Abstract
A bioautographic assay based on thin layer chromatography was developed for phosphoenolpyruvate (PEP) detecting as a known but rarely studied inhibitor of phosphoglucose isomerase (PGI). The protocol with NADP+/NBT/PMS (β-nicotinamide adenine dinucleotide phosphate/nitrotetrazolium blue chloride/phenazine methosulfate) staining was capable of detecting Mycobacterium tuberculosis H37Ra PGI inhibition using PEP. According to this method, visibly brighter spots (zones) against purple background are observed in the area of inhibition of the above-mentioned enzyme activity. The detection limit for PEP as an inhibitor of Mycobacterium tuberculosis H37Ra PGI was 226 μg per spot/zone. Noteworthy is that we are the first authors to have successfully used a bioautographic assay to detect Mycobacterium tuberculosis H37Ra PGI inhibition by PEP.
Introduction
Mycobacterium tuberculosis (Myc. tbc), the causative agent of tuberculosis, is still a major burden to human healthCitation1. The World Health Organisation reported 5.7 million new TB cases in 2013Citation2. Phosphoglucose isomerase (PGI) (d-glucose-6-phosphate aldose-ketose isomerase, PGI, EC 5.3.1.9) is an enzyme that catalyses reversible isomerisation of glucose-6-phosphate to fructose-6-phosphate. PGI plays a key role in the metabolic pathways of phosphorylated sugars, such as glycolysis, phosphate pentose cycle, Entner–Doudoroff cycle, gluconeogenesis, glycogenogenesis and glycogenolysis. Due to this, PGI is sometimes described as a “workhorse” or “housekeeping” enzyme of sugar metabolismCitation3,Citation4. Apart from its enzymatic function, this protein has a number of other “moonlighting” functionsCitation5. In mycobacteria, PGI is engaged through glucose-6P with l-rhamnose and is involved in the biosynthesis of the galactan residue of arabinogalactan, the components of the cell wall of these microorganismsCitation6–8.
The major application of bioautography is screening of the antimicrobial and antifungal properties of various plant samples with Thin Layer Chromatography (TLC)-platesCitation9. The term “autography” is used to detect other biological processes, e.g. enzyme inhibitionCitation10. However, the definition of the bioautographic method is often broadened and covers the determination of enzyme inhibition, antioxidant agents as well as oestrogenic compoundsCitation9,11. In our opinion, there is a clear trend towards the use of the TLC bioautography technique as a method for screening active compounds against microorganisms rather than a method for the detection of enzyme activities or inhibitors. The use of TLC (bio)autography was reported for the screening of inhibitors of acetylcholinesteraseCitation12–26, butyrylcholinesteraseCitation12,16,22,23,27, dipeptidyl peptidase IVCitation28, glucose-6-phosphate dehydrogenaseCitation29, α- and β-glucosidasesCitation30–34, lipaseCitation35–37, monoamine oxidases A and BCitation38,39, DNA topoisomerase ICitation40, tyrosinaseCitation41–43 and xanthine oxidaseCitation44. To date, the use of the TLC-based autographic method for the detection of PGI inhibition has only been developed for a biocatalyst isolated from the reference E. coli strain ATCC (American Type Culture Collection) 25922Citation45.
The mechanism of the bioautographic assay for determining the PGI activity is shown in . When PGI is not inhibited by phosphoenolpyruvate (PEP) or other inhibitors, the product of the reaction, glucose-6-phosphate, is converted into gluconolactone-6-phosphate by glucose-6-phosphate dehydrogenase (used as an auxiliary enzyme); the simultaneously generated NADPH (β-nicotinamide adenine dinucleotide phosphate (β-NADP+), reduced form) participates in a cycling reaction that employs the tetrazolium salt NBT (nitrotetrazolium blue chloride) to produce a purple coloured formazan with PMS (phenazine methosulfate) as an electron carrier.
Figure 1. The mechanism of bioautographic assay for the detection of phosphoglucose isomerase activity. Abbreviations used: NADP+, nicotinamide adenine dinucleotide phosphate; NADPH, nicotinamide adenine dinucleotide phosphate, reduced form; PMS, phenazine methosulfate; NBT, nitrotetrazolium blue chloride.
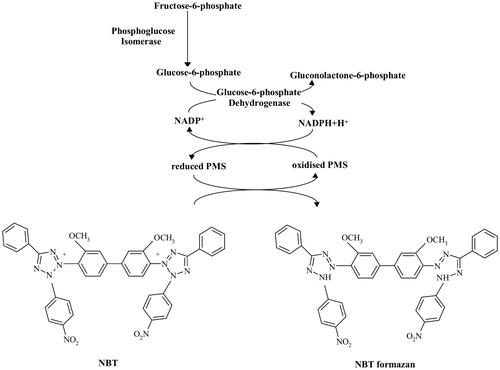
In this paper, a TLC bioautographic assay was established to detect PEP inhibition of PGI isolated from Mycobacterium tuberculosis (Myc. tbc) H37Ra strain (the commonly used surrogate for virulent Myc. tbc H37Rv strain). Noteworthy is that we are the first authors to have successfully used this method to detect mycobacterial PGI inhibition by PEP.
Materials and methods
Microbial cultures
Myc. tbc H37Ra bacteria (ATCC 25177) were maintained as a Löwenstein slant culture. The cells were also grown in mineral salt medium containing: glutamic acid (1% w/v); citric acid (0.2% w/v), KH2PO4 (0.05% w/v); MgSO4×7H2O (0.05% w/v); ferric ammonium citrate (0.005% w/v); ZnSO4×7H2O (0.0025% w/v) and the carbohydrate source – glucose (2.5% w/v). The pH of the medium was adjusted to 5.5 with KOH before autoclaving. The bacterial strain was cultured in 1 l Roux flasks containing 0.2 l medium at 37 °C for eight days. The cells were harvested by centrifugation, washed with sterile deionised water and stored as a paste at −18 °C until use.
Reagents and materials
Bovine serum albumin (BSA), β-NADP+, β-nicotinamide adenine dinucleotide (β-NAD+), dithiothreitol (DTT), fructose 6-phosphate (fru-6P), glucose, glucose 6-phosphate (glc-6P), glutamic acid, deoxyribonuclease I (DNase I) from bovine pancreas, glucose 6-phosphate dehydrogenase from Leuconostoc mesenteroides (L. mesenteroides), NBT, PMS, PEP and Tris-(hydroxymethyl)aminomethane (TRIS, TRIZMA® base) were all purchased from Sigma-Aldrich (St. Louis, MO). The components of the liquid medium for culturing mycobacterial cells (except glutamic acid and glucose), an organic solvent – ethanol (EtOH), and magnesium chloride hexahydrate were obtained from Avantor Performance Materials (Gliwice, Poland). All reagents were of analytical quality and were used without purification. Dialysis tubing cellulose membrane was purchased from Sigma-Aldrich (St. Louis, MO). Diaflo YM-100 (cutoff: 100 kDa) ultrafiltration membranes were obtained from Millipore Corp. (Bedford, MA). DEAE-Macro® Prep Support was obtained from BIO-RAD (Hercules, CA). Cellulose and silica gel 60 F254 plates were purchased from Merck (Darmstadt, Germany).
Purification of the PGI from Myc. tbc H37Ra
The enzyme used for TLC bioautography was partially purified using the steps outlined below. All purification steps were performed at 4 °C unless otherwise specified. The cells (30 g wet weight) were suspended in 150 ml of ice-cold 50 mM TRIS/HCl buffer with 2 mM DTT (pH 7.6) (buffer A), disrupted by sonic oscillation (3 × 5 min, 22 kHz, 180 W) and centrifuged at 3944×g for 30 min (Sigma 3-16K Centrifuge, Germany). Afterwards, DNase I (EC 3.1.21.1) was added (1 U/1 ml) to the supernatant and the sample was incubated overnight. The obtained liquid, designated as “crude extract”, was subjected to (NH4)2SO4 fractionation. The fraction precipitated at 50–70% saturation with (NH4)2SO4 was collected via centrifugation at 1753×g for 45 min (Sigma 3-16K Centrifuge, Germany), dissolved in a minimal volume of buffer A and dialysed against the same buffer overnight (AS-fraction). The AS-fraction was removed from the dialysis tubing and loaded onto the ion-exchange column Macro-Prep® DEAE Support (1.4 × 17 cm) previously equilibrated with buffer A. The column was washed thoroughly with the same buffer (150 ml) and the wash that did not exhibit the activity of PGI was discarded. Myc. tbc H37Ra PGI was eluted from the column with 0.2 M NaCl in buffer A (200 ml). Other proteins were eluted with 0.5 M NaCl in buffer A (150 ml). Fractions containing the active enzyme were pooled and concentrated on the Amicon filtration apparatus (Millipore, Billerica, MA) using a YM-100 membrane.
Enzyme assay
The determination of Myc. tbc H37Ra PGI activity was conducted via a coupled assay with glucose-6-phosphate dehydrogenase from L. mesenteroides (EC 1.1.1.363) as an auxiliary enzyme according to Fraenkel and Levisohn methodCitation46. The reduction of β-NADP+ intermediate with glc-6P dehydrogenase was followed by the increase in A340 nm. The standard assay mixture (1 ml) contained 50 mM TRIS/HCl buffer (pH 7.6), 1 mM d-fructose-6P, 2.5 mM MgCl2×6H2O, 1 mM NADP+ and 1 U of glc-6P dehydrogenase. After 1 min, the reaction was initiated by the addition of the PGI sample and it was followed by 2 min. The activity of the characterized biocatalyst was estimated using a spectrophotometer (double beam spectrophotometer Evolution 300 UV-VIS, Thermo Fischer Scientific, Madison, WI) operating on the kinetic mode at room temperature (RT). One unit (U) of Myc. tbc H37Ra PGI activity was defined as the amount of enzyme catalysing the reduction of 1 μmol of NADP+ per min in the above conditions.
Protein assays
Protein concentration was measured using the method by Lowry et al.Citation47 with BSA as a standard, or spectrophotometrically at 280 nm.
Bioautographic assay
Pure diluent sample (EtOH:H2O deionised 1:2 v/v) as well as PEP dissolved in that diluent were applied onto a TLC cellulose plate (5 × 20 cm) with an aerosol applicator (Automatic TLC Sampler 4, Camag, Muttenz, Switzerland) under the control of WinCATS-4 software. The plate was left to air dry and then sprayed with Myc. tbc H37Ra PGI enzyme solution (2.5 ml, concentration 6 U/ml). Subsequently, the plate was incubated at RT covered with aluminium foil to protect against plate drying which could adversely affect the reaction of the enzyme with the inhibitor. After 40 min, the plate was sprayed with approx. 3 ml NADP+/NBT/PMS staining solution. The final reagent concentrations in the staining solution were as follows: 200 mM TRIS/HCl buffer (pH 7.6), 1.67 mM d-fructose-6P, 33 mM MgCl2×6H2O, 6.1 mM NADP+, 35 U of glc-6P dehydrogenase, 3.26 mM NBT and 0.78 mM PMS. After application of the solution, the plate covered with aluminium foil was placed at RT for 15 min. Visibly brighter spots against a dark purple background indicated lack of characterized PGI activity.
In order to optimize the staining solution for the detection of Myc. tbc H37Ra PGI activity, TLC bioautography was conducted in parallel on two separate plates using NADP+/NBT/PMS and NAD+/NBT/PMS solutions. The only difference between the compositions of the solutions was the coenzyme used.
In order to confirm the lack of L. mesenteroides glc-6P dehydrogenase inhibition by PEP, additional TLC bioautography was performed. Briefly, diluent sample and PEP samples dissolved in diluent (EtOH:H2O deionised 1:2 v/v) were applied onto a 2.5 × 20 cm TLC cellulose plate. Next the dried TLC plate was sprayed solution with compounds as follows: TRIS/HCl buffer (pH 7.6), d-glucose-6P, MgCl2×6H2O, NADP+, L. mesenteroides glc-6P dehydrogenase, NBT and PMS in a total volume of 1.5 ml.
The results were viewed and documented by means of photography taken under white light with an ATS-4 visualizer (Camag, Muttenz, Switzerland) and processed using the WinCATS-4 software.
Results and discussion
TLC hyphenation with bioautography for the detection of enzyme inhibition is a technique with a great potential for implementation in the light of increasing drug resistance of microorganisms. It is worth noting that there is a vast variety of known microorganism enzymes that could be considered as potential drug targetsCitation48. Thus, there is a significant need to develop new techniques that would allow detection of microbial enzyme inhibition and fast screening of compounds with potential antimicrobial activity, in particular antimycobacterial one.
First of all, during Myc. tbc infection in macrophages, the bacteria harvest approx. 70% of energy from anaerobic glycolysisCitation5,49. From this aspect, PGI, an enzyme essential for the survival of Myc. tbc, may be treated as a potential target of compounds with antituberculosis activityCitation5,7. Secondly, as mentioned in the Introduction section, the characterized biocatalyst is known to be responsible for the formation of arabinogalactan, a key component of mycobacterial cell wall. Considering both above-mentioned reasons, studies regarding the usefulness of the TLC bioautographic method for the determination of PGI inhibition are fully justified.
The main problem with enzyme bioautographic assays is their high sensitivity to organic solvents. In this study, the inhibitory influence of pure compound (PEP) on enzyme activity (PGI from Myc. tbc H37Ra) was analysed. Therefore, an analogous test to TLC-direct bioautography “dot-blot”Citation9 in which compounds to be detected are not separated on the TLC plate was performed.
The best results were obtained when the TLC bioautographic technique was performed on a cellulose plate using NADP+/NBT/PMS staining solution. The lowest amount of PEP inhibitor (μg) that produced visibly brighter zones was 226 μg per zone (detection limit). Thus, PEP inhibited Myc. tbc H37Ra PGI at amount above 226 μg (, lane 4). Noteworthy are the visibly brighter inhibition spots (zones) in a purple background reflecting Myc. tbc H37Ra PGI inhibition by PEP were visible after only 5 min upon the use of NADP+/NBT/PMS staining solution.
Figure 2. Bioautographic thin layer chromatogram showing the inhibition of Mycobacterium tuberculosis H37Ra phosphoglucose isomerase (Myc. tbc H37Ra PGI) activity by phosphoenolpyruvate using NADP+/NBT/PMS staining. Lanes 2, 3, 4, 5, 7, 8, 9 and 10 were spotted with 113, 170, 226, 339, 452, 565, 678 and 904 μg of phosphoenolpyruvate, respectively. Lanes 1 and 6 were spotted with the solvent of phosphoenolpyruvate. The experiment was performed with 15 U of Myc. tbc H37Ra PGI activity.
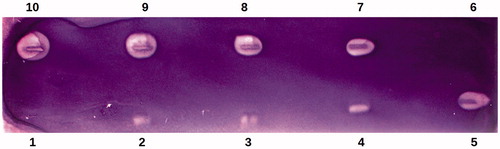
The initial attempts to measure the Myc. tbc H37Ra PGI activity directly on the glass-backed TLC layer of silica gel 60 F254 were unsuccessful (results are not presented). From this aspect, we wanted to check the possibility of using cellulose plates to determine the characterized enzymatic activity. In preliminary studies, we estimated Myc. tbc H37Ra PGI activity in order to obtain the background of cellulose plates with clearly purple colour visible with a naked eye. In our opinion, the activity was approx. 12 U (over a 5 × 20 cm TLC plate), results are not included. It should be mentioned that the activity of the enzyme applied on TLC cellulose plates to optimize the composition of the staining solution and the inhibition of Myc. tbc H37Ra by PEP (Materials and methods, Bioautographic assay) was similar and amounted to 15 U.
In the light of the literature available, glc-6P dehydrogenase from L. mesenteroides was approx. equally effective in using NAD+ and NADP+ as coenzymesCitation50,51. Nevertheless, TLC bioautography experiments employing both compounds were performed. The results of TLC bioautography of Myc. tbc H37Ra PGI with the use of NAD+/NBT/PMS and NADP+/NBT/PMS staining solutions are presented in . It was observed that the purple colour in the background of the cellulose plate was darker after the use of NADP+ as a coenzyme of L. mesenteroides glc-6P dehydrogenase () in comparison with the reaction conducted in the presence of NAD+ (). Thus, NADP+ appeared to be a more suitable component of the staining solution than NAD+ in the TLC bioautographic assay of Myc. tbc H37Ra PGI activity.
Figure 3. Comparison of bioautographic thin layer chromatograms obtained for Mycobacterium tuberculosis H37Ra phosphoglucose isomerase (Myc. tbc H37Ra PGI) with the use of staining solutions containing different coenzymes of the auxiliary enzyme Leuconostoc mesenteroides glucose-6-phosphate dehydrogenase. A – reaction with NAD+; B – reaction with NADP+. The experiments were performed with 15 U of Myc. tbc H37Ra PGI activity. Staining was performed in darkness for 5 min at room temperature.
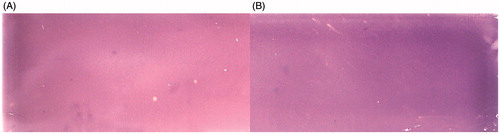
The glc-6P dehydrogenase derived from L. mesenteroides was expected not to be inhibited by PEP. In the literature survey, the presented kinetic results demonstrated that PEP was an allosteric inhibitor of glc-6P dehydrogenases from various species, e.g. Zymomonas mobilisCitation52, but not of that from L. mesenteroidesCitation53,54. However, additional TLC bioautography, in which glucose-6-phosphate was used instead of fructose-6-phosphate, was conducted as described in Materials and methods, bioautography assay, in order to confirm the lack of inhibition of glc-6P dehydrogenase from L. mesenteroides by PEP. This compound did not exhibit any inhibitory activity against glc-6P dehydrogenase (.
Figure 4. Bioautographic thin layer chromatogram showing the lack of inhibition of glucose-6-phosphate dehydrogenase from Leuconostoc mesenteroides by phosphoenolpyruvate using NADP+/NBT/PMS staining. Lane 1 was spotted with the solvent of phosphoenolpyruvate. Lanes 2, 3 and 4 were spotted with 226, 339 and 452 μg of phosphoenolpyruvate, respectively.

To our best knowledge, in contrast to 6-phosphogluconate, which has been listed as one of the most important inhibitors of the PGI activityCitation4,7, the influence of PEP on the activity of mycobacterial PGI had never been examined before. PEP was reported to be an effective inhibitor of PGIs from Bacillus caldotenaxCitation55 and E. coliCitation56 Ogawa et al.Citation56 found that PEP exhibited a competitive type of inhibition of E. coli PGI with respect to fru-6P.
Conclusions
In conclusion, the study resulted in a successful implementation of the TLC bioautographic assay as a simple and effective technique for the detection of inhibition of Myc. tbc H37Ra PGI, a key enzyme in the metabolism of phosphorylated sugars in mycobacteria. However, in order to fully confirm the applicability of this technique, further studies regarding the use of the mobile phase are needed. Nevertheless, the authors believe that this method will be extremely helpful in the identification of novel compounds (e.g. plant-derived) with antimycobacterial activity.
Acknowledgements
The authors would like to thank Dr. Agata Przekora from Department of Biochemistry and Biotechnology, Medical University of Lublin, Poland, for providing valuable suggestions on preparing the manuscript.
Declaration of interest
The authors declare no conflict of interest. Financial assistance was provided by Ministry of Science and Higher Education in Poland within DS2/15 project of Medical University of Lublin. This study was conducted using the equipment purchased within the agreement No. POPW.01.03.00-06-010/09-00 Operational Program Development of Eastern Poland 2007–2013, Priority Axis I, Modern Economy, Operations 1.3. Innovations Promotion.
References
- Meena LS. Rajni. Survival mechanisms of pathogenic Mycobacterium tuberculosis H37Rv. FEBS J 2010;277:2416–27
- World Health Organization. Global tuberculosis report 2014. Geneva, Switzerland; 2014:14
- Davies C, Muirhead H, Chirgwin J. The structure of human phosphoglucose isomerase complexed with a transition-state analogue. Acta Crystallogr D Biol Crystallogr 2003;59:1111–13
- Mathur D, Garg LC. Functional phosphoglucose isomerase from Mycobacterium tuberculosis H37Rv: rapid purification with high yield and purity. Protein Expr Purif 2007;52:373–8
- Anand K, Mathur D, Anant A, Garg LC. Structural studies of phosphoglucose isomerase from Mycobacterium tuberculosis H37Rv. Acta Crystallogr Sect F Struct Biol Cryst Commun 2010;66:490–7
- Tuckman D, Donnelly RJ, Zhao FX, et al. Interruption of the phosphoglucose isomerase gene results in glucose auxotrophy in Mycobacterium smegmatis. J Bacteriol 1997;179:2724–30
- Mathur D, Ahsan Z, Tiwari M, Garg LC. Biochemical characterization of recombinant phosphoglucose isomerase of Mycobacterium tuberculosis. Biochem Biophys Res Commun 2005;337:626–32
- Hasan MR, Rahman M, Jaques S, et al. Glucose 6-phosphate accumulation in Mycobacteria: implications for a novel F420-dependent anti-oxidant defense system. J Biol Chem 2010;285:19135–44
- Choma IM, Jesionek W. TLC-direct bioautography as high throughput method for detection of antimicrobials in plants. Chromatography 2015;2:225–38
- Marston A. Thin-layer chromatography with biological detection in phytochemistry. J Chromatogr A 2011;1218:2676–83
- Dewanjee S, Gangopadhyay M, Bhattabharya N, et al. Bioautography and its scope in the field of natural product chemistry. J Pharm Anal 2015;5:75–84
- Marston A, Kissling J, Hostettmann K. A rapid TLC bioautographic method for the detection of acetylcholinesterase and butyrylcholinesterase inhibitors in plants. Phytochem Anal 2002;13:51–4
- Mroczek T, Mazurek J. Pressurized liquid extraction and anticholinesterase activity-based thin-layer chromatography with bioautography of Amaryllidaceae alkaloids. Anal Chim Acta 2009;633:188–96
- Zheng X-Y, Zhang Z-J, Chou G-X, et al. Acetylcholinesterase inhibitive activity-guided isolation of two new alkaloids from seeds of Peganum nigellastrum Bunge by an in vitro TLC-bioautographic assay. Arch Pharm Res 2009;32:1245–51
- Benamar H, Rached W, Derdour A, Marouf A. Screening of Algerian medicinal plants for acetylcholinesterase inhibitory activity. J Biol Sci 2010;10:1–9
- Wszelaki N, Kociun A, Kiss AK. Screening of traditional European herbal medicines for acetylcholinesterase and butyrylcholinesterase inhibitory activity. Acta Pharm 2010;60:119–28
- Adhami H-R, Farsam H, Krenn L. Screening of medicinal plants from Iranian traditional medicine for acetylcholinesterase inhibition. Phytother Res 2011;25:1148–52
- Bhadra S, Mukherjee PK, Bandyopadhyay A. Cholinesterase inhibition activity of Marsilea quadrifolia Linn. an edible leafy vegetable from West Bengal, India. Nat Prod Res 2012;26:1519–22
- Cabral RS, Sartori MC, Cordeiro I, et al. Anticholinesterase activity evaluation of alkaloids and coumarin from steams of Cochocarpus fontanesianus. Braz J Pharmacogn 2012;22:374–80
- Adhami H-R, Lutz J, Kählig H, et al. Compounds from gum ammoniacum with acetylcholinesterase inhibitory activity. Sci Pharm 2013;81:793–805
- Kongkiatpaiboon S, Rojsanga P, Pattarajinda V, Gritsanapan W. Acetylcholinesterase inhibitory activity of didehydrostemofoline, stemofoline alkaloids and extracts from Stemona collinsiae Craib roots. Pharmacognosy J 2013;5:56–9
- Dalai MK, Bhadra S, Chaudhary SK, et al. Anti-cholinesterase potential of Cinnamomum tamala (Buch.-Ham.) T.Ness & Eberm leaves. Indian J Tradit Know 2014;13:691–7
- Dalai MK, Bhadra S, Chaudhary SK, et al. Anticholinesterase activity of Cinnamomum zeylanicum L. leaf extract. TANG Human Med 2014;4:21–6
- Pandey S, Sree A, Sehti DP, et al. A marine sponge associated strain of Bacillus subtilis and other marine bacteria can produce anticholinesterase compounds. Microb Cell Fact 2014;13:24
- Silva NNS, Silva JRA, Alves CN, et al. Acetylcholinesterase inhibitory activity and molecular docking study of 1-nitro-2-phenylethane, the main constituent of Aniba canelilla essential oil. Chem Biol Drug Des 2014;84:192–8
- Ramallo A, Salazar MO, Furlan RLE. Thin layer chromatography-autography-high resolution mass spectrometry analysis: accelerating the identification of acetylcholinesterase inhibitors. Phytochem Anal 2015;26:404–12
- Wszelaki N, Paradowska K, Jamróz MK, et al. Bioactivity-guided fractionation for the butyrylcholinesterase inhibitory activity of furanocoumarins from Angelica archangelica L. roots and fruits. J Agric Food Chem 2011;59:9186–93
- Gu LH, Liao LP, Hu HJ, et al. A thin-layer chromatography-bioautographic method for detecting dipeptidyl peptidase IV inhibitors in plants. J Chromatogr A 2015;1411:116–22
- Camara MA, Tian M, Yang L, Wang S. Application of thin-layer chromatography in enzyme activity and inhibitors studies of glucose-6-phosphate dehydrogenase. J Planar Chromatogr-Modern TLC 2015;28:326–33
- Salazar MO, Furlan RLE. A rapid TLC autographic method for the detection of glucosidase inhibitors. Phytochem Anal 2007;18:209–12
- Simões-Pires CA, Hmicha B, Marston A, Hostettmann K. A TLC bioautographic method for the detection of α- and β-glucosidase inhibitors in plant extracts. Phytochem Anal 2009;20:511–15
- Verma N, Behera BC, Sharma BO. Glucosidase inhibitory and radical scavenging properties of Lichen metabolites salazinic acid, sekikkaic acid and usnic acid. Hacettepe J Biol Chem 2012;40:7–21
- Pandey S, Sree A, Dash SS, Sehti DP. A novel method for screening beta-glucosidase inhibitors. BMC Microbiol 2013;13:55
- Yang Y, Gu L, Xiao Y, et al. Rapid identification of α-glucosidase inhibitors from Phlomis tuberosa by Sepbox chromatography and thin-layer chromatography bioautography. PLoS One 2015;10:e0116922
- Hassan AMS. TLC bioautographic method for detecting lipase inhibitors. Phytochem Anal 2012;23:405–7
- Bayineni VK, Suresh S, Singh G, Kadappagari R-K. Development of a bioautographic method for the detection of lipase inhibitors. Biochem Biophys Res Commun 2014;453:784–6
- Tang J, Zhou J, Tang Q, et al. A new TLC bioautographic assay for qualitative and quantitative estimation of lipase inhibitors. Phytochem Anal 2016;27:5–12
- Liang JB, Yang ZD, Shu ZM, Yu CC. A rapid thin-layer chromatography bioautographic method for detecting the monoamine oxidase inhibitors in plants. Nat Prod Res 2014;28:1318–21
- Zarmouh NO, Mazzio EA, Elshami FM, et al. Evaluation of the inhibitory effects of bavachinin and bavachin on human monoamine oxidases A and B. Evid Based Complement Alternat Med 2015;2015:852194. doi:10.1155/2015/852194
- Patil A, Patil S, Mahure S, Kale A. UV, FTIR, HPLC confirmation of camptothecin an anticancer metabolite from bark extract of Nothapodytes nimmoniana (J. Graham). Am J Ethnomed 2014;1:174–85
- Wangthong S, Tonsiripakdee J, Monhaphol T, et al. Post TLC developing technique for tyrosinase inhibitor detection. Biomed Chromatogr 2007;21:94–100
- Kamagaju L, Morandini R, Bizuru E, et al. Tyrosinase modulation by five Rwandese herbal medicines traditionally used for skin treatment. J Ethnopharmacol 2013;146:824–34
- García P, Furlan RLE. Multiresponse optimisation applied to the development of a TLC autography for the detection of tyrosinase inhibitors. Phytochem Anal 2015;26:287–92
- Ramallo A, Zacchino SA, Furlan RLE. A rapid TLC autographic method for the detection of xanthine oxidase inhibitors and superoxide scavengers. Phytochem Anal 2006;17:15–19
- Paradowska K, Lutek J, Ginalska G. A rapid detection for the inhibition of phosphoglucose isomerase from Escherichia coli by mercury(II) chloride based on TLC-autographic analysis – preliminary studies. Curr Issues Pharm Med Sci 2014;27:127–30
- Fraenkel DG, Levisohn SR. Glucose and gluconate metabolism in an Escherichia coli mutant lacking phosphoglucose isomerase. J Bacteriol 1967;93:1571–8
- Lowry OH, Rosebrough NR, Farr AL, Randall RJ. Protein measurement with the Folin phenol reagent. J Biol Chem 1951;193:265–75
- Amir A, Rana K, Arya A, et al. Mycobacterium tuberculosis H37Rv: in silico drug targets identification by metabolic pathways analysis. Int J Evol Biol 2014;2014:284170. doi:10.1155/2014/284170
- Singhal N, Sharma P, Kumar M, et al. Analysis of intracellular expressed proteins of Mycobacterium tuberculosis clinical isolates. Proteome Sci 2012;10:14
- Olive C, Geroch ME, Levy HR. Glucose 6-phosphate dehydrogenase from Leuconostoc mesenteroides. J Biol Chem 1971;246:2047–57
- Singh S, Anand A, Srivastava PK. Regulation and properties of glucose-6-phosphate dehydrogenase: a review. Int J Plant Physiol Biochem 2012;4:1–19
- Scopes RK. Allosteric control of Zymomonas mobilis glucose-6-phosphate dehydrogenase by phosphoenolpyruvate. Biochem J 1997;326:731–5
- Anderson BM, Wise DJ, Anderson CD. Azotobacter vinelandii glucose-6-phosphate dehydrogenase properties of NAD- and NADP-linked reactions. Biochim Biophys Acta 1997;1340:268–76
- Asensio C, Levoin N, Guillaume C, et al. Irreversible inhibition of glucose-6-phosphate dehydrogenase by the coenzyme A conjugate of ketoprofen: a key to oxidative stress induced by non-steroidal anti-inflammatory drugs? Biochem Pharmacol 2007;73:405–16
- Takama M, Nosoh Y. Effect of ATP on glucose-6-phosphate isomerase from Bacillus caldotenax. Biochim Biophys Acta 1982;705:127–30
- Ogawa T, Mori H, Tomita M, Yoshino M. Inhibitory effect of phosphoenolpyruvate on glycolytic enzymes in Escherichia coli. Res Microbiol 2007;158:159–63