Abstract
There are several hypotheses that explain stomatal behavior. These include the concept of osmoregulation mediated by potassium and its counterions malate and chlorine and the more recent starch–sugar hypothesis. We have previously reported that the activity of the sucrose cleavage enzyme, vacuolar invertase (VIN), is significantly higher in guard cells than in other leaf epidermal cells and its activity is correlated with stomatal aperture. Here, we examined whether VIN indeed controls stomatal movement under normal and drought conditions by transforming Arabidopsis with a tobacco vacuolar invertase inhibitor homolog (Nt-inhh) under the control of an abscisic acid-sensitive and guard cell-specific promoter (AtRab18). The data obtained showed that guard cells of transgenic Arabidopsis plants had lower VIN activity, stomatal aperture and conductance than that of wild-type plants. Moreover, the transgenic plants also displayed higher drought tolerance than wild-type plants. The data indicate that VIN is a promising target for manipulating stomatal function to increase drought tolerance.
Introduction
Stomatal movement is critical in plant response to drought. Plants naturally exhibit drought stress tolerance by developing a relative large and deep root system for water absorption from soil. Other adaptation to drought include the development of wax on the leaf surface and the rapid closure of stomata when abscisic acid (ABA) or other drought signals are detected by the guard cells to minimize or to avoid water evaporation. Abiotic stresses, specifically drought, exert severe negative effects on crop yield. Therefore, research on drought tolerance has attracted considerable attention and investment from private, public, academic and philanthropic sectorsCitation1.
Numerous strategies have been developed to genetically induce drought tolerance in plants. However, most of these strategies reduce fitness or associate with yield loss. An effective strategy to induce drought tolerance is the utilization of organ- or tissue-specific and/or drought-inducible promoters. Accumulation of osmoticum, mainly sugars and ions in guard cells generates osmotic potential to attract influx of water, thereby allowing expansion of the guard cells and subsequently leading to the opening of the stomata. On the other hand, ABA concentration increases when plants are grown or cultured under drought conditions, which often induces the closure of stomata. The post-transcriptional regulation of invertase by invertase inhibitors is an highly effective way to reduce invertase activity in transgenic plantsCitation2,Citation3. In the present study, we tested the hypothesis that reducing the activity of vacuolar invertase (VIN) in guard cells may decrease stomatal aperture and confer drought tolerance. This possibility was examined by expression of the tobacco VIN inhibitor gene Nt-inhhCitation2 in Arabidopsis under the control of the ABA-sensitive, anion channel-dependent and guard cell-specific promoter AtRab184–6.
The activity of VIN was considerably higher in guard cells than in other leaf epidermal cells and correlated with stomatal aperture in wild type ArabidopsisCitation4. We also compared the stomatal aperture of Arabidopsis T-DNA insertion mutant in At1g12240 (AtVIN2) to that of wild type and transgenic Arabidopsis expressing the cotton vacuolar invertase gene (GhVIN1) under 35S promoter. However, the mutant and overexpression transgenic plants did not exhibit obvious changes in susceptibility or resistance to drought stress as compared to the wild-type plants. To elucidate the relationship between VIN activity in guard cells and drought stress, Arabidopsis plants were introduced with a construct of the tobacco VIN inhibitor gene Nt-inhh controlled by the ABA-inducible and guard cell specific AtRab18 promoterCitation5–7 through Agrobacterium tumefaciens-mediated transformation. The transgenic lines were primarily selected on kanamycin, and the presence of the transgene was further confirmed by genomic polymerase chain reaction (PCR) analysis. We observed strong drought resistance of the resultant transgenic plants throughout their entire development without negative effects on plant fitness under optimal growth conditions.
Methods
Plant materials
Seeds of A. thaliana (Columbia wild type) were surface sterilized and sown on one half strength Murashige and Skoog medium containing 2% (w/v) sucrose and 0.75% (w/v) agar under short-day conditions (8-h light, 16-h dark) at 22 °C. After 2 weeks, the plants were transplanted on soil and grown under long-day conditions (16-h light, 8-h dark) at 22 °C.
Plasmid construction and plant transformation
To clone the AtRab18 promoter, a 2.4 kb fragment upstream from the start codon and 5′untransformed region was amplified by PCR from the genomic DNA of Arabidopsis ecotype Col-0. The AtRab18 promoter was more than 2 kb long with two SalI restriction sites, two fragments, a 1570 bp 5′ fragment and a 900-bp 3′ fragment. The AtRab18 promoter was amplified by PCR from the Arabidopsis genomic DNA via the primers listed in Table S1. One XbaI restriction site was introduced to the 3′ end of the AtRab18 promoter via primer (3′ fragment –R, listed in Table S1). The PCR products were cloned into the pUCm-T vector (Sangon Biotech, Shanghai, China) and sequenced. The promoter fragments were then excised via XbaI/SacI and Sal1 restrictions and subcloned into the binary vector pBI101 to form pBI101–1-AtRab18::gus.
To construct pBI101-AtRab18::Nt-inhh, 560 bp Nt-inhh fragments were amplified by PCR from the tobacco Nt-inhh cDNA via the primers listed in Table S1. The PCR products were cloned into the pUCm-T vector and then sequenced. The Nt-inhh fragment was then excised via XbaI/SacI restriction and subcloned into the binary vector pBI101–1-AtRab18::gus to replace the gus gene and generate pBI101-AtRab18::Nt-inhh.
The resulting binary vectors (pBI101-AtRab18::gus and pBI101-AtRab18::Nt-inhh) were introduced into Arabidopsis via Agrobacterium-mediated transformation.
Semiquantitative and quantitative RT-PCR analysis
Total RNA was extracted from rosette leaves of Arabidopsis using MiniBEST Plant RNA Extraction Kit (TaKaRa) and was digested with DNase (TaKaRa). First-strand cDNA was synthesized from total RNA using PrimeScriptTMII (TaKaRa) with oligo dT primer. PCR employed the primers (Nt-inhh-RT-F and Nt-inhh-RT-R) to determine AtRab18:.:Nt-inhh transcript. The Arabidopsis actin2 gene was used as an internal control.
For quantitative RT-PCR analysis, reverse transcription was performed by PrimeScriptTM RT Master Mix (TaKaRa). The cDNA was mixed with primers and SYBR® Premix Ex Taq (Tli RNaseH Plus; TaKaRa) in accordance with the manufacturer’s instructions. The products were amplified, and fluorescence signals were acquired with a LightCycler®II480 (Roche) detection system. Actin2 was used as an internal control. The relative quantization method (ΔΔCT) was used to evaluate the quantitative variation between the replicates examinedCitation8. The primers used in semi-quantitative and quantitative RT-PCR analysis are listed in Table S2.
Histochemical analysis of GUS activity
T1 and T2 lines were analyzed for GUS activity following the protocol described at http://preuss.bsd.uchicago.edu/gus.html, except that the reaction was performed for 1 h to 2 h. After incubation in different concentrations of alcohol for dehydration and rehydration, the specimens were observed by light microscopy (Motic BA300, Motic China Co. Ltd.) with a cooled CCD camera for imaging.
Vacuolar invertase activity analysis of guard cells
Isolation of Arabidopsis guard cells was isolated from rosette leaves following the protocol described by Pandey et al.Citation9. Vacuolar invertase activity of guard cells was analyzed in accordance with the method described by Tomlinson et al.Citation10.
Measurement of stomatal aperture and conductance
The tested Arabidopsis plants were subjected to long-day conditions (16 h) to ensure that the stomata were open the night before the experiment. Intact Arabidopsis rosette leaves were collected for stomatal aperture measurement the next morning. Epidermal strips were manually separated carefully from each leaf and floated in a solution containing 50 μL CaCl2, 10 mM KCl and 10 mM MES [2-(N-morpholino) ethanesulfonic acid]-Tris (pH 6.15). Subsequently, treated strips were mounted onto a slide for observation by light microscopy (Motic BA300) after ABA treatment for 15 or 30 min. The aperture (ratio of stoma width to length) was calculated from the records of more than 50 digitized images of abaxial stomata in three epidermal strips using image analysis software (Image J). Each experiment was performed in triplicate. Results presented are the mean aperture values of all replicates. Standard deviations were calculated to compare the stomatal apertures among all the different treatments. The LI-6400 XT portable photosynthesis system (LI-COR Biosciences) was used to measure the stomatal conductance of transgenic and wild-type plants.
Drought stress treatments
For water loss measurement, leaves were detached from wild-type plants and from transgenic lines that express Nt-inhh under the control of the AtRab18 promoter. Fresh weight (FW) loss was monitored at indicated time points. RWC was measured after drought stress. The leaves were detached and weighed for the initial FW, submerged in water for 24 h to measure the saturated weight (SW), and then dried in an oven at 85 °C for 48 h to measure the dry weight (DW). RWC was expressed as (FW − DW)/(SW − DW) × 100.
Results
To confirm the cell specificity of the AtRab18 promoter, transgenic plants that express the β-glucuronidase (gus) gene under AtRab18 were generated. We found that the gus gene was specifically expressed in guard cells (Figure S1) and was highly stimulated by exogenous ABA application () as expectedCitation5.
Figure 1. Expression of gus of transgenic Arabidopsis plants expressing AtRab18::gus fuze gene after ABA (10 μmol/L) application was significantly higher than that without ABA application. Asterisk indicate significant differences (Student’s t-test, **p < 0.01) between ABA treatment samples and the controls.
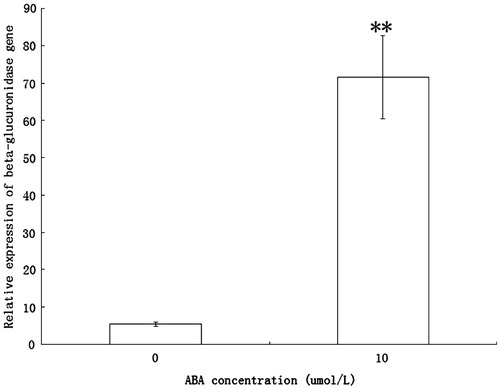
Semiquantitative reverse transcription PCR (RT-PCR) analysis was performed to detect the ectopic expression of Nt-inhh. Pronounced Nt-inhh expression was observed in transgenic lines 4 and 5 but not in wild-type plants and other transgenic lines tested (). Quantitative RT-PCR analysis was then performed to confirm the expression results. As shown in , transgenic lines 4 and 5 had relatively strong transgene expression, whereas line 2 displayed only low level of Nt-inhh mRNA. No transgene expression was detected in wild-type plants and line 7. Therefore lines 4 and 5 were chosen for further analysis.
Figure 2. (A) Quantitative RT-PCR analysis of Nt-inhh mRNA in guard cells of transgenic Arabidopsis lines that expressed Nt-inhh under the control of AtRab18. (B) Semiquantitative RT-PCR analysis of guard cells from transgenic Arabidopsis lines that express Nt-inhh under the control of AtRab18. The Arabidopsis actin2 gene was used as an internal control. Wild type was untransformed control; 2#, 4#, 5# and 7# were transgenic lines.
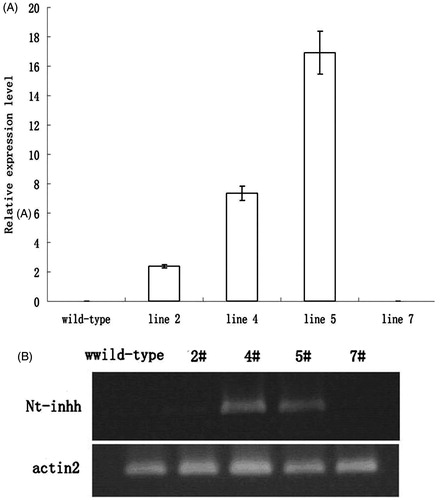
Because guard cell-specific and ABA-sensitive promoter was used to generate transgenic Arabidopsis, invertase activity of guard cells was measured. Compared with wild-type plants, transgenic lines showed lower vacuolar invertase activities of guard cells significantly (p < 0.01), and it was decreased when treated with exogenous ABA (10 μmol/L) application (). Then, we investigated the potential changes in stomatal aperture of the transgenic lines because we have previously reported that VIN activity positively correlates with stomatal apertureCitation4. Here, the measurement revealed that the stomatal aperture of the VIN-suppressed transgenic lines was considerably smaller than that of wild-type plants (). The stomatal conductance of transgenic lines was significantly reduced as compared to that of wild-type plants (Figure S2). Furthermore, there were not any significant differences in rosette diameter, number of seeds and seed germination rate between transgenic plants and the control (Table S3)
Figure 3. (A) Vacuolar invertase activity in guard cells of transgenic Arabidopsis expressing Nt-inhh under the control of AtRab18 was less than that of wild-type plants, and it decreased after treated with ABA (10 μmol/L) for 0, 15, 30 min. Wild type was untransformed control; 4# and 5# were transgenic lines. (B) VIN activity in guard cells of vin2 mutant was less than that of wild type. Asterisk indicate significant differences (Student’s t-test, *p < 0.05) between inv2 (T-DNA insertion mutant line, At1g12240, SALK_100813) and the control (wild type) .
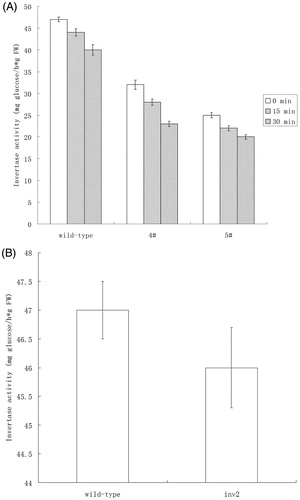
Figure 4. Stomatal aperture of transgenic Arabidopsis expressing Nt-inhh under the control of AtRab18 was less than that of wild-type plants. Wild type was untransformed control; 4# and 5# were transgenic lines. Asterisk indicate significant differences (Student’s t-test, **p < 0.01) between transgenic plant and the controls.
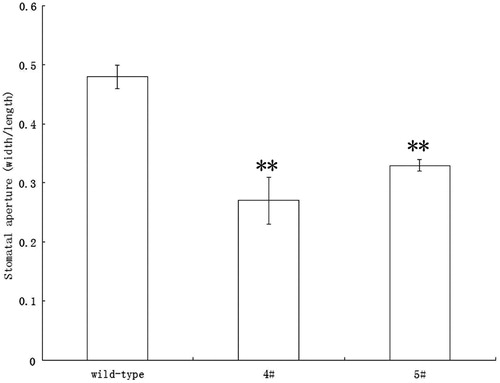
Since stomatal movement is critical in the drought stress tolerance of plants, the potential effect of suppressing VIN activity on drought tolerance was investigated. The transgenic plants displayed evident drought resistance phenotype (Figure S3). We then measured water loss from the rosette leaves of wild type and transgenic lines in the presence of light. As shown in , water loss was much slower in detached leaves of transgenic plants than in those of wild-type plants. To confirm this result, the relative water content (RWC) was determined. As shown in , the RWC of transgenic lines after drought treatment for 18 days was considerably lower than that of wild-type plants. These results strongly indicate that the introduction of tobacco VIN inhibitor (Nt-inhh) under the control of the AtRab18 promoter improves drought tolerance in Arabidopsis. The findings provide a compelling case for generating drought tolerant crops through suppressing VIN activity in guard cells.
Figure 5. Fresh weight loss was significantly slower in transgenic lines than that in wild-type plants. Wild type was untransformed control; 4# and 5# were transgenic lines. Asterisks indicate significant differences (Student’s t-test, *p < 0.05 and **p < 0.01) between transgenic plants and the controls.
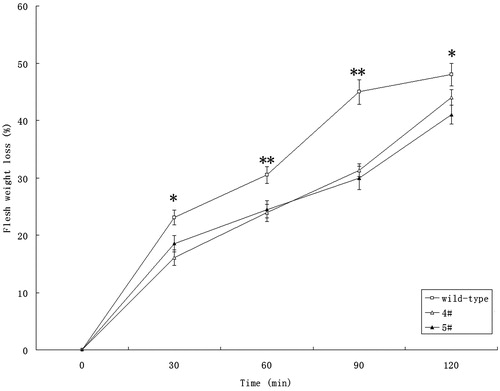
Figure 6. Relative water content (RWC) after 18 days of drought treatment was considerably higher in the two transgenic lines than that in wild-type plants. Wild type was untransformed control; 4# and 5# were transgenic lines. Asterisk indicate significant differences (Student’s t-test, **p < 0.01) between transgenic plant and the controls.
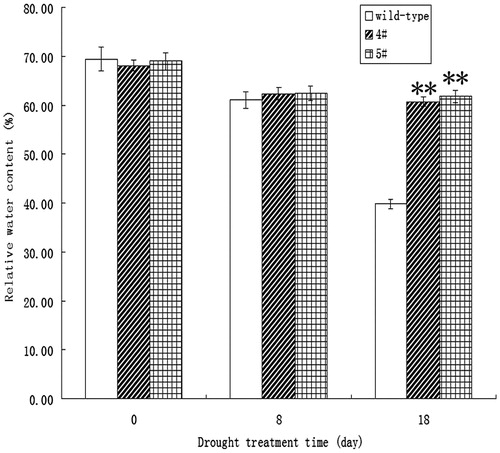
Discussion
Here, we show that VIN activity indeed controls stomatal aperture based on the observation that repression of VIN activity by ectopically expressing a VIN inhibitor under the control of a guard cell-specific promoter resulted in a smaller stomatal aperture in transgenic A. thaliana as compared to the wild-type plants (). The exact mechanism of stomatal movement regulated by guard cell VIN activity remains to be elucidated. A likely scenario is that VIN hydrolyses sucrose into glucose and fructose, thereby doubling the osmolality of the sugars, leading to influx of water to the vacuole to drive the opening of the guard cellsCitation11,Citation12. Other possibilities include the potential impact of VIN-mediated sugar signaling on the behavior of guard cellsCitation13. Changes in VIN activity may also be regulated by the pH within the vacuole through potential modulation of the activities of H+/sugar antiporters on the tonoplast or other transporters for potassium, chloride and malate, across guard cell membranesCitation13. To this end, it has been reported that guard cell vacuolar pH increases during stomatal closingCitation14, but it decreases during stomatal openingCitation15.
The present results also showed that the introduction of tobacco VIN inhibitor (Nt-inhh) under the control of the AtRab18 promoter greatly improved drought tolerance in Arabidopsis. In this context, we have reported previously that the T-DNA insertion mutant line (At1g12240, SALK_100813) for AtVIN2 did not show drought stress toleranceCitation7. The possible reason for the lack of phenotype could be that VIN activity of mutant line may not be reduced enough in the leaves, since AtVIN2 was mainly expressed in roots and the second VIN gene, AtVIN1, may function normally in the leaves of the mutantCitation12. Indeed, VIN activity in guard cells of vin2 mutant was less than that of wild type (). In this study, we used a guard cell specific and ABA inducible promoter to drive the expression of an inhibitor against VIN activity. Thus, the VIN activity was reduced much more in the guard cells in these transgenic lines as compared to the vin2 mutant since the AtRab18 promoter activity is not only expressed in guard cells, but also expected to increase rapidly when ABA concentration increases under drought ().
Although plant responses to drought stress have been intensively studied for decades, progress in the development of tolerant crops has been slowCitation1. Maintaining growth, photosynthesis and metabolism are the major goals to minimize the impact of abiotic stress on crop yieldCitation1. Here, we showed that expression of the Nt-inhh specifically in guard cells improved A. thliana adaptation to drought by reducing VIN activity and consequently stomatal aperture without negative effect on growth.
Supplementary material available online
IENZ_1142981_Supplementary_Figures.pdf
Download PDF (248 KB)Acknowledgements
We would like to thank Dr. Ke Duan (Shanghai Academy of Agricultural Sciences) for her assistance in quantitative RT-PCR analysis.
Declaration of interest
This work was initially funded by National Science Foundation of China (30425043) and subsequently supported by grants from the Science Foundation of the Shanghai Municipal Science and Technology Commission (13ZR1441300) and the Shanghai Institute of Technology Scientific Research Foundation for Introduced Talent (YJ2012–26). The authors report no conflicts of interest.
References
- Albacete A, Cantero-Navarro E, Grosskinsky DK, et al. Ectopic overexpression of the cell wall invertase gene CIN1 leads to dehydration avoidance in tomato. J Exp Bot 2015;66:863–78
- Greiner S, Rausch T, Sonnewald U, Herbers K. Ectopic expression of a tobacco invertase inhibitor homolog prevents cold-induced sweetening of potato tubers. Nat Biotechnol 1999;17:708–11
- Jin Y, Ni DA, Ruan YL. Posttranslational elevation of cell wall invertase activity by silencing its inhibitor in tomato delays leaf senescence and increases seed weight and fruit hexose level. Plant Cell 2009;21:2072–89
- Ni DA. Role of vacuolar invertase in regulating Arabidopsis stomatal opening. Acta Physiol Plant 2012;34:2449–52
- Ghelis T, Dellis O, Jeannette E, et al. Abscissic acid specific expression of RAB18 involves activation of anion channels in Arabidopsis thaliana suspension cells. FEBS Lett 2000;474:43–7
- Ghelis T, Dellis O, Jeannette E, et al. Abscisic acid plasmalemma perception triggers a calcium influx essential for RAB18 gene expression in Arabidopsis thaliana suspension cells. FEBS Lett 2000;483:67–70
- Yang Y, Costa A, Leonhardt N, et al. Isolation of a strong Arabidopsis guard cell promoter and its potential as a research tool. Plant Methods 2008;4:6
- Livak KJ, Schmittgen TD. Analysis of relative gene expression data using real-time quantitative PCR and the 2(-Delta Delta C(T)) method. Methods 2001;25:402–8
- Pandey S, Wang XQ, Coursol AS, Assmann SM. Preparation and applications of Arabidopsis thaliana guard cell protoplasts. New Phytologist 2002;153:517–26
- Tomlinson KL, McHugh S, Labbe H, et al. Evidence that the hexose-to-sucrose ratio does not control the switch to storage product accumulation in oilseeds: analysis of tobacco seed development and effects of overexpressing apoplastic invertase. J Exp Bot 2004;55:2291–303
- Ruan YL, Jin Y, Yang YJ, et al. Sugar input, metabolism, and signaling mediated by invertase: roles in development, yield potential, and response to drought and heat. Mol Plant 2010;3:942–55
- Wang L, Li XR, Lian H, et al. Evidence that high activity of vacuolar invertase is required for cotton fiber and Arabidopsis root elongation through osmotic dependent and independent pathways, respectively. Plant Physiol 2010;154:744–56
- Wang L, Cook A, Patrick JW, et al. Silencing the vacuolar invertase gene GhVIN1 blocks cotton fiber initiation from the ovule epidermis, probably by suppressing a cohort of regulatory genes via sugar signaling. Plant J 2014;78:686–96
- Li YX, Sun P, Zhu GL. Preliminary study on reversible polymerization and depolymerization of particles regulated by guard cell vacuolar pH. J China Agri Univ 2005;10:10–14
- Ye Q, Zhu GL, Lou CH. Effects of some polymer depolymerization of guard cell vacuole on osmotic regulation during stomatal opening. Chin Sci Bull 2003;48:260–3