Abstract
Candida albicans represents the most prevalent microbial population in mucosal and systemic infections, usually confined to severely immunocompromised people. Considering the increase of resistant strains and the demand for new antifungal drugs endowed with innovative mechanism of action, we performed a ligand-based virtual screening in order to identify new anti-Candida compounds. Starting from a large library of natural/semisynthetic products and several published synthesized compounds, three coumarin derivatives were discovered in silico as new hit compounds and submitted to the in vitro assay in order to confirm their predicted biological activity.
Introduction
The frequency of Candida infections has increased in recent years and Sanguinetti et al.Citation1 recently reviewed the mechanisms of resistance. Clinically, azoles are first-line agents in treating fungal infections; however, there is an emergent demand for the discovery of new antifungal agents to open the possibility of a novel therapeutic approachCitation2. As regards natural products, in literature there are several studies investigating the promising antimicrobial activity of chemical compounds content in essential oils and medicinal plants, also traditionally used for the treatment of various health disordersCitation3–5.
Both structure and ligand-based virtual screening (SBVS and LBVS) represent alternative and innovative approaches for identifying new compounds, but, until now, in the field of anti-Candida, computational techniques were used only to explain the structure–activity relationships, to design synthetic derivatives for the lead optimization and to display hypothetic mechanisms of actionCitation6–8. LBVS often relies on 3D pharmacophore model that is a set of interactions, or chemical features, aligned in three-dimensional space and developed by two possible approaches: one based on the known X-ray or NMR structure of the receptor, and the other one starting from a set of ligands that are supposed to bind to the same unknown targetCitation9. Therefore, we exploited our synthetic library of (4-aryl-thiazol-2-yl)hydrazine derivatives (Table S1), previously reported in other works as potent anti-Candida spp. compounds endowed with low cytotoxicity and synergy with clotrimazoleCitation2,10–12, without a determinate molecular target, to generate a ligand-based pharmacophore model with the purpose to virtually screen a library of natural/semisynthetic products and to identify new potential antifungal drugs. Some 2-thiazolylhydrazine scaffold derivatives were also alreadyCitation13 studied for their selective human monoamine oxidase inhibitory effects by computational methods.
Ligand-based approach
The 109 structurally similar molecules were ranked according to their MIC values, expressed in μg/mL against several clinical isolates of Candida albicans, as follows: compounds with MIC values ≤0.25 μg/mL were classified as “active”, while compounds with MIC equal to the value of 256 μg/mL were considered as “inactive”. Ligand-based pharmacophore modeling usually requires the “Training-Set” molecules, generally the most active, that are used for the pharmacophore creation and the “Test-Set” ligands, active and inactive, used to verify the resulting pharmacophore hypothesis. Due to the fact that the anti-Candida activity of most of the compounds (without asterisk in Table S1) was evaluated using a mixture of E and Z isomers and the biological activity is usually due to one of the isomers, the molecules 14, 33, 36 and 61 () (with asterisk in Table S1 because without isomers) were used as “Training Set” for the pharmacophore creation, into the Ligand Scout’s Ligand-Based Modeling Perspective version 3.19. In order to verify the resulting pharmacophore hypothesis, compounds 1–3, 12–15, 33–41, 44 and 59–64 were selected as “Test-Set”.
Figure 1. 2D chemical structures of the “active” compounds 14, 33, 36 and 61, with MIC values of 0.25 μg/mL, used as “Training-Set” into the LigandScout’s Ligand-Based Modeling Perspective, in order to generate the pharmacophore model for the LBVS.
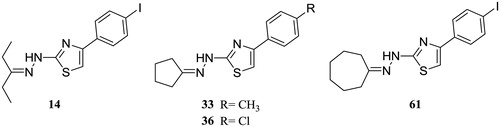
All 2D molecules were built into the graphic interface Maestro of the Scrödinger Suite 2014Citation14, and saved as .mol2 files, then converted in the .lbd format by the “Idbgen” tool of LigandScout. Ligand conformations were generated using the default settings of OMEGA best, which produced a maximum of 500 conformations per molecule. Finally, LigandScout interpolated common pharmacophore features for a configurable number of best alignment solutions. We observed that shared and merged features were located in the same spatial positions, in particular, 10 pharmacophore hypotheses were found with the feature tolerance scale of 1.0 and ranked using the pharmacophore fit and atom overlap as scoring functions. The chemical functionalities of the selected model are all matched by the chemical groups of the “Training-Set” molecules, except the aliphatic portion (): the thiazole nucleus is located inside an aromatic ring and a hydrophobic feature (AR-HF1), moreover, it fits a region of hydrogen bond acceptor (HBA1) by the nitrogen atom; the phenyl portion at C4 of the thiazole is located inside the second aromatic ring and another hydrophobic feature (AR-HF2), while the substituents at the para position of this ring map an additional HF; finally, the hydrazone linker defines a region of hydrogen bond donor (HBD) and the second site of HBA2. The best model () was validated to prospectively evaluate its performance in a VS approach. To this aim, E and Z isomers of all molecules in Table S1, except those with the asterisk, were included for the construction of two libraries (“active” and “inactive” compounds). Therefore, 42 “active” compounds were used to generate 2100 decoys (1:50), downloaded from the DUD-E siteCitation15 and then added to the 21 “inactive” for a total of 2121 compounds. The screening process of the built libraries and the receiver-operating curve (ROC) analysis showed a good discrimination of the pharmacophore model; in fact, 34 hits (32 “active” and 2 “inactive”) were found with a sensitivity of about 78% and the AUC value of 88.0% ().
Figure 2. The “Training-Set” compounds, rendered as stick with the carbon color indicated in brackets, 14 (blue), 33 (cyan), 36 (red) and 61 (grey) in the best alignment solution, mapped to the different chemical features of the final pharmacophore hypothesis. The feature dictionary used is the following: aromatic ring and hydrophobic features (AR-HF1 and AR-HF2) displayed as grey toroids into the yellow spheres, hydrogen bond acceptors (HBA1 and HBA2) defined as red spheres, hydrophobic feature (HF) as yellow sphere and hydrogen bond donor (HBD) as green arrow. All non-carbon atoms are colored according to atom types. (For the colored version of this Figure, please refer to the online article.).
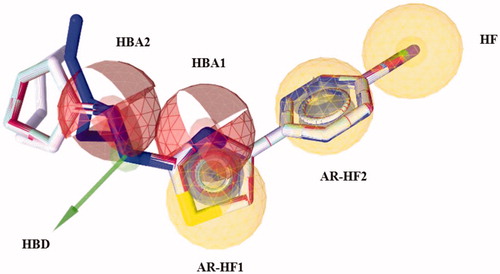
Figure 3. Into the LigandScout, the automated generation of the ROC curve for performance assessments, demonstrated the good discrimination of the selected pharmacophore model. In fact, 34 hits (32 “active” and 2 “inactive”) were found, starting from a library of 2163 compounds, with a sensitivity of about 78% and the AUC value of 88.0%.
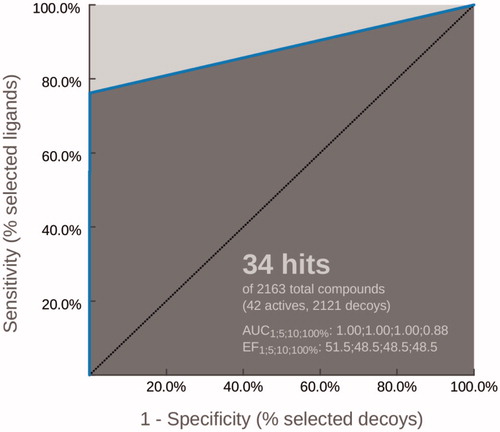
The LBVS was performed into the LigandScout’s Screening Perspective using the validated pharmacophore model displayed in . A library of 152 056 natural compounds was downloaded from the Zinc Database siteCitation16, using all entries of the Natural Products Catalogs, while 105 490 natural/semi-synthetic compounds were available from our in house database. Therefore 257 546 ligands were prepared as follows: (i) the software CanvasCitation17 was used to remove the duplicates and to select 237 950 compounds with drug-like properties; (ii) the 3D structures were generated by the “LigPrep” utility of the Scrödinger package 2014Citation18; (iii) tautomeric forms and protonated states at the pH range 5–9 were also considered, ending up with a library of 238 377 molecules, further converted in the .ldb format using the same protocol previously reported for the “active” and “inactive” libraries. The LBVS permitted to early identify three virtual hit compounds, belonging to the coumarin scaffold, with the corresponding ZINC codes 72325331, 72325699 and 72325788 (). The potential anti-Candida compounds, only results of the LBVS study, displayed the same alignment, except for the different aliphatic portion, with appropriate chemical functions for a good fit into the six features of the pharmacophore hypothesis. In fact, for each of them, the coumarin portion fulfils the AR-HF2 and the HF features; the pyridine ring maps the AR-HF1 and, in addition, the HBA1 region by the nitrogen atom; finally, the amide group is located within the HBA2 feature, by the carbonylic portion, and within the HBD by the protonated nitrogen atom (). In order to verify a possible therapeutic use of these bioactive molecules, the chemico-physical properties prediction was carried out by Canvas and reported in Table S2, concluding that all compounds have a drug-like profileCitation19–21.
Figure 4. 2D chemical structures of the coumarin derivatives, with the respective ZINC codes, identified as new potential C. albicans inhibitors, by the LBVS performed from a large library of natural/semisynthetic compounds. The 3 new hits, on the right of the figure, are rendered as stick with the carbon color indicated in brackets, ZINC 72325331 (green), 72325699 (violet), 72325788 (grey). They are in the best alignment solution, mapped to the different chemical features of the pharmacophore hypothesis described in the previous figure. All non-carbon atoms are colored according to atom types. (For the colored version of this Figure, please refer to the online article.).
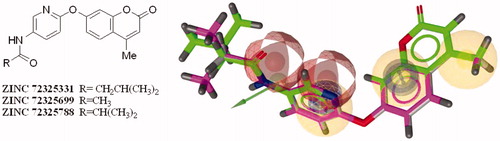
Antifungal activity
The antifungal activity of the three coumarins, purchased by MolPort with purity of 92–95%, was evaluated against six clinical fungal isolates of Candida albicans and compared with topical and systemic reference drug fluconazole () by using the same experimental protocols carried out for our synthetic library of (4-aryl-thiazol-2-yl)hydrazine derivatives. The used clinical isolates were recently collected from specimens of patients at the “Azienda Policlinico Umberto I” (Sapienza University of Rome) and were obtained from haematology/oncology and surgery departments, which also included an intensive care unit. In particular, the samples were isolated from the upper and lower respiratory tract, blood and indwelling venous catheters; the isolates were identified by conventional methodologies. Prior to testing, each isolate was subcultured on a qualified medium to ensure purity and optimal growth. All derivatives were dissolved in DMSO. The in vitro antifungal activities were determined by the broth microdilution method with Sabouraud dextrose broth (BBL Microbiology Systems, Cockeysville, MD) as recommended by the NCCLS. Briefly, microtiter plates containing serial dilutions of each compound were inoculated with each organism to yield the appropriate density (103/mL) in a 100 μL final volume; each plate included positive controls (fungi without a compound) and a negative control (medium only). The plates were incubated for 24 h at 37 °C. The MIC for all isolates was defined as the lowest concentration of antifungal agents that completely inhibited the growth of the organism, as detected by unaided eyeCitation22,Citation23. From these data it was possible to extrapolate that these new compounds are active antifungal agents endowed with the same anti-Candida efficacy of Fluconazole in vitro.
Table 1. Minimal inhibitory concentration (MIC) of the coumarin derivatives, displayed as ZINC codes, and reference compound (fluconazole) against six clinical strains of Candida albicans.
Results and discussion
The selected coumarin scaffold displayed good biological activity confirming that the aliphatic chain (methyl, isopropyl and isobutyl) at the lateral amidic portion was not crucial for the in vitro anti-Candida efficacy. Actually, in literature, several works demonstrate the anti-fungal activity of coumarin derivativesCitation24–29. In particular, for the methyl and isopropyl ZINC compounds, we found a reference that previously reported the antimicrobial activityCitation30, confirming the good ability of the pharmacophore hypothesis to early retrieve anti-Candida compounds. In fact, our computational studies permitted to identify in silico, with reduced time and costs, the most promising candidates to focus the experimental efforts on, starting from a large database of natural and semisynthetic ligands. In particular, by a comparison between the “Training Set” molecules, used to generate the ligand-based pharmacophore model, and the three coumarin hit compounds identified by our LBVS study, we observed the importance of a shared heterocycle with a HBA (e.g. thiazole and pyridine) as central feature linked to HBD/acceptor sites (e.g. hydrazone and carbonylic moiety) and to aromatic/hydrophobic features (e.g. phenyl and coumarin portions). In conclusion, our study represents the first example of a successful application of a LBVS approach to identify promising anti-Candida compounds. The ligand-based pharmacophore hypothesis generated in silico, and validated by the experimental results, may be used for future virtual screening studies starting from in house libraries of natural, semisynthetic and synthetic compounds. Further investigations will also focus on the identification of the macromolecular target and more potent anti-Candida agents.
Supplementary material available online
IENZ_1156103_SupplementaryMaterial.pdf
Download PDF (1.1 MB)Declaration of interest
The authors report no declaration of interest. This work was supported by Interregional Research Center for Food Safety and Health, the Magna Græcia University of Catanzaro (MIUR PON a3_00359) and local grants from University “G. d’Annunzio” of Chieti (Dr. S. Carradori 2015).
References
- Sanguinetti M, Posteraro B, Lass-Flörl C. Antifungal drug resistance among Candida species: mechanisms and clinical impact. Mycoses 2015;58:2–13
- Carradori S, Secci D, Bolasco A, et al. Synthesis and cytotoxicity of novel (thiazol-2-yl)hydrazine derivatives as promising anti-Candida agents. Eur J Med Chem 2013;65:102–11
- (a) Vandal J, Abou-Zaid MM, Ferroni G, Leduc LG. Antimicrobial activity of natural products from the flora of Northern Ontario, Canada. Pharm Biol 2015;53:800–6 (b) De Monte C, Bizzarri B, Gidaro MC, et al. Bioactive compounds of Crocus sativus L. and their semi-synthetic derivatives as promising anti-Helicobacter pylori, anti-malarial and anti-leishmanial agents. J Enzyme Inhib Med Chem 2015;30:1027–33
- Cavaleiro C, Salgueiro L, Gonçalves MJ, et al. Antifungal activity of the essential oil of Angelica major against Candida, Cryptococcus, Aspergillus and dermatophyte species. J Nat Med 2015;69:241–8
- Cannas S, Molicotti P, Usai D, et al. Antifungal, anti-biofilm and adhesion activity of the essential oil of Myrtus communis L. against Candida species. Nat Prod Res 2014;28:2173–7
- Rani N, Kumar P, Singh R, Sharma A. Molecular docking evaluation of imidazole analogues as potent Candida albicans 14α-demethylase inhibitors. Curr Comput Aided Drug Des 2015;11:8–20
- Jiang Z, Gu J, Wang C, et al. Design, synthesis and antifungal activity of novel triazole derivatives containing substituted 1,2,3-triazole-piperdine side chains. Eur J Med Chem 2014;82:490–7
- Xiao ZP, Wang XD, Wang PF, et al. Design, synthesis, and evaluation of novel fluoroquinolone-flavonoid hybrids as potent antibiotics against drug-resistant microorganisms. Eur J Med Chem 2014;80:92–100
- Wolber G, Langer T. LigandScout: 3-D pharmacophores derived from protein-bound ligands and their use as virtual screening filters. J Chem Inf Model 2005;45:160–9
- Secci D, Bizzarri B, Bolasco A, et al. Synthesis, anti-Candida activity, and cytotoxicity of new (4-(4-iodophenyl)thiazol-2-yl)hydrazine derivatives. Eur J Med Chem 2012;53:246–53
- Chimenti F, Bizzarri B, Bolasco A, et al. Synthesis and biological evaluation of novel 2,4-disubstituted-1,3-thiazoles as anti-Candida spp. agents. Eur J Med Chem 2011;46:378–82
- Chimenti F, Bizzarri B, Maccioni E, et al. Synthesis and in vitro activity of 2-thiazolylhydrazone derivatives compared with the activity of clotrimazole against clinical isolates of Candida spp. Bioorg Med Chem Lett 2007;17:4635–40
- (a) Chimenti F, Bolasco A, Secci D, et al. Investigations on the 2-thiazolylhydrazyne scaffold: synthesis and molecular modeling of selective human monoamine oxidase inhibitors. Bioorg Med Chem Lett 2010;18:5715–23 (b) D’Ascenzio M, Carradori S, Secci D, et al. Identification of the stereochemical requirements in the 4-aryl-2-cycloalkylidenhydrazinylthiazole scaffold for the design of selective human monoamine oxidase B inhibitors. Bioorg Med Chem 2014;22:2887–95 (c) Chimenti P, Petzer A, Carradori S, et al. Exploring 4-substituted-2-thiazolylhydrazones from 2-, 3-, and 4-acetylpyridine as selective and reversible hMAO-B inhibitors. Eur J Med Chem 2013;66:221–7 (d) Chimenti F, Secci D, Bolasco A, et al. Synthesis, stereochemical separation, and biological evaluation of selective inhibitors of human MAO-B: 1-(4-arylthiazol-2-yl)-2-(3-methylcyclohexylidene)hydrazines. J Med Chem 2010;53:6516–20
- Maestro, version 9.7. New York, NY: Schrödinger, LLC; 2014
- Mysinger MM, Carchia M, Irwin JJ, Shoichet BK. Directory of useful decoys, enhanced (DUD-E): better ligands and decoys for better benchmarking. J Med Chem 2012;55:6582–94
- Irwin JJ, Shoichet BK. ZINC – a free database of commercially available compounds for virtual screening. J Chem Inf Model 2005;45:177–82
- Canvas, version 2.2. New York, NY: Schrödinger, LLC; 2014
- LigPrep, version 2.9. New York, NY: Schrödinger, LLC; 2014
- Lipinski CA, Lombardo F, Dominy BW, Feeney PJ. Experimental and computational approaches to estimate solubility and permeability in drug discovery and development settings. Adv Drug Deliv Rev 2001;46:3–26
- Veber D, Johnson S, Cheng H, et al. Molecular properties that influence the oral bioavailability of drug candidates. J Med Chem 2002;45:2615–23
- Walters W, Murcko M. Prediction of ‘drug-likeness’. Adv Drug Deliv Rev 2002;54:255–71
- National Committee for Clinical Laboratory Standard. Reference method for broth dilution antifungal susceptibility testing of yeasts; approved standard. 3rd ed. DM27-A3 vol. 28, n° 14. Wayne, PA: National Committee for Clinical Laboratory Standard; 2008
- De Monte C, Carradori S, Bizzarri B, et al. Anti-Candida activity and cytotoxicity of a large library of new N-substituted-1,3-thiazolidin-4-one derivatives. Eur J Med Chem 2016;107:82–96
- Shi Y, Zhou CH. Synthesis and evaluation of a class of new coumarin triazole derivatives as potential antimicrobial agents. Bioorg Med Chem Lett 2011;21:956–60
- Singh LK, Priyanka SV, Katiyar D. Design, synthesis and biological evaluation of some new coumarin derivatives as potential antimicrobial agents. Med Chem 2015;11:128–34
- Damu GL, Cui SF, Peng XM, et al. Synthesis and bioactive evaluation of a novel series of coumarinazoles. Bioorg Med Chem Lett 2014;24:3605–8
- Arshad A, Osman H, Bagley MC, et al. Synthesis and antimicrobial properties of some new thiazolyl coumarin derivatives. Eur J Med Chem 2011;46:3788–94
- Mladenović M, Vuković N, Sukdolak S, Solujić S. Design of novel 4-hydroxy-chromene-2-one derivatives as antimicrobial agents. Molecules 2010;15:4294–308
- Chimenti F, Carradori S, Secci D, et al. Synthesis and biological evaluation of novel conjugated coumarin–thiazole systems. J Heterocl Chem 2009;46:575–8
- Chai X, Yu S, Wang X, et al. Synthesis and antifungal activity of novel 7-O-substituted pyridyl-4-methyl coumarin derivatives. Med Chem Res 2013;22:4654–62