Abstract
Xylarinase is a bi-functional fibrinolytic metalloprotease isolated from the culture filtrate of endophytic fungus Xylaria curta which is monomeric with a molecular mass of ∼33.76 kDa. The enzyme displayed both plasmin and tissue plasminogen activator like activity under in vitro conditions. It hydrolyses Aα and Bβ chains of the fibrinogen. Optimal fibrinolytic activity of xylarinase is observed at 35 °C, pH 8. Ca2+ stimulated the fibrinolytic activity of xylarinase while Fe2+ and Zn2+ inhibited suggesting it to be a metalloprotease. The Km and Vmax values of xylarinase were 240.9 μM and 1.10 U/ml for fibrinogen and 246 μM and 1.22 U/ml for fibrin, respectively. Xylarinase was found to prolong the activated partial thromboplastin time and prothrombin time. The N-terminal sequence of xylarinase (SNGPLPGGVVWAG) did not show any homology with previously known fibrinolytic enzymes. Thus xylarinase is a novel fibrinolytic metalloprotease which could be possibly used as a new clot busting enzyme.
Introduction
Cardiovascular disorders are a major health threat across the globe and are responsible for over 18 million deaths annually throughout the worldCitation1–3. Heart related diseases such as myocardial infarctionCitation4, high blood pressure; ischemic heart diseasesCitation5 are primarily caused due to accumulation of fibrin in the walls of the blood vessels resulting in the formation of a haemostatic plug or a clotCitation6. In such conditions, drugs like urokinase and streptokinase are administrated intravenously to dissolve the fibrin clotsCitation7. For thrombolytic therapy, plasminogen activators (PA) or tissue plasminogen activators (tPA) are considered as gold standardCitation8. However, these agents are reported to have been associated with some undesirable effects such as gastrointestinal bleeding, immunogenicity, short-half life, thermolabile nature and their cost intensivenessCitation9,Citation10. Such concerns call for an urgent need to explore new sources of fibrinolytic agents which could have better efficacy, specificity and fewer side effects.
Fibrinolytic enzymes have been successfully discovered from various plants, animal and microbial sources including bacteria, actinomycetes, fungi, algae, snake venom, vampire bat saliva and earth wormsCitation11. Microbes are the most preferred source of such enzymes due to their diversity, feasibility in mass culture and ease in genetic manipulationCitation1. The most commonly used thrombolytic agent “Streptokinase” is produced by several stains of β-hemolytic Streptococci of Lancefield group A, C and GCitation12,Citation13. Apart from bacterial sources, fungal proteases possessing fibrinolytic activity have also been reported from Aspergillus ochraceus, Cochliobolus lunatus, Fusarium spp., Penicillum chrysogenum and Rhizopus chinensisCitation14,Citation15.
Endophytic fungi are a special class of fungi which reside within the host plant without exhibiting any sign of their presence or existenceCitation16. Endophytes are known to increase the overall fitness of the plant by providing abiotic and biotic stress tolerance, decreasing water consumption and defending from pathogenic microorganismsCitation17–19. Recently endophytic fungi have been used as fountainheads for the discovery of new bioactive compounds possessing anti-cancer, anti-microbial properties and resource of putative phytomedicinals. However, endophytic fungi have also been sparsely studied for their fibrinolytic potential. Only a few endophytic fungi such as Clonostachys sp., Cladosporium sp., Fusarium strain CPCC 480097, Fusarium sp. BLB and Verticillium sp., have been reported to possess fibrinolytic activityCitation20–22. Hence, endophytic fungi stand as an unexplored bioresource of fibrinolytic agents that can play an important role in thrombolytic therapy.
In the present study, a putative metalloprotease “xylarinase” was purified from the culture broth of endophytic Xylaria curta species and was characterised in terms of its fibrinolytic activity, fibrinogenolysis pattern, thrombolytic effect and other biochemical properties. We found that the enzyme could degrade artificial fibrin clots with or without the presence of plasminogen. The enzyme selectively degraded the Aα and Bβ chains of fibrin. The fibrinolytic activity of xylarinase was stimulated in the presence of Ca2+ and inhibited in the presence of Zn2+, Fe2+, Cu2+ and metal-ion chelators such as EDTA and EGTA. Our data implies that xylarinase is a bi-functional metalloprotease capable of degrading fibrin clots. Further, this is the first report of an endophytic Xylaria sp. exhibiting a fibrinolytic activity.
Materials and methods
Isolation of endophytic fungus
Healthy twigs of Cathranthus roseus were collected from the rain forest area of the BRT Wildlife Sanctuary, Karnataka, India. The plant twigs were thoroughly washed under running tap water for 15 min, surface sterilized with 3% sodium hypochlorite for 10 min followed by 70% ethanol for 2 min and 30% ethanol for 1 min and finally allowed to surface dry under sterile conditions. The surface sterilized samples were then cut into 1–2 mm segments with the help of sterile blade and placed on water agar plates with ventral side facing the medium. The plates were then incubated at 26 ± 1 °C for 10 days with 12 h light/dark cycles. After 10 days, individual colonies were picked from the edge of an advancing colony with a sterile fine needle under stereo-zoom microscope (Nikon) and transferred onto Potato Dextrose Agar (PDA). The pure endophytic fungal isolates so obtained were stored on rye grain and PDA slants supplemented with 10% glycerolCitation23.
Culture condition for enzyme production
Endophytic fungus was subjected for production of enzyme extract in a chemically defined medium. Briefly, the method comprised of inoculation of pre-sterilised Czapek Dox broth (CDB, HiMedia, Mumbai, India) aseptically with 5 mm mycelial disc of seven-day old culture of the endophytic fungus followed by incubation at 28 ± 1 °C, 130 rpm. After completion of the incubation period, the mycelial mass was separated using Whatmann filter paper no. 4 followed by centrifugation at 8000 rpm for 10 min at room temperature (Hitachi RX II series, Tokyo, Japan). The supernatant was then passed through a 0.22 μm nitrocellulose membrane to obtain cell free culture brothCitation24.
Enzyme purification
All the steps were carried out at 4 °C otherwise mentioned. Solid ammonium sulphate was slowly added to 10 l of the culture filtrate to make 60% saturation and stored overnight. Precipitate was collected by centrifugation at 12 500 rpm for 15 min (Hitachi RX II series, Tokyo, Japan). The pellet was dissolved in minimum volume of 20 mM Tris–HCl buffer (pH 7.8) and the solution was dialysed against the same buffer overnight at 4 °C. The protein solution was loaded onto a column containing Q-sepharose anion exchange resin (Sigma Aldrich, St. Louis, MO) with a bed volume of 3 cm equilibrated with 20 mM Tris–HCl, pH 7.8. The bound proteins were eluted with sodium chloride (NaCl) solution in a linear gradient of 0–0.5 mM. The fractions were pooled and analysed by sodium dodecyl sulphate-polyacrylamide gel electrophoresis (SDS-PAGE). The protein fractions were further purified by medium pressure liquid chromatography (AKTA Prime, GE) using Sephacryl S-300 column (72 cm column length, 1.5 cm internal diameter, 60 cm gel bed volume) with a flow rate of 1 ml/min, pressure 1 MPa and fraction size of 2 ml. Protein content was analysed at 280 nm. Protein fractions having fibrinolytic activity were pooled, concentrated and used as pure enzyme. The homogeneity of the pure enzyme was confirmed by SDS-PAGECitation9,Citation25,Citation26.
Determination of protein content
Bradford’s method was used to determine the concentration of protein, using BSA as a standardCitation27.
SDS-PAGE and fibrin zymography
The molecular weight of xylarinase was determined using 10% SDS-PAGE performed by following Laemmli’s methodCitation28. The resolved protein was detected in the gel by modified silver staining methodCitation29. The molecular mass of the denatured enzymes was estimated using a standard protein weight marker (Merck Millipore, Billerica, MA). The purity of the fractions obtained through gel filtration chromatography was analysed using High Performance liquid chromatography (Shimadzu LC System, Kyoto, Japan) equipped with C18 column. The mobile phase was water and acetonitrile in the ratio 1:1. The purity was authenticated at 250 nmCitation30.
Fibrin zymography was carried out following a modified procedure described by Kim et al.Citation31. A separating gel (10% w/v) supplemented with 0.12% fibrinogen (w/v) solution and 12 μl of thrombin (100 NIH U/ml) was prepared. The purified enzyme (20 μl) was electrophoresed into fibrin-copolymerized gel at a 20 mA constant current at 4 °C. After electrophoresis, the gel was soaked in 2.5% Triton X-100 containing Tris–HCl (20 mM) buffer (pH 7.8) for 30 min at room temperature to renature the protein. After washing the gel in distilled water for 30 min, the gel was incubated in 20 mM Tris–HCl buffer (pH 7.8) containing 200 mM NaCl, 10 mM CaCl2, and 0.02% NaN3 at 37 °C for overnight. The gel was stained with Coomassie brilliant blue R-250 for 2 h and then destained.
MALDI-TOF
The actual molecular weight of xylarinase was determined by Matrix-assisted Laser desorption/ionization-time of flight (MALDI-TOF) mass spectrometry using an AB SCIEX TOF/TOF™ 5800 system and molecular mass was calculated using MASCOT and Protein Pilot softwareCitation32.
Fibrinolytic activity of xylarinase
Fibrinolytic activity of xylarinase was determined by following a modified procedure described by Astrup and MullertzCitation33 using both plasminogen rich and plasminogen free plates. Briefly, plasminogen free plates were prepared by mixing 5 ml of 0.5% w/v fibrinogen (Calbiochem, Darmstadt, Germany) in 20 mM Tris–HCl buffer (pH 7.8) with 1% agarose and 100 μl of thrombin (100 NIH U/ml, Sigma Aldrich). Plasminogen rich plates were supplemented with 5 U of plasminogen (Calbiochem, Darmstadt, Germany). Plates were allowed to stand for 30 min at room temperature to form fibrin clot. Subsequently, 20 μl of the enzyme was dispensed into 5 mm wells previously made with sterile cork borer. Plates were incubated at 37 °C for 24 h. After incubation, the dimension of the clear zone was measured and number of units was determined against a calibration curve of plasmin (from human plasma, Calbiochem, Darmstadt, Germany)Citation9,Citation22.
Fibrinogenolytic activity
Fibrinogenolytic activity was determined by modified method of Matsubara et al.Citation34. Briefly, 250 μl of 1% fibrinogen in 20 mM Tris–HCl buffer (pH 7.8) was incubated with 50 μl (1 mg/ml) of purified enzyme at 37 °C. At different time intervals, 20 μl fraction was withdrawn and analysed by SDS-PAGE-commassie stainingCitation9,Citation35.
Effect of pH and temperature on fibrinolytic activity of xylarinase
The optimal pH for xylarinase activity was determined within a pH range of 4.0–9.0. The purified enzyme (1 mg/ml,10 μl) was added to 90 μl of 20 mM glycine HCl (pH 4), sodium citrate (pH 5), sodium phosphate (pH 6 and pH 7), Tris–HCl (pH 8) and glycine NaOH (pH 9) buffer system. The master mixtures were incubated for 1 h and the enzyme activities were measured by fibrin plate assay as described earlier. The pH stability was determined by incubating the purified enzyme in the respective buffer for overnight at 4 °CCitation26,Citation35.
The optimal temperature for xylarinase activity was determined by measuring the residual activity after incubating the enzyme (1 mg/ml, 10 μl) with 90 μl of 20 mM Tris–HCl buffer at different temperatures from 25 to 70 °C for 1 h. The residual fibrinolytic activity was assessed by fibrin plate methodCitation26,Citation35.
Effect of metal ions on fibrinolytic activity of xylarinase
Effect of metal ions on xylarinase activity was evaluated using CaCl2, MgCl2, KCl, CoCl2, ZnCl2, MnCl2, CuCl2, FeCl3, AlCl3 and NaCl. The purified enzyme (1 mg/ml, 10 μl) was incubated in presence and absence of cations such as Ca+, Mg+, Co+, Cu+, Al+, Mn+, Zn+, Fe+ and Na+ with a final concentration of 2 mM in Tris–HCl buffer for 1 h at 37 °C. The effect of metal ions on xylarinase activity was then analysed by fibrin plate assays as described earlier and was expressed as relative fibrinolytic activityCitation25,Citation35.
Effect of inhibitors on fibrinolytic activity of xylarinase
The nature of the enzyme was determined by assessing the effect of inhibitors like phenylmethylsulfonyl fluoride (PMSF), tosyl-phenylalanyl-chloromethyl ketone (TPCK), ethylenediaminetetraacetic acid (EDTA), ethyleneglycolbis-(2-aminoethyl)-N′, N′, N′, N′-tetraacetic acid (EGTA), leupeptin, aprotinin and β-mercaptoethanol over xylarinase. The purified enzyme (1 mg/ml, 10 μl) was incubated with 2 mM of the inhibitors for 1 h at 37 °C. The residual fibrinolytic activity was analysed by fibrin plate assay and expressed in terms of relative fibrinolytic activityCitation25,Citation35.
Determination of N-terminal sequence
To determine the N-terminal amino acid sequence, the purified enzyme was first subjected to SDS-PAGE and then transferred onto polyvinylidene difluoride (PVDF) membrane (Merck Millipore, Billerica, MA) at 350–400 mA for 35 min. The desired band was excised from the membrane and N-terminal amino acid sequence was determined using Applied Biosystems PROCISE 491 cLC protein sequencer at Institute of Microbial Technology (IMTECH), Chandigarh, India. The sequence homology prediction of the purified enzyme with other proteases was performed using NCBI “Blastp” search programCitation9,Citation25,Citation30.
Fibrinogen clotting time assay
Fibrinogen clotting time assay was performed according to the method described by Wu et al.Citation36 with minor modifications. First, xylarinase (1 mg/ml) was incubated with 50 μl of 1% fibrinogen (20 mM Tris–HCl, pH 7.8) for 5 min at 37 °C. Subsequently 30 μl of 5 U/ml of thrombin was added and clotting time was determined (a). Second, xylarinase was again incubated with 100 μl and 150 μl of 1% fibrinogen (20 mM Tris–HCl, pH 7.8) for 5 min at 37 °C, respectively (b). Subsequently 30 μl of 5 U/ml of thrombin was added and clotting time was determined. Finally, xylarinase was incubated with 50 μl of 1% fibrinogen (20 mM Tris–HCl, pH 7.8) for 5 min at 37 °C. Subsequently 60 and 90 μl of 5 U/ml of thrombin was respectively added and clotting time was determined.
Determination of kinetic constant of xylarinase
The kinetic constants Km and Vmax of xylarinase were calculated by fitting the calculated enzyme activities at different fibrin concentration (2–10 mg/ml) on Lineweaver–Burk plot. Plasmin (Calbiochem, Darmstadt, Germany) and Streptokinase (Sigma Aldrich, St. Louis, MO) were used as standards. In case of streptokinase, the fibrin was supplemented with 5 U of plasminogen. One unit of enzyme activity was defined as the amount of enzyme causing conversion of 1 μM of substrate per minute per milligram of protein at 37 °CCitation37. In addition, the Km and Vmax of xylarinase were also determined for fibrinogen.
In vitro thrombolytic activity assay
To assess the in vitro thrombolytic activity of xylarinase, fresh human blood was collected from a healthy volunteer. About 500 μl of the blood was dispensed in a microcentrifuge tube and allowed to coagulate at 37 °C for 1 h after which it was rinsed, weighed and then dipped in different concentrations of xylarinase (10–50 μg). Physiological saline was used as negative control whereas plasmin served as positive control. After 1 h, the residual thrombus was weighed and the dissolution ratio of the thrombus was calculatedCitation12,Citation36.
In vitro anticoagulant activity assay
Blood sample was collected from a healthy volunteer in 3.2% sodium citrate solution (1:9 v/v) and platelet poor plasma was obtained by centrifugation at 2000 g for 10 min. The effect of xylarinase on activated partial thromboplastin time (APTT), prothrombin time (PT) and thrombin time (TT) was performed according to the manufacturer’s instructions by using an automated coagulometer (Chem 7, Erba Mannheim, Germany) with reagents from Tulip Diagnostics Enterprises, Goa, India. Platelet poor plasma without addition of xylarinase was used as controlCitation32.
ITS-rDNA based molecular taxonomy
For genomic DNA extraction, about 0.1–0.2 g of cultured mycelia were scrapped off from 3 to 4 days old culture with sterile inoculation loop and crushed to very fine powder in a pestle and mortar under liquid nitrogen. Further DNA extraction was done by using the Wizard® Genomic DNA purification kit (Promega, Madison, WI) as per manufacturer’s instructions. The Internal Transcribed Spacer (ITS) region 1, 5.8S and 2 amplified using ITS 1 and ITS 4 primersCitation38. Twenty five microliters of the reaction mixture containing 1 μl of extracted genomic DNA, 10 μM of each primer, 2.5 mM of dNTP, 1.5 U of Taq DNA Polymerase in 10X Taq buffer containing 25 mM MgCl2 (Bangalore GeNei, Bengaluru, India). The conditions for the polymerase chain reaction were 96 °C for 5 min followed by 39 cycles of 95 °C for 45 s, 60 °C for 45 s, 72 °C for 45 s followed by final extension at 72 °C for 5 minCitation39. The ITS amplicons were examined using a 1.5% agarose gel under UV light in Bio-Rad Gel documentation System using Quantity-1-D analysis software (Bio-Rad, Hercules, CA). The PCR products were purified with Wizard® SV gel and PCR clean up system (Promega, Madison, WI) as per manufacturer’s instructions. The obtained PCR products were sequenced at Xcelris Labs (Ahmedabad, Gujarat, India). The final sequence was obtained by assembling the sequences using Sequencher ver. 5 (www.genecodes.com) and submitted to the GenBank under the accession number KM880132.
Sequence similarity search of the ITS sequences of #37 CRSTBRT was performed using the BLAST algorithm tool of NCBI. The ITS sequences were aligned with selected sequences of reference taxa obtained from BLAST using the Clustal W option in MEGA 5. The alignment file involved sequences which comprised of one sequence under study, 13 sequences from BLAST search which are representative sequences of respective genera/species and one Fusarium incarnatum species which served as an outgroup to root the tree. The aligned sequences were then trimmed so as to make alignment uniform and the aligned files were then saved in FASTA and MEGA format. The evolutionary relationship was inferred by employing Neighbor-Joining method using Maximum Composite Likelihood methodCitation40. Thousand bootstraps were used to assess the evolutionary history. Gaps were considered as missing data.
Results
Isolation of the endophytic fungi
In the current study, 18 endophytic fungi were isolated from the stem tissue of C. roseus plant. The isolated endophytic fungi were screened for their fibrinolytic potential. In the screening study (data not shown), strain #37 CRSTBRT showed highest fibrinolytic activity and hence was chosen for further characterization.
Enzyme purification
Xylarinase is a new fibrinolytic enzyme isolated and purified from the culture broth of endophytic fungus #37 CRSTBRT by combination of two chromatographic techniques i.e. ion exchange chromatography with Q-sepharose and gel filtration chromatography with Sephacryl S-300 columns. As summarised in , the culture broth containing 9004.73 mg of protein showed a specific activity of 3.99 U/mg. After final purification, 0.93 mg of the protein was recovered from 10 l of the culture broth with a recovery of 0.095%. The purified protein exhibited a specific activity of 36.67 U/mg representing a 9.19-fold increase in the fibrinolytic activity over the native culture broth (and .
Table 1. Purification summary of xylarinase from Xylaria curta.
Molecular weight determination by MALDI-TOF, SDS-PAGE and fibrin zymography
The purified enzyme was monomeric in nature and exhibited a single band in ∼33 kDa region (). The purified enzyme also exhibited strong fibrinolytic activity in similar region molecular mass over fibrin zymography gel. The MALDI-TOF analysis predicted the actual molecular mass of the xylarinase to be 33.76 kDa with 98% purity ().
Figure 2. Determination of homogeneity, molecular mass and fibrinolytic activity of xylarinase. (a) SDS-PAGE analysis of purified xylarinase. Xylarinase resolved from MPLC, Sephacryl S-300 column was electrophoresed on 10% SDS gel. The gels were stained using silver staining method. (b) Determination of molecular weight by MALDI-TOF method.
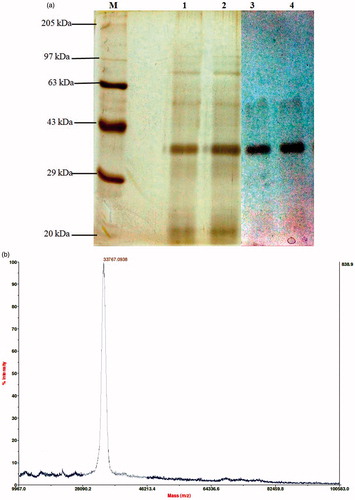
N-terminal amino acid sequence
The N-terminal sequence of the purified fibrinolytic enzyme was determined by automated Edman degradation method. The first 13 amino acid residues of xylarinase were determined as SNGPLPGGVVWAG (). No putative conserved domains were detected in the NCBI database. However, this N-terminal sequence showed 100% identity with proteases and peptidases from various Streptomyces species. The N-terminal sequence of xylarinase showed differences from previously reported fibrinolytic enzymes from fungi. These results suggest that xylarinase could be a distinct protease.
Table 2. N-terminal acid sequence of xylarinase and alignment with other enzymes.
Table 3. Effect of xylarinase on fibrinogen clotting time.
Table 4. Effect of xylarinase on coagulation parameters.
Fibrinolytic and fibrinogenolytic activity
To determine the fibrin hydrolysing potential of xylarinase, both plasminogen rich and plasminogen free fibrin plate method was used. As demonstrated in , xylarinase produced clear zone in plasminogen free fibrin plates and the size of zone was larger than that of plasmin whereas clear zone formation in plasminogen rich plate was enhanced. This shows that xylarinase has dual property which can directly cleave fibrin or activate plasminogen to do so. Xylarinase produces similar clear zone as that of plasmin which could be due to similar cleaving pattern of fibrin chain.
Figure 3. Fibrinolytic activity of xylarinase on plasminogen deficient plate by fibrin plate method. Fibrin plate was prepared by mixing fibrinogen with thrombin and agarose. Twenty microliters of xylarinase was dispensed into wells and incubated for 12 h at 37 °C.
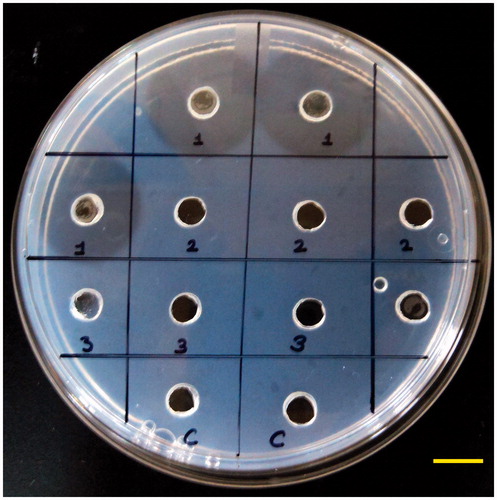
The obtained fibrinogen degradation pattern reveals that xylarinase rapidly hydrolyses Aα chain of fibrin in about 5 min followed by Bβ chain in ∼10 min, however, the enzyme could not display any detectable activity against the γ chain of fibrinogen over a period of 24 h (. This suggests the xylarinase mode of action to be similar to that of α and β fibrinogenase in selectively cleaving the Aα and Bβ chain of fibrinogen rather than γ chains.
Effect of pH and temperature on fibrinolytic activity of xylarinase
Xylarinase exhibited fibrinolytic activity in the pH range 5.0–9.0 with maximum activity at pH 8. The enzyme showed decreased activity in acidic and basic environment. However, the activity of the enzyme was found to be stable in the pH range of 7.0–8.0. The optimum pH for xylarinase is shown in . The optimum temperature for xylarinase activity was 35 °C. The enzyme was relatively stable till 40 °C. However, above 40 °C the activity of the enzyme was sharply reduced ()
Effect of metal ions and protease inhibitors on fibrinolytic activity
The effect of metal ions on fibrinolytic activity of xylarinase was examined in the presence and absence of metal ions for 1 h at 37 °C. The fibrinolytic activity of xylarinase decreased in the presence of Cu2+ and Mn2+ ions whereas it was completely inhibited by Zn2+ and Fe2+ ions. Interestingly, the fibrinolytic activity of xylarinase was increased in the presence of Ca2+ ions ().
Figure 6. Effect of protease inhibitors (2 mM) (a) and metal ions (2 mM) (b) on xylarinase. The fibrinolytic activity in absence of protease inhibitors and metal ions were regarded as 100%. Each value represents the mean ± SD for three determinations.
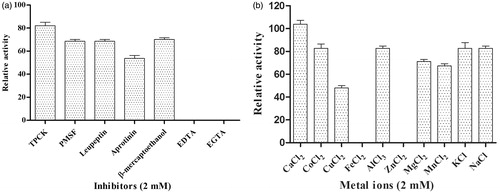
To determine the class of xylarinase, inhibition assay was carried out with different class of protease inhibitors such as PMSF, TPCK, EDTA, EGTA, leupeptin, aprotinin and β-mercaptoethanol. As shown in , the relative activity of xylarinase was completely suppressed by metalloprotease inhibitors like EDTA and EGTA. On the other hand, the fibrinolytic activity was not greatly affected by PMSF, TPCK and leupeptin, hence, indicating that xylarinase could be a metalloprotease.
Fibrinogen clotting time assay
The fibrinogen clotting time assay was performed to check whether xylarinase inhibited the conversion of fibrinogen to fibrin. Delay in clotting time suggests two possible action sites for xylarinase fibrinogen and thrombin. As shown in , compared with the control, xylarinase significantly extends the clotting time and no coagulation was observed till 5 min using 1 mg/ml xylarinase. Augmentation with thrombin showed no effect on clotting time. However, addition of fibrinogen shows a prominent effect on the prolongation of clotting time and thus clotting time was extended to 5 min. These results implied that xylarinase could inhibit fibrin formation and its probable action site is fibrinogen.
Kinetic constant of xylarinase
As shown in , the Michaelis constant (Km) and the maximum reaction velocity (Vmax) values of xylarinase towards fibrin were determined as 246 μM and 1.22 U/ml, respectively. Similarly, the Km and Vmax values of plasmin and streptokinase were found to be 282 μM and 0.13 U/ml and 298.2 μM and 0.15 U/ml, respectively. Moreover, the Km and Vmax values of xylarinase for fibrinogen were found to be 240.9 μM and 1.10 U/ml.
In vitro thrombolytic activity of xylarinase
The in vitro thrombolytic activity of xylarinase was observed in the microfuge tube by spreading the thrombus in the solution after 1 h (. Addition of xylarinase resulted in dose dependent dissolution of the thrombus. Fifty micrograms of xylarinase completely dissolved the thrombus whereas similar concentration of plasmin resulted in 84% hydrolysis of the thrombus. These results showed that xylarinase possesses better fibrinolytic efficacy than plasmin under tested conditions.
In vitro anticoagulant activity assay
In order to determine the effect of xylarinase on coagulation parameters such as APTT, PT and TT were measured in platelet poor plasma collected from human blood. As shown in Table 4, xylarinase strongly prolonged the APTT. Addition of 20 μg of xylarinase resulted in substantial elongation of APTT over 180 s. Further, xylarinase induced a dose-dependent prolongation of PT. However, xylarinase does not have significant effect on TT.
Identification of endophytic fungi, #37 CRSTBRT
The phylogenetic relationship inferred from ITS1-5.8S-ITS2 region sequences of xylaria species is shown in . The phylogenetic tree is resolved into two major clades – Clade I and Clade II. In clade I, seven strains of Xylaria curta were clustered including the isolate under study (#37 CRSTBRT). The isolate under study (#37 CRSTBRT) showed closed relatedness with X. curta ST2382 strain with 98% bootstrap support value thereby confirming its identity as a Xylaria curta species. In clade II, three strains of Xylaria laevis were clustered with 98% bootstrap support value whereas Xylaria montagnei, Xylaria apiculata and Xylaria polymorpha emerged basal to clade II with 95%, 100% and 79% bootstrap support values, respectively. Fusarium incarnatum was used as an outgroup to root the tree (.
Discussion
The current armamentarium of thrombolytic drugs comprises of PAs or t-PA which are considered as one of the most effective agents in thrombolytic therapyCitation9,Citation35. However, these agents suffer from major drawbacks like short half-life, internal bleeding and cost effectiveness, therefore studies are now oriented towards safe and site specific thrombolytic strategiesCitation26,Citation36. Fibrinolytic agents that can directly act on fibrin represent a viable alternative to the present arsenal of thrombolytic drugs. These agents have gained much attention in the recent time due to their impressive thrombolytic efficacy and safety from bleedingCitation41. Fibrinolytic agents with dual activity could be a potential candidate for thrombolytic therapy. In the current study, we have described isolation, purification and biochemical characterisation of a 33.76 kDa bi-functional metalloprotease enzyme designated as “xylarinase” produced by endophytic fungi, Xylaria curta species.
Xylarinase can independently hydrolyse fibrin imitating plasmin activity. Plasmin has high affinity towards fibrin and fibrin zymography is considered as one of the most sensitive and reliable technique to identify plasmin like enzyme. Therefore, we subjected xylarinase to fibrin zymography and it produced a clear halo in the fibrin polymerised gel at ∼33 kDa indicating that xylarinase possesses plasmin like activity. The actual molecular mass of xylarinase analysed by MALDI-TOF was calculated to be 33.76 kDa. The molecular mass of xylarinase is much closer to that of Cordyceps militaris; Fusarium sp. BLB, Fusarium sp. CPCC 480097 (27 kDa), Verticase (31 kDa)Citation20–22,26. The mechanism of fibrinolysis was investigated by examining the fibrinolytic activity on plasminogen free and rich plates. Xylarinase exhibited fibrinolytic activity on both plasminogen rich and plasminogen deficient plate indicating that xylarinase can directly hydrolyse fibrin clots or can activate plasminogen like streptokinase, urokinase and tPA. Only few microbial fibrinolytic agents like herinase, ulvease and UFEII have been reported to possess such bi-functionalityCitation9,Citation30,Citation32. Bi-functionality is the distinctive characteristic of xylarinase which makes it better than other fibrinolytic agents.
Another striking feature of xylarinase is that it can hydrolyse both fibrin and its precursor. The fibrinogenolytic pattern of xylarinase differs from other fibrinolytic enzymes because it hydrolysis Aα and Bβ chain of fibrinogen. Based on its cleavage pattern xylarinase could be αβ fibrinogenase. Fibrinolytic enzymes from Plueurotous eryngii, Paecilomyces tenuipes, Ulva pertusa (ulvease), Bacillus subtilis DC 33 and TP-6 (TPAse) digested both Aα and Bβ chain of fibrinogen. TPAse digested Aα and Bβ chains of fibrinogen to generate D′ and E′ fragments which is similar to that generated by plasminCitation10,32,42–44. Fibrinolytic enzymes from Streptomyces sp. CS684 and C. militaris only digested Bβ chain whereas Streptomyces sp. CS624 and XZNUM 0004 digested Aα, Bβ and γ chain of fibrinogenCitation26,Citation35,Citation45.
The N-terminal sequence of xylarinase revealed that it is a novel protease. Xylarinase shares high sequence similarities with protease and peptidases of various Streptomyces sp. but has no or less similarities in the N-terminal sequence of the previously reported fibrinolytic enzymes. Furthermore, the novelty of xylarinase lies in the dependence of fibrinolytic activity on protease inhibitors and metal ions. To clarify its biochemical nature, fibrinolytic activity of xylarinase was tested in presence and absence of protease inhibitors and metal ions. The fibrinolytic activity of xylarinase was further completely inhibited by EDTA and EGTA. Xylarinase also showed Ca2+-dependence in terms of its fibrinolytic activity and a relative resistance toward protease inhibitors such as PMSF, TPCK and leupeptin. This behaviour has earlier been reported by FurinCitation46 in case of Ca-dependent serine protease. The fibrinolytic activity of xylarinase was completely suppressed by zinc and ferrous ion. The N-terminal sequence comparison and inhibition of xylarinase by these chelating agents established it as a metalloprotease. The metalloprotease nature of xylarinase will protect it from serpin attack which usually targets serine proteases involved in blood coagulation. The biochemical nature of xylarinase is somewhat similar to other metalloprotease like herinase and ulveaseCitation9,Citation32. Xylarinase showed high specificity towards fibrinogen which is evident in fibrinogen clotting time assay where the variation fibrinogen concentration prolong the clotting time whereas no such effect was observed when the concentration of thrombin was changed. The Km and Vmax of xylarinase were 240.9 μM and 1.10 for fibrinogen and 246 μM and 1.22 U/ml for fibrin, respectively, which were almost similar and quite lowCitation37. These results were in concurrence with the fibrinogen clotting time assay where it was clearly evident that xylarinase is a fibrinogen dependent enzyme. Thus either of the substrates can be used for studying the kinetic parameters. Both plasmin and streptokinase exhibited higher Km than xylarinase. Further, in vitro assay for thrombolytic activity assay of xylarinase showed dose dependent dissolution of thrombus. Fifty microliters of xylarinase was required to completely dissolve the thrombus which is similar to that of streptokinaseCitation12. It showed better efficacy than plasmin. The blood clotting cascade involved three pathways: intrinsic, extrinsic and a common pathway. The present study demonstrated that xylarinase possesses anticoagulation properties as shown by elongation of PT and APTT. The prolongation of APTT might be due to decrease in coagulation factors such as VIII, IX and XI which results into inhibition of intrinsic and common pathways whereas the prolongation of PT suggests inhibition of extrinsic pathway due to decrease in clotting factors such as V, VII and X. Thus, xylarinase might block the activation of clotting cascade by suppressing the thrombin pathway. Similar prolongation in APTT and PTT was observed in case of bi-functional fibrinolytic enzymes like ulvease and codiaseCitation32,Citation47.
Conclusion
Xylarinase thus is a novel metalloprotease possessing dual activity including direct degradation of fibrin(ogen) or by activating the tissue plasminogen. Xylarinase possesses no hemorrhagic effect under in vitro conditions. Thus xylarinase stands out as a potential candidate in therapeutic agents in preclinical studies in thrombolytic therapy.
Acknowledgements
Vineet Meshram is thankful to University Grants Commission, Govt. of India, New Delhi for providing financial support via Rajiv Gandhi National Fellowship (2011). We express our gratitude to Dr. Girish Sahni, Director, IMTECH (Now DG, CSIR), Mrs. Paramjit Kaur and Ms. Neha Rana, Technical officer and Ms. Navneet Kaur, Research Scholar, IMTECH, Chandigarh, India for their kind cooperation in N-terminal sequencing and MALDI-TOF analysis. We sincerely thank Dr. Anu Garg, MD (Microbiology), Sigma Laboratories, Patiala, Punjab for her kind cooperation in studying coagulating parameters.
Declaration of interest
The authors have no declarations of interest to report.
References
- Mander P, Cho SS, Simkhada JR, et al. A low molecular weight chymotrypsin-like novel fibrinolytic enzyme from Streptomyces sp. CS624. Process Biochem 2011;46:1449–55
- Mensah GA, Morany AE, Rothz GA, Narulah J. The global burden of ischemic heart disease in 1990 and 2010: the Global Burden of Disease 2010 study. Circulation 2014;9:183–4
- Shirasaka N, Naitou M, Okamura K, et al. Purification and characterization of a fibrinolytic protease from Aspergillus oryzae KSK-3. Mycoscience 2012;53:354–64
- White HD, Van de Werf FJJ. Thrombolysis for acute myocardial infarction. Circulation 1998;97:1632–46
- Thames MD, Sease DR, Damian A. Ischemic heart diseases: an overview. Adv Stud Med 2004;4:794–802
- Lu F, Sun L, Lu Z, et al. Isolation and identification of an endophytic strain EJS-3 producing novel fibrinolytic enzymes. Curr Microbiol 2007;54:435–9
- Ellis K, Brener S. New fibrinolytic agents for MI: as effective as current agents, but easier to administer. Cleve Clin J Med 2004;71:20–37
- Mackman N. Triggers, targets and treatments for thrombosis. Nature 2008;451:914–18
- Choi BS, Sapkota K, Choi JH, et al. Herinase: a novel bi-functional fibrinolytic protease from the monkey head mushroom, Hericium erinaceum. Appl Biochem Biotechnol 2013;170:609–22
- Kim HC, Choi BS, Sapkota K, et al. Purification and characterization of a novel, highly potent fibrinolytic enzyme from Paecilomyces tenuipes. Process Biochem 2011;46:1545–53
- Kotb E. Activity assessment of microbial fibrinolytic enzymes. Appl Microbiol Biotechnol 2013;97:6647–65
- Babu V, Devi CS. In vitro thrombolytic activity of purified streptokinase extracted from Streptococcus equinus VIT_VB2 isolated from bovine milk. J Thromb Thrombolysis 2015;39:71–8
- Yadav S, Datt M, Singh S, Sahani S. Role of the 88–97 loop in plasminogen activation by streptokinase probed through site-specific mutagenesis. Biochim Biophys Acta 2008;1784:1310–18
- Peng Y, Yang X, Zhang Y. Microbial fibrinolytic enzymes: an overview of source, production, properties, and thrombolytic activity in vivo. Appl Microbiol Biotechnol 2005;69:126–32
- Rovati JI, Delgado OD, Figueroa LIC, Farina JI. A novel source of fibrinolytic activity: Bionectria sp., an unconventional enzyme-producing fungus isolated from Las Yungas rainforest (Tucumán, Argentina). World J Microb Biot 2010;26:55–62
- Bacon CW, White JF. Microbial endophytes. New York: Marcel Dekker; 2000
- Rodriguez RJ, White JF, Arnold AE, Redman RS. Fungal endophytes: diversity and functional roles. New Phytol 2009;182:314–30
- White JFJ, Torres MS. Is plant endophyte-mediated defensive mutualism the result of oxidative stress protection? Physiol Plant 2010;138:440–6
- White J, Bacon CW. The secret world of endophytes in perspective. Fungal Ecol 2012;5:287–8
- Li Y, Shuang JL, Yuan WW, et al. Verticase: a fibrinolytic enzyme produced by Verticillium sp. Tj33, an endophyte of Trachelospermum jasminoides. J Integr Plant Biol 2007;49:1548–54
- Ueda M, Kubo T, Miyatake K, Nakamura T. Purification and characterization of fibrinolytic alkaline protease from Fusarium sp. BLB. Appl Microbiol Biotechnol 2007;74:331–8
- Wu B, Wu L, Chen D, et al. Purification and characterization of a novel fibrinolytic protease from Fusarium sp. CPCC 480097. J Ind Microbiol Biotechnol 2009;36:451–9
- Schulz B, Wanke U, Draeger S, Aust HJ. Endophytes from herbaceous plants and shrubs: effectiveness of surface sterilization methods. Mycol Res 1993;97:1447–50
- Ali UF, Ibrahim ZM. Production and some properties of fibrinolytic enzyme from Rhizomucor miehei (Cooney & Emerson) Schipper. J Appl Sci Res 2008;4:892–9
- Bi Q, Chu J, Feng Y, et al. Purification and characterization of a new serine protease with fibrinolytic activity from the marine invertebrate, Urechis unicinctus. Appl Biochem Biotechnol 2013;170:525–40
- Cui L, Dong MS, Chen XH, et al. A novel fibrinolytic enzyme from Cordyceps militaris, a Chinese traditional medicinal mushroom. World J Microb Biot 2008;24:483–9
- Bradford MM. A rapid and sensitive method for the quantitation of microgram quantities of protein utilizing the principle of protein–dye binding. Anal Biochem 1976;72:248–54
- Laemmli UK. Cleavage of structural proteins during the assembly of the head of bacteriophage T4. Nature (Lond) 1970;227:680–5
- Blum H, Beier H, Gross HJ. Improved silver staining of plant proteins, RNA and DNA in polyacrylamide gels. Electrophoresis 1987;8:93–9
- Bi Q, Han B, Liu W, et al. UFEIII, a fibrinolytic protease from the marine invertebrate, Urechis unicinctus. Biotechnol Lett 2013;35:1115–20
- Kim SH, Choi NS, Lee WY. Fibrin zymography: a direct analysis of fibrinolytic enzymes on gels. Anal Biochem 1998;263:115–16
- Kang SR, Choi JH, Kim DW, et al. A bifunctional protease from green alga Ulva pertusa with anticoagulant properties: partial purification and characterization. J Appl Phycol 2016;28:599--607
- Astrup T, Mullertz S. The fibrin plate method for estimating fibrinolytic activity. Arch Biochem Biophys 1952;40:346–51
- Matsubara K, Hori K, Matsuura Y, Miyazawa K. Purification and characterization of a fibrinolytic enzyme and identification of fibrinogen clotting enzyme in a marine green alga, Codium divaricatum. Comp Biochem Physiol B, Biochem Mol Biol 2000;125:137–43
- Ju X, Cao X, Sun Y, et al. Purification and characterization of a fibrinolytic enzyme from Streptomyces sp. XZNUM 00004. World J Microbiol Biotechnol 2012;28:2479–86
- Wu B, Wu L, Ruan L, et al. Screening of endophytic fungi with antithrombotic activity and identification of a bioactive metabolite from the endophytic fungal strain CPCC 480097. Curr Microbiol 2009;58:522–7
- Mukherjee AK, Rai SK, Thakur R, et al. Bafibrinase: a non-toxic, non-hemorrhagic, direct-acting fibrinolytic serine protease from Bacillus sp. strain AS-S20-I exhibits in vivo anticoagulant activity and thrombolytic potency. Biochimie 2012;94:1300–8
- White TJ, Bruns T, Lee S, Taylor J. Amplification and direct sequencing of fungal ribosomal RNA genes for phylogenetics. In: White BA, ed. PCR protocols: a guide to methods and applications. New York (NY): Academic Press; 1990;315–22
- Meshram V, Kapoor N, Saxena S. Muscodor kashayum sp. nov. – a new volatile anti-microbial producing endophytic fungus. Mycology 2013;4:196–204
- Tamura K, Peterson D, Peterson N, et al. MEGA5: molecular evolutionary genetics analysis using maximum likelihood, evolutionary distance, and maximum parsimony methods. Mol Biol Evol 2011;28:2731–9
- Marder VJ, Novokhatny V. Direct fibrinolytic agents: biochemical attributes, preclinical foundation and clinical potential. J Thromb Haemost 2010;8:433–44
- Cha WS, Park SS, Kim SJ, Choi D. Biochemical and enzymatic properties of a fibrinolytic enzyme from Pleurotus eryngii cultivated under solid-state conditions using corn cob. Bioresour Technol 2010;101:6475–81
- Kim SB, Lee DW, Cheigh CI, et al. Purification and characterization of a fibrinolytic subtilisin-like protease of Bacillus subtilis TP-6 from an Indonesian fermented soybean, Tempeh. J Ind Microbiol Biotechnol 2006;33:436–44
- Wang CT, Ji BP, Li B, et al. Purification and characterization of a fibrinolytic enzyme of Bacillus subtilis DC33, isolated from Chinese traditional Douchi. J Ind Microbiol Biotechnol 2006;33:750–8
- Simkhada JR, Cho SS, Mander P, et al. Purification, biochemical properties and antithrombotic effect of a novel Streptomyces enzyme on carrageenan-induced mice tail thrombosis model. Thromb Res 2012;129:176–82
- Hatsuzawa K, Nagahama M, Takahashi S, et al. Purification and characterization of furin, a Kex2-like processing endoprotease, produced in Chinese hamster ovary cells. J Biol Chem 1992;267:16094–9
- Choi JH, Sapkota K, Park SE, et al. Thrombolytic, anticoagulant and antiplatelet activities of codiase, a bi-functional fibrinolytic enzyme from Codium fragile. Biochimie 2013;95:1266–77