Abstract
A 16-kDa trypsin inhibitor was isolated from an edible legume using various chromatographic procedures. The protein was unadsorbed on Affi-gel blue gel but adsorbed on DEAE-Sepharose and Mono Q following which media the protein was subsequently subjected to gel filtration on Superdex 75 and a final 21-fold purification was achieved. This trypsin inhibitor showed remarkable pH and thermal stability. Its inhibitory activity was impaired in the presence of 1 mM dithiothreitol. The anti-proliferative and anti-mobility activities of this trypsin inhibitor and a hemagglutinin isolated from the same legume were tested on nasopharyngeal carcinoma cells. These two defense proteins demonstrated discrepant anti-proliferative efficacies that the hemagglutinin could greatly suppress the proliferation of nasopharyngeal carcinoma cells, while the trypsin inhibitor revealed a minor effect. However, these two proteins could both attenuate the mobility of nasopharyngeal carcinoma cells. The present study revealed the potential of applying plant defense proteins in cancer treatment.
Introduction
Thousands of years’ evolution has granted all organisms a self-defending system to survive from aggressive invasions. Mammals like human beings are protected by a strong immune system from the frequent attacks by ubiquitous pathogens. Though the defending mechanism of plants is not as distinct as that in mammals, they definitely have a defense system against pathogens and plants under threat can react with alterations in morphology, structural features and production of chemicals to augment resistance against the threatCitation1. The expression of defense proteins, like lectins/hemagglutininsCitation2,Citation3, anti-fungal proteinsCitation4, ribosome inactivating proteinsCitation5, protease inhibitorsCitation6, etc., is one of the common strategies adopted by plants. As one of the defense proteins in plants, protease inhibitors have been isolated from a variety of plants, like Moringa oleifera flowersCitation7, buckwheatCitation8, legumes and Lawsonia inermisCitation9. They could be classified into aspartic protease inhibitors, cysteine protease inhibitors, metalloprotease inhibitors, trypsin inhibitors, etc., by the kind of protease they inhibit. Trypsin inhibitors are the kind of inhibitors that could reduce the availability of trypsin which is a serine protease that cleaves proteins specifically at the C-terminus of lysine or arginine residueCitation10. Protease inhibitors are suspected to protect plants from herbivorous insects by attenuating the activity of digestive proteasesCitation6. Vulnerable organs of plants, like seeds, usually possess abundant protease inhibitors to prevent tissues from undesirable degradationCitation11. For instance, the percentage of trypsin inhibitors in soybean proteins is approximately 11.3%Citation12. Consumption of legume protease inhibitors has a major impact on protein digestion by suppressing the activity of pancreatic serine proteases. Lectins/hemagglutinins also play vital self-defending roles by negatively affecting the growth of insects or hindering oviposition behaviors of insectsCitation13.
The expression of lectins/hemagglutininsCitation14 and protease inhibitorsCitation11 in plants is sensitive to stress factors and environmental variations, therefore they can be regulated and induced in response to attack from insects and other pathogens. For example, tobacco leaves normally express very low levels of lectin, however, when treated with jasmonates or insect herbivory, expression of the lectin is up-regulatedCitation15,Citation16.
The content of lectins/hemagglutinins and protease inhibitors is more abundant in legumes compared with other plants. Many different lectins/hemagglutinins and protease inhibitors have been successfully isolated from legumes and some of them are even commercialized. For example, the purified trypsin inhibitor from soybean and concanavalin A from Jack bean could both be purchased from Sigma-Aldrich (St. Louis, MO). However, defense proteins isolated from different species or even the same species may possess distinct biological functions and have different applications.
In the present study, we purified a trypsin inhibitor from the Northeast China black beans from which no trypsin inhibitor has been isolated before. Its pH and thermal stabilities as well as its stability in the presence of DTT were characterized. The potency of this trypsin inhibitor and another defense protein isolated from the same legume in the treatment of nasopharyngeal carcinoma cells is also evaluated.
Materials and methods
The Northeast China black beans, a cultivar of Phaseolus vulgaris, were purchased from a local vender and authenticated by Professor Shiuying HU, Honorary Professor of Chinese Medicine, The Chinese University of Hong Kong Affi-Gel Blue gel, Mono Q 5/50 GL, Superdex 75 10/300 GL columns and Akta Purifier were bought from GE Healthcare, Buckinghamshire, UK. Human nasopharyngeal cancer cell lines CNE-1 and HONE-1 were purchased from the Sun Yat-sen University of Medicinal Sciences, Guangzhou, China. The CNE-1 and HONE-1 cells were cultured in DMEM medium containing 10% FBS, 100 U/ml penicillin and 100 μg/ml streptomycin (Gibco, Invitrogen Ltd, Paisley, UK). All cell lines were maintained at 37 °C in a humidified incubator under an atmosphere of 95% air and 5% CO2.
Protein purification
The slurry of dried beans was prepared by homogenization in a blender. After centrifugation (14 000 rpm for 30 min at 4 °C) and filtration through filter paper, the crude extract was first applied to an Affi-gel blue gel column (5 cm × 12 cm; 240 ml bed volume) which had been pre-equilibrated with 20 mM Tris–HCl buffer (pH 7.6). The column was then eluted, first with NaCl-free buffer to obtain unbound proteins and then 20 mM Tris–Hcl buffer with 1 M NaCl to desorb the bound proteins. The unbound fraction, which carried the trypsin inhibitory activity, was subsequently loaded on a DEAE Sepharose column (5 cm ×17 cm; 340 ml bed volume) in 20 mM NH4HCO3 (pH 9.6) buffer. The adsorbed fraction was successively eluted with 20 mM NH4HCO3 buffer containing 0.2 M NaCl and 1 M NaCl. The bound fraction (eluted by 0.2 M NaCl) from DEAE-Sepharose was run on a Mono Q column (5 mm × 50 mm; 1 ml bed volume) on an FPLC system in 20 mM NH4HCO3 (pH 9.6) buffer. The bound proteins were eluted with three successive linear gradients of NaCl (0–0.2 M in 5 ml, 0.2–0.25 M in 15 ml, 0.25–1 M in 5 ml) in 20 mM NH4HCO3 (pH 9.6) buffer. The active fraction was then loaded on a Superdex 75 column (10 mm × 300 mm; 24 ml bed volume). The isolated protein from each step was dialyzed against water, lyophilized, dissolved in the corresponding buffer before chromatography on the next column. The hemagglutinin (NCBBH) was purified from the Northeast China black beansCitation17 by following the methods that have previously been reported by us.
Molecular weight determination
The molecular weight of the purified protein was determined by running sodium dodecyl sufate–polyacrylamide gel electrophoresis (SDS–PAGE). A 15% separating gel with a 5% stacking gel was used. At the end of electrophoresis, the gel was stained with Coomassie brilliant blue with shaking overnight and subsequently destained with 10% acetic acid until the background was clear. The molecular weight of the protein was estimated with reference to the molecular weight marker.
Assay of trypsin inhibitory activity
A 60-μl aliquot of the test sample (120 μg) was mixed with 30 μl of 0.02 M Tris–HCl buffer (pH 7.6) and 40 μl 1 mg/ml tryspin, while the negative control was prepared by mixing 90 μl 0.02 M Tris–HCl buffer with 40 μl 1 mg/ml tryspin. After incubating the mixture at 37 °C for 5 min, 80 μl 1 mg/ml casein was added and incubated for another 20 min at 37 °C. The reaction was terminated by adding 0.5 ml 5% trichloroacetic acid (TCA). The solution was then centrifuged at 14 000 rpm at 4 °C for 15 min and the OD (280 nm) of the supernatant was determined. The specific activity of trypsin inhibitor was computed by applying the following formula:
Thermo and pH stability
To determine the thermo-stability of the inhibitor, purified protein was dissolved in 0.02 M Tris–HCl buffer (pH 7.6) and incubated at different temperatures for 30 min. The remaining trypsin inhibitory activity of the mixture was assessed after it had cooled down to room temperature. To determine pH stability, an aqueous solution of the protein was adjusted with NaOH and HCl to different pH values (0–13). After incubation at room temperature for 30 min, the mixture was neutralized to pH 7 before assessing its trypsin inhibitory activity.
Effect of dithiothreitol on trypsin inhibitory activity
The purified trypsin inhibitor (4 μM) was pretreated with dithiothreitol (DTT) at the final concentrations of 0, 0.1, 1 and 10 mM at 37 °C for 30 min. Iodoacetamide was supplied at twice the amount of thiol functions at each DTT concentration to terminate the reaction. The residual trypsin inhibitory activity was then tested as described above.
Assay of anti-proliferative activity
The anti-proliferative activity of purified trypsin inhibitor and hemagglutinin on HONE-1 and CNE-1 cells was tested by employing the 3-(4,5-dimethylthiazol-2-yl)-2,5-diphenyltetrazolium bromide (MTT) method. Briefly, approximately 5 × 103 cells in 100 μl DMEM medium were seeded in each well of a 96-well plate and incubated overnight in a cell incubator. Fresh medium containing different concentrations of the purified proteins was supplied after the old medium was discarded. The cells were incubated for another 48 h in a humidified atmosphere of 5% CO2 at 37 °C. The medium was then replaced by 25 μl MTT (5 mg/ml in PBS) and incubated for 2 h in darkness at 37 °C. Dimethyl sulfoxide (150 μl) was added into each well to dissolve the formazan. The absorbance was then recorded with a microplate reader at 570 nm as the measurement wavelength and 630 nm as the reference wavelength. The percentage of inhibition was calculated by using the formula: percentage of inhibition = (ODC−ODT)/(ODC × 0.01); ODC = ODC570– ODC630; ODT = ODT570−ODT630. ODC570/ODT570: the OD reading of control/treated wells at the wavelength of 570 nm; ODC630/ODS630: the OD reading of control/treated wells at the wavelength of 630 nm.
Monolayer wound-healing assays
HONE-1 and CNE-1 cells were seeded on the 24-well plates in DMEM medium. A wound was created by scraping the cell layers with a 10-μl micropipette tip when a confluent monolayer has formed. The cells were washed with PBS twice, supplied with FBS-free DMEM containing purified trypsin inhibitor or hemagglutinin and incubated in a humidified atmosphere of 5% CO2 at 37 °C. The healing condition at 24 h was monitored by observing under a microscope. The motility of cells was calculated by following the method previous describedCitation18: M= [(D0−Dt)/D0] × 100, where D0 is the average distance of the initial wound; Dt is the average distance of the wound at different time pointsCitation18.
Results
Protein purification
The crude extract was separated into two fractions, B1 and B2, on Affi-gel blue gel as shown in . The unbound fraction B1, the only fraction with trypsin inhibitory activity, was collected for the next step of purification on DEAE-Sepharose. The proteins bound on DEAE-Sepharose were eluted successively with 0.2 M NaCl and 1 M NaCl in 20 mM NH4HCO3 buffer (pH 9.6). Peak D2 eluted with 0.2 M NaCl (), the only fraction with trypsin inhibitory activity, represented the target fraction. Chromatography of peak D2 on Mono Q 5/50 GL and elution with three successive linear gradients of NaCl (0–0.2 in 5 ml, 0.2–0.25 M in 15 ml, 0.25–1 M in 5 ml) resulted in separation into three major fractions M1, M2 and M3 (). The active fraction M2 was applied on Superdex 75 for final purification. After assay of each fraction generated by Superdex 75 for trypsin inhibitory activity, the major peak S2 () was found to be the only fraction with trypsin inhibitory activity and thus constituted the target protein. The image of SDS gel () attested to the purity of the isolated protein which appeared as a single band with a molecular weight around 16 kDa. summarizes the yield and specific activity at each step of purification. The purified trypsin inhibitor from Northeast China black beans was referred to as NCBBTI. The purified hemagglutinin from the same beans was referred to as NCBBH.
Figure 1. Purification of NCBBTI by liquid chromatography techniques. (A) Crude extract of Northeast China black beans was subjected to affinity chromatography on Affi-gel blue gel and the adsorbed fraction was eluted with 1 M NaCl. (B) Unadsorbed fraction B1 was loaded on a DEAE-Sepharose column and the adsorbed fraction was eluted with 0.2 M NaCl and 1 M NaCl. (C) Adsorbed fraction D2 was subsequently run on a Mono Q 5/50 GL column and the adsorbed fraction was eluted with three successive linear gradients of NaCl (0–0.2 in 5 ml, 0.2–0.25 M in 15 ml, 0.25–1 M in 5 ml) in 20 mM NH4HCO3 (pH 9.6) buffer. (D) Adsorbed fraction M2 fraction was separated by Superdex 75 10/300 GL column and the major peak S2 was found to the target protein. All fractions derived from each chromatography step were tested for tryspin inhibitory activity to make sure that the active fraction was chosen for further fractionation.
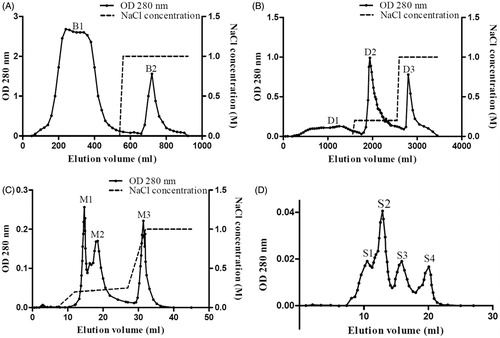
Figure 2. SDS–PAGE patterns of the purification of NCBBTI. From the right to the left, the lanes, respectively, represented the molecular weight marker proteins, the unbound fraction B1 from Affi-gel blue gel, the bound fraction D2 from DEAE-Sepharose, the bound fraction M2 from Mono Q and the fraction S2 from Superdex 75 and the molecular weight marker proteins.
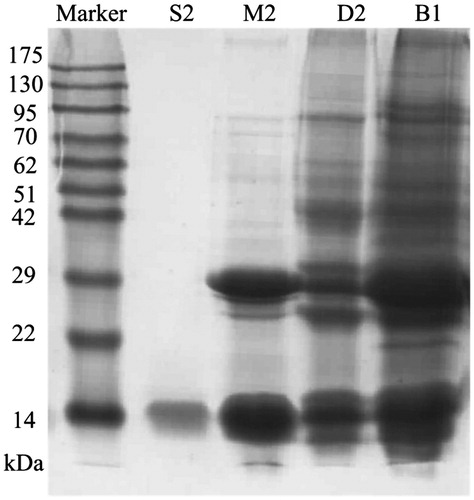
Table 1. Summary of purification of NCBBTI from 30 g Northeast China black beans.
Characterization of NCBBTI
The trypsin inhibitory activity of NCBBTI at different temperatures or pH values was, respectively, compared with its activity at 30 °C or pH 7. The results revealed that the trypsin inhibitory activity of NCBBTI remained almost intact up to 90 °C with 92.5% of its activity remaining (). Besides, this protein exhibited a wide range of pH tolerance, from pH 2 to 11 (). At the acidic pH value of 1 and the alkaline pH value of 13, there was, respectively, 82.9% and 78.1% of the activity left. After pretreatment of NCBBTI with DTT, its trypsin inhibitory activity was, respectively, curtailed by 81.8% and 86.4% at the concentrations of 1 and 10 mM DTT (. However, the trypsin inhibitory activity of NCBBTI remained almost intact after treatment with a lower concentration of DTT (0.1 mM).
Figure 3. Effect of (A) different temperatures and (B) pH values on NCBBTI. Results represent mean ± SD (n = 3).
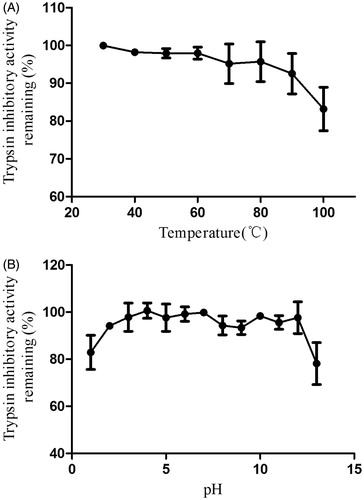
Figure 4. Inhibition rate (%) of DTT on the trypsin inhibitory activity of NCBBTI. NCBBTI were pretreated with different concentrations of DTT for 30 min before it was tested for trypsin inhibitory activity. A p values < 0.05 was considered as statistically significant, p < 0.05 (*), p < 0.01 (**), p < 0.001 (***) versus respective control groups. Results represent mean ± SD (n = 3).
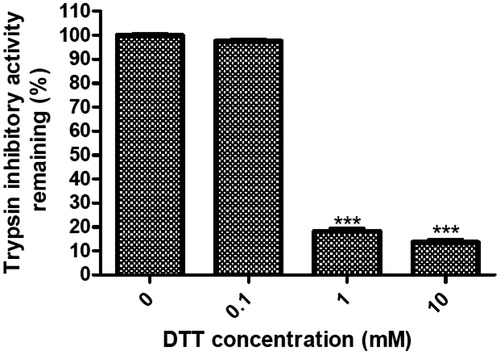
Anti-proliferative activity of NCBBTI and NCBBH
As the MTT results presented in , the presence of NCBBTI (up to 30 μM) had no inhibitory effect on proliferation of HONE-1 cells and a very weak proliferation inhibitory effect on CNE-1 cells. On the contrary, NCBBH could greatly attenuate the proliferation of both HONE-1 cells (IC50: 1.1 μM) and CNE-1 cells (IC50: 2 μM) in a dose-dependent manner ().
NCBBTI and NCBBH attenuated the mobility of nasopharyngeal carcinoma cells
The migration of HONE-1 and CNE-1 cells to the wound was observed in the absence or presence of NCBBTI and NCBBH. The widths of scratched gaps of treated cells were compared with control after the wound had been created for 24 h. For HONE-1 cells which, respectively, received no treatment of proteins, 31.25 μM NCBBTI () or 0.68 μM NCBBH (), the width of the gaps was decreased by 45%, 30% and 0.8% at 24 h. For CNE-1 cells which, respectively, received no treatment of proteins, 31.25 μM NCBBTI () or 0.68 μM NCBBH (), the width of the gaps was decreased by 20%, 13% and 1.1% when compared with the initial width of the gaps (0 h).
Figure 6. Wound-healing assay of NCBBTI and NCBBH on nasopharyngeal carcinoma cells. After the wound had been created, cells were cultured with FBS-free medium (control) or FBS-free medium containing (A) 31.25 μM NCBBTI or (B) 0.68 μM NCBBH for 24 h before the wound closure was measured. p < 0.05 (*), p < 0.01 (**), p < 0.001 (***) versus respective control groups. Results are represented as mean ± SD (n = 6).
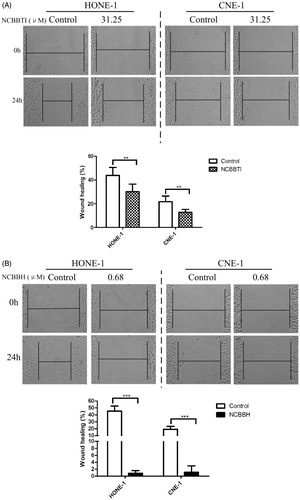
Discussion and conclusion
In the present study, two legume defense proteins were isolated from an edible legume, the Northeast China black beans. The hemagglutinin, NCBBH, was purified by following the methods that have been previously reported by usCitation17. The trypsin inhibitor, NCBBTI, which has not been reported before, was isolated in four steps which included the application of Affi-gel blue gel, DEAE Sepharose, Mono Q Sepharose and Superdex-75. This method of purification resulted in a yield of approximately 10%. The specific activity of NCBBTI towards trypsin was approximately 4,194 U/mg, which was highly efficient compared with other legume trypsin inhibitors. For example, the specific activity of trypsin inhibitors isolated from small pinto beansCitation10 and brown kidney beansCitation12 were, respectively, 2.398 and 1.240 unit/mg. This trypsin inhibitor had a molecular weight of around 16 kDa and its trypsin inhibitory activity was resistant to high temperatures. It was stable over a wide range of pH values. As a matter of fact, the majority of legume trypsin inhibitors or trypsin inhibitors isolated from other plants are highly pH- and thermo-stable. This property is mainly attributed to a large number of internal disulfide bonds and polar interactions between sub-domainsCitation19.
Legume protease inhibitors have no carbohydrates and belong to Kunitz trypsin inhibitors (KTI) or Bowman–Birk inhibitors (BBI)Citation20. The molecular weights of BBI vary from 1513 to 20 000 Da and they inhibit both trypsin and chymotrypsin, while KTI are larger and exhibit strong inhibition effect on trypsinCitation21. BBI share similar structures and a common fold for the reactive site, which is formed by a disulfide-linked β-sheet loopCitation19,Citation22. Trypsin inhibitors inactivate the digestive property of trypsin by blocking its active cite and the binding process requires a specific three-dimensional structure which is held by disulfide bonds. Therefore, the inhibitory functions of trypsin inhibitors could be reduced by treatment with DTT which could damage the disulfide bonds of proteins. Consistently, the trypsin inhibitory activity of NCBBTI could also be impaired by DTT treatment as what had been described in the results.
Lectins/hemagglutinins, which are another important type of defense proteins, are found to be ubiquitously expressed at genome and transcriptome levels in plantsCitation23. This type of proteins is characterized by the unique property of initiating reversible and specific binding with carbohydrate structures. By the overall domain architectures, plant lectins are distinguished into merolectins, hololectins, superlectins and chimerolectins, among which the first three types, respectively, possess only one, two or more identical/homologous and two or more different carbohydrate-binding domains while chimerolectins, the last type, have at least one carbohydrate-binding domains that are fused to a domain with different biological functionsCitation1. Plant lectins/hemagglutinins could defend against plant attackers through their interactions with the glycoconjugates present in organismsCitation1. For example, the midgut epithelium of many insects is rich in glycoproteins and becomes a target of plan lectins. Clear aberrations could be detected in the peritrophic matrix of cotton leafworm larvae fed on diet containing lectinsCitation1. Due to their special roles in plant self-protection, such defense proteins have also been proposed to be applied in agriculture. For instance, transgenic rice which expressed snowdrop lectin was found to be more resistant to pest insects by reducing the survival rates and fecundityCitation24.
Other than the potential application in agriculture, many plant defense proteins have also been reported to demonstrate other biological functions. According to the report of Fang et al., the trypsin inhibitors isolated from Bauhinia purpurea L. seeds could induce apoptosis in hepatocellular carcinoma HepG2 cells and had anti-proliferative effects on several other cancer cell lines, like CNE-1, CNE-2 and MCF-7 cellsCitation25. A Bowman–Birk trypsin inhibitor from Hokkaido large black soybeans could also suppress the proliferation of MCF-7 and Hep G2 cells, but these two cell lines were not equally sensitive to the anti-proliferative effect of black soybean that the IC50 were, respectively, 35 and 140 μM for MCF-7 cells and Hep G2 cells at 72 hCitation26. A tuber lectin from Arisaema heleborifolium Schott was reported to inhibit the proliferation of several human cancer cell linesCitation27. The application of lectins, like concanavalin A, P. vulgaris lectin (PHA) and mistletoe to the treatment of cancer has even been put through pre-clinical studiesCitation28.
In the present study, the anti-proliferative effects of the two defense proteins (NCBBTI and NCBBH) isolated from the Northeast China black beans were evaluated on two nasopharyngeal carcinoma cell lines. Our results indicated that NCBBTI and NCBBH demonstrated different anti-proliferative capacities. NCBBTI could hardly or only slightly attenuate the proliferation of nasopharyngeal carcinoma cells, while NCBBH could markedly suppress their proliferation even at a very low concentration. Interestingly, though NCBBTI failed to attenuate the proliferation of nasopharyngeal carcinoma cells, both defense proteins were found to impair mobility of nasopharyngeal carcinoma cells. The anti-mobility activity of NCBBTI could be explained by the fact that proteases are important factors in many biological processes including cancer progressionCitation29 and migration of cancer cells, which require the activation of several extracellular matrix-degrading enzymes which may include matrix metalloproteinases, serine proteases and cathepsins to detach cancer cells from the original tissue matrixCitation30. Therefore, protease inhibitors, like NCBBTI, could possible attenuate the mobility of cancer cells by suppressing the activity of certain proteases required in the migration process. Consistently, a research group from Japan had reported that the trypsin inhibitor isolated from human urine could inhibit the lung metastasis of mouse Lewis lung carcinoma cells and they proposed an anti-metastatic therapy by combining this urine trypsin inhibitor with anti-cancer drugsCitation31. However, NCBBH, which is a type of hemagglutinin/lectin, may modulate the mobility of nasopharyngeal carcinoma cells in a different way by interacting with the glycoproteins present on the cell membranes and interrupting the mobility of cells.
Declaration of interest
The author(s) confirm that this article content has no conflicts of interest.
References
- Vandenborre G, Smagghe G, Van Damme EJ. Plant lectins as defense proteins against phytophagous insects. Phytochemistry 2011;72:1538–50
- Michiels K, Van Damme EJ, Smagghe G. Plant–insect interactions: what can we learn from plant lectins? Arch Insect Biochem Physiol 2010;73:193–212
- Peumans WJ, Vandamme EJM. Lectins as plant defense proteins. Plant Physiol 1995;109:347–52
- Terras FRG, Eggermont K, Kovaleva V, et al. Small cysteine-rich antifungal proteins from radish: their role in host defense. Plant Cell 1995;7:573–88
- Reinbothe S, Reinbothe C, Lehmann J, et al. Jip60, a methyl jasmonate-induced ribosome-inactivating protein involved in plant stress reactions. Proc Natl Acad Sci USA 1994;91:7012–16
- Hartl M, Giri AP, Kaur H, Baldwin IT. The multiple functions of plant serine protease inhibitors: defense against herbivores and beyond. Plant Signal Behav 2011;6:1009–11
- Pontual EV, de Lima Santos ND, de Moura MC, et al. Trypsin inhibitor from Moringa oleifera flowers interferes with survival and development of Aedes aegypti larvae and kills bacteria inhabitant of larvae midgut. Parasitol Res 2014;113:727–33
- Oparin PB, Mineev KS, Dunaevsky YE, et al. Buckwheat trypsin inhibitor with helical hairpin structure belongs to a new family of plant defence peptides. Biochem J 2012;446:69–77
- Dabhade A, Patel P, Pati U. Proteinaceous protease inhibitor from Lawsonia inermis: purification, characterization and antibacterial activity. Nat Prod Commun 2013;8:1467–70
- Chan YS, Zhang Y, Sze SC, Ng TB. A thermostable trypsin inhibitor with antiproliferative activity from small pinto beans. J Enzym Inhib Med Chem 2014;29:485–90
- Ryan CA. Protease inhibitors in plants – genes for improving defenses against insects and pathogens. Annu Rev Phytopathol 1990;28:425–49
- Chan YS, Zhang Y, Ng TB. Brown kidney bean Bowman–Birk trypsin inhibitor is heat and pH stable and exhibits anti-proliferative activity. Appl Biochem Biotechnol 2013;169:1306–14
- Toscano MA, Ilarregui JM, Bianco GA, et al. Dissecting the pathophysiologic role of endogenous lectins: glycan-binding proteins with cytokine-like activity? Cytokine Growth Factor Rev 2007;18:57–71
- Zhang W, Peumans WJ, Barre A, et al. Isolation and characterization of a jacalin-related mannose-binding lectin from salt-stressed rice (Oryza sativa) plants. Planta 2000;210:970–8
- Chen Y, Peumans WJ, Hause B, et al. Jasmonic acid methyl ester induces the synthesis of a cytoplasmic/nuclear chito-oligosaccharide binding lectin in tobacco leaves. FASEB J 2002;16:905–7
- Lannoo N, Vandenborre G, Miersch O, et al. The jasmonate-induced expression of the Nicotiana tabacum leaf lectin. Plant Cell Physiol 2007;48:1207–18
- Dan XL, Wong JH, Fang EF, et al. Purification and characterization of a novel hemagglutinin with inhibitory activity toward osteocarcinoma cells from Northeast China Black beans. J Agric Food Chem 2015;63:3903–14
- Chia JS, Du JL, Hsu WB, et al. Inhibition of metastasis, angiogenesis, and tumor growth by Chinese herbal cocktail Tien–Hsien liquid. BMC Cancer 2010;10:175
- Qi RF, Song ZW, Chi CW. Structural features and molecular evolution of Bowman–Birk protease inhibitors and their potential application. Acta Biochim Biophys Sin 2005;37:283–92
- Guillamon E, Pedrosa MM, Burbano C, et al. The trypsin inhibitors present in seed of different grain legume species and cultivar. Food Chem 2008;107:68–74
- Losso JN. The biochemical and functional food properties of the Bowman–Birk inhibitor. Crit Rev Food Sci Nutr 2008;48:94–118
- Barbosa JA, Silva LP, Teles RC, et al. Crystal structure of the Bowman–Birk inhibitor from Vigna unguiculata seeds in complex with beta-trypsin at 1.55 A resolution and its structural properties in association with proteinases. Biophys J 2007;92:1638–50
- Van Damme EJM, Lannoo N, Peumans WJ. Plant lectins. Adv Bot Res 2008;48:107–209
- Rao KV, Rathore KS, Hodges TK, et al. Expression of snowdrop lectin (GNA) in transgenic rice plants confers resistance to rice brown planthopper. Plant J 1998;15:469–77
- Fang EF, Bah CS, Wong JH, et al. A potential human hepatocellular carcinoma inhibitor from Bauhinia purpurea L. seeds: from purification to mechanism exploration. Arch Toxicol 2012;86:293–304
- Ho VS, Ng TB. A Bowman–Birk trypsin inhibitor with antiproliferative activity from Hokkaido large black soybeans. J Pept Sci 2008;14:278–82
- Kaur M, Singh K, Rup PJ, et al. A tuber lectin from Arisaema helleborifolium Schott with anti-insect activity against melon fruit fly, Bactrocera cucurbitae (Coquillett) and anti-cancer effect on human cancer cell lines. Arch Biochem Biophys 2006;445:156–65
- Liu B, Bian HJ, Bao JK. Plant lectins: potential antineoplastic drugs from bench to clinic. Cancer Lett 2010;287:1–12
- Clemente A, MacKenzie DA, Johnson IT, Domoney C. Investigation of legume seed protease inhibitors as potential anticarcinogenic proteins. EAAP Publication No 110. Wageningen Academic Publishers; 2004:137–41
- Birkedalhansen H. Proteolytic remodeling of extracellular-matrix. Curr Opin Cell Biol 1995;7:728–35
- Kobayashi H, Shinohara H, Gotoh J, et al. Anti-metastatic therapy by urinary trypsin inhibitor in combination with an anti-cancer agent. Br J Cancer 1995;72:1131–7