Abstract
Cancer cachexia is a progressive metabolic disorder that results in depletion of adipose tissue and skeletal muscle. A growing body of literature suggests that maintaining adipose tissue mass in cachexia may improve quality-of-life and survival outcomes. Studies of lipid metabolism in cachexia, however, have generally focused on later stages of the disorder when severe loss of adipose tissue has already occurred. Here, we investigated lipid metabolism in adipose, liver and muscle tissues during early stage cachexia – before severe fat loss – in the colon-26 murine model of cachexia. White adipose tissue mass in cachectic mice was moderately reduced (34–42%) and weight loss was less than 10% of initial body weight in this study of early cachexia. In white adipose depots of cachectic mice, we found evidence of enhanced protein kinase A - activated lipolysis which coincided with elevated total energy expenditure and increased expression of markers of brown (but not white) adipose tissue thermogenesis and the acute phase response. Total lipids in liver and muscle were unchanged in early cachexia while markers of fatty oxidation were increased. Many of these initial metabolic responses contrast with reports of lipid metabolism in later stages of cachexia. Our observations suggest intervention studies to preserve fat mass in cachexia should be tailored to the stage of cachexia. Our observations also highlight a need for studies that delineate the contribution of cachexia stage and animal model to altered lipid metabolism in cancer cachexia and identify those that most closely mimic the human condition.
Abbreviations
eWAT | = | epididymal white adipose tissue |
iWAT | = | inguinal white adipose tissue |
iBAT | = | interscapular brown adipose tissue |
PKA | = | protein kinase A |
ATGL | = | adipose triglyceride lipase |
HSL | = | hormone sensitive lipase |
UCP | = | uncoupling protein |
PPAR | = | peroxisome proliferator activated receptor |
PGC | = | peroxisome proliferator activated receptor gamma coactivator |
CPT | = | carnitine palmitoyltransferase |
DIO | = | iodothyronine deiodinase |
MuRF | = | muscle ring finger protein |
COX | = | cytochrome c oxidase subunits |
GYK | = | glycerokinase |
PRDM | = | PR domain zinc finger protein |
ACOX | = | acyl CoA oxidase |
CRP | = | C-reactive protein |
LPL | = | lipoprotein lipase |
H&E | = | hematoxylin and eosin |
TEE | = | total energy expenditure |
RER | = | respiratory exchange ratio |
Introduction
Cancer cachexia is a progressive, multi-factorial metabolic syndrome that results in marked loss of adipose tissue and skeletal muscle mass.Citation1 Cachexia effects approximately 30% of cancer patients and is associated with significant morbidity and mortalityCitation2 that is predominantly due to muscle loss.Citation3,4 Fat loss, however, may precede muscle wasting in cachexiaCitation5 and several recent longitudinal studies in cancer patients found accelerated loss of adipose tissue predicted poorer survival.Citation6-8 Moreover, in 2 different murine models of cancer cachexia, preserving fat mass by genetically inhibiting lipolysis also preserved skeletal muscle mass.Citation9 Thus, unveiling the mechanisms of adipose tissue depletion may contribute to the development of therapies that improve both survival and quality-of-life outcomes in cachectic patients.
Fat loss from adipose tissue in cancer cachexia is partly the result of increased lipolysis.Citation10-16 Elevated rates of lipolysis in cachexia would contribute to loss of total body fat only if lipid utilization were also increased. Indeed, clinical studies have reported elevated rates of whole body fatty acid oxidation in weight losing (≥10% of initial body weight) cancer patients.Citation12,17,18 Studies of cachexia in rodents suggest activation of thermogenesis (i.e., increased mitochondrial uncoupling of oxidative phosphorylation and elevated fatty acid β-oxidation) in brown adipose tissueCitation19,20 may contribute to elevated whole body lipid utilization in cachexia, at least in later stages of cachexia.Citation20 Additionally, recent studies in healthy rodents and human adipocytes have linked stimulation of lipolysis in white adipose tissue with increased fatty acid oxidationCitation21,22 and uncouplingCitation21,23,24 in white adipose depots. This “brown-like” adipocyte phenotype in white adipose tissue might also contribute to elevated whole body lipid utilization in cachexia and thus depletion of adipose tissue. Consistent with these studies, a gene expression profile of subcutaneous adipose tissue comparing cachectic cancer patients (∼10% weight loss) to weight-stable cancer patients demonstrated genes related to fatty acid degradation and oxidative capacity were more highly expressed in cachectic patients and coincided with elevated levels of whole body fatty acid oxidation.Citation18
While studies of adipose tissue suggest lipolysis and oxidation of fatty acids within adipocytes may be enhanced in later stages of cachexia, studies of liver and muscle tissue suggest there is a reduction in fatty acid oxidation/oxidative capacity in hepatocytesCitation25-27 and myocytesCitation28 and consequently, lipid accumulation in these tissuesCitation26,29,30 in more advanced stages of cachexia.
To our knowledge, studies of lipid metabolism in adipose, liver and muscle tissues have not been reported for early stages of cachexia when weight loss is less than 10% of initial body weight and adipose tissue loss is significant, but not severe. Therefore, the purpose of the current study was to characterize lipolysis and processes that promote lipid utilization within adipose tissue in the colon-26 model of cancer-induced cachexia during the early stage of wasting; that is, at the onset of anorexia and before severe body weight loss occurred. The secondary objective was to determine the extent of lipid accumulation and the capacity for lipid utilization in muscle and liver in early cachexia in this model. Results presented here demonstrate that lipid metabolism in the early stage of colon-26 tumor-induced cachexia is distinctly different from that reported in later stages of cachexia in the same model and in other cachexia models. These initial metabolic changes preceding later stages of cachexiaCitation3 may offer new treatment and intervention targets to preserve fat mass.
Results
Effects of colon-26 carcinoma on adipose and muscle tissues in early cachexia
Because C-26 carcinoma may induce anorexia in mice,Citation31,32 we included a pair-fed group in our study to determine effects of tumor independent of food intake. Cumulative food intake in the Tumor group was significantly lower compared to the No Tumor group beginning on day 11 of the study (). On day 12 (study end), cumulative food intake in the Tumor group was decreased 9% compared to the No Tumor group but was not different from Pairfed mice (). Tumor mice lost 8% of their initial body weight by the end of the study (initial body weight, 20.7 ± 0.3 g; final tumor-free body weight, 19.0 ± 0.6 g, P < 0.05). Tumor mass (1125 ± 127 mg) was markedly lower than in our previous studies of late cachexia.Citation31,32 Compared to the No Tumor group, final tumor-free body weight and muscle mass in the Tumor group were each reduced 13% but were not different from the Pairfed group (). Markers of muscle proteolysis, however, were significantly increased in the Tumor group compared to Pairfed mice () indicating a significant effect of tumor on proteolytic gene expression exceeding that explained by reduced food intake.
Figure 1. Food intake, body and tissue weights, and muscle and adipose tissue catabolism. (A) Cumulative food intakes, n = 10 per group. (B) Tumor-free body weights at study end, n = 10 per group. (C) Muscle and adipose tissue weights at study end, n = 10 per group. (D) Expression of genes involved in proteolysis in quadriceps muscle, n=8 –10 per group. (E) eWAT adipocyte cross-sectional area, 150 cells/mouse x 9 mice per group with representative images of H&E stained eWAT. Magnification: 200×; Scale Bar: 50 μm. (F) eWAT mass by tumor-free body weight. eWAT mass in Tumor mice was significantly lower than Pairfed mice but no different than the No Tumor group by ANCOVA with tumor-free body weight as a covariate (P < 0.001, post-hoc LSD test; n = 10 per group). Values represent means ± s.e.m. Data in A - E were evaluated by ANOVA with post-hoc Fisher's test. Different letters indicate statistically significant differences among groups, P < 0.05. quad, quadriceps; eWAT, epididymal white adipose tissue; iWAT, inguinal white adipose tissue; iBAT, interscapular brown adipose tissue; MuRF1, muscle ring finger protein 1.
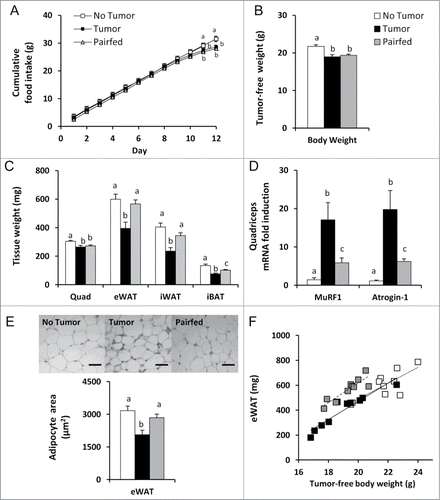
Despite similar food intakes, body weights and muscle mass, epididymal white adipose tissue (eWAT), inguinal white adipose tissue (iWAT), and interscapular brown adipose tissue (iBAT) mass were decreased 30, 31, and 26% respectively in Tumor mice compared to Pairfed mice (). These losses in the Tumor group were even greater when compared to the No Tumor group (eWAT, −34% vs No Tumor; iWAT, −42% vs No Tumor; iBAT, −44% vs No Tumor) (). In contrast, iWAT and eWAT mass were not different between Pairfed and No Tumor groups (). Consistent with eWAT mass, cross-sectional area of epididymal adipocytes was also significantly decreased in Tumor mice compared to Pairfed and No Tumor groups, 28 and 35% respectively (), indicating decreased adipocyte size partly explains reductions in adipose mass in Tumor mice. Adipocyte cross-sectional area, however, was not different between No Tumor and Pairfed groups ().
There were significant correlations between tumor-free body weight and adipose tissue mass (eWAT, r = 0.81, P < 0.001; iWAT, r = 0.84, P < 0.001). Because there were differences in tumor-free body weights among groups and body weight had a significant effect on adipose tissue mass (iWAT and eWAT, P < 0.001), we adjusted for this difference when comparing adipose tissue weights using ANCOVA. eWAT and iWAT mass were significantly decreased in Tumor mice compared to Pairfed mice (P < 0.001) but were not different than the No Tumor group after controlling for body weight. eWAT mass in No Tumor mice was also significantly decreased compared to Pairfed mice (P < 0.001) after adjusting for body weight. This analysis, coupled with the muscle mass data, demonstrates that eWAT mass is preferentially preserved in response to reduced food intake in Pairfed mice which does not occur in tumor-bearing mice. The relationship between tumor-free body weight and eWAT mass is plotted in .
Colon-26 carcinoma increases PKA activated lipolysis in early cachexia
The totality of evidence from human and animal studies suggests elevated lipolysis plays a key role in adipose tissue wasting in cachexia.Citation33 To determine the contribution of lipolysis to reductions in white adipose mass in Tumor mice in early cachexia, we measured unstimulated (basal) and stimulated lipolysis in fresh eWAT explants of all mice in the fed state at necropsy. Basal lipolysis, expressed as glycerol released per mg of eWAT explant, was significantly higher in Tumor mice compared to No Tumor mice, but was not different from Pairfed mice (). In contrast, when explants were stimulated in vitro with the β adrenergic agonist isoproterenol, glycerol released was not different among groups ().
Figure 2. Basal and stimulated lipolysis and lipolytic gene/protein expression (A) Unstimulated (basal) and isoproterenol stimulated release of glycerol per mg of eWAT explants, n = 9–10 per group. (B) Ratio of phosphorylated HSL Ser563 to total HSL protein with representative blots in eWAT, n = 9–10 per group. (C) eWAT protein expression with representative blots of ATGL and HSL, n = 7–8 per group. (C) eWAT mRNA expression, n = 9–10 per group. (E) Western blots of phosphorylated PKA substrates in eWAT. (F) Ratio of phosphorylated HSL Ser563 to total HSL protein with representative blots in iWAT, n = 8–10 per group. Values represent means ± s.e.m. Data in A-D, F were analyzed using one-way ANOVA with post-hoc Fisher's test. Different letters indicate statistically significant differences among groups, P < 0.05. ATGL, adipose triglyceride lipase; HSL, hormone sensitive lipase; PKA, protein kinase A.
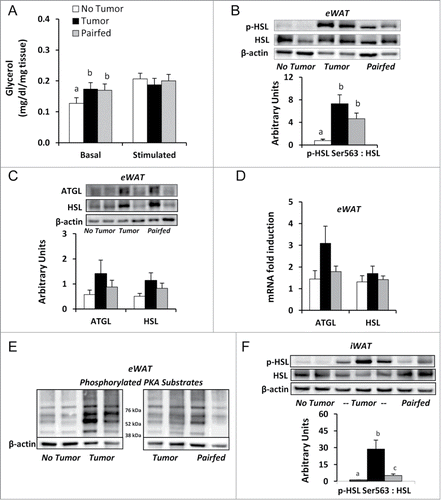
In an attempt to explain the basis of the lipolysis assay results, we measured lipase activation (phosphorylation) and lipase expression levels in the adipose tissues excised at necropsy. Consistent with the basal lipolysis explant assay findings, phosphorylated hormone sensitive lipase (HSL) Ser563 protein levels in eWAT, a surrogate marker for protein kinase A (PKA) activated lipolysis, was 9-fold higher in Tumor mice compared to No Tumor mice, but was not different from Pairfed mice (). There were no differences in protein or mRNA expression of the key lipases, adipose triglyceride lipase (ATGL) and HSL, among groups (). To further investigate our finding of PKA activated HSL, we immunoblotted proteins extracted from eWAT with a primary antibody specific for substrates phosphorylated by PKA at the consensus sequence RRXS/T. Qualitative analysis revealed enhanced phosphorylation of this consensus site in the Tumor group (n = 8) compared to No Tumor group (n = 4). Representative blots are shown in . Similar to our findings in eWAT, analysis in iWAT revealed phosphorylated HSL Ser563 protein levels in iWAT were significantly increased in Tumor mice compared to No Tumor and Pairfed mice ().
There was a significant correlation between basal lipolysis rates and eWAT mass (r = −0.48, P < 0.01). Because there were differences in eWAT mass among groups and eWAT mass had a significant effect on basal lipolysis rate (P < 0.05), we adjusted for this difference to compare basal lipolysis data. There were no differences in basal lipolysis between Tumor and No Tumor (P = 0.381) or Tumor and Pairfed groups (P = 0.337) after adjusting for eWAT mass. This analysis indicates eWAT mass may modulate glycerol release in Tumor mice.
Colon-26 carcinoma increases energy expenditure and temperature, but reduces activity in early cachexia
To investigate the extent to which differences in energy expenditure might explain decreased adipose mass in the Tumor group in early cachexia, we utilized indirect calorimetry to measure metabolic parameters for 24 hours in 6 mice from each group. We found 24 h total energy expenditure (TEE) – measured on study days 10 and 11 – was significantly increased in Tumor mice compared to Pairfed mice but was no different than the No Tumor group (). Energy balance (energy intake minus TEE) for this 24 h period was significantly decreased in the Tumor group compared to the No Tumor group but no different than the Pairfed group (). To further investigate the effects of the colon-26 tumor on energy expenditure, we analyzed calorimetry data after controlling for potential confounders (body weight and activity). Body weights, however, were not different among groups during indirect calorimetry (No Tumor, 21.6 ± 0.6; Tumor, 21.9 ± 0.4; Pairfed, 21.5 ± 0.3; p = 0.775) and did not affect energy expenditure (P = 0.350). Locomotor activity (beam-break counts), however, was significantly lower in the Tumor group compared to No Tumor and Pairfed groups () and tended to affect energy expenditure (P = 0.109). After adjusting for activity, energy expenditure was significantly higher in the Tumor group compared to the Pairfed group but still not different than the No Tumor group (). A plot of energy expenditure against activity counts every 20 minutes for Tumor and Pairfed mice is presented in . Consistent with energy expenditure data, rectal temperatures of Tumor mice taken on the day of indirect calorimetry measurements were significantly increased compared to Pairfed mice (P < 0.05) but were not different than No Tumor mice (No Tumor, 36.4 ± 0.4°C; Tumor, 36.4 ± 0.2°C; Pairfed, 35.3 ± 0.1°C). Mean rectal temperatures of all mice in the Tumor group over the 12 d of the study, however, were increased compared to both Pairfed and No Tumor groups (No Tumor, 36.4 ± 0.1°C; Tumor, 36.7 ± 0.1°C; Pairfed, 36.1 ± 0.1°C, P < 0.001). A plot of daily temperatures () demonstrates an effect of tumor on temperature as early as day 3 and shows reduced food intake in Pairfed mice coincides with a significant reduction in temperature that is not evident in the Tumor group.
Figure 3. Energy metabolism in cachectic mice (A) Total energy expenditure, energy intake and energy balance on study days 10–11 (24 h), n = 6 per group. (B) Locomotor activity (beam break counts) and energy expenditure analyzed by ANCOVA with activity as a covariate on study days 10–11. Energy expenditure was significantly increased in Tumor mice compared to Pairfed mice (P < 0.01, post-hoc LSD test; n = 6 per group). (C) Plot of average energy expenditure by average activity count every 20 minutes for Tumor and Pairfed mice. (D) Rectal temperatures on study days 0–12, n = 10 per group. Values represent means ± s.e.m. Except when otherwise noted, data were analyzed using one-way ANOVA with post-hoc Fisher's test. Different letters indicate statistically significant differences among groups, P < 0.05.
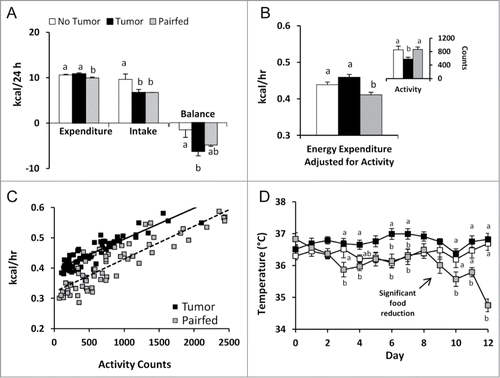
Colon-26 carcinoma increases lipid utilization in early cachexia
Analysis of calorimetric data showed the respiratory exchange ratio (RER) in the Tumor group was significantly lower than mice in the No Tumor group (P < 0.01), but no different than the Pairfed group (No Tumor, 0.86 ± 0.02; Tumor, 0.79 ± 0.01, Pairfed, 0.80 ± 0.00). Based on RER values, 70% of the Tumor and Pairfed group's total energy was derived from lipid whereas 48% of total energy was derived from lipid in the No Tumor group.
Colon-26 carcinoma increases markers of fatty acid oxidation and thermogenesis in brown adipose tissue in early cachexia
In iBAT, mRNA expression of the fatty acid uptake enzyme, lipoprotein lipase (LPL), was increased in Tumor mice compared to No Tumor mice (). Expression of the key lipid uptake and transporter protein [fatty acid translocase, cluster of differentiation (CD36)] and genes involved in promoting fatty acid oxidation [peroxisome proliferator activated receptor δ (PPARδ)] and mitochondrial oxidative phosphorylation [cytochrome c oxidase subunit 4 (COX4), cytochrome c oxidase subunit 8b (COX8B)] were increased in Tumor mice compared to Pairfed mice but were no different than the No Tumor group (). Glycerokinase (GYK), the key kinase involved in maintaining adequate stores of triglycerides for oxidation in BATCitation34 was also significantly increased in Tumor mice compared to both Pairfed and No Tumor groups (). Previous studies have suggested brown adipose thermogenesis is associated with wasting in later stages of cachexiaCitation19,20,35 which would explain increased expression of genes related to lipid uptake and utilization in iBAT. mRNA expression of the key kinase (p38α MAPK), transcriptional co-activators [peroxisome proliferator activated receptor gamma coactivator 1α (PGC1α), PR domain zinc finger protein 16 (PRDM16)], activating enzyme [Type II iodothyronine deiodinase (DIO2)] and uncoupling protein [uncoupling protein 1 (UCP1)] contributing to thermogenic capacity were significantly increased in Tumor mice compared to Pairfed mice but were no different than the No Tumor group with the exception of DIO2 (). There were no differences in protein expression of UCP1 (normalized to β-actin) among groups (). However, significant correlations were found between UCP1 protein expression and rectal temperatures (r = 0.64, P < 0.05) and basal lipolysis (r = 0.68, P < 0.05) within the Tumor group. Taken together these findings suggest compensatory suppression of thermogenic gene expression in response to reduced food intake is lost in tumor-bearing mice.
Figure 4. Gene and protein expression in brown adipose tissue (A) mRNA expression of markers of fatty acid uptake and utilization in iBAT, n = 8–10. (B) mRNA expression of genes related to brown adipose thermogenesis in iBAT, n = 9–10. (C) Ratio of UCP1 protein to β-actin with representative blots in iBAT, n = 8–10 per group. Values represent means ± s.e.m. Data were analyzed using one-way ANOVA with post-hoc Fisher's test. Different letters indicate statistically significant differences among groups, P < 0.05.
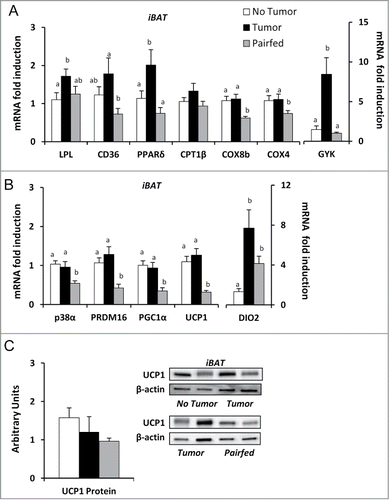
Colon-26 carcinoma increases markers of fatty acid oxidation, not thermogenesis in white adipose tissues in early cachexia
Next, we investigated whether markers of fatty acid oxidation and thermogenesis were altered in white adipose tissue. In eWAT, mRNA expression of the transcription factor involved in promoting fatty acid oxidation, PPARδ, was increased in Tumor mice compared to Pairfed mice but was no different than the No Tumor group (). The downstream target of PPARδ that promotes β oxidation, acyl CoA oxidase 1 (ACOX1), was significantly increased in the Tumor group compared to both No Tumor and Pairfed groups (). No significant differences were detected, however, in carnitine palmitoyltransferase 1β (CPT1β) or the mitochondrial cytochrome c oxidase subunits, COX4 or COX5a, among groups (). mRNA expression of the key kinase (p38α MAPK) and activating enzyme (DIO2) involved in promoting the expression of UCP1 (thus thermogenesis) were increased in Tumor mice compared to both No Tumor and Pairfed groups (). However, no differences in mRNA expression of the thermogenic regulatory proteins PGC1-α or PRDM16 () or protein expression of UCP1 () were detected among groups. Similar results were found for iWAT for mRNA () and protein expression (). These findings indicate early cachexia is not marked by WAT browning.
Figure 5. Gene and protein expression in eWAT (A) mRNA expression of markers of fatty acid utilization in eWAT, n = 8–10 per group. (B) mRNA expression of genes related to thermogenesis in eWAT, n = 9–10 per group. (C) Protein expression of UCP1 normalized to β-actin in eWAT, n = 8–10. Values represent means ± s.e.m. Data were analyzed using one-way ANOVA with post hoc Fisher's test. Different letters indicate statistically significant differences among groups, P < 0.05.
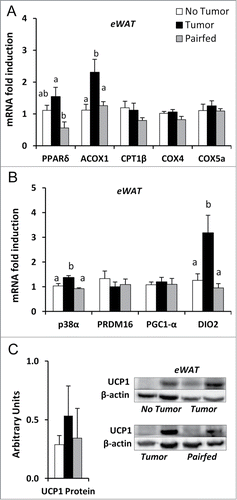
Figure 6. Gene and protein expression in iWAT (A) mRNA expression of genes related to thermogenesis in iWAT, n = 8–10 per group. (B) Protein expression of UCP1 normalized to β-actin in iWAT, n = 8 per group. Values represent means ± s.e.m. Data were analyzed using one-way ANOVA with post hoc Fisher's test. Different letters indicate statistically significant differences among groups, P < 0.05.
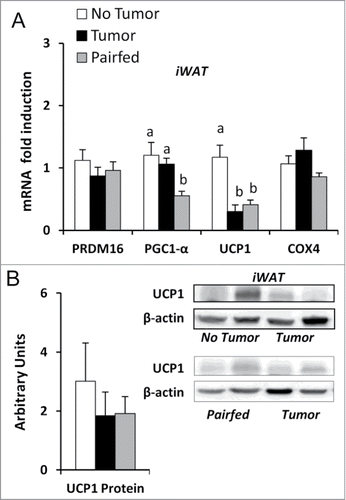
Colon-26 carcinoma increases markers of fatty acid oxidation in liver and muscle, not lipid accumulation in early cachexia
To further investigate whole body lipid utilization, we measured lipid accumulation and markers of fatty acid oxidation in liver and muscle. Total extracted hepatic lipids in the Tumor group were not different than in Pairfed and No Tumor groups in this early stage of cachexia (). Furthermore, gene expression of markers of fatty acid oxidation (ACOX1, CPT1α) were increased in Tumor mice compared to No Tumor mice () and coincided with elevated mRNA expression of the hepatic acute phase proteins fibrinogen and C-reactive protein (CRP) (). Livers were enlarged in Tumor mice compared only to Pairfed mice (). Similarly, in quadriceps muscle, total extracted lipids were not different among groups (). mRNA expression of LPL was increased in Tumor mice compared to Pairfed mice while pyruvate dehydrogenase kinase-4 (PDK4), the key gene involved in the switch from glucose to fatty acid utilizationCitation36 was significantly elevated in Tumor mice compared to both No Tumor and Pairfed mice ().
Figure 7. Liver/quadriceps lipid stores and gene expression (A) Total hepatic lipids, n = 10 per group and mRNA expression of genes related to fatty acid oxidation in liver, n = 9–10 per group. (B) mRNA expression of genes related to hepatic acute phase protein response, n = 10 per group. (C) Total quadriceps muscle lipids, n = 10 per group and mRNA expression of genes related to lipid metabolism in quadriceps, n = 8–10 per group. Values represent means ± s.e.m. Data were analyzed using one-way ANOVA with post hoc Fisher's test. Different letters indicate statistically significant differences among groups, P < 0.05.
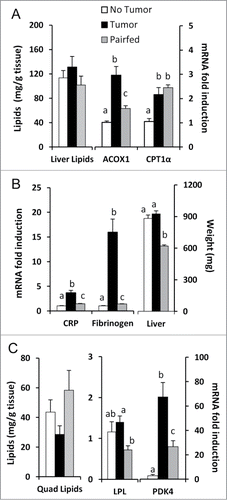
Discussion
Loss of adipose tissue in cancer cachexia has been intensely studied.Citation6,11-14,37,38 Mechanistic studies suggest PKA-induced lipolysis is not enhanced in late stage cachexia;Citation38 instead, increased expression of lipases may be responsible for lipid catabolism in adipose tissue in these more advanced stages of cachexia.Citation13,38 Studies have also reported enhanced lipid accumulation in muscle in cachectic patients with increased weight lossCitation29,39 and hepatic steatosis in fully-established cachexia in experimental tumor-bearing animals.Citation26,30 Lipid metabolism in early cachexia, when weight loss is less severe, has been minimally studied. In the present study, we investigated lipid metabolism in the colon-26 murine model of cancer cachexia at the onset of anorexia and weight loss. We report here that early cachexia is characterized by moderate reductions in adipose tissue mass, mild muscle wasting, and PKA-activated lipolysis without enhanced accumulation of hepatic and muscle lipids. Additionally, mice in the early phase of the cachexia trajectory exhibited abnormally elevated markers of thermogenesis in brown, but not white, adipose tissue and elevated energy expenditure when food intake was considered. Our observations suggest that different lipid metabolic responses may occur in different stagesCitation40 and/or models of cancer cachexia.Citation41
Studies of both early and late cachexia consistently demonstrate that reduced food intake alone cannot explain loss of body weightCitation5,20 or fat mass.Citation32,37,38 In the present study, by adjusting for tumor-free body weight, we also demonstrated that adipose mass in pair-fed mice is preferentially preserved in response to reduced food intake, a metabolic adjustment that does not occur in tumor-bearing mice. Healthy mammals compensate for reduced caloric intake by reducing resting energy expenditure and/or activity.Citation42-45 When food restrictions are mild, saved energy may be preferentially directed to fat stores at the expense of fat free mass.Citation44,45 Indeed, liver and muscle mass in pair-fed mice were reduced. Although cachectic mice significantly reduced their locomotor activity in our study, total energy expenditure in cachectic mice remained elevated compared to pair-fed mice. While differences in muscle work efficiency may have contributed to the differences in total energy expenditure,Citation46,47 these data suggest that resting energy expenditure in cachectic mice is abnormally elevated in early cachexia when food intake is taken into consideration.
Elevated total and resting energy expenditures have been reported in both human and rodent studies of cachexia.Citation12,20,48,49 Several mechanisms have been suggested to be responsible for this increase, including futile cycling, systemic inflammation, adrenergic stimulation, and brown adipose tissue thermogenesis.Citation50 In the present study of early cachexia, we show increased expression of genes related to BAT thermogenesis in cachectic mice compared only to pair-fed mice. Our results are subtly different with Tsoli et al who reported activation of BAT thermogenesis in anorectic C-26 mice in a later stage of cachexia relative to ad-libitum fed controls.Citation20 Thus, in early cachexia, markers of brown adipose thermogenesis are not increased per se (compared to ad-libitum fed controls), but rather these genes are not appropriately suppressed in response to reduced food intake. Markers of the hepatic acute phase protein response, on the other hand, were significantly elevated in our cachectic mice compared to both control groups in this study of early cachexia. Because this response is the first reaction to immunological stressCitation51 and is linked to cancer cachexia,Citation52,53 this finding is not surprising. The acute phase response is associated with significant increases in resting energy expenditureCitation49,54 which may explain the increase in body temperature in cachectic mice as early as day 3 of our study.
Although markers of BAT thermogenesis were abnormally elevated, we did not find evidence of “browning” of white adipose tissues in early cachexia. In theory, any signal that can sufficiently stimulate protein kinase A (PKA) pathways could induce brown-type adipocytes with thermogenic capacity in white adipose tissue.Citation55 While some markers of fatty acid oxidation were increased in eWAT, many markers of "browning" and mitochondrial electron transport in white adipose tissues of cachectic mice did not differ from control mice in our study of early cachexia. This contrasts with findings in late cachexia in the MAC-16 model of cachexia, where analysis of epididymal adipose tissue revealed remodeling reminiscent of brown-type adipocytes acco-mpanied by increased mRNA expression of PGC-1α.Citation37 Our findings are also in contrast with a recent study by Petruzzeli et al. that demonstrated a switch from white to brown fat in several different mouse models of cachexiaCitation56 where the change occurred before muscle wasting in some models (so called, initial stages of cachexia). However, in each of the studies, loss of gonadal and inguinal adipose tissue was severe (more than 50%).Citation56 Our study captured lipid metabolism when adipose tissue loss was less severe (approximately 30–40%); thus, in an earlier stage of adipose tissue depletion. In our study, tumor-bearing mice with greater adipose tissue loss tended to have higher UCP1 protein expression in eWAT (data not shown) compared to cachectic mice with less adipose tissue loss supporting the high variability of our data when averaged per group (). In iWAT a similar trend was noted although less uniform. However, even cachectic mice with relatively lower UCP1 protein expression incurred moderate losses of adipose tissue (data not shown) suggesting that notable wasting of adipose tissue may be required before WAT browning occurs in the colon-26 model.
Our data strongly suggest PKA-activated lipolysis in white adipose tissues is enhanced in mice during early cachexia. Lipolytic rates (unstimulated) and PKA-phosphorylated residues in eWAT of cachectic mice were consistent with pair-fed controls. This decidedly contrasts with a report of late stage cachexia where, unlike in pair-fed mice, PKA-induced lipolytic pathways were not activated in colon-26 tumor-bearing mice. Instead, increased expression of ATGL protein coupled with activation by 5'-AMP activated kinase (AMPK) was suggested to explain enhanced lipolysis.Citation38 We detected no significant difference in ATGL protein expression in our study of early cachexia although a trend to increase was noted. Stimulated lipolytic rates were not different among groups suggesting the non-significant increase in ATGL protein expression did not influence lipolytic rate in our study. Additionally, phosphorylated levels of AMPK were unchanged in our study (data not shown). Thus, in early cachexia, lipolysis may more closely resemble the normal metabolic response to an energy deficit. This conclusion and our results mirror one of the few fatty acid kinetic studies in cachectic cancer patients with low levels of metabolic stress. In this study, lipolytic rates of unstressed cachectic patients (perhaps analogous to early cachexia) were similar to nutritionally matched controls suggesting a commensurate and “normal” metabolic response to equivalent energy imbalances in cachectic patients and matched controls.Citation11 Interestingly, in this kinetic study and others,Citation57,58 body composition was also suggested to influence lipolytic rate, and we also found adiposity (eWAT mass) had a significant effect on unstimulated lipolysis in early cachexia.
Unlike reports in humans and other experimental models of progressed cachexia, lipid accumulation was not enhanced in liver and quadriceps muscle in our colon-26 tumor- bearing mice in early cachexia. Increased expression of genes related to fatty acid utilization and the absence of lipid accumulation in liver and quadriceps muscle suggests fatty acids were effectively oxidized to meet energy demands in these tissues. This contrasts with findings in humans where significant intramyocellular lipid accumulation occurs in later stages of cachexiaCitation29,39 which has been speculated to be a consequence of alterations to mitochondrial oxidative capacity found in advanced states of cachexia.Citation28 That ubiquitin-proteosome-dependent proteolysis is an ATP-consuming process may explain the increased expression of markers of lipid uptake and utilization in muscle in early cachexia when proteolysis, accompanied by anorexia, commences.
Our findings in liver also are in contrast to the few other studies of hepatic lipid metabolism in cachexia, albeit in late cachexia. SteatosisCitation26,30 and reductions in fatty acid oxidation/oxidative capacityCitation25,27,59 in the liver have been reported in the Walker 256 carcinosarcoma cachexia model when weight loss was substantial. In our study of early cachexia, markers of fatty acid oxidation were increased in liver and measurements of hepatic total lipids were not different among groups. Our findings are surprising given the strong association between the acute phase response and reduced hepatic fatty acid oxidation.Citation60 Although it is possible that the acute phase response was not as robust in our study of early cachexia, differences in cytokine production (thus, the acute phase response) between the colon-26 and Walker 256 cachexia modelsCitation61,62 may better explain these disparate findings.
In summary, in the present study we found PKA-induced lipolysis is enhanced in early cancer cachexia which coincided with elevated total energy expenditure and increased expression of markers of BAT thermogenesis when food intake is considered and the hepatic acute phase response. Lipid utilization in muscle and liver did not appear compromised in this early stage of colon-26 tumor-induced cachexia. That adipose tissue lipid metabolism was distinctly different from reports of later cachexia in the same model suggests studies of treatment interventions to preserve fat mass should be tailored to the stage of cachexia. Additionally, hepatic lipid metabolism in the colon-26 cachexia model may be different than other rodent models of cachexia because of tumor-type, species, and/or stage of cachexia. This warrants further investigation to delineate the contribution of cachexia stage and model to altered lipid metabolism in cancer cachexia.
Materials and Methods
Experimental animals and study design
Five- week-old male CD2F1 mice (BALB/c × DBA/2, Charles River Laboratories) weighing between 19 and 22 g were randomized by weight on study day 0 into one of 3 groups: No Tumor (n = 10), Tumor (n = 10), and Pairfed (n = 10). Mice were then injected subcutaneously into the right flank with 1.0 × 106 colon-26 carcinoma cells suspended in 100 μl PBS (Tumor group) or PBS only (No Tumor, Pairfed groups). We used the established colon-26 model of cachexia with young mice,Citation63 a model shown to induce similar effects in older mice.Citation64 Mice were housed individually at a temperature of 22 ± 0.5°C on a 12 h light (0600–1800 h) and 12 h dark (1800–0600 h) cycle and provided free access to water. Mice in the No Tumor and Tumor groups were fed ad-libitum with AIN-93G semi-purified pelleted diet (Research Diets). Pairfed mice were rationed the average amount of AIN-93G diet consumed by the Tumor group the day before. On study days 10 and 11, mice were housed in Comprehensive Lab Animal Monitoring System chambers (Columbus Instruments) at a temperature of 22 ± 0.5°C on a 12 h light/dark cycle for 24 h of indirect calorimetry measurements. Mice were sacrificed in the fed state on study day 12 (No Tumor, n = 5; Tumor, n = 10) and day 13 (No Tumor, n = 5 ; Pairfed, n = 10). Data from the Pairfed group were shifted back one day to ensure equivalent food intake for comparisons with Tumor mice. Mice were anesthetized with isoflurane and euthanized by cervical dislocation. Adipose tissues, quadriceps muscle, and liver were excised, weighed, frozen in liquid nitrogen, and then stored at −80°C until further analysis. Sections of eWAT were collected for fixation in 4% paraformaldehyde for paraffin embedding and for ex vivo lipolysis assays (see below). All study procedures were approved by the Institutional Animal Care and Use Committees at The Ohio State University.
Colon-26 carcinoma cell culture
Murine colon-26 (C-26) carcinoma cells were cultured in a growth media containing RPMI 1640 (Invitrogen) + L-glutamine (Sigma-Aldrich) supplemented with 5% fetal bovine serum (Invitrogen) and 1% Penicillin-Streptomycin (Invitrogen) at 37°C with 5% CO2. Cells were harvested at 80% confluence and resuspended in PBS at a concentration of 1.0 × 106/100 μl for injection.
Body weight, food intake, and rectal temperature measurements
Body weight, food intake, and rectal temperatures using a Thermalert TH-8 rodent rectal thermometer (Physitemp Instruments) were measured daily between 0830–0930 h.
RT- PCR
Total RNA was extracted from adipose tissue using the RNeasy® Lipid Tissue Mini Kit (Qiagen) according to manufacturer directions. Total RNA was extracted from liver and quadriceps muscle using Qiazol (Qiagen). RNA concentration was assessed using spectrophotometry by measuring absorbance at 260 nm (A260). RNA was reverse transcribed to cDNA with a High Capacity cDNA Archive Kit (Applied Biosystems). Using an Applied Biosystems 7300 instrument, cDNA was amplified via real-time PCR using predesigned primers and probes (TaqMan Gene Expression Assays, Applied Biosystems) for target and housekeeping genes. Target gene expression was normalized to 18S rRNA or GAPDH and expressed as 2-ΔΔCT relative to the No Tumor group.Citation65
Histology
Histology sections (5 μm) of epididymal white adipose tissues from each mouse were mounted on glass slides and stained with hematoxylin and eosin (H&E) by the Histology Core Laboratory, OSU College of Veterinary Medicine for analysis by microscopy. Images were captured using a light microscope (Olympus 1 × 50 microscope) with a Pixera Pro 150ES digital camera (Pixera). Microscopic fields (3–4 fields per slide) were randomly chosen by one evaluator for imaging. ImageJ software (NIH) was used by the same evaluator to quantify the cross-sectional area of 150 adipocytes for each mouse (9 mice per group).
Hepatic and muscle lipids
Total lipids were extracted from ∼25 and 65 mg sections of livers and muscle respectively from each mouse using the Folch method.Citation66 Lipids were extracted using a 2:1 v/v solution of chloroform and methanol and a 0.88% KCl solution wash. Samples were centrifuged at 1000 g to facilitate phase separation. The organic lower phase was transferred into a pre-weighed tube and then dried under nitrogen. After drying, tubes were reweighed to determine final total lipid weight per sample.
Ex-vivo lipolysis assay
Epididymal adipose tissue explants (∼30 mg) freshly isolated from each mouse during necropsy were incubated at 37°C in 600 μl of Krebs-Ringer bicarbonate HEPES buffer containing 3% fatty acid free BSA Fraction V (Calbiochem) with or without 10 μM isoproterenol (Calbiochem). After 3 h of incubation, aliquots were collected and assayed for glycerol content using the Free Glycerol Determination Kit (Sigma-Aldrich). Levels of glycerol were normalized to the weight of the explants.
Immunoblotting
Frozen adipose tissues were homogenized in lysis buffer composed of 20 mM Trizma base, 1% Triton-X100, 50 mM NaCl, 250 mM sucrose, 50 mM NaF, and 5 mM Na4P2O7 · 10H2O with the addition of Complete Mini Protease Inhibitor Cocktail Tablets (Roche Diagnostics) and PhosSTOP Phosphatase Inhibitor Cocktail Tablets (Roche Diagnostics). Homogenates were rocked for 1 h at 4°C and then centrifuged at 16.1k × g for 15 minutes at 4°C. Supernatant was collected and protein concentration was determined by BCA Protein Assay Kit (Pierce). Proteins analyzed were separated using sodium dodecylsulfate polyacrilamide gel electrophoresis and transferred to nitrocellulose membranes using Towbin transfer buffer (25 mM Tris, 192 mM glycine, and 20% methanol). Membranes were blocked with 5% non-fat dry milk in Tris Buffered Saline with 0.1% Tween-20 for 2 h and incubated overnight at 4oC with primary antibodies (Cell Signaling Technology and Abcam). After washing, membranes were incubated for 1 h at room temperature with HRP-linked anti-rabbit IgG, (Cell Signaling Technology). Bands were detected by chemiluminescence using SuperSignal West Femto Maximum Sensitivity Substrate (Thermoscientific) followed by exposure using Carestream Image Station 4000GL PRO (Carestream Molecular Imaging). Densiometric analysis was conducted using Carestream Molecular Imaging Software. β-actin was used as a loading control.
Indirect calorimetry
Six mice from each group were housed individually in Comprehensive Laboratory Animal Monitoring System chambers for metabolic measurements on days 10–11 (No Tumor, n=3 ; Tumor, n=6) and days 11–12 (No Tumor, n=3 ; Pairfed, =6). Measurements in Pairfed mice were performed the day after Tumor mice to ensure Pairfed mice were provided food rations equivalent to that consumed by the Tumor group during indirect calorimetry. Oxygen consumption rate (VO2), carbon dioxide production rate (VCO2), and locomotor activity (beam breaks) were collected every 20 minutes over 24 hours using CLAMS. Respiratory exchange ratio (RER) was calculated as the ratio of VCO2 to VO2. Energy expenditure was calculated as calorific value (CV) x VO2, where CV is 3.815 + 1.232 X RER.Citation67 To approximate energy balance, absolute energy expenditure was subtracted from the caloric value of food intake for each mouse. To determine the effect of cachexia on energy metabolism, energy expenditure data were analyzed by ANCOVA.Citation68
Statistics
Data are expressed as means ± s.e.m. Statistical analyses consisted of 2-tailed unpaired Student's t-test and one-way ANOVA with post-hoc Fisher's test when differences between groups were significant. Some measures were log-transformed for analysis to meet test assumptions: muscle MuRF1, Atrogin-1; iBAT LPL, COX4, DIO2; WAT phosphorylated HSL:HSL ratios; liver ACOX1, CRP, fibrinogen. However, untransformed means are presented for interpretability. Some data were analyzed using ANCOVA to remove the effects of confounding variables.Citation68 MINITAB 16, Microsoft Excel, and IBM SPSS v21 were used for data analysis. P-value < 0.05 was considered significant.
Disclosure of Potential Conflicts of Interest
No potential conflicts of interest were disclosed.
Acknowledgments
The authors would like to thank Dr. Santosh K Maurya and Dr. Muthu Periasamy for support and assistance with indirect calorimetry measurements.
Additional information
Funding
References
- Tisdale M. Mechanisms of cancer cachexia. Physiol Rev 2009; 89:381-410; PMID:19342610; http://dx.doi.org/10.1152/physrev.00016.2008
- Tan BH, Fearon KC. Cachexia: prevalence and impact in medicine. Curr Opin Clin Nutr Metab Care 2008; 11:400-7; PMID:18541999; http://dx.doi.org/10.1097/MCO.0b013e328300ecc1
- Fearon K, Strasser F, Anker SD, Bosaeus I, Bruera E, Fainsinger RL, Jatoi A, Loprinzi C, MacDonald N, Mantovani G, et al. Definition and classification of cancer cachexia: an international consensus. Lancet Oncol 2011; 12:489-95; PMID:21296615; http://dx.doi.org/10.1016/S1470-2045(10)70218-7
- Zhou X, Wang JL, Lu J, Song Y, Kwak KS, Jiao Q, Rosenfeld R, Chen Q, Boone T, Simonet WS, et al. Reversal of cancer cachexia and muscle wasting by ActRIIB antagonism leads to prolonged survival. Cell 2010; 142:531-43; PMID:20723755; http://dx.doi.org/10.1016/j.cell.2010.07.011
- Byerley LO, Lee SH, Redmann S, Culberson C, Clemens M, Lively MO. Evidence for a novel serum factor distinct from zinc α-2 glycoprotein that promotes body fat loss early in the development of cachexia. Nutr Cancer 2010; 62:484-94; PMID:20432169; http://dx.doi.org/10.1080/01635580903441220
- Murphy RA, Wilke MS, Perrine M, Pawlowicz M, Mourtzakis M, Lieffers JR, Maneshgar M, Bruera E, Clandinin MT, Baracos VE, et al. Loss of adipose tissue and plasma phospholipids: relationship to survival in advanced cancer patients. Clin Nutr 2010; 29:482-7; PMID:19959263; http://dx.doi.org/10.1016/j.clnu.2009.11.006
- Dalal S, Hui D, Bidaut L, Lem K, Del Fabbro E, Crane C, Reyes-Gibby CC, Bedi D, Bruera E. Relationships among body mass index, longitudinal body composition alterations, and survival in patients with locally advanced pancreatic cancer receiving chemoradiation: a pilot study. J Pain Symptom Manage 2012; 44:181-91; PMID:22695045; http://dx.doi.org/10.1016/j.jpainsymman.2011.09.010
- Di Sebastiano KM, Yang L, Zbuk K, Wong RK, Chow T, Koff D, Moran GR, Mourtzakis M. Accelerated muscle and adipose tissue loss may predict survival in pancreatic cancer patients: the relationship with diabetes and anaemia. Br J Nutr 2013; 109:302-12; PMID:23021109; http://dx.doi.org/10.1017/S0007114512001067
- Das SK, Eder S, Schauer S, Diwoky C, Temmel H, Guertl B, Gorkiewicz G, Tamilarasan KP, Kumari P, Trauner M, et al. Adipose triglyceride lipase contributes to cancer-associated cachexia. Science 2011; 333:233-8; PMID:21680814; http://dx.doi.org/10.1126/science.1198973
- Shaw JH, Wolfe RR. Fatty acid and glycerol kinetics in septic patients and in patients with gastrointestinal cancer. The response to glucose infusion and parenteral feeding. Ann Surg 1987; 205:368-76; PMID:3105476; http://dx.doi.org/10.1097/00000658-198704000-00005
- Klein S, Wolfe RR. Whole-body lipolysis and triglyceride-fatty acid cycling in cachectic patients with esophageal cancer. J Clin Invest 1990; 86:1403-8; PMID:2243120; http://dx.doi.org/10.1172/JCI114854
- Zuijdgeest-van Leeuwen SD, van den Berg JW, Wattimena JL, van der Gaast A, Swart GR, Wilson JH, Dagnelie PC. Lipolysis and lipid oxidation in weight-losing cancer patients and healthy subjects. Metabolism 2000; 49:931-6; PMID:10910006; http://dx.doi.org/10.1053/meta.2000.6740
- Agustsson T, Ryden M, Hoffstedt J, van Harmelen V, Dicker A, Laurencikiene J, Isaksson B, Permert J, Arner P. Mechanism of increased lipolysis in cancer cachexia. Cancer Res 2007; 67:5531-7; PMID:17545636; http://dx.doi.org/10.1158/0008-5472.CAN-06-4585
- Ryden M, Agustsson T, Laurencikiene J, Britton T, Sjolin E, Isaksson B, Permert J, Arner P. Lipolysis-not inflammation, cell death, or lipogenesis-is involved in adipose tissue loss in cancer cachexia. Cancer 2008; 113:1695-704; PMID:18704987; http://dx.doi.org/10.1002/cncr.23802
- Thompson MP, Koons JE, Tan ET, Grigor MR. Modified lipoprotein lipase activities, rates of lipogenesis, and lipolysis as factors leading to lipid depletion in C57BL mice bearing the preputial gland tumor, ESR-586. Cancer Res 1981; 41:3228-32; PMID:7248977
- Islam-Ali B, Khan S, Price SA, Tisdale MJ. Modulation of adipocyte G-protein expression in cancer cachexia by a lipid-mobilizing factor (LMF). Br J Cancer 2001; 85:758-63; PMID:11531264; http://dx.doi.org/10.1054/bjoc.2001.1992
- Korber J, Pricelius S, Heidrich M, Muller MJ. Increased lipid utilization in weight losing and weight stable cancer patients with normal body weight. Eur J Clin Nutr 1999; 53:740-5; PMID:10509772; http://dx.doi.org/10.1038/sj.ejcn.1600843
- Dahlman I, Mejhert N, Linder K, Agustsson T, Mutch DM, Kulyte A, Isaksson B, Permert J, Petrovic N, Nedergaard J, et al. Adipose tissue pathways involved in weight loss of cancer cachexia. Br J Cancer 2010; 102:1541-8; PMID:20407445; http://dx.doi.org/10.1038/sj.bjc.6605665
- Arruda AP, Milanski M, Romanatto T, Solon C, Coope A, Alberici LC, Festuccia WT, Hirabara SM, Ropelle E, Curi R, et al. Hypothalamic actions of tumor necrosis factor α provide the thermogenic core for the wastage syndrome in cachexia. Endocrinology 2010; 151:683-94; PMID:19996183; http://dx.doi.org/10.1210/en.2009-0865
- Tsoli M, Moore M, Burg D, Painter A, Taylor R, Lockie SH, Turner N, Warren A, Cooney G, Oldfield B, et al. Activation of thermogenesis in brown adipose tissue and dysregulated lipid metabolism associated with cancer cachexia in mice. Cancer Res 2012; 72:4372-82; PMID:22719069; http://dx.doi.org/10.1158/0008-5472.CAN-11-3536
- Jaworski K, Ahmadian M, Duncan RE, Sarkadi-Nagy E, Varady KA, Hellerstein MK, Lee HY, Samuel VT, Shulman GI, Kim KH, et al. AdPLA ablation increases lipolysis and prevents obesity induced by high-fat feeding or leptin deficiency. Nat Med 2009; 15:159-68; PMID:19136964; http://dx.doi.org/10.1038/nm.1904
- Ahmadian M, Duncan RE, Varady KA, Frasson D, Hellerstein MK, Birkenfeld AL, Samuel VT, Shulman GI, Wang Y, Kang C, et al. Adipose overexpression of desnutrin promotes fatty acid use and attenuates diet-induced obesity. Diabetes 2009; 58:855-66; PMID:19136649; http://dx.doi.org/10.2337/db08-1644
- Bordicchia M, Liu D, Amri EZ, Ailhaud G, Dessi-Fulgheri P, Zhang C, Takahashi N, Sarzani R, Collins S. Cardiac natriuretic peptides act via p38 MAPK to induce the brown fat thermogenic program in mouse and human adipocytes. J Clin Invest 2012; 122:1022-36; PMID:22307324; http://dx.doi.org/10.1172/JCI59701
- Yehuda-Shnaidman E, Buehrer B, Pi J, Kumar N, Collins S. Acute stimulation of white adipocyte respiration by PKA-induced lipolysis. Diabetes 2010; 59:2474-83; PMID:20682684; http://dx.doi.org/10.2337/db10-0245
- Kazantzis M, Seelaender MC. Cancer cachexia modifies the zonal distribution of lipid metabolism-related proteins in rat liver. Cell Tissue Res 2005; 321:419-27; PMID:16021474; http://dx.doi.org/10.1007/s00441-005-1138-0
- Silverio R, Laviano A, Rossi Fanelli F, Seelaender M. L-Carnitine induces recovery of liver lipid metabolism in cancer cachexia. Amino Acids 2012; 42:1783-92; PMID:21465256; http://dx.doi.org/10.1007/s00726-011-0898-y
- Siddiqui RA, Williams JF. The regulation of fatty acid and branched-chain amino acid oxidation in cancer cachectic rats: a proposed role for a cytokine, eicosanoid, and hormone trilogy. Biochem Med Metab Biol 1989; 42:71-86; PMID:2775564; http://dx.doi.org/10.1016/0885-4505(89)90043-1
- Julienne CM, Dumas JF, Goupille C, Pinault M, Berri C, Collin A, Tesseraud S, Couet C, Servais S. Cancer cachexia is associated with a decrease in skeletal muscle mitochondrial oxidative capacities without alteration of ATP production efficiency. J Cachexia Sarcopenia Muscle 2012; 3:265-75; PMID:22648737; http://dx.doi.org/10.1007/s13539-012-0071-9
- Weber MA, Krakowski-Roosen H, Schroder L, Kinscherf R, Krix M, Kopp-Schneider A, Essig M, Bachert P, Kauczor HU, Hildebrandt W. Morphology, metabolism, microcirculation, and strength of skeletal muscles in cancer-related cachexia. Acta Oncol 2009; 48:116-24; PMID:18607877; http://dx.doi.org/10.1080/02841860802130001
- Seelaender MC, Nascimento CM, Curi R, Williams JF. Studies on the lipid metabolism of Walker 256 tumour-bearing rats during the development of cancer cachexia. Biochem Mol Biol Int 1996; 39:1037-47; PMID:8866022
- Asp ML, Tian M, Kliewer KL, Belury MA. Rosiglitazone delayed weight loss and anorexia while attenuating adipose depletion in mice with cancer cachexia. Cancer Biol Ther 2011; 12:957-65; PMID:22104958; http://dx.doi.org/10.4161/cbt.12.11.18134
- Tian M, Kliewer KL, Asp ML, Stout MB, Belury MA. c9t11-Conjugated linoleic acid-rich oil fails to attenuate wasting in colon-26 tumor-induced late-stage cancer cachexia in male CD2F1 mice. Mol Nutr Food Res 2011; 55:268-77; PMID:20827675; http://dx.doi.org/10.1002/mnfr.201000176
- Bing C. Lipid mobilization in cachexia: mechanisms and mediators. Curr Opin Support Palliat Care 2011; 5:356-60; PMID:21934502; http://dx.doi.org/10.1097/SPC.0b013e32834bde0e
- Festuccia WT, Guerra-Sa R, Kawashita NH, Garofalo MA, Evangelista EA, Rodrigues V, Kettelhut IC, Migliorini RH. Expression of glycerokinase in brown adipose tissue is stimulated by the sympathetic nervous system. Am J Physiol Regul Integr Comp Physiol 2003; 284:R1536-41; PMID:12736183
- Bing C, Brown M, King P, Collins P, Tisdale MJ, Williams G. Increased gene expression of brown fat uncoupling protein (UCP)1 and skeletal muscle UCP2 and UCP3 in MAC16-induced cancer cachexia. Cancer Res 2000; 60:2405-10; PMID:10811117
- Wu P, Inskeep K, Bowker-Kinley MM, Popov KM, Harris RA. Mechanism responsible for inactivation of skeletal muscle pyruvate dehydrogenase complex in starvation and diabetes. Diabetes 1999; 48:1593-9; PMID:10426378; http://dx.doi.org/10.2337/diabetes.48.8.1593
- Bing C, Russell S, Becket E, Pope M, Tisdale MJ, Trayhurn P, Jenkins JR. Adipose atrophy in cancer cachexia: morphologic and molecular analysis of adipose tissue in tumour-bearing mice. Br J Cancer 2006; 95:1028-37; PMID:17047651; http://dx.doi.org/10.1038/sj.bjc.6603360
- Tsoli M, Schweiger M, Vanniasinghe AS, Painter A, Zechner R, Clarke S, Robertson G. Depletion of white adipose tissue in cancer cachexia syndrome is associated with inflammatory signaling and disrupted circadian regulation. PLoS One 2014; 9:e92966; PMID:24667661; http://dx.doi.org/10.1371/journal.pone.0092966
- Stephens NA, Skipworth RJ, Macdonald AJ, Greig CA, Ross JA, Fearon KC. Intramyocellular lipid droplets increase with progression of cachexia in cancer patients. J Cachexia Sarcopenia Muscle 2011; 2:111-7; PMID:21766057; http://dx.doi.org/10.1007/s13539-011-0030-x
- Blum D, Stene GB, Solheim TS, Fayers P, Hjermstad MJ, Baracos VE, Fearon K, Strasser F, Kaasa S. Validation of the consensus-definition for cancer cachexia and evaluation of a classification model-a study based on data from an international multicentre project (EPCRC-CSA). Ann Oncol 2014; 25:1635-42; PMID:24562443; http://dx.doi.org/10.1093/annonc/mdu086
- Kir S, White JP, Kleiner S, Kazak L, Cohen P, Baracos VE, Spiegelman BM. Tumour-derived PTH-related protein triggers adipose tissue browning and cancer cachexia. Nature 2014; 513:100-4; PMID:25043053; http://dx.doi.org/10.1038/nature13528
- Maclean PS, Bergouignan A, Cornier MA, Jackman MR. Biology's response to dieting: the impetus for weight regain. Am J Physiol Regul Integr Comp Physiol 2011; 301:R581-600; PMID:21677272; http://dx.doi.org/10.1152/ajpregu.00755.2010
- Hambly C, Simpson CA, McIntosh S, Duncan JS, Dalgleish GD, Speakman JR. Calorie-restricted mice that gorge show less ability to compensate for reduced energy intake. Physiol Behav 2007; 92:985-92; PMID:17706730; http://dx.doi.org/10.1016/j.physbeh.2007.07.005
- Hambly C, Speakman JR. Contribution of different mechanisms to compensation for energy restriction in the mouse. Obes Res 2005; 13:1548-57; PMID:16222057; http://dx.doi.org/10.1038/oby.2005.190
- Li X, Cope MB, Johnson MS, Smith DL, Jr., Nagy TR. Mild calorie restriction induces fat accumulation in female C57BL/6J mice. Obesity (Silver Spring) 2010; 18:456-62; PMID:19798071; http://dx.doi.org/10.1038/oby.2009.312
- Goldsmith R, Joanisse DR, Gallagher D, Pavlovich K, Shamoon E, Leibel RL, Rosenbaum M. Effects of experimental weight perturbation on skeletal muscle work efficiency, fuel utilization, and biochemistry in human subjects. Am J Physiol Regul Integr Comp Physiol 2010; 298:R79-88; PMID:19889869; http://dx.doi.org/10.1152/ajpregu.00053.2009
- Rosenbaum M, Vandenborne K, Goldsmith R, Simoneau JA, Heymsfield S, Joanisse DR, Hirsch J, Murphy E, Matthews D, Segal KR, et al. Effects of experimental weight perturbation on skeletal muscle work efficiency in human subjects. Am J Physiol Regul Integr Comp Physiol 2003; 285:R183-92; PMID:12609816
- Hyltander A, Daneryd P, Sandstrom R, Korner U, Lundholm K. Beta-adrenoceptor activity and resting energy metabolism in weight losing cancer patients. Eur J Cancer 2000; 36:330-4; PMID:10708933; http://dx.doi.org/10.1016/S0959-8049(99)00273-7
- Falconer JS, Fearon KC, Plester CE, Ross JA, Carter DC. Cytokines, the acute-phase response, and resting energy expenditure in cachectic patients with pancreatic cancer. Ann Surg 1994; 219:325-31; PMID:7512810; http://dx.doi.org/10.1097/00000658-199404000-00001
- Fearon KC, Glass DJ, Guttridge DC. Cancer cachexia: mediators, signaling, and metabolic pathways. Cell Metab 2012; 16:153-66; PMID:22795476; http://dx.doi.org/10.1016/j.cmet.2012.06.011
- Cray C, Zaias J, Altman NH. Acute phase response in animals: a review. Comp Med 2009; 59:517-26; PMID:20034426
- Stephens NA, Skipworth RJ, Fearon KC. Cachexia, survival and the acute phase response. Curr Opin Support Palliat Care 2008; 2:267-74; PMID:19060563; http://dx.doi.org/10.1097/SPC.0b013e3283186be2
- Falconer JS, Fearon KC, Ross JA, Elton R, Wigmore SJ, Garden OJ, Carter DC. Acute-phase protein response and survival duration of patients with pancreatic cancer. Cancer 1995; 75:2077-82; PMID:7535184; http://dx.doi.org/10.1002/1097-0142(19950415)75:8%3c2077::AID-CNCR2820750808%3e3.0.CO;2-9
- Wigmore SJ, Falconer JS, Plester CE, Ross JA, Maingay JP, Carter DC, Fearon KC. Ibuprofen reduces energy expenditure and acute-phase protein production compared with placebo in pancreatic cancer patients. Br J Cancer 1995; 72:185-8; PMID:7541236; http://dx.doi.org/10.1038/bjc.1995.300
- Bartelt A, Heeren J. Adipose tissue browning and metabolic health. Nat Rev Endocrinol 2014; 10:24-36; PMID:24146030; http://dx.doi.org/10.1038/nrendo.2013.204
- Petruzzelli M, Schweiger M, Schreiber R, Campos-Olivas R, Tsoli M, Allen J, Swarbrick M, Rose-John S, Rincon M, Robertson G, et al. A Switch from White to Brown Fat Increases Energy Expenditure in Cancer-Associated Cachexia. Cell Metab 2014; 20:433-47; PMID:25043816; http://dx.doi.org/10.1016/j.cmet.2014.06.011
- Klein S, Young VR, Blackburn GL, Bistrian BR, Wolfe RR. The impact of body composition on the regulation of lipolysis during short-term fasting. J Am Coll Nutr 1988; 7:77-84; PMID:3343478; http://dx.doi.org/10.1080/07315724.1988.10720223
- Mittendorfer B, Magkos F, Fabbrini E, Mohammed BS, Klein S. Relationship between body fat mass and free fatty acid kinetics in men and women. Obesity (Silver Spring) 2009; 17:1872-7; PMID:19629053; http://dx.doi.org/10.1038/oby.2009.224
- Seelaender MC, Curi R, Colquhoun A, Williams JF, Zammitt VA. Carnitine palmitoyltransferase II activity is decreased in liver mitochondria of cachectic rats bearing the Walker 256 carcinosarcoma: effect of indomethacin treatment. Biochem Mol Biol Int 1998; 44:185-93; PMID:9503162
- Nachiappan V, Curtiss D, Corkey BE, Kilpatrick L. Cytokines inhibit fatty acid oxidation in isolated rat hepatocytes: synergy among TNF, IL-6, and IL-1. Shock 1994; 1:123-9; PMID:7749930; http://dx.doi.org/10.1097/00024382-199402000-00007
- Rebeca R, Bracht L, Noleto GR, Martinez GR, Cadena SM, Carnieri EG, Rocha ME, de Oliveira MB. Production of cachexia mediators by Walker 256 cells from ascitic tumors. Cell Biochem Funct 2008; 26:731-8; PMID:18646274; http://dx.doi.org/10.1002/cbf.1497
- Yasumoto K, Mukaida N, Harada A, Kuno K, Akiyama M, Nakashima E, Fujioka N, Mai M, Kasahara T, Fujimoto-Ouchi K, et al. Molecular analysis of the cytokine network involved in cachexia in colon 26 adenocarcinoma-bearing mice. Cancer Res 1995; 55:921-7; PMID:7850809
- Tanaka Y, Eda H, Tanaka T, Udagawa T, Ishikawa T, Horii I, Ishitsuka H, Kataoka T, Taguchi T. Experimental cancer cachexia induced by transplantable colon 26 adenocarcinoma in mice. Cancer Res 1990; 50:2290-5; PMID:2317817
- Talbert EE, Metzger GA, He WA, Guttridge DC. Modeling human cancer cachexia in colon 26 tumor-bearing adult mice. J Cachexia Sarcopenia Muscle 2014; PMID:24668658
- Livak KJ, Schmittgen TD. Analysis of relative gene expression data using real-time quantitative PCR and the 2(-Delta Delta C(T)) Method. Methods 2001; 25:402-8; PMID:11846609; http://dx.doi.org/10.1006/meth.2001.1262
- Folch J, Lees M, Sloane Stanley GH. A simple method for the isolation and purification of total lipides from animal tissues. J Biol Chem 1957; 226:497-509; PMID:13428781
- McLean J, Tobin G. Animal and Human Calorimetry. Cambridge UK: Cambridge University Press, 1987
- Tschop MH, Speakman JR, Arch JR, Auwerx J, Bruning JC, Chan L, Eckel RH, Farese RV, Jr., Galgani JE, Hambly C, et al. A guide to analysis of mouse energy metabolism. Nat Methods 2012; 9:57-63; PMID:22205519; http://dx.doi.org/10.1038/nmeth.1806