Abstract
Trimethylamine (TMA) is produced by gut bacteria from dietary ingredients. In individuals with a hereditary defect in flavin-containing monooxygenase 3, bacterial TMA production is believed to contribute to the symptoms of trimethylaminuria (TMAU; fish-odor syndrome). Intestinal microbiota TMA metabolism may also modulate atherosclerosis risk by affecting trimethylamine oxide (TMAO) production levels. We propose that reducing TMA formation in the gut by converting it to an inert molecule could be used to prevent or limit these human diseases, while avoiding the major drawbacks of other clinical interventions. Reducing TMA levels by microbiological interventions could also be helpful in some vaginoses. Particular members of a recently discovered group of methanogens, that are variably present in the human gut, are unusual in being apparently restricted to utilizing only methyl compounds including TMA as substrates. We confirmed experimentally that one of these strains tested, Methanomassiliicoccus luminyensis B10, is able to deplete TMA, by reducing it with H2 for methanogenesis. We therefore suggest that members of this archaeal lineage could be used as treatments for metabolic disorders.
The Gut Origin of TMA in Human
The tertiary amine trimethylamine (TMA) is a volatile compound which has a characteristic fishy odor, and is formed by bacterial reduction of trimethylamine oxide (TMAO). This alkylamine is detected as unpleasant by the human olfactory system at even very low levels, thereby preventing humans from ingesting rotting fish. Ironically, this molecule is also endogenous in humans, being synthesized in the gut and sometimes in the vagina by the endogenous microbiota. The microbiome of the gastrointestinal tract is better characterized than that of other body sites, so only this niche will be discussed here, although relevant metabolic processes in the vagina are probably similar.
In the gut, TMA is formed by microbial conversion of dietary ingredientsCitation1 and is further absorbed before being oxidized into TMAO in the liver (see ). Such TMA precursors include TMAO (in abundance in seafood), and choline (eggs, soybean, cauliflower), which is likely the most important TMA source. Recent data also show that L-carnitineCitation2 found in red meat (and in some energy drinks and dietary supplements) is converted into TMA. The metabolic pathways leading to TMA are thought to be exclusively microbial in humans: the mechanism of conversion of choline by an anaerobic mammalian gut Desulfovibrio has recently been identified and relies on the cut (choline utilization) operon, in which cutC encodes a glycyl radical enzyme with a choline trimethylamine-lyase activity.Citation3 Choline is an important factor for human health, with an adequate intake for adults of 425 mg and 550 mg per day being recommended for women and men respectively in the US.Citation4 Choline depletion resulting from a choline-deficient diet causes clinical consequences such as non-alcoholic fatty-liver disease (NAFLD) and muscle damage.Citation5,Citation6 Dietary lecithin (phosphatidylcholine, PC) also feeds into plasma TMAO levels by gut conversion into choline (and then to TMA), as shown by mouse studies.Citation7 Thus, limiting intake of choline or its precursors to reduce TMA production is not feasible. However such a reduction of TMA levels is highly desirable because TMA is related to at least two disorders.
Figure 1. Origin and fate of TMA in the human gut, and the Archaebiotics concept. Gut microbiota synthesis of TMA is realized from TMAO, choline, PC and L-carnitine. The TMA is then absorbed and goes to the liver, routes (A or (B). In the case of route (A), a partial or total defect in FMO3-oxidation into TMAO leads to increased level and diffusion of TMA in breath, urine and sweat. When FMO3 liver oxidation is functional (B), the increase of TMAO in blood is associated with atherosclerosis.Citation2,Citation7,Citation11 Therefore, converting TMA directly in the gut using Archaebiotics belonging to the seventh methanogenic order, naturally-occurring in the gut, route (C) should be envisaged. Interestingly, these archaea are only able to perform methanogenesis using methyl compounds (see ), because the two other pathways are absent (CO2 reduction with H2 and aceticlastic pathway): this would increase the efficiency of TMA conversion.
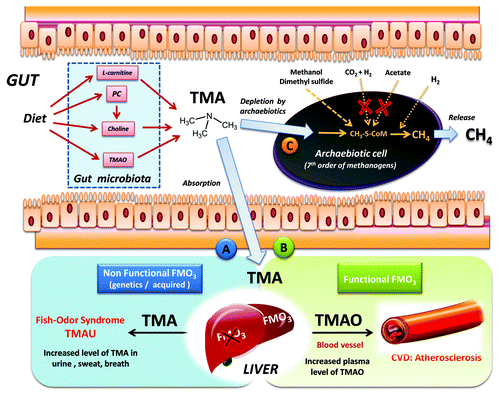
TMA is Implicated in Disease
Trimethylaminuria (TMAU) is caused by a metabolic anomaly whereby sufferers emit a pervasive fishy odor caused by the excretion of TMA in their breath, sweat and urine. As TMAU is still under-recognized and often goes undiagnosed, those affected often suffer from psychological problems and social stress.Citation8 Although no large prevalence studies are currently available, people with compromised ability to N-oxidize trimethylamine into the odorless TMAO are probably common.Citation9 TMAU can be classified into a primary genetic form and an acquired (or secondary) form. The genetic form is now well characterized with approximately 15% of single nucleotide polymorphisms (SNPs) in the FMO3 gene (encoding a flavin-containing monooxygenase) associated with either lack of or diminished enzymatic oxidation in the liver of TMA into TMAO.Citation9 In the healthy individual, more than 95% of TMA is oxidized into TMAO in this way, while genetic defects in FMO3 result in accumulation of TMA in blood, and then in breath, sweat and urine (, pathway A). Acquired TMAU, sometimes transient, includes conditions featuring elevated urinary levels of TMA due to dietary and hormonal factors in combination with enzymatic activity and metabolism in the gut. Important examples include transient menstrual TMAU, overload of dietary precursors of TMA (see above), and impaired hepatic function.Citation10 Treatment relies mainly on an empirical dietary approach, or several complementary therapeutic adjuncts, including soaps which limit the volatility of TMA from the skin, together with brief courses of antimicrobials (neomycin, metronidazole) which are not always effective.Citation10 This last approach limits the bacterial load, and in extenso, gut conversion of TMAO, choline and derivatives into TMA.
Gut microbiota metabolism of phosphatidylcholine (PC) and L-carnitine can promote atherosclerosis.Citation2,Citation7,Citation11 This is because their plasma metabolites (choline, betaine and TMAO) are risk factors for cardiovascular disease (CVD) in humans. Experimentally, dietary choline supplementation has been shown in a seminal paper to significantly enhance aortic lesion area in mice genetically prone to atherosclerosis, as did supplementation of the diet with TMAO.Citation7 Furthermore, gut microbiota depletion by antibiotics led to inhibition of the dietary choline-induced cardiovascular effects. Mice with depleted gut microbiota did not experience increased TMAO plasma levelsCitation7 (, pathway B) nor did humans.Citation11 Human gut microbiota is also required to form TMAO from L-carnitine, and plasma TMAO is likely the primary driver of cardiovascular risks rather than L-carnitine itself.Citation2
A fishy odor frequently occurs in bacterial vaginosis.Citation12 It is important to stress that, in this instance, TMA does not originate from urine, but rather from in situ production of TMA by the vaginal microbiota through a process similar to that which occurs in the gut.
Hypothesis: That TMA Could Be Depleted as it is Synthesized
We were prompted by recent microbiology studies to ask if TMA could be depleted in vivo by bioconversion into a molecule with no undesirable properties. The underlying biochemistry was described more than 35 y ago in the rumen of cows, where choline is metabolized via TMA and onwards into methane.Citation13 Rumen microbes like the methanogenic archaea Methanosarcina barkeri metabolize methyl compounds, including TMA, to methane for growth.Citation14 A distinct archaeal group (the Rumen Cluster C; RCC) was subsequently identified as being putatively able to convert methyl compounds including TMA and methylamines into methane. Half the human subjects examined to date also produce methane, usually by harboring the methanogen Methanobrevibacter smithii, or less frequently, Methanosphaera stadtmanae (reviewed in ref. Citation15). It is also noteworthy that M. smithii has been identified in vaginal samples.Citation15 We thus asked if methylotrophic methanogens would be able to survive and deplete TMA in the human gut (or in the vagina), a notion that would be greatly supported if methanogens that naturally occur in the human gut could metabolize TMA.
The trigger for proposing this hypothesis now is that, until recently, only the methanogens M. smithii and M. stadtmanae were recognized as residents of the human gut. They belong to the order Methanobacteriales, one of the six known orders of methanogens. However in 2008, the existence of a seventh order inhabiting the human gut (hereafter referred to as the Mx-lineage) was proposed,Citation16 particularly common in older subjects,Citation17 and Methanomassiliicoccus luminyensis, the first and unique isolated member of this order metabolizes methanol with hydrogen for methane production.Citation18 Genomic sequences of three of these unusual methanogens are currently available, that of M. luminyensis,Citation19 and genome sequences that we recently determined for “Candidatus Methanomethylophilus alvus”Citation20 and “Candidatus Methanomassiliicoccus intestinalis”Citation21 grown in an enrichment culture from human stool. “Ca. Methanomethylophilus alvus” belongs to the RCC cluster that was recently highlighted for its putative involvement in TMA consumption in the rumen,Citation23 while M. luminyensis and “Ca. Methanomassiliicoccus intestinalis” are phylogenetically closely related to the RCC cluster.Citation24 These three genomes have all of the genes necessary for reduction of methanol using hydrogen, but also for the reduction of tri- di- and monomethylamine with hydrogen (), while the two other possible pathways for methanogenesis (CO2 reduction with H2 and acetoclastic methanogenesis) are genetically incomplete. Whereas the capacity of these strains to reduce methanol with hydrogen was previously demonstrated, their ability to grow on TMA with hydrogen has not been investigated. We therefore tested this hypothesis on the sole strain of this group available in pure culture, M. luminyensis B10, and thus confirmed for the first time that this strain is able to grow on TMA with hydrogen (). M. luminyensis B10 was also able to grow on the by-products of TMA catabolism, dimethylamine and then monomethylamine, both being used with hydrogen for methanogenesis (data not shown). These results are in agreement with the annotated genetic complement of this strain and strongly suggest the same metabolic capacity in “Ca. M. alvus” and “Ca. M. intestinalis” because they harbor orthologs of all the relevant M. luminyensis genes involved in these pathways. Collectively this suggests to us that natural methanogenic inhabitants of the human gut will be able to metabolize TMA, and could deplete this metabolite as it is formed by bacterial elements of the microbiota.
Figure 2. Metabolic pathway of TMA conversion deduced from genomic data from species of the 7th order of methanogens. The pathway of TMA conversion involves a trimethylamine:corrinoid methyltransferase (MttB) which contains a pyrrolysine, an unusual amino-acid. The synthesis of this amino-acid and its transfer on a tRNAPyl to form a pyrrolysyl-tRNAPyl, and its insertion during the translation of mttB are presented in the green part. The pathway of trimethylamine (TMA) conversion, involving the pyrrolysine containing MttB, is presented in the orange part. Steps in the pathway between named compounds are indicated by blue arrows. The purple arrow indicates the transcription and translation of mttB. The dotted arrows indicate steps that are not detailed for the clarity of the scheme. Recognized enzyme names (see KEGG map 00680) are provided, flanked by the corresponding protein accession numbers in the “Ca. M. alvus” (blue) and “Ca. M. intestinalis” (red) genomes. Only accession numbers of enzymes specifically involved in the depletion of TMA are presented, some being shared with dimethylamine (DMA) and monomethylamine (MMA) depletion (not shown here).
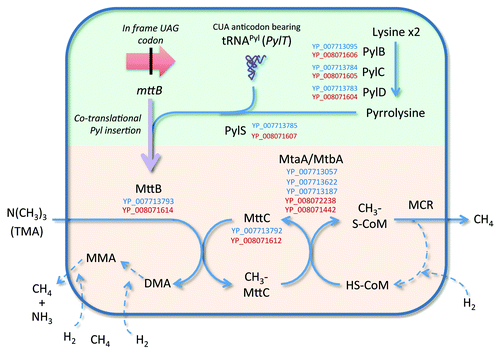
Figure 3. Growth of Methanomassiliicoccus luminyensis strain B10 on trimethylamine (TMA) and hydrogen (H2). (A) The growth of M. luminyensis B10 on methanol (M, 50 mM) and H2 (previously described by Dridi et al.Citation18) was used as a positive control for growth and maximal cell density. (B) We now show that M. luminyensis B10 also grows on TMA (15 mM) in the presence of H2 (filled diamonds) but not on TMA in the absence of H2 (open diamonds). Accordingly, TMA is depleted in presence of H2 (filled triangles) and not in the absence of H2 (open triangles). The composition of the gas phase was measured at the end of the experiment (C) and revealed the production of methane (CH4) and the depletion of H2 in presence of either M or TMA. No CH4 was produced in the absence of H2 and CH4 was not produced nor H2 depleted in the absence of the methylated compound substrate (M or TMA). M. luminyensis B10 was obtained from DSMZ (DSM No. 25720) and cultivated in DSMZ medium 119, with rumen fluid replacing the sludge fluid. When H2 was present, initial atmosphere composition was: N2/H2/CO2 (55:35:10), and in absence of H2: N2/CO2 (75:25). Growth in each condition was performed in triplicate. Trimethylamine concentration was measured as described by Krätzer et al.Citation30 OD, Optical Density.
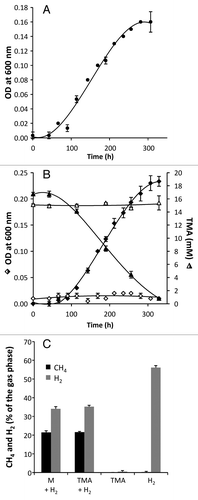
Proposal: The Archaebiotics Concept
Drawing together these ecological and biochemical strands, we now propose the Archaebiotics Concept viz. the intestinal application of specific archaeal strains for the treatment of human diseases including cardiovascular disease and TMAU (). The fishy-odor aspect of some vaginal conditions could be addressed using pessary delivery. Focusing on CVD and TMAU, there are several advantages. Methane, the TMA-metabolite produced by these archaea is usually considered biologically inert in humans. The pathway and relevant coding sequences for catalyzing TMA conversion to methane by “Ca. Methanomethylophilus alvus” and “Ca. Methanomassiliicoccus intestinalis” based on annotation of the genomes we recently sequenced is shown in ; a similar pathway is inferred from the M. luminyensis genome. As for other methanogenic archaea, it includes a methyltransferase MttB with a predicted pyrrolysine residue,Citation22 which is associated with its synthetic machinery. The fact that these strains were isolated from humans might facilitate regulatory compliance. M. luminyensis like M. smithii is susceptible to bacitracin, metronidazole, ordinazole and squalamine,Citation25 providing the reassurance of contingency eradication. An alternative to administering live cells might be therapeutic administration of the relevant enzymes (), all of which are also encoded by M. luminyensis.
Paralleling the experience with the development of probiotic bacterial strains for human consumption, it has been shown that particular archaeal methanogen strains are likely to be more suitable for clinical use in this application. For example, we established that some strains seem to be evolutionarily more adapted to the human gut ecosystem, harboring genes predicted to confer resistance to bile salts (bile salt hydrolases occur in “Ca. Methanomethylophilus alvus” species, but not in M. luminyensis nor in “Ca. Methanomassiliicoccus intestinalis”). Consideration of factors other than TMA depletion alone is thus appropriate (see also below).
Challenges for Therapeutic Usage for Archaea to Deplete TMA
Technological hurdles to overcome will include establishing the best way to deliver oxygen-sensitive microorganisms into the human gut, and whether or not this administration will lead to cell levels that are sufficient to allow clinically relevant TMA depletion. Continuous administrations may be necessary if subjects do not become colonized. There are additional biological challenges; will these strains be able to effectively deplete TMA in the gut? Are the ensuing risk reductions for cardiovascular disease achieved, as they were by antibiotic therapy to limit TMA production?Citation11 It is also important to concede that archaeal abundance is not uniformly associated with increased health in mammals; for example, Bacteroides thetaiotaomicron-M. smithii co-colonization of germ-free mice increased host adiposity.Citation26 Archaea in general appear to be more abundant in native Africans than in African Americans, likely indicating dietary influences on the microbiota.Citation27,Citation28 Diet could conceivably be controlled or modulated to promote methanogen levels in recipients of archaebiotics. We cannot currently explain why the Mx-lineage of Archaea appears from the literature to be more abundant in older subjects, so we are determining fecal archaeal levels in the ELDERMET cohort subjects, that are well phenotyped for diet, health and bacterial microbiota.Citation29 A possible factor is the trophic interaction with other microbiota elements, and that a more favorable ecology emerges in some older subjects. This raises the provocative issue of what the effects of introducing a methanogenic archaea might be on the microbiota of the recipient. The Mx-methanogens are very likely not acetoclastic but would compete with other hydrogenotrophic archaea and bacteria, so the downstream effects on microbiome, metabolome and host physiology would need detailed exploration in vitro, in animal models, and ultimately in humans. Although the application of archaebiotics as proposed here faces significant challenges, we contend that the anticipated benefit of their use to mitigate the life-time risk of CVD and to treat TMAU warrants a concerted research effort in this area by the scientific community.
Acknowledgments
This work was supported by two PhD. Scholarship supports, one from the French “Ministère de l’Enseignement Supérieur et de la Recherche” to NG, the other of the European Union (UE) and the Auvergne Council to WT (FEDER). PWOT was supported by Science Foundation Ireland through a Principal Investigator award and by an FHRI award to the ELDERMET project by the Dept. Agriculture, Fisheries and Marine of the Government of Ireland. We thank F Shanahan and EMM Quigley for helpful critique of the manuscript. In Memoriam to Albert Jacquard (b. 12-23-1925, † 09-11-2013), geneticist and great humanist.
Disclosure of Potential Conflict of Interests
No potential conflict of interest was disclosed.
References
- Zhang AQ, Mitchell SC, Smith RL. Dietary precursors of trimethylamine in man: a pilot study. Food Chem Toxicol 1999; 37:515 - 20; http://dx.doi.org/10.1016/S0278-6915(99)00028-9; PMID: 10456680
- Koeth RA, Wang Z, Levison BS, Buffa JA, Org E, Sheehy BT, Britt EB, Fu X, Wu Y, Li L, et al. Intestinal microbiota metabolism of L-carnitine, a nutrient in red meat, promotes atherosclerosis. Nat Med 2013; 19:576 - 85; http://dx.doi.org/10.1038/nm.3145; PMID: 23563705
- Craciun S, Balskus EP. Microbial conversion of choline to trimethylamine requires a glycyl radical enzyme. Proc Natl Acad Sci U S A 2012; 109:21307 - 12; http://dx.doi.org/10.1073/pnas.1215689109; PMID: 23151509
- Institute of Medicine (U.S.). Standing Committee on the Scientific Evaluation of Dietary Reference Intakes., Institute of Medicine (U.S.). Panel on Folate Other B Vitamins and Choline., Institute of Medicine (U.S.). Subcommittee on Upper Reference Levels of Nutrients. Dietary reference intakes for thiamin, riboflavin, niacin, vitamin B6, folate, vitamin B12, pantothenic acid, biotin, and choline. Washington, D.C.: National Academy Press, 1998.
- Buchman AL, Dubin MD, Moukarzel AA, Jenden DJ, Roch M, Rice KM, Gornbein J, Ament ME. Choline deficiency: a cause of hepatic steatosis during parenteral nutrition that can be reversed with intravenous choline supplementation. Hepatology 1995; 22:1399 - 403; PMID: 7590654
- Spencer MD, Hamp TJ, Reid RW, Fischer LM, Zeisel SH, Fodor AA. Association between composition of the human gastrointestinal microbiome and development of fatty liver with choline deficiency. Gastroenterology 2011; 140:976 - 86; http://dx.doi.org/10.1053/j.gastro.2010.11.049; PMID: 21129376
- Wang Z, Klipfell E, Bennett BJ, Koeth R, Levison BS, Dugar B, Feldstein AE, Britt EB, Fu X, Chung YM, et al. Gut flora metabolism of phosphatidylcholine promotes cardiovascular disease. Nature 2011; 472:57 - 63; http://dx.doi.org/10.1038/nature09922; PMID: 21475195
- Ayesh R, Mitchell SC, Zhang A, Smith RL. The fish odour syndrome: biochemical, familial, and clinical aspects. BMJ 1993; 307:655 - 7; http://dx.doi.org/10.1136/bmj.307.6905.655; PMID: 8401051
- D’Angelo R, Esposito T, Calabrò M, Rinaldi C, Robledo R, Varriale B, Sidoti A. FMO3 allelic variants in Sicilian and Sardinian populations: trimethylaminuria and absence of fish-like body odor. Gene 2013; 515:410 - 5; http://dx.doi.org/10.1016/j.gene.2012.12.047; PMID: 23266626
- Mackay RJ, McEntyre CJ, Henderson C, Lever M, George PM. Trimethylaminuria: causes and diagnosis of a socially distressing condition. Clin Biochem Rev 2011; 32:33 - 43; PMID: 21451776
- Tang WH, Wang Z, Levison BS, Koeth RA, Britt EB, Fu X, Wu Y, Hazen SL. Intestinal microbial metabolism of phosphatidylcholine and cardiovascular risk. N Engl J Med 2013; 368:1575 - 84; http://dx.doi.org/10.1056/NEJMoa1109400; PMID: 23614584
- Wolrath H, Ståhlbom B, Hallén A, Forsum U. Trimethylamine and trimethylamine oxide levels in normal women and women with bacterial vaginosis reflect a local metabolism in vaginal secretion as compared to urine. APMIS 2005; 113:513 - 6; http://dx.doi.org/10.1111/j.1600-0463.2005.apm_175.x; PMID: 16086821
- Neill AR, Grime DW, Dawson RM. Conversion of choline methyl groups through trimethylamine into methane in the rumen. Biochem J 1978; 170:529 - 35; PMID: 646798
- Hippe H, Caspari D, Fiebig K, Gottschalk G. Utilization of trimethylamine and other N-methyl compounds for growth and methane formation by Methanosarcina barkeri. Proc Natl Acad Sci U S A 1979; 76:494 - 8; http://dx.doi.org/10.1073/pnas.76.1.494; PMID: 284366
- Conway de Macario E, Macario AJ. Methanogenic archaea in health and disease: a novel paradigm of microbial pathogenesis. Int J Med Microbiol 2009; 299:99 - 108; http://dx.doi.org/10.1016/j.ijmm.2008.06.011; PMID: 18757236
- Mihajlovski A, Alric M, Brugère JF. A putative new order of methanogenic Archaea inhabiting the human gut, as revealed by molecular analyses of the mcrA gene. Res Microbiol 2008; 159:516 - 21; http://dx.doi.org/10.1016/j.resmic.2008.06.007; PMID: 18644435
- Mihajlovski A, Doré J, Levenez F, Alric M, Brugère J-F. Molecular evaluation of the human gut methanogenic archaeal microbiota reveals an age-associated increase of the diversity. Environ Microbiol Rep 2010; 2:272 - 80; http://dx.doi.org/10.1111/j.1758-2229.2009.00116.x; PMID: 23766078
- Dridi B, Fardeau ML, Ollivier B, Raoult D, Drancourt M. Methanomassiliicoccus luminyensis gen. nov., sp. nov., a methanogenic archaeon isolated from human faeces. Int J Syst Evol Microbiol 2012; 62:1902 - 7; http://dx.doi.org/10.1099/ijs.0.033712-0; PMID: 22859731
- Gorlas A, Robert C, Gimenez G, Drancourt M, Raoult D. Complete genome sequence of Methanomassiliicoccus luminyensis, the largest genome of a human-associated Archaea species. J Bacteriol 2012; 194:4745; http://dx.doi.org/10.1128/JB.00956-12; PMID: 22887657
- Borrel G, Harris HM, Tottey W, Mihajlovski A, Parisot N, Peyretaillade E, Peyret P, Gribaldo S, O’Toole PW, Brugère JF. Genome sequence of “Candidatus Methanomethylophilus alvus” Mx1201, a methanogenic archaeon from the human gut belonging to a seventh order of methanogens. J Bacteriol 2012; 194:6944 - 5; http://dx.doi.org/10.1128/JB.01867-12; PMID: 23209209
- Borrel G, Harris HM, Parisot N, Gaci N, Tottey W, Mihajlovski A, et al. Genome Sequence of “Candidatus Methanomassiliicoccus intestinalis” Issoire-Mx1, a Third Thermoplasmatales-Related Methanogenic Archaeon from Human Feces. Genome Announc 2013; 1.
- Krzycki JA. The direct genetic encoding of pyrrolysine. Curr Opin Microbiol 2005; 8:706 - 12; http://dx.doi.org/10.1016/j.mib.2005.10.009; PMID: 16256420
- Poulsen M, Schwab C, Jensen BB, Engberg RM, Spang A, Canibe N, Højberg O, Milinovich G, Fragner L, Schleper C, et al. Methylotrophic methanogenic Thermoplasmata implicated in reduced methane emissions from bovine rumen. Nat Commun 2013; 4:1428; http://dx.doi.org/10.1038/ncomms2432; PMID: 23385573
- Borrel G, O’Toole PW, Harris HM, Peyret P, Brugère JF, Gribaldo S. Phylogenomic data support a seventh order of methylotrophic methanogens and provide insights into the evolution of methanogenesis. Genome Biol Evol 2013; 5:1769 - 80; http://dx.doi.org/10.1093/gbe/evt128; PMID: 23985970
- Dridi B, Fardeau ML, Ollivier B, Raoult D, Drancourt M. The antimicrobial resistance pattern of cultured human methanogens reflects the unique phylogenetic position of archaea. J Antimicrob Chemother 2011; 66:2038 - 44; http://dx.doi.org/10.1093/jac/dkr251; PMID: 21680581
- Samuel BS, Gordon JI. A humanized gnotobiotic mouse model of host-archaeal-bacterial mutualism. Proc Natl Acad Sci U S A 2006; 103:10011 - 6; http://dx.doi.org/10.1073/pnas.0602187103; PMID: 16782812
- O’Keefe SJ, Chung D, Mahmoud N, Sepulveda AR, Manafe M, Arch J, Adada H, van der Merwe T. Why do African Americans get more colon cancer than Native Africans?. J Nutr 2007; 137:Suppl 175S - 82S; PMID: 17182822
- Nava GM, Carbonero F, Ou J, Benefiel AC, O’Keefe SJ, Gaskins HR. Hydrogenotrophic microbiota distinguish native Africans from African and European Americans. Environ Microbiol Rep 2012; 4:307 - 15; http://dx.doi.org/10.1111/j.1758-2229.2012.00334.x; PMID: 23760794
- Claesson MJ, Jeffery IB, Conde S, Power SE, O’Connor EM, Cusack S, Harris HM, Coakley M, Lakshminarayanan B, O’Sullivan O, et al. Gut microbiota composition correlates with diet and health in the elderly. Nature 2012; 488:178 - 84; http://dx.doi.org/10.1038/nature11319; PMID: 22797518
- Krätzer C, Carini P, Hovey R, Deppenmeier U. Transcriptional profiling of methyltransferase genes during growth of Methanosarcina mazei on trimethylamine. J Bacteriol 2009; 191:5108 - 15; http://dx.doi.org/10.1128/JB.00420-09; PMID: 19525341