a hope to obtain promising and a new class of diuretic agents. Structures of all the newly synthesized compounds were characterized by spectral data and elemental analysis. The pharmacological studies in experimental rats indicates that compound 4c possesses excellent in vivo diuretic activity of 1.13 and appears to be a better diuretic agent than the reference drugs, acetazolamide (1.0) and urea (0.88). Insight of the binding mode of the synthesized compounds (ligand) into the binding sites of carbonic anhydrase enzyme (PDF code: 4KUV) was provided by docking studies, performed with the help of Maestro 9.0 docking software. Further pharmacokinetic and toxicological studies are needed to confirm the safety of compound 4c which emerged as a lead diuretic compound.
Introduction
Diuretics, occasionally referred to as water pills, increase the urine output. A diuretic primarily reduces the volume of extracellular fluid, enhances the urinary excretion of sodium chloride and secondarily increases the volume of urine excreted by kidneys. The drugs of this class are frequently prescribed in various heart and kidney related disorders. Diuretics are commonly indicated in the treatment of retention of fluid (edema), hypertension, congestive heart failure, liver cirrhosis, epilepsy, glaucoma and some kidney diseases. These drugs produce diuresis by acting on distinct sites on nephron, however, the primary mechanism involves inhibition of sodium ions re-absorption in the renal tubules of the kidneyCitation1. The various classes of therapeutic agents belonging to diuretics include xanthine derivatives, mercurial agents, sugars or sugar derivatives, thiazides, phenoxyacetic acids, aminomethylphenols, aromatic sulfonamides, steroids, pteridines and pyrazines. The most common therapeutic class of diuretic includes loop diuretics (high ceiling diuretic), thiazides, carbonic anhydrase (CA) inhibitors, potassium-sparing diuretics and osmotic diuretics. Recently, diuretics have been proposed to be safe and effective therapy in the management of hypertensionCitation2. In addition, outcome of many studies suggested them to be the first line therapy in management of hypertension in elderlyCitation3. Some diuretics are also used in glaucoma, hyperkalemia, bromide intoxication, anginal syndrome, epilepsy, migraine and premenstrual depression. One of the common adverse effects of diuretic therapy is “hyperuricemia” which is due to diminished extracellular and high degree of urate re-absorptionCitation4.
CA or carbonate dehydratase is a family of enzymes that occurs in various isoforms in kidney. CA derives its name due to removal of a water molecule from carbonic acid. The mammalian CA enzyme belongs to the alpha class and it catalyzes the rapid interconversion of carbon dioxide and water to bicarbonate and protons through a reversible reaction, which is otherwise slow in its absence. In animals, the CA is primarily responsible for maintaining acid base balance in blood and other body tissues, and to help transport carbon dioxide out of tissues. Acetazolamide (compound I) is a thiadiazole derivative which was introduced into the clinical practice after World War II as a safer non mercurial diuretic agent. It is a classical example of CA inhibitor (CAI) which upon its administration alkalinizes and increases the production of urine. Because of its alkalinizing property, it is sometime administered along with methotrexate to speed up its excretion in urine and thus prevents methotrexate induced nephrotoxicity. Though, it is clinically used to lower blood pressure, intraocular pressure, intracranial pressure, edema and epilepsy, however, its clinical usefulness is limited. The inhibition of CA by acetazolamide leads to accumulation of carbonic acid and reduction in blood pH accompanied with inhibition of re-absorption of bicarbonate, sodium and chloride ions from proximal tubule of kidney. This led to increased excretion of these ions along with water, leading to diuresis. It has been reported that sulfonamide derivatives induce diuresis by inhibiting CA II in cytosol as well as CA IV, XII and XIV isozymes bound to membrane. Few of the benzothiadiazine derivatives such as chlorothiazide (II), quinethazone, a quinazolinone derivative (III), metolazone (IV), chlorthalidone, a pthalimindine diuretic (V), indapamide (VI), furosemide (VII) and bumetanide (VIII) act as CAIs with varying degree of therapeutic diuretic efficiencies and different mechanisms (. This could be due to the presence of an unsubstituted primary sulfonamide moiety in their structures which is responsible for effective zinc binding in CAI activityCitation5,Citation6.
Diuretics constitute an important part of cardiovascular therapy especially in controlling blood pressure in heterogeneous population. These drugs are generally inexpensive, effective and are well tolerated by the patients of all age groups at low doses. However, the currently available diuretic therapy is associated with many unpleasant side effects which warrant the design and synthesis of a potent diuretic agent. Thereafter, we designed and synthesized some new benzothiazole sulfonamides containing quinoxaline ring and evaluated them for anticipated in vivo diuretic activity.
Materials and methods
Chemicals and instrumentation
Chemicals (reagents and solvents) of high purity were purchased from E. Merck Ltd (Mumbai, India), SD Fine Chemicals (Mumbai, India)E. Merck Ltd (Mumbai, India), SD Fine Chemicals (Mumbai, India) and used as such in all the experiments. One end open capillary tubes were used to measure the melting points on a liquid paraffin bath and are uncorrected. Silica gel G-coated TLC plates (Merck, Darmstadt, Germany) with the solvent systems (toluene: ethyl acetate: formic acid (5:4:1, v/v/v) or benzene: acetone (9:1, v/v)] were used to check the purity and completion of reactions. Iodine vapors or UV light were used to locate the spots on TLC chromatogram. Bruker spectrospin DPX-400 MHz (Rheinstetten, Germany) was used to record the 1H NMR and 13C NMR spectra of compounds in DMSO-d6/CDCl3 using tetramethylsilane (TMS) as an internal reference. The chemical shift (δ) values are reported in parts per million (ppm) while coupling constants (J) in Hz. The exchangeable protons of (OH and NH) were ascertained by the D2O shaking test. Mass and IR spectra were recorded on LCMS/MS (Perkin–Elmer and LABINDIA, Applied Biosystem) model no. API 3000 and FT/IR (Jasco, Japan), model no.410, respectively. The peaks in mass and IR spectra are presented as m/z and cm − 1. Elemental analysis (C, H and N) were performed on a Perkin–Elmer 240 analyzer (Waltham, MA) and were found within the acceptable range of ± 0.4% for each analyzed element.
Synthesis
Synthesis of 2-amino-benzothiazole-6-sulfonic acid amide (1)
Pre-cooled (5 °C) glacial acetic acid (150 ml), KSCN (0.06 mol) and p-amino sulfanilamide (0.06 mol) were added to form a mixtureCitation7. This mixture was kept in ice bath and stirred mechanically with gradual addition of bromine (0.02 mol) in 10 ml glacial acetic acid from a dropping funnel at such a rate that temperature does not rise >5 °C. A continuous stirring was done for an additional 3 h at temperature 0–10 °C in an ice bath. The separated hydrochloride salt was filtered, washed with acetic acid, dried and then dissolved in hot water. The aqueous solution was neutralized with aq. NH3 solution (25%) followed by filtration, and washing with water. The pure compound (1) was obtained by re-crystallization in benzene.
Radish brown; m.p. 230 °C; Yield 80%. Anal. Calcd. for C7H7N3O2S2: C, 36.60; H, 3.18; N, 18.43. Found: C, 36.63; H, 4.61; N, 18.91. FTIR (KBr) νmax (cm − 1): 3376 (N–Hstr), 3266 and 3244 (N–Hstr, –SO2NH2), 3015 (C–Hstr, Aromatic), 1694 (C=Nstr); 1H NMR (400 MHz, DMSO-d6, TMS): δ/ppm: 5.81(s, 2H, –NH2), 6.58 (d, 1H), 7.45 (d, 1H), 6.89 (s, 1H); 13C-NMR (DMSO-d6): δ/ppm: 154.63 (C=N), 141.74, 138.49, 129.13, 128.17, 127.53, 125.71(Ar-C); ESI-MS (m/z): 230 (M+).
Synthesis of 3-methylquinoxaline-2(1H)-one (2)
3-Methylquinoxaline-2(1H)-one was synthesized from pyruvic acid and o-phenylenediamine by the following procedureCitation8: equimolar quantities of pyruvic acid (0.01) and o-phenylenediamine (0.01) were refluxed in ethanol for 3 h. Upon completion of the reaction, the contents were cooled down to separate out the solid. The compound was filtered out and re-crystallized with ethanol.
Dark gray; m.p. 206 °C; Yield 80%. Anal. Calcd. for C9H8N2O: C, 67.49; H, 5.03; N, 17.49. Found: C, 67.51; H, 5.21; N, 17.45. FTIR (KBr) νmax (cm − 1) 3322 (N–Hstr), 3051 (C–Hstr, Aromatic), 2955 (C–Hstr, Aliphatic), 1694 (C=Ostr); 1H NMR (400 MHz, CDCl3, TMS): δ/ppm: 2.5 (s, 3H, CH3), 6.70–7.81 (m, 4H, Ar-H), 8.39 (s, 1H, NH); 13C-NMR (DMSO-d6): δ/ppm: 167.51(C=O), 157.63(C=N), 135.27, 133.21, 128.52, 124.45, 123.72, 121.17 (Ar-C), 23.42(CH3); ESI-MS (m/z): 168 (M+).
Synthesis of 3-[2-substituted phenylethenyl]quinoxalin-2(1H)-one (3a–f)
A mixture of compound 2 (0.01 mol) and different aromatic aldehydes (0.01 mol) were fused at 165 °C for 3 h in presence of piperidine (0.5 ml). The obtained product was re-crystallized using ethanol to obtain compound 3a–f.
General procedure for the synthesis of 6-substituted-N-[3-{2–(4-substitutedphenyl)-ethenyl} quinoxaline-2(1H)-ylidene] -1,3-benzothiazole-2-amine (4a–f)
6-Substituted-N-[3-{2–(4-substitutedphenyl)-ethenyl} quinoxaline-2(1H)-ylidene]-1,3 benzothia zole-2-amine (4a–f) were synthesized from compounds 1 and 3a–f. Compounds 1 and 3a–f were refluxed in an equimolar ratio in ethanol for 13–16 h. The reaction mixture was heated to obtain a concentrated mixture which upon cooling afforded a solid product which was filtered and purified by recrystallization in ethanol.
2-{3-[2-Phenylethenyl]-1H-quinoxalin-2-ylideneamino}-1,3 benzothiazole-6-sulfonic acid amide (4a)
Brown solid; m.p. 230–231 °C; Yield 62%. Anal. Calcd. for C23H17N5O2S2: C, 60.11; H, 3.73; N, 15.24. Found: C, 60.15; H, 3.75; N, 16.45. FTIR (KBr) νmax (cm − 1): 3330 (N–Hstr), 3266 and 3244 (N–Hstr, –SO2NH2), 1629 (C=Nstr), 1338 (C–Nstr), 711 (C–Hdef, monosubst. Bz), 628 (C–S–C str.); 1H NMR (400 MHz, CDCl3, TMS): δ/ppm: 6.9 (d,1H, =CH–C=), 7.2 (d, 1H, =CH-Ar), 6.5–8.79 (m, 12H, Ar-H), 8.8 (s, 1H, NH); 13C-NMR (DMSO-d6): δ/ppm: 164.15, 158.27, 154.63 (C=N), 140.32, 139.73 (CH=CH), 135.91, 134.28, 132.64, 131.46, 130.83, 129.25, 129.13, 128.37, 126.41, 124.16, 123.14, 122.92, 120.34, 118.72, 116.85, 115.70, 114.79, 110.51 (Ar-C); ESI-MS (m/z): 459 (M+).
2-{3-[2–(4-Chlorophenyl)-ethenyl]-1H-quinoxalin-2-ylideneamino}-benzothiazole-6-sulfonic acid amide (4b)
Blackish brown; m.p. 235–237 °C; Yield 67%. Anal. Calcd. for C23H16ClN5O2S2: C, 55.92; H, 3.26; N, 14.18. Found: C, 55.95; H, 3.75; N, 14.35. FTIR (KBr) νmax (cm − 1): 3376 (N–Hstr), 3266 & 3244 (N–Hstr, –SO2NH2), 1629 (C=Nstr), 1338 (C–Nstr), 813 (C–Hdef, p-disubst. Bz), 748 (Ar C–Clstr), 628 (C–S–Cstr); 1H NMR (400 MHz, CDCl3, TMS): δ/ppm: 6.9 (d, 1H, =CH–C=), 7.2 (d, 1H, =CH-Ar), 6.5–8.9 (m, 11H, Ar-H), 8.8 (s, 1H, NH); 13C-NMR (DMSO-d6): δ/ppm: 165.76, 156.23, 155.74 (C=N), 141.56, 138.27 (CH=CH), 134.87, 133.21, 132.17, 131.65, 131.17, 130.59, 129.38, 128.49, 125.53, 124.63, 123.01, 121.36, 120.45, 119.31, 116.82, 113.69, 112.56, 110.64 (Ar-C); ESI-MS (m/z): 493 (M+).
2-{3-[2–(4-Methoxyphenyl)-ethenyl]-1H-quinoxalin-2-ylideneamino}-1,3 benzothiazole-6-sulfonic acid amide (4c)
Greenish dark gray; m.p. 234–237 °C; Yield 60%. Anal. Calcd. for C24H19N5O3S2: C, 58.88; H, 3.91; N, 14.31. Found: C, 58.91; H, 3.87; N, 14.56. FTIR (KBr) νmax (cm − 1): 3376 (N–Hstr), 3266 and 3244 (N–Hstr, –SO2NH2), 3058 (C–Hstr, Aromatic), 2853 (C–Hstr, –O–CH3), 1628 (C=Nstr), 1097 (C–Ostr, Ar–O–CH3), 813 (C–Hdef, p-disubst. Bz), 628 (C–S–Cstr); 1H NMR (400 MHz, CDCl3, TMS): δ/ppm: 3.74 (s, 3H, –OCH3), 6.26 (d, =CH–C=), 6.93 (d, 1H, =CH-Ar), 7.13–8.42 (m, 11H, Ar-H), 8.98 (s, 1H, NH); 13C-NMR (DMSO-d6): δ/ppm: 170.53, 166.45, 157.28 (C=N), 138.47, 136.49 (CH=CH), 136.78, 135.21, 134.92, 131.57, 129.03, 128.56, 127.63, 125.53, 124.74, 123.01, 122.87, 121.36, 120.45, 119.31, 116.82, 113.69, 112.56, 110.64 (Ar-C), 30.75 (CH3, OCH3); ESI-MS (m/z): 489 (M+).
2-{3-[2–(3,4-Dimethoxyphenyl)-ethenyl]-1H-quinoxalin-2-ylideneamino}-1,3 benzothiazole-6-sulfonic acid amide (4d)
Light Blue; m.p. 210–211 °C; Yield 82%. Anal. Calcd. for C25H21N5O4S2: C, 57.79; H, 4.07; N, 13.48. Found: C, 58.15; H, 4.13; N, 13.45. FTIR (KBr) νmax (cm − 1): 3330 (N–Hstr), 3266 and 3244 (N–Hstr, –SO2NH2), 3058 (C–Hstr, Aromatic), 2850 (C–Hstr, –O–CH3), 1632 (C=Nstr), 1097 (C–Ostr, Ar–O–CH3), 628 (C–S–Cstr); 1H NMR (400 MHz, CDCl3, TMS): δ/ppm: 3.73 (s, 6H, –OCH3), 7.27 (d,1H, =CH–C=),7.67 (d, 1H, =CH-Ar), 7.13–8.42 (m, 10H, Ar-H), 8.88 (s, 1H, NH); 13C-NMR (DMSO-d6): δ/ppm: 169.34, 165.21, 155.46 (C=N), 138.56, 133.27 (CH=CH), 136.71, 136.13, 135.05, 132.45, 130.29, 127.15, 126.89, 125.32, 124.63, 123.69, 121.81, 121.17, 120.45, 118.58, 117.37, 115.61, 111.89, 111.13 (Ar-C), 34.19, 34.15 (CH3, OCH3); ESI-MS (m/z): 519 (M+).
2-{3-[2–(4-Nitrophenyl)-ethenyl]-1H-quinoxalin-2-ylideneamino}-1,3 benzothiazole-6-sulfonic acid amide (4e)
Yellowish brown; m.p. 238–240 °C; Yield 75%. Anal. Calcd. for C23H16N6O4S2: C, 54.75; H, 3.20; N, 16.66. Found: C, 54.81; H, 3.27; N, 16.71. FTIR (KBr) νmax (cm − 1): 3300 (N–Hstr), 3360 and 3270 (N–Hstr, –SO2NH2), 3058 (C–Hstr, Aromatic), 1629 (C=Nstr), 1570 (N=Ostr., Ar-NO2), 1338 (C–Nstr), 857 (C–Hdef, p-disubst. Bz), 628 (C-S-Cstr); 1H NMR (400 MHz, DMSO-d6, TMS): δ/ppm: 6.9 (d, 1H, =CH-C=),7.2 (d, 1H, =CH-Ar), 7.13–8.42 (m, 11H, Ar-H), 8.8 (s, 1H, NH); 13C-NMR (DMSO-d6): δ/ppm: 170.85, 167.34, 156.89 (C=N), 139.72, 137.19 (CH=CH), 138.56, 137.45, 134.19, 133.59, 131.78, 129.36, 127.81, 126.67, 125.39, 120.93, 120.15, 119.27, 118.34, 117.13, 116.58, 115.25, 113.42, 112.74 (Ar-C); ESI-MS (m/z): 504 (M+).
2-{3-[2–(4-Dimethylaminophenyl)-ethenyl]-1H-quinoxalin-2-ylideneamino}-1,3 benzothiazole -6-sulfonic acid amide (4f)
Brownish gray; m.p. 228–230 °C; Yield 72%. Anal. Calcd. for C25H22N6O2S2: C, 59.74; H, 4.41; N, 16.72. Found: C, 59.78; H, 3.91; N, 16.75. FTIR (KBr) νmax (cm − 1): 3395 (N–Hstr), 3366 and 3244 (N–Hstr, –SO2NH2), 3150 (C–Hstr, N–Me2), 3058 (C–Hstr, Aromatic), 1629 (C=Nstr), 1338 (C–Nstr), 837 (C–Hdef, p-disubst. Bz), 687 (C–S–Cstr); 1H NMR (400 MHz, CDCl3, TMS): δ/ppm: 3.07 (s, 6H, NMe2), 6.73 (d, 1H, =CH-Ar), 7.41 (d,1H, =CH–C=), 8.7 (s, 1H, NH), 7.44–8.64 (m, 11H, Ar-H); 13C-NMR (DMSO-d6): δ/ppm: 169.85, 165.71, 155.35 (C=N), 138.83, 138.13 (CH=CH), 139.01, 137.15, 135.26, 132.75, 132.13, 130.72, 128.54, 126.35, 125.71, 124.36, 123.17, 120.68, 119.92, 115.23, 115.12, 112.63, 111.28, 110.81 (Ar-C), 27.81, 27.76 (CH3); ESI-MS (m/z): 502 (M+).
Pharmacology
Diuretic activity
The diuretic activity was performed by adopting the Lipschitz methodCitation9. An approval of experimental protocol was obtained from the Institutional Animal Ethical Committee (173 CPCSEA 28 January 2010 – Proposal No. 647), Central Animal House Facility, Jamia Hamdard University, New Delhi, India. Fifty-four healthy adult Wistar albino rats (180–200 g) were selected and divided in to nine groups having six animals in each (n=6) group. The rats were acclimatized in standard environmental conditions for one week. The standard diet and water ad libitum were given to all rats during the protocol of the study which was withdrawn from all animals 15 h prior to the experiment. The rats were kept at room temperature 25 ± 5 °C throughout the experiment. The total excreted urine (from 0 to 5 h) of rats kept in metabolic cage was collected to measure the diuretic activity of the compounds. In the beginning of each experiment, the animal’s cages, funnel and measuring cylinder were pre-coated with liquid paraffin to facilitate the better collection with a minimum loss of urine. Each animal was placed in a separate metabolic cage which was fitted with a wire mesh at the bottom and a funnel to collect the urine. Stainless steel sieves were placed in the funnel to retain and separate feces from urine and allow the urine alone to pass through the sieves. The urine was collected for a total duration of 5 h. The test compounds were administered orally (p.o.) at a dose of 45 mg/kg body weight in 5 ml of 0.9% NaCl solution. Control group received 5 ml of 0.9% NaCl solution only. The activity of test compounds was compared with standard diuretic acetazolamide (45 mg/kg body weight in 5 ml of 0.9% NaCl solution). Diuretic activity was calculated by using following formulaCitation9–13.
Effect on electrolyte concentration
The concentration of Na+ and K+ ions was measured by calibration method using flame photometry. The primary standard solutions of sodium and potassium of 1000 ppm concentration by weight was prepared for flame photometric analysis.
These solutions were diluted before use to the proper concentrations in the following manner. The collected urine (1–3 ml) was diluted with distilled water up to 100 ml and the resultant diluted solution was used directly for the measurement of both Na+ and K+ ions. The calibration charts used for these determinations depend entirely concentration of urine. Trial runs were made in order to establish whether the scale is to be set at 100 ppm or higherCitation13.
Molecular docking studies
Molecular docking studies help in understanding and predicting the enzyme–ligand interactions in vivo. Docking studies of the newly synthesized compounds against CA enzyme were carried out to rationalize the results obtained in this study. The newly synthesized compounds were evaluated through molecular modeling and docking studies. The molecular docking studies of all compounds were performed on 3D structure of CA enzyme with the help of Maestro 9.0 program (Schrodinger Inc., NY) by 64 bit operating system under window 7 with an HCl computer [Intel (R) Core (TM) i5–2400 CPU at 3.10 GHz, 8 GB memory].
The 3D structure of the enzyme for this study was downloaded from the Protein Data Bank (code; PDB ID: 4KUV). This enzyme is known to have 96% similarity with the human cell enzyme and it is also reported that all active site residues in the vicinity of cofactor of this enzyme have exactly the same counterpartsCitation14. The enzyme structure was refined and checked for any missing atoms, bonds and contacts. All residues excluding ligand molecules and water molecules were deleted manually. The ligand molecules were build using the builder molecule and then their energy was minimized. The grid box tools were used to generate an active site. The conformer having the lowest energy was chosen and its energy was minimized. The best binding modes of compound 4c in the active site of 4KUV is shown in and .
Figure 2. Binding mode of compound 4c into the binding sites of carbonic anhydrase enzyme (PDB code: 4KUV) showing hydrogen bond (dotted lines) with ASN62 and pi–pi interaction with HIE64 and HIS94.
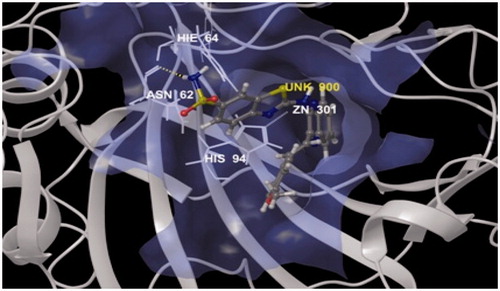
Figure 3. Lig plot of compound 4c showing interaction into the binding sites of carbonic anhydrase enzyme (PDB code: 4KUV), hydrogen bond (pink dotted line on top left) with ASN62 (2.12 Å) and pi–pi interaction (green solid line shown as three arrows on right) with HIE64 (4.30 Å) and HIS94 (5.43 Å and 4.78).
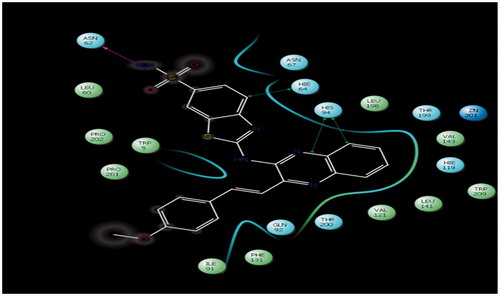
Results and discussion
The synthesis of the quinoxaline derivatized benzothiazole sulfonamides were started from chalcones (3a–f) and 6-sulfonylamino-1,3-benzothiazole-2-amine (1). 6-sulfonylamino-1,3-benzothiazole-2-amine was synthesized by the reaction of KSCN (0.06 mol) and p-sulfanilamide (0.06 mol) in glacial acetic acid (150 ml) pre-cooled to 5 °C using bromine as a catalyst. The series (4a–f) was synthesized in a single step from different chalcones (3a–f) and 6-sulfonylamino-1,3-benzothiazole-2-amine (1) by refluxing them in absolute alcohol for 12–13 h. The 3-[2-(substituted phenyl)-ethenyl] quinoxalin-2(1H)-one (3a–f) were prepared from 3-methylquinoxaline-2(1H)-one (2) and substituted aryl amines. 3-methylquinoxaline-2(1H)-one was synthesized by refluxing o-phenylenediamine with pyruvic acid in the presence of ethanol as outlined in synthetic scheme () and space fill model of compounds (4a–f) in .
In the IR spectral analysis, compounds, showed absorption bands in the range of 3390–3318 cm − 1 due to trans-ene C–H stretching bonds, 2600–2550 cm − 1 due to S–H, 1600–1550 cm − 1 due to aromatic C–H groups, 1540–1500 cm − 1 due to imine C=N stretching and 800–720 cm − 1 due to aromatic C–H deformation. The 1H NMR spectra further confirmed the chemical structure and the peaks found in the range of 6.5–8.00 ppm were due to presence of different aromatic protons, signals at 8–10 ppm was characteristic of imino proton and the peaks in the region of 10–13.1 ppm was ascribed to sulfonamide-proton. The peaks for the methylene protons were observed in the range of 3.8–4.9 ppm. Two doublets in the range of 7.5–8 ppm having coupling constant of 15.6 Hz were very characteristic of a trans-proton of the ethylene group.
The results of docking studies of compound 4c into the active sites of enzyme showed hydrogen bond, π interaction and hydrophobic molecular interactions which are believed to be responsible for the observed affinity of the tested molecule. Interestingly, compound 4c does not have a zwitter ion, however, it attaches to the ASN62 residue of enzyme by making hydrogen bonds through its amino and carbonyl group. ASN62 residue is said to be the same residue at which the natural inhibitor is known to bindCitation14.
A hydrogen bond interaction was observed between the active hydrogen of amino group (–NH2) of thiazole ring of compound 4c which acts as a hydrogen bond donor with the carboxyl group (C=O) of the side chain residue of ASN62 (2.12 Å) that acts as hydrogen bond acceptor is illustrated in . The sulfonamide group of compound 4c appears to play an important role in formation of strong hydrogen bond because the lone pair electrons on nitrogen atom of the amide group delocalized into the carbonyl group (C=O) of compound. Pi–Pi interaction of the compound with the target also seems to play an important role in inhibitory activity, as phenyl ring of benzothiazole nucleus appears to be properly oriented toward the more lipophilic area of HIE64 (4.30 Å) and formed CH–π interaction. Another Pi–Pi interaction as quinoxaline ring properly oriented toward HIS94 (5.43 Å and 4.78) and formed CH–π interaction is illustrated in Lig plot (. The other hydrophobic interactions formed between the phenyl ring and aliphatic portion with the remains of amino acids residues are shown in and . Compound 4c showed a glide score value of −5.94 which clearly indicates that the synthesized molecule interacts in a befitting manner with the enzyme.
Heterocyclic sulfonamides act by inhibiting enzyme CAs. It has been known that Zn2+ metal ions are essential for catalysis in all α-CA enzymes catalyzed reactions investigated till date. The ion is shown to be situated at the base of a 15-Å deep active-site cleft by X-ray crystallographic data and is synchronized by three histidine residues viz His94, His96 and His119 and a hydroxide ion/water molecule. It was also evidenced that the cluster of histidine residues comprising of His64, His4, His3, His17, His15 and His10 were involved in the proton-shuttling processes between the active site and the environment. Further, it was also observed that amino-acid residues 92 and 131 are found to be involved in the binding of quite a few sulfonamide/sulfamate inhibitors. The zinc-bound water is also involved in formation of hydrogen bond interactions with the –OH group of Thr199 amino acid, which in turn form a bridge to the carboxylate moiety of Glu106. These hydrogen ion interactions proved to be quite helpful in enhancing the nucleophilicity of the zinc-bound water molecule, and to orient the substrate (CO2) in a favorable position for a nucleophilic attack. The enzyme with hydroxide bound to Zn2+ (a) is an active form of the enzyme which is a basic in nature. This strong nucleophile attacks carbon dioxide (CO2) molecule, bound in a hydrophobic pocket in its neighborhood. This elusive substrate-binding site contains residues Val121, Val143 and Leu198 in the case of the human isozyme CA (II) (b) which leads to the formation of bicarbonate coordinated to Zn2+ (c). The water molecules then displaces bicarbonate ion which is liberated into the solution, forming a catalytically inactive acid form of the enzyme, with water coordinated to Zn2+ (d). To regenerate the active basic form, i.e. hydroxide bound to Zn2+ (a), a proton transfer reaction from the active site to the environment may be assisted either by active-site residues (such as His64, the proton shuttle in isozymes I, II, IV, VI, VII, IX and XII–XIV among others) or by the buffers present in the medium (. The schematical representation of the process may be shown by reactions 2 and 3. In reaction 3, the proton transfer regenerating the zinc-hydroxide species of the enzyme is a rate-limiting step in the catalysis ()Citation15–18.
Figure 7. Proposed mechanism of inhabitation of carbonic anhydrase: by binding to the ZN2+ ion of the enzyme by substituting non-protein zinc ligand, t generate a tetrahedral adduct.
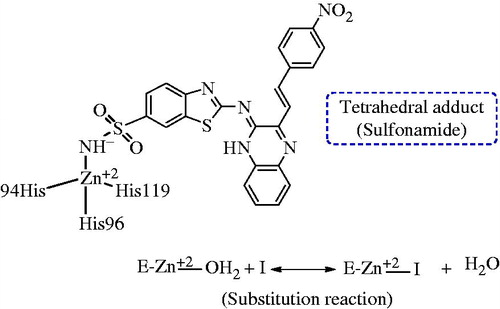
The rats were kept in metabolic cages for the measurement of the diuretic activity of the synthesized compounds. The diuretic activity was measured by collecting the total excreted urine of rats after administration of the test compounds and was compared with standard and control groups of the rats. The diuretic activity was evaluated as per the reported methodCitation14. Compounds 1 and 4a–f were evaluated for diuretic activity using acetazolamide as reference drug.
The results of the in vivo diuretic activity of the newly synthesized compounds are presented in . Compounds 1, 4a, 4b, 4c and 4e demonstrated excellent cumulative urine output, among these compound, the urinary output of 2-{3-[2–(4-methoxyphenyl)-ethenyl]-1H-quinoxalin-2-ylideneamino}-1,3 benzothiazole-6-sulfonic acid amide (4c) was highly significant, i.e. 5.30 ± 0.413 (p < 0.01), which is increased by >200% as compared to the control group. Urinary excretion of compound 1 was found to be in between urea (136.6%) and standard acetazolamide (155.17%). Diuretic action of compound 4c was observed to be 2.4 in comparison with urea (2.11) and acetazolamide (1.86). Diuretic activity of compound 4c was found to be 1.13 times more potent than acetazolamide, while 1, 4a, 4b and 4e were calculated as 1.07, 0.75, 0.75 and 0.78, respectively (). The Lipschitz value (the ratio T/U, in which T is response of the test compound, and U, that of urea treatment, indices of 1.0 and higher are regarded as a positive effect in terms of diuretic activity) shows that 4c is 1.28 times as potent as urea, as far as urinary output is concerned, and compound 1 is also more potent (1.21 times) than urea. Compound 1 showed a significant increase in Na+ excretion, 2.41 ± 0.102 (p < 0.01) which was slightly higher than the standards, urea (1.72 ± 0.073) and acetazolamide (1.80 ± 0.041) (). It is evident from the obtained results that compound 1 possess significant kaliuretic property (p < 0.01), 1.08 ± 0.036, however, 4e emerged as a stronger kaliuretic (1.75) with regards to the Na+/K+ ratio ().
Table 1. In vivo diuretic activity of synthesized compounds (4a–f) using a rat animal model.
Table 2. Effect of synthesized compounds (4a–f) on electrolyte concentration in excreted urine of rats.
The structure activity relationship study illustrated that the substitution with halogens at 6th position of benzothiazole ring decreases the activity, but shows a good promising effect as compared to other standard diuretics. From the analysis of results, compound 4c (2-{3-[2–(4-methoxyphenyl)-ethenyl]-1H-quinoxalin-2-ylideneamino}-benzothiazole-6-sulfonic acid amide) appeared to be the most promising and the lead compound as diuretic among all the newly synthesized aromatic sulfonamide compounds.
Conclusions
Six differently substituted quinoxaline derivatives of benzothiazole (4a–f) were synthesized with an aim to obtain diuretic compounds with a better spectrum. These compounds were evaluated for their diuretic activity in experimental rat animal model. The data obtained from pharmacological studies indicates that compound 4c showed excellent diuretic activity of 1.13 in comparison to 1.0 for acetazolamide and 0.88 for Urea. Compound 1 also showed very good diuretic activity (0.81). Additionally, molecular docking studies were also carried out with the help of Maestro 9.0 program (Schrodinger Inc) to ascertain the binding patterns of compound 4c into the binding sites of CA enzyme (PDB code: 4KUV). In light of the current results, the compound 4c may be worth to explore and is a subject of further detailed studies in search of prospective new diuretics.
Declaration of interest
The authors report no declarations of interest.
References
- Carta F, Supuran CT. Diuretics with carbonic anhydrase inhibitory action: a patent and literature review (2005–2013). Expert Opin Ther Pat 2013;23:681–91
- Ernst ME, Moser M. Use of diuretics in patients with hypertension. N Engl J Med 2009;361:2153–64
- Bakht MA, Shahar Yar M, Siddiqui AA, et al. Molecular properties prediction, synthesis, and diuretic activity of phenoxy acetic acid bearing pyrazolines. Med Chem Res 2013;22:916–26
- Abraham DJ. Burger’s medicinal chemistry and drug discovery. Vol. 3. 6th ed. New Jersey: Wiley Interscience Publication; 2003:56–62
- Husain A, Madhesia D. Heterocyclic compounds as carbonic anhydrase inhibitor. J Enzyme Inhib Med Chem 2012;27:773–83
- Supuran CT. Carbonic anhydrases: novel therapeutic applications for inhibitors and activators. Nat Rev Drug Discov 2008;7:168–81
- Lin AJ, Kasina S. Synthesis of 3-substituted 7-(3,3-dimethyl-1-triazeno)-10-methyl phenothiazines as potential antitumor agents. J Heterocyclic Chem 1981;18:759–61
- Sain DK, Thadhaney B, Joshi A, et al. Synthesis, characterization and biological evaluation of some heterocyclic compounds containing ethoxyphthalimide moiety via key intermediate 6-chloro 1,3 benzothiazole 2-amine. Indian J Chem 2010;49B:818–25
- Lipschitz WL, Hadidian Z, Kerpcsar A. Bioassay of diuretics. J Pharmacol Exp Ther 1943;79:97–110
- Majeed J, Shaharyar M. Synthesis and in-vivo diuretic activity of some novel pyrimidine derivatives. J Enzyme Inhib Med Chem 2011;26:819–26
- Meera R, Devi P, Muthumani P, et al. Evaluation of diuretic activity from Tylophora indica leaves extracts. J Pharm Sci Res 2009;1:112–16
- Shahar Yar M, Ansari ZH. Synthesis and in vivo diuretic activity of biphenyl benzothiazole-2-carboxamide derivatives. Acta Poloniae Pharmaceutica Drug Res 2009;66:387–92
- Vogel HG. Drug discovery and evaluation. 2nd ed. Berlin, Heidelberg, New York: Springer Verlag; 2002:323–5
- Bozdag M, Ferraroni M, Nuti E, et al. Combining the tail and the ring approaches for obtaining potent and isoform-selective carbonic anhydrase inhibitors: solution and X-ray crystallographic studies. Bioorg Med Chem 2014;22:334–40
- Supuran CT, Scozzafava A, Casini A. Carbonic anhydrase inhibitors. Med Res Rev 2003;23:146–89
- Kohler K, Hillebrecht A, Schulze Wischeler J, et al. Saccharin inhibits carbonic anhydrases: possible explanation for its unpleasant metallic after taste. Angew Chem Int Ed Engl 2007;46:7697–9
- Supuran CT. Carbonic anhydrase inhibitors. Bioorg Med Chem Lett 2010;20:3467–74
- Nishimori I, Minakuchi T, Onishi S, et al. Carbonic anhydrase inhibitors: cloning, characterization, and inhibition studies of the cytosolic isozyme III with sulfonamides. Bioorg Med Chem 2007;15:7229–36